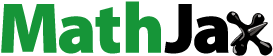
Abstract
There are over 50,000 telecommunication base transceiver stations (BTS) operating on conventional diesel generators across Nigeria, giving rise to a high operational cost and emission of Greenhouse gases which can be minimized by the adoption of greener energy generation. Presented in this study, is an analysis of the techno-economic and emission impact of a stand-alone hybrid energy system designed for base transceiver stations (BTS) in the Nigerian telecom industry. Using various performance criteria the feasibility of adopting hybrid photovoltaic-diesel generator and battery (PV/DG/Battery) system is analyzed under two different diesel pump price regimes. In all, it is observed that all BTS locations across the six geopolitical zones could adopt PV/battery/DG hybrid renewable energy system in place of the diesel generator (DG) which is, presently, the main technology being used across the BTSs. Besides its economic advantages over the diesel generator, HRES configurations also performed better with respect to diesel consumption and carbon dioxide (CO2) emission.
PUBLIC INTEREST STATEMENT
The addition of renewable energy sources to the energy mix is gradually gaining momentum in developing economies. This is due to a reduction in emissions as well as energy security. One of the sectors that can take the delivery of the multiple benefits of acquiring renewable energy technologies is the telecommunication sector. In order to prepare a sound framework for the adoption of a Photovoltaic system for powering telecommunication base stations in sub-Sahara Africa-specifically Nigeria, this study explores the feasibility (technical, environmental and economical) of including photovoltaic in the energy mix for supplying a typical base transceiver station. A sensitivity analysis at two diesel price regime is also presented. These analyses will help in building a robust framework for ensuring a comprehensive adoption of renewable energy, for powering telecommunication base transceiver stations.
1. Introduction
Telecommunication is a major driving force of national development. There are several mobile communication companies with great potentials of meeting the teeming demand but the fuel consumption and its associated cost is scary. The mobile telecommunication industry in Nigeria has witnessed significant growth and has contributed largely to the growth of the country in the Gross Domestic Product (GDP) (Ekwujuru & Ofili, Citation2016). The sub-sector which commenced economic activities in 2001 has contributed remarkably to the creation of job opportunities across the country. Since it replaced the expensive and limited traditional fixed lines telephony system, it has provided Nigerians both in urban and rural settlements at all income levels an effective, reliable and cheaper means of communication. Presently, Nigeria has the largest mobile communication industry in Africa with a teledensity of about 103.91 present in 2015 (Adepetun, Citation2015). From available data, the telecommunication sector has grown its investment to over $32 billion in 2014 from $500million in 2011 (Adepetun, Citation2015). This is due to the swift rise in the number of subscribers across the country.
Though mobile telecommunication has experienced significant growth in acceptability by the teeming population, the lack of reliable supply of power for the base transceiver station (BTS) could impede the realization of the inherent benefits of its service provision. In line with this, the mobile telecommunication providers resolve to countrywide supplementary energy sources such as diesel generators for mitigating unreliable power supply to BTSs. The utilization of conventional diesel generators results in economic, logistic as well as environmental challenges (Olatomiwa, Blanchard, Mekhilef, & Akinyele, Citation2018). According to Adegoke and Babalola (Citation2011), the operation and maintenance of diesel generators account for 78% of the total cost of operations in the telecom industry. This is equivalent to about 35% of the total cost of ownership for sites of base transceiver stations (BTS). Furthermore, an estimated N8.4 billion ($16.8 million) is spent monthly on fossil fuel to supply electricity to over 25,000 BTS stations nationwide (Ihekwoaba, Citation2017). This is partly due to epileptic electricity supply by the utility companies while on the other hand the inaccessibility of grid to BTSs at remote locations. Consequently, this may lead to subscribers paying more to maintain their Global System of Mobile Communication (GSM) lines. Likewise, there are other challenges that are attendant in the deployment of diesel-fired generators which include; adulterated fuel, security challenges during haulage, fuel theft, frequent hike in fuel prices and the emission of greenhouse gases (GHGs) which causes global warming and health-related sicknesses and diseases (Akinyele, Belikov, & Levron, Citation2018; Ogunjuyigbe, Citation2016).
Utilization of renewable energy (RE) for powering electric loads can significantly reduce CO2 emission, as well as dependence on fossil fuel (Adaramola, Quansah, Agelin-chaab, & Paul, Citation2017; Adeyeye, Tsado, & Olatomiwa, Citation2018; Krishan and Sathans, Citation2018). Furthermore, it has the advantage of being clean/green, inexhaustible and complementary (Akinyele, Citation2017a; Babatunde, Akinyele, Akinbulire, & Oluseyi, Citation2018; Rashid, Rana, Shezan, Karim, & Anower, Citation2017; Shahzad et al., Citation2017). It is also a cost-effective way of electrifying rural communities in developing countries considering the high cost of grid extension. Consequently, hybrid renewable energy systems (HRES) have been seen as affordable, reliable and cost-effective in rural electrification especially in places where grid extension is either difficult or economically unviable (Samson, Babatunde, & Denwigwe, Citation2019). Application of RE to power GSM base station will potentially minimize the cost spent on fossil fuel and consequently result in greener production. Reduction in tariff on the part of subscribers and significant operational cost savings on the part of service providers can also be achieved through the adoption of HRES. In addition, significant CO2 emission reduction can be achieved because of less dependency on fossil fuel most especially diesel. Nigeria is endowed with abundant sources of renewable energy as presented in Table (Nwulua & Agboolab, Citation2011). Hence the possibility of utilizing HRES in powering telecom industry base stations (BTSs) across Nigeria is high. Sources of Renewable energy such as solar, wind, and biomass can be adopted for the telecom industry in Nigeria. Previous studies have evaluated the cost-effectiveness of deploying hybrid renewable energy systems (HRES), especially in residential applications in remote communities (Dusabe, Munda, & Jimoh, Citation2007; Adaramola, Paul, & Oyewola, Citation2014; Akinbulire, Oluseyi, & Babatunde, Citation2014; Ayodele, Citation2014; Ohunakin, Adaramola, Oyewola, & Fagbenle, Citation2014; Amutha & Rajini, Citation2015; Olatomiwa, Mekhilef, Huda, & Ohunakin, Citation2015a; S. Ogunjuyigbe, Citation2016; Leon, Shoeb, Rahman, Ahmed, & Islam, Citation2016; Mazzola, Astolfi, & Macchi, Citation2016; Ayodele, Ogunjuyigbe, & Amusan, Citation2018).
