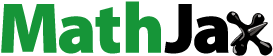
Abstract
Inefficient near-surface characterizations prior to building construction have largely contributed to the incessant building failures in the form of structural defects and building collapses recently occurring in southern parts of Nigeria. Combined geophysical and geotechnical investigations have been used to select suitable foundation type and depth at a building construction site in part of the Lagos Island, Nigeria. Three geoelectrical resistivity survey profiles of length 150 m each were conducted using minimum and maximum electrode spacing of 5.0 m and 45.0 m, respectively. Boring, in-situ geomaterials samplings, cone penetrating tests, standard penetrometer tests and laboratory tests were among the geotechnical investigations carried out in accordance with the British standard code of practice. The geoelectrical resistivity imaging results provide the lateral and spatial spread of the geoelectrical units stratification within the study area, their clay and water contents. The results of the laboratory and geotechnical tests also reveal the subsoils lithologic units, their compressibility and shear strengths. A deeper pile-type foundation on a more competent stable subsoil stratum at the depth greater than 13 m is recommended for the proposed buildings in the study area so as to effectively transmit their loads.
PUBLIC INTEREST STATEMENT
The recent incessant building collapse in Lagos State, Nigeria is worrisome to all and sundry. This is due to a huge loss of lives and properties running into millions of dollars yearly. The major findings have revealed that one major factor causing the failure and collapse of buildings in Nigeria is the fact that a culture of conducting reliable geophysical and geotechnical investigations for accurate subsoil characterization information prior to building construction is non-existent in Nigeria. This research has made an effort to integrate both geophysical and geotechnical techniques to characterize near-surface heterogeneity of the area of study. The results obtained were used to choose a suitable foundation type for the investigated building construction site.
1. Introduction
Buildings are infrastructures that contribute significantly to the enhancement of the sustainable development, it is, therefore, necessary to have adequate plan for sustainable building constructions that will stand the test of time (Akpabot, Ede, Olofinnade, & Bamigboye, Citation2019; Ede, Olofinnade, Bamigboye, Shittu, & Ugwu, Citation2017; Windapo & Rotimi, Citation2012). One of the factors contributing to incessant building collapse and foundation failures in most developing countries is the lack of adequate information about the near-surface characterization prior to construction. Some of these catastrophic occurrences may include wall cracks, distress, subsidence, tilting, and a partial or total collapse of building due to foundation failures (Oyeyemi & Olofinnade, Citation2016). It is also important to take into cognizance accurate structural analysis and good understanding of the supporting subsurface geology which are prerequisites for designing stable foundation base for the successful execution of building constructions (Oyeyemi et al., Citation2017; Oyeyemi & Olofinnade, Citation2016; Ubido, Igwe, Ukah, & Idris, Citation2017). Therefore, to avoid foundation failures and building collapse, there is a need for detailed geophysical and geotechnical investigations.
Several studies have used the integration of geophysical and geotechnical investigations to characterize the near surface with a view to determine its suitability for road and building constructions (Al Fouzan & Dafalla, Citation2013; Oladunjoye, Salami, Aizebeokhai, Sanuade, & Kaka, Citation2017). For example, Adewoyin et al. (Citation2019) adopted the use of seismic refraction geophysical method to determine the geotechnical engineering properties of a site prior to development and uses these parameters to developed model equations for purpose of near-surface soil characterization. Oladunjoye et al. (Citation2017) employed geoelectrical resistivity and seismic refraction methods to characterize the near surface of a proposed conference center with a view to understand the weathered profile at the site. Al Fouzan and Dafalla (Citation2013) successfully used the integration of geotechnical and geophysical methods to characterize the near surface for possible cracks and ground distress in Saudi Arabia.
