Abstract
Three out of every 100 people in this world have some form of scoliosis. A doctor would suggest surgery if scoliosis is severe in certain conditions to prevent it from getting worse. The deformity of spine can be visualized well in 3D rather than in 2D as it is time-consuming to evaluate the degree of deformity. CATIA V5 is used to develop feature-based modeling. The angles of vertebrae orientation from biplanar X-rays are fed into the CATIA interface which forms the orientation of the 3D spine model. The feature-based model is modified using the morpho-realistic model for increasing the accuracy. The validation procedure for the feature-based model is divided into quantitative and qualitative analysis. In quantitative analysis, the One Sided Hausdorff Distance (OSHD), Average Surface Distance (ASD), Cobb angle and Axial Vertebral Rotation (AVR) metrics are obtained for inter-observer variability and intra-observer variability. In qualitative analysis, the model is projected along the frontal and lateral radiographs and compared with reference radiographs. The accuracy of the model can be estimated by the uncertainty in these parameters. The mean surface model reconstruction errors were found to be smaller than 1.5 mm in comparing cadaver Computed Tomography (CT) scan as well as for the 10 cases including inter-observer and intra-observer variability. The average differences for AVR and Cobb angle were less than 2 ̊. The modified feature-based 3D modeling allows for true 3D pre-operative planning which helps the doctor for better treatment with much less time.
Public interest statement
The human spine is made up of complex anatomical structures. Therefore, pathological deformations of the spine are difficult to diagnose using X-rays. The advanced 3D imaging modalities like CT and MRI have limitations on assessment of 3D spinal deformities as they are obtained in supine position. The supine position can change the actual deformation. Although CT can provide more information for the deformity assessment, the subjects are exposed to higher levels of radiation. Since the subjects with spinal deformities may have implants and corrective tools, MRI cannot be used. Hence, stereo-radiography is the most widely used technique for 3D reconstruction of the human spine. For this purpose, we have developed a feature-based stereo-radiographic method. This paper mainly focuses on the validation of 3D models obtained through this method. It includes both qualitative and quantitative methods with respect to the gold standard.
1. Introduction
Scoliosis is a three-dimensional deformity of the spine that occurs mainly in adolescent. There are several types of scoliosis the curve develops based on the cause and age. Curvature of the spine is the most common symptom of scoliosis. It also involves other factors like vertebral rotation, deformations, fusion, etc. The diagnosis would be more effective with the help of 3D model of the spine, as the deformity is three dimensional. In order to see the severity of deformation, any of the imaging techniques such as X-rays, computed tomography (CT) scans or MRI can be used for the diagnosis. In case of CT and MRI, the patient is made to lie on his back, which might alter the degree of curve and even the effect of radiation exposure can develop cancers when used repetitively. In both the cases, the patient needs to be in supine position during imaging and is expensive. X-ray imaging is mostly preferred by doctors for scoliosis. The patient data available for this technique are in standing position compared with other techniques and it avails only 2D data, but for effective diagnosis, the development of 3D model of the spine with the above data can be very useful. Mostly, the stereo-radiographic methods were used for 3D reconstruction of scoliotic spine (Andre, Dansereau, & Labelle, Citation1994).
Figure 5. An illustrated view of frontal and lateral radiographs (a) and the 3D model generated (b) analyzed for inter-observer variability in Meshlab (c)
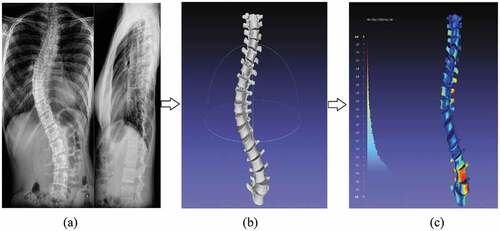
Features like stereo-corresponding points present in both the radiographs were used for 3D reconstruction. As this method makes use of expensive hardware and involves complex mathematical computations for reconstruction (Humbert, De Guise, Aubert, Godbout, & Skalli, Citation2009), this makes diagnosis difficult. Many other techniques have been tried to automate the 3D reconstruction of the spine from calibrated biplanar radiographs. These techniques tend to be complicated and need more knowledge in reading radiographs due to the anatomical complexity. Many attempts have been made for the reconstruction of 3D spine along with the human intervention; however, the time and accuracy required for reconstruction did not meet the clinical limitation (Pomero, Mitton, Laporte, de Guise, & Skalli, Citation2004).