Table 1. Nigeria’s renewable resources (Akinyele et al., Citation2019)
Akinbulire et al. (Citation2014), presented a demand-side management approach to the sizing of hybrid energy systems for a typical rural community. Results show that 100% of the load could be met using the PV/battery configuration. Feasibility study of powering residential rural communities using hybrid renewables has been investigated (Olatomiwa et al., Citation2015a). The study was conducted in the six geopolitical zones of Nigeria. It was discovered that the most viable architecture is the PV/diesel/battery configuration. As a contribution to the rural community developmental programme, Dusabe, Munda, and Jimoh (Citation2009) investigated the status as well as basic characteristics for evaluating and sizing the standalone PV system in Rwanda. The study made a comparison between Hybrid Optimization of Multiple Electric Renewables (HOMER) software and the numerical method of dimensioning the system. Results show the superiority of HOMER software over the numerical method. In a similar manner, Dusabe et al. (Citation2007), examined the effect of energy efficiency involved in the operation procedure for the sizing and optimization of the off-grid hybrid PV/DG (photovoltaic/diesel generator) system. The case study was aimed at supplying electricity to a rural community comprising of 30 households in South Africa. The result shows the possibility of eliminating the diesel generator from the system, without significantly increasing the cost of energy, thereby achieving less CO2 emission. Ayodele (Citation2014) in a study, proposed the use of a hybrid renewable energy system for meeting the energy demand of an off-grid remote community. The study identified a wind/solar/diesel hybrid system as the most viable configuration due to the availability of resources at the location. Results indicate that renewable energy resource contributed 74% of the total energy production. Leon et al. (Citation2016) presented the economic viability and design of an autonomous hybrid energy system for a remote community in Bangladesh using RETscreen software. The simulation result shows PV/Biogas/diesel/battery configuration as the optimal system architecture. Mazzola et al. (Citation2016) examined the techno-economic feasibility of hybrid micro-grid in India designed to serve a population of 2000 inhabitants in a typical rural community of Bihar. The potential of wood biomass for generating electricity was analyzed. Results show that renewable energy sources have great potentials for meeting the energy needs of rural communities with a reduction in the cost of energy as compared with the operation of diesel generators. In another study, the environmental and techno-economic performance of various hybrid power systems for powering telecommunication site was studied by Amutha and Rajini (Citation2015). Through simulation with HOMER software, the study suggests a system consisting of PV/Wind/Battery and PV/Wind/Battery/Fuel cell as the most viable both economically and environmentally.
The design, cost and operational analysis benefits of HRES for BTS stations have also been carried out in some other studies. A techno-economic evaluation of a standalone hybrid energy system for a BTS of Telecom Company in a single location in Nigeria is presented by Ogunjuyigbe and Ayodele (Citation2016). The BTS under consideration operated an average load of 87.2 kWh/day and 47.6 kWh/day for AC and DC load respectively. Using HOMER software as a simulation tool, the optimal hybrid energy system was estimated for a 20-year period. The result indicated that the PV/diesel/battery offers the best option with respect to the net present cost. With the objective of ensuring a stable and reliable cost-effective energy solution for BTS, electrification, Okundamiya, Emagbetere, and Ogujor (Citation2014), presented a comprehensive techno-economic viability of deploying HRES in Nigeria. With the aid of MATLAB®, results revealed that HRES is environmentally friendly, capable of increasing overall energy thereby improving the quality of BTS services. Faruk, Ayeni, Muhammad, Abdulkarim, and Moses (Citation2012) in a study reported that the optimal hybrid power system for off-grid telecom cell sites is a solar photovoltaic system hybridized with both diesel generator and battery. Furthermore, it was discovered that over a 15-year period, reliability figures of the hybrid system were much higher than 80% as compared with the 15% of generator only. Goel and Ali (Citation2014) examined the possibility of deploying a PV/wind/diesel/battery hybrid system for telecom towers in a remote location in India. From the results, the PV/wind/diesel/battery hybrid system architecture returned the minimum cost of energy (0.552 $/kWh) at 83 kWh/day while at 22.7 kWh/day a COE of 0.787 $/kWh was recorded. Nema, Nema, and Rangnekar (Citation2010) proposed a suitable alternative solution to unreliable grid power for BTS sites in India. The study specified a hybrid combination of PV/wind/diesel configuration as the best in comparison to the conventional diesel-only generator. If implemented, the proposed system is expected to save between 70-80% fuel costs over the conventional diesel generator. Olatomiwa, Mekhilef, Huda, and Sanusi (Citation2015b) illustrated the optimization and sizing of a PV/wind/diesel generator/battery hybrid system targeted to supply a mobile BTS located in a remote area in Nigeria. Findings reveal that the PV/diesel generator/battery performed better than all other configurations in terms of economics and sustainability.