Geoelectrical resistivity technique has been affirmed to be very efficient and applicable in various contexts such as groundwater exploration, engineering site investigations, agronomy, determination of compaction and soil horizon thickness, archaeological prospecting, assessment of soil hydrological properties and foundation stability assessment (Aizebeokhai, Ogungbade, & Oyeyemi, Citation2017; Aizebeokhai & Oyeyemi, Citation2014, Citation2017; Aizebeokhai, Oyeyemi, & Joel, Citation2016; Aizebeokhai, Oyeyemi, & Kayode, Citation2015; Aizebeokhai et al., Citation2017; Oyeyemi, Aizebeokhai, & Oladunjoye, Citation2015a; Oyeyemi, Oladunjoye, Aizebeokhai, Ajekigbe, & Ogunfolakan, Citation2015b). This technique is particularly applicable to engineering site investigations because it measures apparent electrical resistivity within the subsurface which is a function of several factors such as grain sizes distribution, mineralogy, soil porosity and permeability, degree of water saturation, electrical resistivity of the interstitial fluid (depending on the concentration of the solute) and temperature. In addition, electrical resistivity technique, as a non-destructive method for subsurface characterizations, gives spatial and temporal variations of many physical properties of the subsoil such as soil structure and stratification, and fluid composition or water content without digging.
Geotechnical investigations such as boring, drilling, Dutch cone penetrating test (CPT), standard penetrating test (SPT) and several laboratory tests (including Atterberg limits, moisture contents, quick undrained triaxial and Oedometer consolidation tests) are designed within a site to understand the engineering characteristics and bearing capacity of the subsurface geomaterials in the site. The information from both geoelectrical and geotechnical investigations of subsurface geomaterials can be used to determine the kind of building design, foundation type, settlement rate and subsoil bearing capacity for a particular site prior to building construction work. Therefore, this study is aimed at integrating geoelectrical resistivity surveys and geotechnical investigations using CPT and SPT with a view to identifying and characterizing the near-surface strata at Osborne Foreshore Estate Phase II, Lagos, Nigeria in order to evaluate the bearing capacity of the study area. The findings from this study will assist greatly in recommending a suitable foundation type for the proposed building constructions within the study area.
2. Methodology
2.1. Site location and geological setting
The two sites designated as block A and block B for the building construction are located within the Osborne Foreshore Estate Phase II (Lat. N and Long.
E), Ikoyi, Lagos Island, southwestern Nigeria as indicated in Figure . This part of southwestern Nigeria is within the coastal zone marked by lagoons and coastal creeks (Longe, Malomo, & Oloruniwo, Citation1987), wherein the sand ridges formed by barrier bridges are linked with sand accumulation (Webb & Hill, Citation1958). The geological setting is that of Dahomey basin consisting of six lithostratigraphic formations, namely: Abeokuta, Ewekoro, Akinbo, Oshosun, Ilaro and Benin Formations from youngest to the oldest; these formations have been extensively discussed in many studies (e.g. Adegoke & Omatsola, Citation1981; Ako, Adegoke, & Petters, Citation1980; Elueze & Nton, Citation2004; Okosun, Citation1990).
2.2. Electrical resistivity survey
The 2D electrical resistivity imaging (ERI) investigation was conducted using the ABEM Terameter (SAS 1000/4000 series) along three profile lines (L1-3). The ERI profile lines were oriented in northwestern to southeastern directions with a length of 150 m each. The data were acquired using Wenner array electrode configuration as shown in Figure , with minimum and maximum electrode spacing of 5.0 m and 45.0 m, respectively. The data obtained were processed and inverted using the RES2DINV software, with a least-squares inversion algorithm using a regularization technique (Loke & Barker, Citation1996).
2.3. Boring, sampling, SPT and CPT
The procedures adopted for boring, sampling in-situ geomaterials, cone penetrometer test (CPT), standard penetrometer test (SPT) and laboratory tests were in accordance with the British standard code of practice for construction site investigations, B.S 5930 (Citation1999). Two (2) holes designated as BH1 and BH2 were bored within the study area to a depth of 30 m using percussion boring method. The boring involves the use of shell and auger tools to cut through the soil strata to depth of boring. Disturbed soil samples were collected at every 75 mm. Also, undisturbed samples were collected in the cohesive soil using a 100 mm internal diameter open tube sampler fitted with a cutting shoe. The SPT was conducted in cohesionless soil using a thick-walled split spoon that was about 35 mm in internal diameter driven into the soils through several blows from 65 kg hammer falling from about 760 mm height. The resistance “N” value of the SPT shows the empirical evaluation of the soil’s consistencies; it is used to assess the strength, bearing capacity and compressibility of the granular soil. The collected soil samples were well preserved and transferred to the laboratory for further testing. In addition, a 2.5 ton capacity penetrometer probing machine (CPT) was equally used to measure the in-situ strength of the soil within the study area. A total number of six (6) cone penetrometer tests denoted as CP1-6 were carried out within the area of study.