A generic model is deformed with respect to stereo-radiographic data and developed to get the 3D spine model. CATIA V5 software is being used to develop a feature-based model (Kumar, Bhat, Nayak, & Hareesh, Citation2014). Necessary shape approximations are done ensuring sufficient visual agreement with actual vertebrae, due to irregularity in different cross-sections of various regions of vertebrae (Jones & Wilcox, Citation2008). The articular facets are assumed to have no lateral inclination and are modeled so as to provide a clear distinction between thoracic and lumbar vertebra. With reference to the feature-based model, a morpho-realistic spine model is obtained with the proposed method. To reduce work and modeling time, the method of assembly is semi-automated. This generic model can be deformed to get a subject-specific model. Through image processing techniques, the vertebral orientations are automatically extracted from biplanar radiographs. Equivalent 3D spine model is produced when these radiographs are given as input to the CATIA interface (Li & Wang, Citation2006).
The purpose of this paper is to validate the results obtained from the deformation. Validation is checked for both qualitative and quantitative approaches. In quantitative approach, the models obtained are compared for OSHD, ASD, Cobb angle and AVR metrics. And in qualitative approach, the model is compared by projecting it over the frontal and lateral radiographs.
2. Materials and methods
2.1. Feature-based spine model
The feature-based spine model is produced using the anthropometric data available from S.H. Tan et al. (Tan, Teo, & Chua, Citation2004) in a modeling software, CATIA V5 R19. This information relates to Asian (explicitly Chinese Singaporeans) specimen. Every vertebra has been modeled as an assembly of five major parts. They are cortical shell, cancellous core, posterior elements, upper and lower endplate (Humbert et al., Citation2009). A few parameters are assigned for defining the dimensions of these parts. Irregular cross-sectional shapes were displayed using rearranged shapes as proposed by Panjabi et al. (Dumas et al., Citation2008). The assembly of vertebra is shown in Figure .
Figure 1. Five major parts of a vertebra (a) Cortical shell, (b) Cancellous core, (c) posterior elements, (d) upper endplate, (e) lower endplate and (f) Assembly of vertebra (L1)
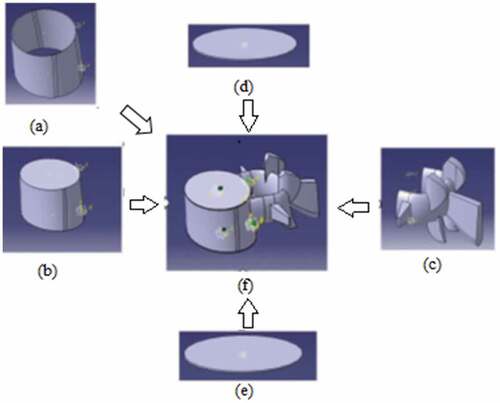
For the assembly of both thoracic and lumbar vertebrae, same procedure is followed. Initially, the vertebrae are assembled for which the articular facets are in perfect contrast to give the normal spine. Next, for obtaining 3D model of a scoliotic spine through a semi-automated process, an attempt is made to use the developed feature-based model. The vertebrae are assembled using “connectors” and any endplate can be called in and used as reference with respect to which the angles of other vertebrae are set. The generic spine model obtained from feature-based 3D modeling is shown in Figure .
To maintain intervertebral spacing, the connectors pass through the vertebrae endplate centers and extend on either end. These extensions are kept to only on one side for T1 and L5 vertebrae. Even after the change in orientation of vertebrae, the connectors retain continuity of spine. This procedure is explained in detail in our previous work (Kumar & Shetty et al., Citation2014).