2. Aim of the study
Due to the poor state of electricity supply in Nigeria, the Nigeria telecommunication operators’ power over 25,000 BTS stations spread across the six geopolitical zones with more than 50,000 generating set of varying capacities (Adepetun, Citation2015). Meanwhile, ideally, the telecommunication operators will require about 80,000 BTS to meet the growing appetite of its consumers in the industry in Nigeria (Adepetun, Citation2015). The addition of more BTS to the industry will also increase the number of diesel generator sets that will be deployed. This means the cost of operating and maintaining the generators set which presently stands at $589.5 million (N 214.3 billion) will be more than double the present cost. Apart from this, the pollution level which causes climate change and emission-related health challenges may likely be on the rise. Replacement of the present diesel-only generator system with a hybrid renewable alternative may be a solution for the aforementioned challenges. This is likely to reduce the skyrocketing cost for running the BTS, as well as the carbon footprint. Although some studies investigated the use of HRES for BTS stations in Nigeria (Faruk et al., Citation2012; Ogunjuyigbe & Ayodele, Citation2016; Olatomiwa, Mekhilef, & Huda, Citation2014; Olatomiwa et al., Citation2015b), they were only able to conduct the techno-economic analysis of its utilization at a single location in the country. Interestingly, results from these studies indicate the optimal system as consisting of PV/Diesel/battery architecture as the best. It is observed that the diesel generator still remains an integral part of hybrid energy system BTS applications. It is worth to note that it is important to add diesel generator so as to serve as a backup in times of system failure or maintenance of the RE source since telecommunication industries cannot afford the equipment going offline for a long time. Therefore, the use of diesel fuel is inevitable. Little is known about the impact of fluctuating diesel pump prices on the implementation of HRES for BTSs as little is found in the existing literature. Fluctuation in fuel prices coupled with the unreliable grid system remains a major challenge in the telecom industry. We consider two fuel price regimes in this paper.
This paper, therefore, evaluates the most techno-economically and environmentally viable solution for providing electricity to off-grid BTS originally powered by a diesel generator set across the six geopolitical zones in Nigeria under varying diesel pump prices. It further determines the most viable location for the HRES implementation in Nigeria. The study compares the various hybrid system configuration using indices such as total net present cost (TNPC), levelised cost of energy (LCOE), the renewable fraction (RF), and emission savings. This will provide more understanding of the operational configuration requirements, environmental impact as well as cost requirement for the different geopolitical zones in Nigeria. Table gives a summary of RE potentials in Nigeria (Akinyele et al., Citation2019).
3. Material and method
This section provides the methodology on which this study is based (see Figure ). The viability of adopting a PV-diesel generator system for powering BTS was tested across the six geopolitical zones in Nigeria. A site was selected for each location- these sites are representative of the climatic conditions for each geopolitical zone. First, the metrological data, energy demand, technical details, as well as the economic details of system components were obtained. The search space for each component is also determined. These serve as input into the simulation software. The software then performs the simulation and optimization to give an output that is ranked based on the least TNPC. In order to observe the behavior of change in fuel price, the last part of the analysis presents a sensitivity analysis.
3.1. Hybrid systems architecture
The intermittent nature of renewable energy sources (RES) due to fluctuation in varying climatic conditions requires that the RES should be developed as a hybridized system. To determine the most efficient hybrid RES system configuration, an environmental and techno-economic assessment of such configuration must be determined. The proposed topology for the hybrid energy system is as shown in Figure . It consists of PV, battery, converter, diesel generator, AC bus, DC bus and loads (AC and DC).
3.1.1. PV module output power
The PV panel’s output varies according to the time of the day and season. This is largely due to the variations in meteorological data at these periods. To adequately ascertain the size of the PV panel that will serve the BTSs at the proposed locations, it is important to understand the performance of the PV module. EquationEquation 1(1)
(1) can be used to estimate the power output (Pspv) of the PV module (Akinyele, Citation2017b).
Where is the rated power of the PV module,
is the derating factor,
is the solar radiation on an inclined surface (W/m2),
is the solar radiation at existing standard test conditions based on the manufacturer’s specification,
is the power temperature coefficient of the panel,
is the module cell temperature and
is the temperature at standard test conditions based on the manufacturer’s specification.
Where is the ambient temperature (°C),
is the normal cell temperature (°C), and
3.1.2. Diesel generator
Losing the BTS load can have a negative technical and economic implication for the telecommunication company. Since the output of the PV panel is intermittent in nature, it is necessary to have a backup source to support the load. This can be achieved using the diesel generator and the battery bank. The fuel consumption model for a diesel generator is expressed in EquationEquation 3(3)
(3) (Akinyele, Citation2017b).
Where the operating power of the generator is , the rating of the generator is
, X is the fuel curve slope and Y fuel curve intercept and
is the fuel consumption.
3.1.3. Battery bank storage
The battery bank is used to complement the PV panel during intermittency and at periods when the PV panel cannot supply power (raining period, night, etc). The battery stores energy from renewable sources and the diesel generator. This energy is used during times of power insufficiency. EquationEquation (4)(4)
(4) is used to calculate a system storage capacity (Bsc) (Babatunde et al., Citation2018):
Where ,
,
, and
are the demands of the load, days of battery autonomy, round-trip efficiency of the battery, depth of battery discharge and the nominal voltage of the system, respectively.
EquationEquations (5)(5)
(5) and (Equation6
(6)
(6) ) are used to address a battery system over-charge or over-discharge problem.
Where ,
,
,
denote minimum battery size, the battery size, maximum battery size, maximum depth of discharge and the minimum state of charge at a given time, respectively.
EquationEquation (7)(7)
(7) is used to constraint the battery state of charge (Akinyele, Citation2017b).
3.2. System modelling
To model the economic viability of HRES, the climatic data for the respective regions under consideration is needed and the load to be supplied is required. These will enable adequate sizing of solar panels, converters, batteries as well as the capacity of diesel generator. Consequently, the feasibility of meeting load demand will be easily determined. For this case study, HOMER software (a micropower optimization model), which is used for simplifying the task of evaluating designs of both an off-grid system and a grid-connected power system for a variety of applications, is deployed. HOMER is a software tool that displays the interaction of various renewables and hybrid systems with respect to the demand for energy. Based on the installation cost, replacement cost, operational cost, fuel cost, interest rate, and some other constraints; HOMER simulates and returns the system with the lowest TNPC as the best.