2.4. Laboratory tests
The soil samples collected were analyzed at the Civil Engineering laboratory, Covenant University, Ota, Nigeria, for the determination of soil type and geotechnical properties necessary for the design of building foundation. Sieve analysis for the particle size distribution of the representative soil samples was conducted by washing an approximately 500 g sample using No. 200 sieve (0.075 mm) to separate the silty clay from the fine-grained sand samples. The retained fraction on the sieve was then dried and later subjected to sieving procedure using the automatic sieve shaker, such that each retained sample in each sieve was weighed and recorded. Other tests carried out on selected soil samples include the liquid limit, plastic limit, plasticity index, determination of moisture contents, while the quick drained triaxial and consolidation tests were carried out on the undisturbed samples. The tests were conducted following the specification of BS 1377 (Citation1990).
Figure 1. Geological map of Nigeria showing the study area (After Obaje, Citation2009)
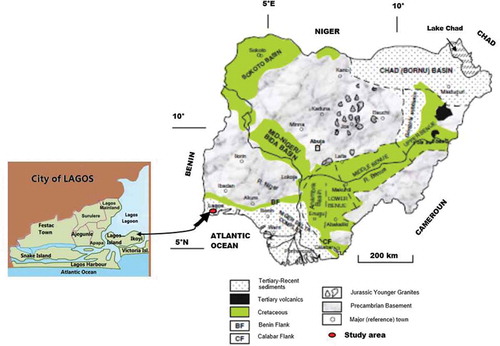
Figure 2. Wenner array for geoelectrical resistivity data measurements (Aizebeokhai & Oyeyemi, Citation2014)
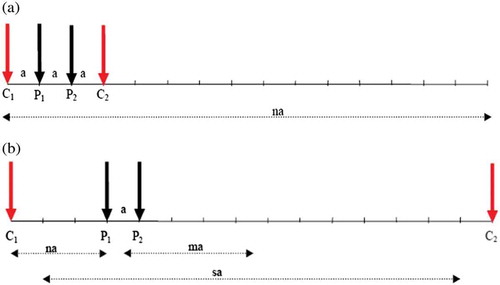
3. Results
The electrical resistivity inverted models for the three 2D profile lines show generally two geoelectric layers in the entire study area as seen in Figures –. They include the top layer, which is loose silty-sand lithologic unit with model resistivity values of 93–400 , 99–450
and 100–400
across profiles L1, L2, and L3, respectively. The second geoelectrical resistivity layer consists of a sandy-clay unit with model resistivity values of 2–46
, 2–47
and 3–51
across profiles L1, L2, and L3, respectively. The results of the borehole logs show seven zones for BH1 and five zones for BH2 (Figure ,). The logs display the stratification of soils and their description on the basis of types, colour and texture.
Figure 6. (a) Results of BH1 soil log stratification and description for the study area. (b) Results of BH2 soil log stratification and description for the study area
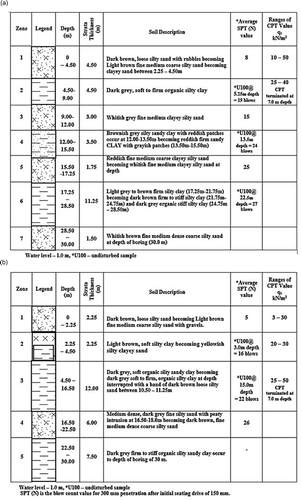
The cone penetrating test (CPT) was conducted to determine the relative strength of the near-surface strata and also to assess the in-situ relative density of the soil over the subsurface depths tested. Tables and show the results of the average cone resistance values for the relative strength of in-situ geomaterials to a depth of about 7 m. Average cone resistance values for CP 1–3 range between 6 kN/m2 and 47 kN/m2, while that of CP4-6 range between 10 kN/m2 and 48 kN/m2. The strata’s allowable bearing pressures and submerged bearing pressure to a depth of about 7 m are also presented and compared (Figures –). The range of the allowable bearing pressure is 18–128 kN/m2 for block A and 28–132 kN/m2 for block B.