2.2. Modified feature-based spine model
The proposed method makes use of morpho-realistic 3D spine model replacing the feature-based 3D model. This we call as Modified feature-based spine model. The biomedical model of the spine is developed from a cadaveric spine using the method 3D biomedical modeling (De Guise & Martel, Citation1988). The cadaveric spine, which is also used as X-ray phantom, is used for this purpose. The CT scan slices of 1 mm thickness are obtained from T1 to L5 vertebrae of the cadaveric spine in DICOM format with dimension 512 × 512 and 12-bit resolution. For each vertebra, the segmented slices are stacked one above the other, and volume rendering technique is used for the 3D reconstruction. The constrained Delaunay tetrahedralization is used to obtain the surface meshes (Boas & Fang, Citation2009). The vertebrae are stacked one above the other with normal kyphotic and lordotic angles to obtain the generic model as shown in Figure . This procedure is explained in detail in our previous work (Kumar, Nayak, & Hareesha, Citation2014).
To feed the angles obtained from uncelebrated biplanar radiographs, an interface is developed using a visual basic application in CATIA (U.S. Patent 3069654, Citation1962). Image processing techniques are used to obtain the angles. Vertebral body contours were extracted from enhanced radiographs using GVF-snake algorithm (Weisenberger, Citation0000). The vertebral orientations are computed using Hough transform after morphological operations are achieved (Kass, Witkin, & Terzopoulos, Citation1998). The interface considers the angles between axes of upper endplates of all the vertebrae and the axes of reference endplate. Change in orientation occurs depending on the axes that run parallel to frontal and lateral views.
2.3. Quantitative analysis
Morpho-realistic 3D spine model of CT data scans of actual cadaveric vertebrae generated is shown in Figure . The reconstruction accuracy is validated by considering surface models segmented from the CT data as the ground truth. The standard model is constructed from CT scan slices of a cadaveric vertebra using the open source software SLICER 4.10. The CT scan of the same cadaveric vertebrae is obtained thrice to compare the accuracy. Corresponding 3D surface models were first rigidly aligned with the ground truth models. After alignment, the reconstructed models were cropped to have the same lengths as the ground truth models. Finally, the distance from each point on the reconstructed models to the associated ground truth models is computed. The segmented 3D model is compared with the morpho-realistic 3D spine model in Meshlab.
Figure 4. An illustrative view of the CT scan (a) and the segmented 3D model (b) compared in Meshlab with the morpho-realistic 3D model of Cadaver (c)
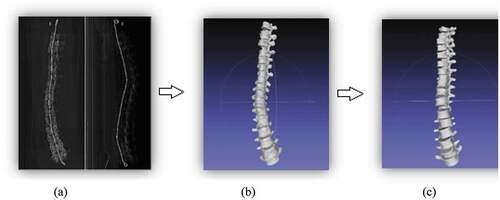
Metrics like ASD was computed as the average of the distances of all the points on a reconstructed surface model to the associated ground truth model, and the OSHD was computed as the maximum of the distances of all the points on a reconstructed surface model to the associated ground truth model. Cobb angle and AVR were measured to find the difference in the angle between the segmented 3D model and morphorealistic 3D spine model. These metrics were used to evaluate the 2D–3D reconstruction accuracy.
2.4. Inter-observer variability and intra-observer variability
The severity of scoliosis is routinely evaluated in accordance with the X-ray pictures in anterio-posterior and lateral projection. The X-ray images of 10 cases were used to study the preoperative planning. Two radiographs, i.e. frontal and lateral images for each patient, are considered. The 3D model generated is compared in two different ways, the Inter-observer variability and intra-observer variability. In inter-observer variability, the model is generated from two or three different experts, and in intra-observer variability, the model is generated by the same expert is considered while comparing the four metrics.
The Hausdorff distance computed in MeshLab uses a sampling approach by taking a set of points over a mesh A, and for each point x on A, it searches the closest point y on the other mesh B. Hausdorff Distance is defined as the greatest of all the distances from a point in one set to the closest point in the other set. A color filter is applied by vertex quality, to visualize the results. The range of colors is based on the maximum and minimum calculated distances. Points with values below the minimum will be colored blue, values above the maximum will be colored red and values in between are interpolated. A histogram reporting the error distribution is also visualized on the left of the Meshlab window. As the distance between the two points is smaller, the similarities between them are greater.