3.3. Case study
Nigeria, a West African country has a total of 923,768 km2 with land being 910.768 km2 and water occupying 13,000 km2. The landmass is categorized into six geopolitical zones namely, namely the south-east, south-west, the south-south, the north-east, the north-central and the north-west. Each of the zones has distinct meteorological attributes at different seasons of the year. Thus, implementing the HRES in each of these zones becomes a veritable and representative means of ensuring equitable assessment of the effectiveness of the research. This paper evaluates the economic and technical viability of providing telecom GSM base stations with hybrid electricity in each of the geopolitical zones in Nigeria. Details of the considered locations is displayed in Table .
Table 2. Location of study
3.4. Modelling data
3.4.1. Designing and modeling with HOMER
Hybrid Optimization Model for Electric Renewables (HOMER) is a software for optimization developed by the NREL (National Renewable Energy Laboratory) which performs a relative economic analysis on the generation configurations of proposed and actual energy systems is used in this research. HOMER models the hybrid energy system performance and its life-cycle cost (Lambert, Gilman, & Lilienthal, Citation2006). This is the total cost of system installation and operation over its lifetime and this is adapted for this study. With HOMER, various energy alternatives can be evaluated to obtain the most suitable choice based on economic, environmental and technical benefits. Fluctuations based on input parameters can also be modeled and quantified with HOMER. Therefore, HOMER is proficient for three basic functional duties namely: optimization, simulation, and sensitivity analysis. During the optimization process, HOMER evaluates various arrangements of energy system to determine the one that will adequately meet all the specified constraints (technical, economic and environmental) at minimum total net present cost. In analyzing the uncertainty of the input parameters, HOMER conducts an analysis of sensitivity. To achieve this, multiple optimizations are carried out under the specified range of input parameters for the evaluation of fluctuations in the model inputs. Optimization technique determines the optimal value of the variables which the system designer can control, such as the combination of components that the system is made of and their numbers. Sensitivity analysis facilitates the determination of the effects of uncertainty or changes in the input variables for future purposes. In this case, it is assumed that the system designer has limited or no control over the input. Such circumstance includes a change in solar radiation or average wind speed which may be due to climatic change or increase in fuel price. HOMER requires three categories of data as input. These include the metrological data, energy demand/load data, and the system component data. Details on the methodology adopted by HOMER are available in the literature (Akinbulire et al., Citation2014; Akinyele, Citation2017b; Bahramara, Moghaddam, & Haghifam, Citation2016; HOMER, Citation2016; Krishan and Sathans, Citation2018; Mandal, Yasmin, Sarker, & Beg, Citation2017).
3.4.2. Metrological data
In this feasibility study, a major city is chosen from each zone earlier discussed. This is selected based on the population. The weather data of each city chosen is a representative sample of other cities in the zone. Geographical details of the area under consideration are given in Table . As seen in Table , coordinate, solar irradiation for each city were extracted from NASA’s website (NASA, Citation2018).
Table 3. Solar irradiation for the understudied sites
3.4.3. Load data
A typical base transceiver station (BTS) load can be obtained based on the rated capacity of all alternating current (AC) and direct current (DC) loads available at such station. The multiplication of the capacity and the interval of usage of all equipment over 24 hours give the load pattern for a typical BTS station. All electrical equipment in the station are utilized at various stages and times throughout the day. BTS equipment are basically powered by DC supply, hence the need for a DC source. The air conditioning units and the lighting system requires AC supplies. The consumption details of a typical BTS station are shown in Table . The load profile is displayed in Figure .
Table 4. Typical power consumption for a GSM base transmission station
The average daily AC energy demand is estimated as 86 kWh/day, while the peak AC power is 3.58 kW. The DC daily average energy demand is 84kWh/day while the peak power is 4.5kW. HOMER, however, allows for randomizing and correlating the load profile to extrapolate the load profile over a year period. The random variability inputs allow for the addition of randomness to the load data (this makes the load data more practical).
3.4.4. Component data
This subsection is dedicated to discussing all the assumptions made with regard to the individual system components. These include costs (in USD), sizes and some technical details. The following are the assumption:
It is assumed that the initial investment cost of getting a 5.5kW diesel generator is $1240 while it will cost $1200 to replace it after a life span of 15,000 hours. It is also assumed that the hourly operating and maintenance cost is $0.05/hour while the minimum load factor is 30%.
Based on the assumption that various internal and external factors will make the pump price of diesel to fluctuate over the life span of the project, two fuel pump prices were considered ($0.7/L and $0.96/L)
It is assumed that the initial investment cost of a kilowatt PV panel is $4250 while it will cost $4200 to replace it after its life span of 20 years. It is also assumed that the yearly maintenance cost is $10/year. The derating factor is taken as 0.9, ground reflectance as 20% with no device to track the sun.
Each battery is assumed to have a capacity of 1,900Ah, with a nominal voltage of 4V. The initial cost of a unit is $2152. Costs of maintenance and replacement were placed at $2100 and $11/year respectively. A string of batteries contained 8 batteries and energy over a lifetime is estimated as 9645 kWh. The battery is not allowed to discharge below 40%.
The capital cost of purchasing a 6 kW converter is assumed to be $3731 while the cost of replacing it is taken as $3700. Other interpolations are based on this assumption. It will require $10 to maintain the converter on an annual basis. The efficiency of the inverter and rectifier is placed at 90% and 85% respectively. The converter is expected to operate for 15 years before it is replaced.
The factor of capacity shortage is set to 0% of the maximum hourly load. The minimum renewable fraction is 70%. This is an indication that all load must be powered at all times and the RE penetration must be high.
An annual real interest rate of 12% is assumed for the entire system.
3.5. Performance criteria
In this study, the performance indices for choosing the most feasible HRES are based on the Levelised Cost of Energy (LCOE), total Net Present Cost (TNPC), Renewable Fraction (RF) and the carbon emission. These are discussed in sub-sections 3.51 to 3.54.