Figure 9. Comparing the estimated average allowable bearing pressures with depth within the two sites of study (blocks A and B)
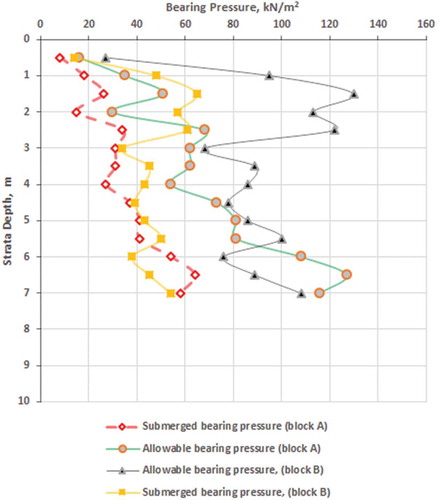
Table 1. Estimated average cone resistance of soil with depth (block A)
Table 2. Estimated average cone resistance of soil with depth (block B)
The particle size distribution values for non-plastic soil materials and soil grained classification according to the unified soil classification system are presented in Table . The sieve analysis reveals that the sand materials encountered in the boreholes are mostly poorly graded sand (SP) according to the soil classification system. The triaxial test results (natural moisture content, bulk density, unstrained cohesion, angle of internal resistance) on selected undisturbed samples at respective depths (Table ). The table shows natural moisture contents (MC) with range 18–28% and an average of about 23%, bulk density with range 1.69–1.91 Mg/m3 and an average of 1.84 Mg/m3, undrained shear strength test with range 20–67 kN/m2 and an average of 35.4 kN/m2. Table shows the results of the consolidation tests on the selected samples with the compressibility values ranging between 0.136 and 0.469 m2/MN for the coefficient of volume compressibility (MV) having a pressure range between 50 kN/m2 to 800 kN/m2.
Table 3. Summary of Particle Size Distribution and Soil Grained Classification
Table 4. Results of Triaxial Tests on selected samples
Table 5. Results of consolidation tests on selected samples
4. Discussion
The efficiency of the ERT techniques is the ability to measure electro-hydraulic properties of the subsoil geomaterials such as geoelectrical resistivity and water saturation, and subsequently determine the lateral and spatial variation of these parameters. The variation of these properties is due to the subsurface heterogeneity in terms of lithologic types, grain size distribution, water content and clay content. The depth of investigations for the 2D electrical resistivity imaging profiles is about 25 m below the ground level; the thickness of the upper silty-sand layer range from 9 m in profile L1 to 13 m in profile L3. This layer overlies conformably the sandy-clay unit at the base with conspicuous upconning intrusions of seawater that have been interpreted to be from the nearby lagoon in consonance with the results of several other researches within the Lagos Island, southwestern Nigeria (Oyeyemi et al., Citation2017, Citation2015b; Oyeyemi & Olofinnade, Citation2016). The results of the geoelectrical imaging give the two-dimension lateral distribution of the geoelectrical layers within the area better than geotechnical investigations which are 1D. Electrical resistivity tomography results have further revealed the absence of any void within the subsurface that may result in subsidence and building collapse within the area. However, getting portable borehole water for buildings within the area would be difficult as the identified upconned saltwater from the nearby lagoon can pollute the borehole water.