The other parameters such as the AVR and Cobb angle obtained from 2D radiographs are compared with results obtained from 3D models. According to the plane of geometry, the Cobb angle is the sum of upper and lower end vertebra tilt angles. To reduce deviation of the observer, the angle data of each patient were measured by two different experts and then the average of differing value was taken as the final result.
2.5. Qualitative analysis
In qualitative approach, the 3D model generated using proposed method is back projected on the corresponding radiographs to observe the similarity. A close similarity can be observed between the spinal curves. Similar procedure is repeated for all the 10 subject cases. In Figure , the model is back projected on both the frontal and lateral radiographs. The models are properly superimposed which shows a close match of the features in the given radiographs.
3. Results
The mean and the standard error of Hausdorff distance and average surface distance indicate the quality of surface reconstruction. The OSHD of 1.2 ± 0.03 mm and the mean ASD of 0.17 ± 0.01 mm were observed for 3D model of the phantom and the CT scan model. The result was compared for the CT scan of cadaver taken from two different hospitals. This is shown in Figure .
Table presents the one-sided Hausdorff distance for 10 subject cases considering inter-observer variability. Figure 5 shows an example for inter-observer variability.
Table 1. Inter-observer variability on measure of OSHD
A mean OSHD of 1.3 ± 0.03 mm was found for the 3D model that was generated by different experts. A mean ASD of 0.18 ± 0.01 mm was found for the 3D model generated for inter-observer variability as shown in Table 2. Similarly, the intra-observer variability of OSHD and ASD is computed. The model generated by the same expert multiple times is compared. A mean OSHD of 0.74 ± 0.1 mm and a mean ASD of 0.08 ± 0.01 mm was found in 10 subjects is shown in Tables and , respectively.
Table 2. Inter-observer variability on measure of ASD
Table 3. Intra-observer variability on measure of OSHD
Table 4. Intra-observer variability measure on ASD
Table shows the difference in Cobb angle and AVR measured by two different experts. An average difference of 1.9̊ ± 1.04̊ was found for axial vertebral rotation and an average difference of 1.6̊ ± 0.55̊ and 1.0̊ ± 0.68̊ was, respectively, found for Cobb angle 1 and Cobb angle 2.
Table 5. Standard deviation of Cobb angle and AVR obtained from measuring the difference
4. Discussion and conclusion
The objective of the present study is to evaluate the accuracy of the morpho-realistic spine model, which can derive a 3D patient-specific surface model of the spine from 2D X-rays. Proposed method uses the interface to obtain a close approximation of actual scoliotic spine from a developed feature-based spine model. The model obtained gives 3D data of the patient in a standing position. And the proposed method for generating 3D model is less expensive.
In case of quantitative analysis, the inter-observer variability and intra-observer variability for 10 scoliotic-affected patients are validated. The model obtained from a CT scan of the cadaver is validated by considering the segmented model of CT scan as ground truth and measured OSHD and ASD. The mean surface model reconstruction errors were around 1.2 mm and 0.17 mm, respectively, with reference to phantom. The Cobb angle and AVR measured compared by two different experts had very close values. An average difference of AVR was found to be 1.9̊, and Cobb angle-1 and Cobb angle-2 was 1.6̊ and 1̊, respectively. In qualitative analysis, the 3D models are projected over biplanar radiographs, which showed the similarity in both the lateral and frontal X-rays.
In summary, 3D model obtained from CT scan of cadaver and 10 such subjects included in the preoperative planning validation study evaluated the surface model reconstruction accuracy. The mean surface model reconstruction errors were found to be smaller than 1.5 mm in comparing cadaver CT scan as well as for the 10 cases. The average differences for AVR and Cobb angle were less than 2̊. Thus, the modified feature-based model obtained from morpho-realistic vertebrae is closely related to the scoliotic spine in the radiograph.
Cover image
Source: Author.
Acknowledgements
This research is partially supported by the Kasturba Medical College (KMC), Manipal, in providing the dataset of 10 scoliosis patients.