3.5.1. The total net present cost
The total net present cost (TNPC) is the present worth of the expenses incurred on a system for the duration of its lifespan minus the present value of all the income that it receives over its lifespan. For this case study, such costs include the costs of replacement, capital costs, maintenance and operation costs, and fuel costs over the project lifespan. Revenues include the salvage value of the system. The system configuration with the least TNPC is returned as the most economically feasible project. The TNPC can be estimated using EquationEquation (8)8
8 .
Where is the total annualized cost,
is the capital recovery factor,
is the project lifespan and
is the nominal interest rate in % that allows for inflation.
3.5.2. Levelised cost of energy
Levelised cost of energy (LCOE) is the average cost per kWh of valuable electrical energy generated by the system. To calculate the LCOE, HOMER divides the cost of annual production by the total electric load supplied. It is estimated using EquationEquation 1010
10 :
Where is the total annualized cost,
is the annualized total DC load served by the system and
is the annualized total AC load served by the system.
3.5.3. Renewable fraction
The ratio of the energy obtained from the renewable energy sources to supply the load to the total energy produced by the entire system is known as the renewable fraction. It is usually desirable that the RF is high without it severely affecting the TNPC. This will reduce the emission level. According to D. E. Babatunde, Babatunde, Akinbulire, and Oluseyi (Citation2018), RF is obtained using EquationEquation 1111
11 .
3.5.4. Emissions
Using the emission factor for each air pollutant, the quantity of pollutant was obtained for the fuel consumed by the diesel generator. This study evaluates the amount of CO2, CO, unburned hydrocarbons, SO2, particulate matter, and NOx. To encourage a greener environment, it is desirable that the emission is kept minimal. This will mitigate greenhouse gas emissions and health hazards.
4. Results and discussion
4.1. System architecture
Table highlights the details of the energy system architectures selected as the optimal for each the geo-political zones based on two diesel prices considered. Inputs such as diesel prices, load profile and solar irradiation are fundamental to HOMER in coming up with the optimized number of components. HOMER prioritizes continuous meeting of the load demand in the component optimization process of the HRES. It is done to ensure minimal diesel consumption as well as make sure the PV system is not over-sized. The specification of space for installation is also taken into consideration when sizing the battery. Table shows that Okirika has the highest PV array of 80 kW for both diesel price sensitivity cases. This is an indication that more PV can be added to reduce the number of batteries needed to store energy because the level of solar irradiation is lowest at that particular site. Katagum, Gboko, Iseyin, and Ihiala have the same PV array configuration which is half of that of Okirika. The smallest number of batteries is recorded at Okirika. Gboko, Kabo, and Katagum returned the same capacity of batteries and converter size for both diesel price sensitivity cases (50 batteries and 10 kW converter). It can be uniquely seen that for all sites and sensitivity cases, the size of the converter and generator size remains unchanged. It is an indication that the inclusion of PV is able to keep the size of the generator at a minimum while meeting the load demand.
Table 5. System configuration for six geopolitical zones
Except for Kabo and Katagum, the PV array size and the number of batteries were directly proportional to the diesel prices. This is attributed to the fact that the costs of operating and maintaining the diesel generator rise with an increase in fuel pump prices. This, therefore, justifies the additional cost of installing a larger PV array and increasing the shortage capacity of the system in order to minimize the operating hours of the generator. This keeps the TNPC as low as possible while also increasing the lifespan of the generator. It is worth to note that it is important to add diesel generator so as to serve as a backup in times of system failure or maintenance of the RE source since telecommunication industries cannot afford the equipment going offline for a long period of time. For this study, the shortage capacity is zero—an indication that all system is well adapted to meet 100% of electric demand by the BTS.
4.2. Economic
Shown in Figure is the summarized TNPC of the HRES solutions for all the cities under consideration for sensitivity cases of 0.7$/L and 0.96$/L. The TNPC is estimated based on a project lifetime of 20 years. A base case for diesel generator is also included in the graph. The base case uses a single diesel generator to supply 100% of the BTS load. The base case is included in order to economically visualize and compare it with the HRES. Consequently, all the case studies for the PV-DG-BAT implementation for the six locations are compared with that base case with respect to TNPC, LCOE, RF carbon emission as well as fuel consumption for both 0.7$/L and 0.96$/L of diesel price. Katagum town in Northeastern Nigeria recorded the lowest TNPC of $354,973 for diesel pump price of 0.7$/L. This is due to the fact that this region receives the highest average annual solar irradiation in the country. The city of Kabo with a TNPC of $354,973 ranks second on the sites with the lowest TNPC. Incidentally, it also has the second-highest annual solar irradiation. Okirika displayed the highest TNPC ($462,119) out of the six zones. As anticipated, out of the sites studied, Okirika in southern Nigeria experiences the lowest annual solar irradiance annually. As compared to the base case, a total of $21,533 can be saved if the hybrid PV-DG-BAT system can be installed at Okirika.
Also shown in Figure , are all simulations results of TNPC for diesel price of 0.96$/L. It follows a similar trend as that of 0.7$/L sensitivity case except that the total possible savings, when compared with the base case simulations, are lower. It can be inferred that in both sensitivity cases, the average solar irradiance level is directly related to the TNPC. Higher solar irradiation would enable the PV array to directly supply the load for a long duration and consequently reducing the operational hours of the DG. This, in turn, decreases the cost spent on fuel and maintenance which is a component of TNPC. Figure shows the comparison of the levelised cost of energy (LCOE) for both sensitivity cases across the geopolitical zones. Katagum has the lowest LCOE for both diesel price sensitivity cases. At higher fuel prices, the LCOE increased.