The borehole logs reveal that the first layer of the subsoil is loose dark brown fine-medium grained silty-sand underlain by a weak layer of soft, organic silty-clay. Moreover, beneath this weak layer of soil exist a medium of dense fine-grained silty-sand and firm-to-stiff grey organic silty-sandy-clay to a boring depth of 30 m. There are intercalations of these delineated lithologies within the subsurface and the results of both the SPT “N” value and CPT indicate that the geomaterials of the first layer are of low relative density, soft consistency and high compressibility potential. The geomaterials revealed in the borehole logs and their geotechnical properties from CPT agree well with the delineated geoelectrical layers from the geoelectrical resistivity imaging. The estimated allowable pressures, as presented in Figures and , are halved due to the submergence of foundation material at 0.30 m from the surface as encountered during the field testing. The strata are submerged implying the soil mass will be saturated; therefore it is important to understand that water content is vital to the strength, compressibility characteristics and behaviour of cohesive geomaterials. However, by contrast, water does not significantly change the properties of a cohesionless geomaterial except it is submerged, in which case the unit weight is reduced. The comparison between the estimated allowable bearing and submerged pressures for both block A and block B within the study area (Figure ). It is obvious that the estimated bearing pressure for block B is higher compared to that of block A which is evident in the SPT “N” values from the borehole logs. The implication of the triaxial test results in the study area is the presence of clayey materials with medium compressibility property. Geotechnical tests reveal that the subsoils possess soft clayey materials at the top with low shear strength, while the base units possess firm clayey materials with moderate shear strength that is adequate enough to support the structural load of foundation piles.
The results of the geotechnical investigations corroborate well with that of the geophysical investigation and revealed that the soft-to-firm clayey geomaterials within the study area have low to moderate shear strength. This implies that the soil materials in the top layer cannot support heavy loads as this could lead to possible foundation failure. Hence, the foundation should not be placed directly on the topsoil. Therefore, a deeper pile-type foundation on the basal geoelectric layer is recommended. This is because using a pile foundation type resting on the second geoelectrical layer will cause the higher loadings due to the proposed buildings to transmit their loads into a more stable and competent basal subsoil stratum within the subsurface.
5. Conclusion
Electrical resistivity imaging and geotechnical investigations have been used for near-surface geomaterials characterization at Osborne Foreshore Estate Phase II, Lagos, Nigeria with a view to characterize the subsoil and determine the subsoils geoengineering properties towards recommending the foundation type and depth for building construction in the site. Geoelectrical resistivity inverted models show two broad geoelectric layers: top layer which is composed of loose silty-sand at depth ranging from 9 to 13 m and the sandy-clay unit with resistivity values ranging from 2 to 51 at the base. The geophysical investigations provide the lateral and spatial distribution of the geologic units within the study area. Electrical resistivity imaging equally revealed the presence of the seawater intrusion within the area that may affect the borehole water of the residents within the area. Generally, the results of the entire geotechnical tests reveal that the soft-to-firm clayey materials within the subsurface that exhibit low-to-moderate shear strength indicating that the soil material close to the surface can only be used to support a very limited structural load. A deeper pile-type foundation on the second geologic layer is recommended in order to guide against the possibility of soil rupture in the absence of soil stratum with adequate strength at a reasonable depth leading to shear failure of the soil. The ability of geophysical investigation in subsurface characterization has been demonstrated. This study has shown the importance of integrating geophysical investigation with geotechnical evaluation for optimal characterization of subsurface geomaterials and we suggest that these techniques be carried out within any building construction site in order to obtain more subsoil information and boost the confidence of structural engineers in taking effective and efficient decisions regarding suitable foundation type for buildings or engineering structures at design stage before embarking on construction.
Acknowledgements
The authors wish to express their appreciations to Covenant University centre for research innovation and development (CUCRID) for sponsoring this research. A lot of thanks due to the anonymous reviewers and the editor for their efforts and their valuable comments that have improved this paper.
Additional information
Funding
Notes on contributors

K. D. Oyeyemi
Dr. Oyeyemi Kehinde David, PhD currently works at the Applied Geophysics Unit of the Department of Physics, Covenant University Ota Nigeria. His research interests include applications of various cutting edge geophysical techniques to proffer solutions to different exploration (hydrocarbon, geothermal and groundwater), geotechnical engineering and environmental problems.