Additional information
Funding
Notes on contributors
Sampath Kumar
Dr. Sampath Kumar, Dr. Hareesha K.S. and Soujanya Shetty
Our main focus is on 3D modeling of human body parts. We are also into augmented reality and virtual reality related to the health care domain. The current project is 3D reconstruction of the human spine using two X-rays. This idea can also be extended to 3D reconstruction of teeth and jaw using X-rays. The product developed using this approach will be economical at the same time less radiation hazardous.
References
- Andre, B., Dansereau, J., & Labelle, H. (1994). Optimized vertical stereo base radiographic setup for the clinical three-dimensional reconstruction of the human spine. Journal of Biomechanics, 27(8), 1023–12. doi:10.1016/0021-9290(94)90219-4
- Boas, K., & Fang, Q. (2009). Tetrahedral Mesh generation from volumetric binary and gray scale images. IEEE Transaction, 9, 1142–1145.
- De Guise, J. A., & Martel, Y. (1988). 3D-Biomedical modeling: Merging image processing and computer aided design. IEEE engineering in medicine & biology society, 10th Annual International Conference.
- Dumas, R., Blanchard, B., Carlier, R., de Loubresse, C. G., Le Huec, J. C., Marty, C., … Vital, J. M. (2008, January). A semi-automated method using interpolation and optimization for the 3-D reconstruction of the spine from bi-planar radiography. Medical & Biological Engineering & Computing, 46, 85–92. doi:10.1007/s11517-007-0253-3
- Hough, P. V. C. (1962, December 18). Method and means for recognizing complex patterns. U.S. Patent 3,069,654.
- Humbert, L., De Guise, J. A., Aubert, B., Godbout, B., & Skalli, W. (2009). 3-D reconstruction of the spine from biplanar x-rays using parametric model based on transversal and longitudinal inferences. Medical Engineering and Physics. Press Article. doi:10.1016/j.medengphy.2009.01.003
- Jones, A. C., & Wilcox, R. K. (2008). Finite element analysis of the spine: Towards a framework of verification, validation and sensitivity analysis. Medical Engineering and Physics, 30, 1287–1304. doi:10.1016/j.medengphy.2008.09.006
- Kass, M., Witkin, A., & Terzopoulos, D. (1998). Snakes: Active contour models. International Journal of Computer Vision, 1, 321–331. doi:10.1007/BF00133570
- Kumar, S., Nayak, K. P., & Hareesha, K. S. (2014). 3D Biomedical modelling of human vertebrae and its deformation. In J. Stephen & R. K. Bhatia (Eds.), Advances in information technology and power electronics (pp. 325–336). India: McGraw-Hill publishing.
- Kumar, S., Shetty, V., Bhat, C., Prabhakar Nayak, K., Hareesha, K. S. (2014). Feature based modelling of lumbar and thoracic vertebrae for 3D reconstruction of human spine. International Conference on Computational Methods in Engineering and Health Sciences. doi:10.13140/RG.2.1.2710.2564
- Kumar, V. S., Bhat, C., Nayak, K. P., & Hareesh, K. S. (2014, December 17–19). Feature based modelling of lumbar and thoracic vertebrae for 3-D reconstruction of spine. Proceedings of International Conference on Computational Methods in Engineering and Health Sciences, Manipal.
- Li, H., & Wang, Z. (2006). Intervertebral disc biomechanical analysis using the finite element modeling based on medical images. Computerized Medical Imaging and Graphics, 30, 363–370. doi:10.1016/j.compmedimag.2006.09.004
- Pomero, V., Mitton, D., Laporte, S., de Guise, J. A., & Skalli, W. (2004, March). Fast accurate stereo-radiographic 3-D reconstruction of the spine using a combined geometric and statistic model. Clinical Biomechanics, 19, 240–247. doi:10.1016/j.clinbiomech.2003.11.014
- Tan, S. H., Teo, E. C., & Chua, H. C. (2004). Quantitative three-dimensional anatomy of cervical, thoracic and lumbar vertebrae of Chinese Singaporeans. European Spine Journal, 13, 137–146. doi:10.1007/s00586-003-0586-z
- Weisenberger, N. VB Scripting for CATIA V5. Expanded eBook Edition, 2012.