4.3. Diesel consumption and CO2 emissions in kg/yr
Generally, CO2 emission is directly related to the liters of fuel consumed by the diesel generator annually. Thus, Figure shows the comparison of the quantity of diesel consumed annually by the PV-diesel-battery systems for each site (i.e. for 0.7$/L and 0.96$/L diesel price). For both diesel price cases, the BTS at Gboko consumed the highest liters of fuel which is estimated as 6766 litres and 5432 litres respectively. In the case of the site at Iseyin; it recorded 6027 litres and 1755 litres at 0.7 $/L and 0.9 $/L of fuel pump price respectively to rank as the site with least fuel consumption.
The annual quantity of CO2 emission (in kg/yr) for both sensitivity cases is presented in Figure . It is obvious that with regard to both sensitivity cases, Iseyin’s site recorded the lowest CO2 emission (i.e. 15,872kg and 44,622kg respectively)-a consequence of higher RF. Gboko’s site has the highest emission of 17,817kg/yr and 14,304kg/yr of CO2 at 0.7$/L and 0.96$/L of fuel pump price respectively (because of its low RF).
4.4. Renewable fraction
Due to environmental and health risks, the RF is desirable to be high so as to mitigate greenhouse gas emissions. It can be observed from Figure that at higher diesel price (0.96$/L) the RF is considerably high. The high penetration of renewables is due to an increase in diesel price which in turn necessitates the addition of more PV panels. Iseyin had the highest RF for both sensitivity cases while the lowest RF occurred at Gboko.
5. Conclusions
The technical, environmental and economic viability of deploying hybrid renewable energy systems (HRES) for remote base transceiver stations (BTS) under varying diesel fuel pump prices in Nigeria has been investigated in this study. The results of this study are consistent with the findings of Okundamiya et al. (Citation2014), Goel and Ali (Citation2015), Ogunjuyigbe and Ayodele (Citation2016), which all reported that a combination of PV-diesel generator and battery is a viable means of powering a BTS. This is however contrary to the conclusion drawn by Olatomiwa et al. (Citation2015b) which reported that a hybrid PV, battery, wind, and diesel energy system is the most feasible alternative for BTS electrification.
From the results of the analysis; a number of findings could be gleaned. Based on the TNPC, Katagum in North-eastern Nigeria is the best site to implement a PV-DG-battery hybrid energy system for BTS applications because of the abundance of solar irradiance resources available in this region. The best system configuration at 0.7$/L is a 40kW PV array, a 10kW diesel generator, 50 batteries, and a 10kW converter. The details of the best energy alternative at 0.96$/L consist of a 50kW PV array, a 10kW converter, a 10kW generator, and 50 batteries. A cost comparison of the optimal hybrid energy systems (at 0.7$/litre and 0.96$/litre fuel pump price) with the diesel generator option system for Katagum is given in Table . The overall results show that the PV-diesel-battery system performs better in terms of costs. Apart from this, the PV-diesel-battery system also reduced emission due to diesel fuel consumption reduction when compared to the conventional diesel generation. Further results show that the standalone diesel generator (base case system) architecture produced the highest LCOE of 1.125$/kWh and emits 72,542kg of CO2 annually. This is undesirable because of its hazardous impact on the environment and on the health of humans. If all the BTS sites across the six geopolitical zones adopt PV-diesel-battery as compared to the DG alone presently obtainable across the country, the operational cost will be reduced.
Table 6. Overall costs of HRES for Katagum
Due to the high solar regime experienced in parts of the country, diesel generator hybridized with PV technology offers an alternative option for powering BTSs in Nigeria. Hence, from the economic, technical, and environmental point of view, Nigeria has the potential for incorporating renewable energy sources such as the PV array in the design and implementation of a microgrid for telecommunication facilities. For example, from Table , even though the initial implementation cost of such a project is usually high, its economic viability is shown in its lower TNPC. At present, the adoption of this technology is low in Nigeria. Therefore, in order to reduce the initial cost of implementation of HRES in Nigeria, the government should subsidize and provide tariff concessions on renewable energy (RE) technologies. This will increase the RE penetration on energy mix thereby mitigating energy poverty and GHGs emissions. In the future, further studies may be carried out to determine the effect of the carbon tax on the system configuration as well as on the life cycle cost of the systems.
Acknowledgements
The authors thank the Management of Covenant University for the fund given in support of the publication of this article. The contribution of Dr Ighravwe Desmond towards the improvement of the paper is also appreciated.
Additional information
Funding
Notes on contributors
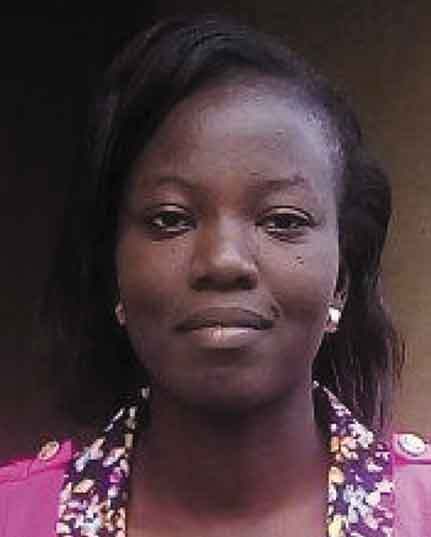
Olubayo Moses Babatunde
Babatunde Olubayo holds an M.Sc. degree in electrical electronic engineering. His research interests include renewable energy, energy management, and power system.
Iheanacho Henry Denwigwe
Denwigwe Iheanacho holds a B.Sc degree in electrical and electronics engineering. His research encompasses power systems analysis, alternative energy, and energy management.
Damilola Elizabeth Babatunde
Babatunde Damilola holds M.Sc. in Chemical Engineering. Her research interests include the environment, energy, and product development.
Augustine Omoniyi Ayeni
Ayeni Augustine holds a Ph.D. degree in Chemical Engineering. His research areas include solid and liquid waste treatment and beneficiation to fuels and chemicals, environmental pollution, sustainable and renewable energy, bioprocess engineering.