References
- Adegoke, O. S., & Omatsola, M. E. (1981). Tectonic evolution and cretaceous stratigraphy of the dahomey basin. Nigeria Journal Of Mining and Geology, 18, 130–15.
- Adewoyin, O. O., Joshua, E. O., Akinyemi, M. L., Omeje, M., & Akinwumi, S. (2019). Predicting dynamic geotechnical parameters in near-surface coastal environment. Cogent Engineering, 6, 1588081.
- Aizebeokhai, A. P., Ogungbade, O., & Oyeyemi, K. D. (2017). Integrating VES and 2D ERT for near-surface characterization in a crystalline basement terrain (pp. 5401–5406). SEG Technical Program Expanded Abstracts. Society of Exploration Geophysics (SEG) International Exposition and 87th Annual Meeting, 2017. Houston, Texas.
- Aizebeokhai, A. P., & Oyeyemi, K. D. (2014). The use of the multiple-gradient array for geoelectrical resistivity and induced polarization imaging. Journal of Applied Geophysics, 111, 364–376. doi:10.1016/j.jappgeo.2014.10.023
- Aizebeokhai, A. P., & Oyeyemi, K. D. (2017). Geoelectrical characterization of basement aquifers: The case of Iberekodo, Southwestern Nigeria. Hydrogeology Journal, 26(2), 651–664. doi:10.1007/s10040-017-1679-9.
- Aizebeokhai, A. P., Oyeyemi, K. D., & Joel, E. S. (2016). Groundwater potential assessment in a sedimentary terrain, southwestern Nigeria. Arabian Journal of Geoscience, 9(7), 496–513. doi:10.1007/s12517-016-2524-5.
- Aizebeokhai, A. P., Oyeyemi, K. D., & Kayode, O. T. (2015). Assessment of soil petrophysical para meters using electrical resistivity tomography (ERT) and induced polarization techniques. Research Journal of Applied Sciences, 10(9), 479–485.
- Aizebeokhai, A. P., Oyeyemi, K. D., Noiki, F. R., Etete, B. I., Arere, A. U. E., Eyo, U. J., & Ogbuehi, V. C. (2017). Geoelectrical resistivity data sets for subsurface characterization and aquifer delineation in Iyesi, southwestern Nigeria. Data in Brief, 15, 812–832. doi:10.1016/j.dib.2017.10.057
- Ako, B. D., Adegoke, O. S., & Petters, S. W. (1980). Stratigraphy of Oshoshun formation in Southwestern Nigeria. Nigerian Journal of Mining and Geology, 17, 99–106.
- Akpabot, A. I., Ede, A. N., Olofinnade, O. M., & Bamigboye, G. O. (2019). Risks of seismic activities on built environment in Nigeria. International Journal of Environment and Sustainable Development, 18(3), 259–269. doi:10.1504/IJESD.2019.10022557
- Al Fouzan, F., & Dafalla, M. A. (2013). Study of cracks and fissures phenomenon in Central Saudi Arabia by applying geotechnical and geophysical techniques. Arabian Journal of Geosciences, 7(3), 1157–1164. doi:10.1007/s12517-013-0884-7
- British Standards Institution (BSI). (1990). British standard methods of test for soils for civil engineering purposes. BS 1377: Part 2. Milton Keynes: British Standards Institute.
- British Standards Institution (BSI). (1999). British standard methods of practice for site investigation. BS 5930. Milton Keynes: British Standards Institute.
- Ede, A. N., Olofinnade, O. M., Bamigboye, G. O., Shittu, K. K., & Ugwu, E. I. (2017). Prediction of fresh and hardened properties of normal concrete via choice of aggregate sizes, concrete mix-ratios and cement. International Journal of Civil Engineering and Technology, 8(10), 288–301.