Toyosi Beatrice Adedoja
Adedoja Beatrice Toyosi holds a B.Sc. in computer science and M.Tech in GIS and remote sensing. Her area of research centers on space technologies, Geographic information systems and Remote sensing.
Oluwaseye Samson Adedoja
Adedoja, Oluwaseye Samson holds an M.Eng. in Mechanical Engineering. Adedoja is a Professional Engineer with research interests in space research and instrumentation, energy studies, modeling and optimization, and environmental studies.
References
- Adaramola, M. S., Paul, S. S., & Oyewola, O. M. (2014). Assessment of decentralized hybrid PV solar-diesel power system for applications in Northern part of Nigeria. Energy for Sustainable Development, 19, 72–19. doi:10.1016/j.esd.2013.12.007
- Adaramola, M. S., Quansah, D. A., Agelin-chaab, M., & Paul, S. S. (2017). Multipurpose renewable energy resources based hybrid energy system for remote community in northern Ghana. Sustainable Energy Technologies and Assessments, 22, 161–170. doi:10.1016/j.seta.2017.02.011
- Adegoke, A. S., & Babalola, I. T. (2011). Quality of service analysis of GSM telephone system in Nigeria. The American Journal of Scientific and Industrial Research, 2(5), 707–712.
- Adepetun, A. (2015). Nigeria’s telecoms operators power BTS with 50,000 gen sets. Accessed 25/01/2019. https://allafrica.com/stories/201501230522.html
- Adeyeye, A., Tsado, J., & Olatomiwa, L. Techno-economic analysis of PV/Diesel/Battery hybrid renewable system for remote primary healthcare center. In: International Conference of Mechanical Engineering, Energy Technology and Management, IMEETMCON 2018. International Conference Centre, University of Ibadan, Nigeria. 2018. p. 1.
- Akinbulire, T. O., Oluseyi, P. O., & Babatunde, O. M. (2014). Techno-economic and environmental evaluation of demand side management techniques for rural electrification in Ibadan, Nigeria. International Journal of Energy and Environmental Engineering, 5, 4. doi:10.1007/s40095-014-0132-2
- Akinyele, D. (2017a). Analysis of photovoltaic mini-grid systems for remote locations: A techno-economic approach. Int J Energy Res, 42(3), 1363–1380.
- Akinyele, D. (2017b). Techno-economic design and performance analysis of nanogrid systems for households in energy-poor villages. Sustainable Cities and Society, 34(June), 335–357. doi:10.1016/j.scs.2017.07.004
- Akinyele, D., Babatunde, O., Monyei, C., Olatomiwa, L., Okediji, A., Ighravwe, D., & Temikotan, K. (2019). Possibility of solar thermal power generation technologies in Nigeria: Challenges and policy directions. Renewable Energy Focus, 29, 24–41. doi:10.1016/j.ref.2019.02.002
- Akinyele, D., Belikov, J., & Levron, Y. (2018). Challenges of microgrids in remote communities: A STEEP model application. Energies, 11(2), 432. doi:10.3390/en11020432
- Amutha, W. M., & Rajini, V. (2015). Techno-economic evaluation of various hybrid power systems for rural telecom. Renewable and Sustainable Energy Reviews, 43, 553–561. doi:10.1016/j.rser.2014.10.103
- Ayodele, T. R. (2014). Feasibility study of stand-alone hybrid energy system for rural electrification in Nigeria: The case study of Ala-Ajagbusi community. International Journal of Renewable Energy Resources, 4(1), 1–12.
- Ayodele, T. R., Ogunjuyigbe, A. S. O., & Amusan, T. O. (2018). Techno-economic analysis of utilizing wind energy for water pumping in some selected communities of Oyo State, Nigeria. Renewable and Sustainable Energy Reviews, 91, 335–343. doi:10.1016/j.rser.2018.03.026
- Babatunde, D. E., Babatunde, O. M., Akinbulire, T. O., & Oluseyi, P. O. (2018). Hybrid energy systems model with the inclusion of energy efficiency measures: A rural application perspective. International Journal of Energy Economics and Policy, 8(4), 310–323.
- Babatunde, O., Akinyele, D., Akinbulire, T., & Oluseyi, P. (2018). Evaluation of a grid-independent solar photovoltaic system for primary health centres (PHCs) in developing countries. Renewable Energy Focus, 24, 16–27. doi:10.1016/j.ref.2017.10.005
- Bahramara, S., Moghaddam, M. P., & Haghifam, M. R. (2016). Optimal planning of hybrid renewable energy systems using HOMER: A review. Renewable and Sustainable Energy Reviews, 62, 609–620. doi:10.1016/j.rser.2016.05.039
- Dusabe, D., Munda, J. L., & Jimoh, A. A. (2007). Rural village electrification in South Africa: Role of energy efficiency in off-grid PV/dg system. Lamp, 4(15), 5.
- Dusabe, D., Munda, J. L., & Jimoh, A. A. (2009). Small scale solar energy systems in Rwanda : Status and sustainability. International Conference Domestic Use Energy, 2009, 1–6.
- Ekwujuru, P, & Ofili, P. (2016). Telecoms, others contribute 8.7% to Nigeria’s GDP. Accessed 23/01/2019.https://www.vanguardngr.com/2016/04/telecoms-others-contribute-8-7-nigerias-gdp/
- Faruk, N., Ayeni, A. A., Muhammad, M. Y., Abdulkarim, A., & Moses, O. (2012). Hybrid power systems for cell sites in mobile cellular networks. Cyber Journals: Multidisciplinary Journals in Science and Technology, Journal of Selected Areas in Renewable and Sustainable Energy (JRSE) (pp. 8–12). January Edition
- Goel, S., & Ali, S. M. (2014). Cost analysis of solar/wind/diesel hybrid energy systems for Telecom tower by using HOMER. International Journal of Renewable Energy Research (IJRER), 4(2), 305–311.
- Goel, S., & Ali, S. M. (2015). Hybrid energy systems for off-grid remote telecom tower in Odisha, India. International Journal of Ambient Energy, 36(3), 116–122. doi:10.1080/01430750.2013.823110
- HOMER. (2016). Homer pro version 3.7 user manual. CO, USA: Author.
- Ihekwoaba, C. (2017). It costs N2.6b monthly to power Nigeria’s GSM base stations, greenwish wants to change that. Accessed 23/02/2019. https://thenerveafrica.com/12635/it-costs-n2-6b-monthly-to-power-nigerias-gsm-base-stations-greenwish-wants-to-change-that/.
- Krishan, O.; Sathans. (2018). Design and Techno-Economic Analysis of a HRES in a Rural Village. Procedia Computer Science, 125, 321–328. doi:10.1016/j.procs.2017.12.043
- Lambert, T., Gilman, P., & Lilienthal, P. (2006). Micropower system modeling with HOMER. Integration of Alternative Sources of Energy, 1(1): 379–418.
- Leon, H. M. R., Shoeb, M. A., Rahman, M. S., Ahmed, M. U., & Islam, M. S. Design and economic feasibility analysis of autonomous hybrid energy system for rural Bangladesh. In: 2016 4th International Conference on the Development in the in Renewable Energy Technology (ICDRET), Dhaka, Bangladesh. 2016. p. 1–6.
- Mandal, S., Yasmin, H., Sarker, M. R. I., & Beg, M. R. A. Prospect of solar-PV/biogas/diesel generator hybrid energy system of an off-grid area in. In: 1st International Conference on Mechanical Engineering and Applied Science. Dhaka, Bangladesh; 2017.
- Mazzola, S., Astolfi, M., & Macchi, E. (2016). The potential role of solid biomass for rural electrification: A techno economic analysis for a hybrid microgrid in India. Applied Energy, 169, 370–383. doi:10.1016/j.apenergy.2016.02.051
- NASA. (2018). NASA surface meteorology and solar energy - location. Accessed on 10/01/2019. https://power.larc.nasa.gov/.
- Nema, P., Nema, R. K., & Rangnekar, S. (2010). PV-solar/wind hybrid energy system for GSM/CDMA type mobile telephony base station. International Journal of Energy and Environment, 1(2), 359–366.
- Nwulua, N. I., & Agboolab, O. P. (2011). Utilizing renewable energy resources to solve Nigeria’s electricity generation problem. International Journal of Thermal and Environmental Engineering, 3(1), 15–20. doi:10.5383/ijtee
- Ogunjuyigbe, A. R. S. (2016). Techno-economic analysis of stand-alone hybrid energy system for nigerian telecom industry. International Journal of Renewable Energy Technology, 7(2), 148–162. doi:10.1504/IJRET.2016.076089
- Ogunjuyigbe, A. S. O., & Ayodele, T. R. (2016). Techno-economic analysis of stand-alone hybrid energy system for Nigerian telecom industry. International Journal of Renewable Energy Technology, 7(2), 148–162. doi:10.1504/IJRET.2016.076089
- Ohunakin, O. S., Adaramola, M. S., Oyewola, O. M., & Fagbenle, R. O. (2014). Solar energy applications and development in Nigeria: Drivers and barriers. Renewable and Sustainable Energy Reviews, 32, 294–301. doi:10.1016/j.rser.2014.01.014
- Okundamiya, M. S., Emagbetere, J. O., & Ogujor, E. A. (2014). Assessment of renewable energy technology and a case of sustainable energy in mobile telecommunication sector. Sci World J, 2014.
- Olatomiwa, L., Blanchard, R., Mekhilef, S., & Akinyele, D. (2018). Hybrid renewable energy supply for rural healthcare facilities: An approach to quality healthcare delivery. Sustainable Energy Technologies and Assessments, 30, 121–138. doi:10.1016/j.seta.2018.09.007
- Olatomiwa, L., Mekhilef, S., Huda, A. S. N., & Ohunakin, O. S. (2015a). Economic evaluation of hybrid energy systems for rural electrification in six geo-political zones of Nigeria. Renewable Energy, 83, 435–446. doi:10.1016/j.renene.2015.04.057
- Olatomiwa, L., Mekhilef, S., Huda, A. S. N., & Sanusi, K. (2015b). Techno-economic analysis of hybrid PV–Diesel–Battery and PV–Wind–Diesel–Battery power systems for mobile BTS: The way forward for rural development. Energy Sci Eng, 3(4), 271–285. doi:10.1002/ese3.2015.3.issue-4
- Olatomiwa, L. J., Mekhilef, S., & Huda, A. S. N. Optimal sizing of hybrid energy system for a remote telecom tower: A case study in Nigeria. In: Energy Conversion (CENCON), 2014 IEEE Conference on. 2014, Johor bahru singapore. p. 243–247. doi:10.9758/cpn.2014.12.3.243
- Rashid, S., Rana, S., Shezan, S. K. A., Karim, S. A. B., & Anower, S. (2017). Optimized design of a hybrid PV-wind-diesel energy system for sustainable development at coastal areas in Bangladesh. Environmental Progress & Sustainable Energy, 36(1), 297–304. doi:10.1002/ep.v36.1
- Samson, A. O., Babatunde, O. M., & Denwigwe, I. H. (2019). Powering a Space Environment Research Laboratory (SERL): Hybrid renewable energy system or diesel system? Energy Engineering, 116(2), 41–64. doi:10.1080/01998595.2019.12054404
- Shahzad, M. K., Zahid, A., Rashid, T., Rehan, M. A., Ali, M., & Ahmad, M. (2017). Techno-economic feasibility analysis of a solar-biomass off grid system for the electri fi cation of remote rural areas in Pakistan using HOMER software. Renewable Energy, 106, 264–273. doi:10.1016/j.renene.2017.01.033