- Elueze, A. A., & Nton, M. E. (2004). Organic geochemical appraisal of limestones and shales in part of eastern Dahomey basin, southwestern Nigeria. Journal of Mining and Geology, 40(1), 29–40. doi:10.4314/jmg.v40i1.18806
- Flagg, C. G. (1979). Geological causes of dam incidents. Bulletin of the International Association of Engineering Geologists, 20(1), 196–201. doi:10.1007/BF02591282
- Joshua, O., Amusan, L. M., Olusola, K. O., Ogunde, A., Ede, A. N., & Tunji-Olayeni, P. F. (2017). Assessment of the utilization of different strength classes of cement in building construction in Lagos, Nigeria. International Journal of Civil Engineering and Technology, 8(9), 1221–1233.
- Loke, M. H., & Barker, R. D. (1996). Rapid least-squares inversion of apparent resistivity pseudo sections by a quasi-Newton method. Geophysical Prospecting, 44, 131–152. doi:10.1111/gpr.1996.44.issue-1
- Longe, E. O., Malomo, S., & Oloruniwo, M. A. (1987). Hydrogeology of Lagos Metropolis. Journal of Africa Earth Science, 6, 163–174. doi:10.1016/0899-5362(87)90058-3
- Obaje, N. C. (2009). Geology and mineral resources of Nigeria. In S. B. Brooklyn, H. J. N. Bonn, J. R. Gottingen, & K. S. Graz (Eds.), Lecture notes in earth sciences book series (pp. 122). Berlin, Heidelberg: Springer. https://doi.org/10.1007/978-3-540-92685-6
- Ogunde, A., Joshua, O., Amusan, L. M., & Akuete, E. (2017). Project management of a panacea to improving the performance of construction project. International Journal of Civil Engineering and Technology, 8(9), 1234–1242.
- Okosun, E. A. (1990). A review of the cretaceous stratigraphy of the dahomey embayment, West Africa. Cretaceous Research, 11, 17–27. doi:10.1016/S0195-6671(05)80040-0
- Oladunjoye, M. A., Salami, A. J., Aizebeokhai, A. P., Sanuade, O. A., & Kaka, S. I. (2017). Preliminary geotechnical characterization of a site in Southwest Nigeria using integrated electrical and seismic methods. Journal of the Geological Society of India, 89(2), 209–215. doi:10.1007/s12594-017-0585-z
- Oyeyemi, K. D., Aizebeokhai, A. P., Adagunodo, T. A., Olofinnade, O. M., Sanuade, O. A., & Olaojo, A. A. (2017). Subsoil characterization using geoelectrical and geotechnical investigations: Implications for foundation studies. International Journal of Civil Engineering and Technology, 8(10), 302–314.
- Oyeyemi, K. D., Aizebeokhai, A. P., & Oladunjoye, M. A. (2015a). Integrated geophysical and geochemical investigations of saline water intrusion in a coastal alluvial terrain, southwestern Nigeria. International Journal of Applied Environmental Sciences, 10(4), 1275–1288.
- Oyeyemi, K. D., Oladunjoye, M. A., Aizebeokhai, A. P., Ajekigbe, P. G., & Ogunfolakan, B. A. (2015b). Integrated geophysical investigations for imaging archaeological structures in ancient city of ile-ife, Nigeria. Asian Journal of Information Technology, 14(7), 246–252.
- Oyeyemi, K. D., & Olofinnade, O. M. (2016). Geoelectrical- Geotechnical studies for near surface characterization, case history: Lagos SW Nigeria. Electronic Journal of Geotechnical Engineering, 21(10), 3735–3750.
- Ubido, E. O., Igwe, O., Ukah, B. U., & Idris, I. G. (2017). The implication of subsoil geology in foundation failure using geotechnical methods: A case study of Lagos Southwestern Nigeria. International Journal of Advanced Research and Publications, 1(5), 165–174.
- Webb, J. E., & Hill, M. B. (1958). The ecology of Lagos Lagoon. In: Lagoons of the Guinea Coast. Royal Society of London Philosophical Transactions of the Royal Society B, 241, 3017–3318.
- Windapo, A. O., & Rotimi, J. O. (2012). Contemporary issues in building collapse and its implications for sustainable development. Buildings, 2(3), pp.283–299. doi:10.3390/buildings2030283