Abstract
In the present work, we have investigated the effect of the chemical structure, concentration and solvent on the morphological properties of diblock copolymers at a certain percentage of humidity. The block copolymers were prepared using the atom transfer radical polymerization (ATRP) to obtain well-defined amphiphilic block copolymers with controlled molecular weights and molecular weight narrow size distribution. Breath figures (BF) method was used for the formation of porous materials or microspheres under certain experimental conditions, where the role of the water involved is crucial. The self-assembly behavior of these copolymers in presence of water drops was investigated by optical microscopy and scanning electron microscopy (SEM) exhibiting their ability to form spherical micelles under certain experimental conditions. The morphological properties of the films indicated that these diblock copolymers promotes the coexistence of porous and microspheres in the surface of the films.
PUBLIC INTEREST STATEMENT
This work shows the approaches to prepare and characterize porous-structured materials by using the breath figure (BF) methodology under certain experimental conditions where the role of the water is crucial. The addition of water in the polymer solution promotes the coexistence of porous films and microspheres. In particular, we have analyzed the topographical modifications of the surface that can be turned with this approach, such as the control of the pore characteristics, changes in the pore morphology. Those porous materials with cavities in the micrometer size range are interesting in catalysis, sensors, membrane preparation or as scaffolds for composite materials.
1. Introduction
In recent decades, widespread attention has been given to the self-assembly of diblock copolymers because of the potential applications of the resulting products in nanoscience and technology (Liu & Chiu, Citation2010; Müller-Buschbaum et al., Citation2009; Pizarro et al., Citation2016, Citation2017; Wang et al., Citation2011; Zhang et al., Citation2010). The amphiphilic block copolymers are expected to have self-assembling nanostructures with domain sizes dependent on their block ratios, compositions, chain length, and molecular architecture. The phase separation of the block copolymer produces ordered nanostructures with different morphologies (Li et al., Citation2012). Due to these microphase separation associated with these block copolymers; the morphology can be tuned from spherical micelles to lamellae, by continuously changing the length of the blocks (Kim et al., Citation2008).
On the other hand, a wide variety of work about the atom transfer radical polymerization (ATRP) to prepare well-defined amphiphilic block copolymers with controlled molecular weights and molecular weight narrow size distribution have been reported (Beers et al., Citation1999; Coca et al., Citation1998; Davis & Matyjaszewski, Citation2000; Davis et al., Citation1999; Lonsdale et al., Citation2009; Moineau et al., Citation1999; Pizarro et al., Citation2010, Citation2013; Xia et al., Citation1999; Zhang et al., Citation2009). The properties of poly(hydroxyethylmethacrylate)-b-poly(N-phenylmaleimide) diblock copolymers and their ability to form large compound spherical micelles was reported (Pizarro et al., Citation2013). In addition, the polymerization by ATRP of several acrylic derivatives such as methyl acrylate (Davis et al., Citation1999), n-butyl acrylate (Matyjaszewski et al., Citation1998), and 2-hydroxyethyl methacrylate (Mühlebach et al., Citation1998; Pizarro et al., Citation2018) have also been reported as materials with technological applications. In the same line, the well-defined amphiphilic poly[(tert-butylacrylate)-block-(N-phenylmaleimide)] diblock copolymers of different lengths have been applied in the manufacture of the hybrid polymer films (Pizarro et al., Citation2018).
Breath figures (BF) method using block copolymer has been associated to the formation of porous materials or microspheres under certain conditions, in which the role of water is crucial (De León et al., Citation2012; Widawski et al., Citation1994; Zhou et al., Citation2001). De León et al. described the formation of honeycomb structures using the blend of poly(methyl methacrylate) (PMMA) and an amphiphilic block copolymer based on MMA and 2-([(D-glucosamin-2-Nyl)carbonyl]oxy)ethyl methacrylate. The addition of water in the polymer solution promotes the coexistence of porous films and microspheres (De León et al., Citation2012).
In other study, the influence of various solvents on the morphology of poly(methyl methacrylate) particles was evaluated. The results showed that adding water to the dilute solutions of PMMA in either, acetone or THF, produced porous particles or honeycomb structured films, respectively (Zhou et al., Citation2001).
In this work, the formation of nanosized spherical or porous polymers was studied under certain experimental conditions. The research was focused on the formation of structured material applying the BF method using N-phenylmaleimide-based block copolymers. Here we have investigated the effect of the concentration and solvent in order to study the influence of the water on the self-assembly behavior of the block copolymer structures and its morphological properties.
2. Experimental
2.1. Materials
Ethyl-2-bromopropionate (EBrP, 98%), N,N,N’,N”,N”-pentamethyl diethylenetriamine (PMDETA, 99%), tetra-butyl ammonium fluoride (1.0 N in THF, 99%) and imidazole (C3H4N2, 99%) were purchased from Sigma-Aldrich and used as received. Tert-butyl acrylate (tBA) (Sigma-Aldrich, 98%) was extracted with 5% aqueous NaOH and then washed with distilled water. Copper(I) bromide (CuBr, Sigma-Aldrich, 98%) was purified by stirring overnight in acetone at room temperature. Hydroxypropylmethacrylate (HPMA) (Sigma-Aldrich) was purified by distillation under-reduced pressure. Aniline (C6H5NH2, 99.5%) and maleic anhydride (C4H2O3, 99%) were purchased from Merck-Schuchardt Chemicals.
2.2. Measurements
The block copolymers were characterized by 1 H-NMR on a Bruker 400 MHz spectrometer using CDCl3 as solvent. FTIR spectra were determined by Bruker Vector 22 equipment (Bruker Optics GmbH, Germany). Elemental analyses (EA) were carried out using a Carlo Erba 1106 analyzer (Italy). The molecular weights and the molecular weight distribution of the polymers were determined by size exclusion chromatography (SEC) using a Shimatzu LC 20 instrument equipped with RI detectors with DMF as the solvent (flow rate: 1.0 mL/min). The absorption spectrum was recorded at 25 ºC between 200 and 800 nm in a spectrophotometer Perkin Elmer model Lambda 35. Photoluminescence (PL) spectra of solid samples were recorded at room temperature with a Perkin Elmer spectrofluorometer model L 55, using an excitation wavelength of 320 nm. Thermal studies were performed using a Mettler Toledo Star Systems, at a heating rate of 10°C min−1 under nitrogen. The morphology was characterized by SEM (JEOL, GSM-6380LV).
2.3. Synthesis of N-phenylmaleimide
N-phenylmaleimide (N-PhMI) was synthesized from maleic anhydride and aniline in diethyl ether using a previously reported procedure (Cava et al., Citation1961) (see Scheme 1). The yield was 77%.
2.4. Synthesis of the block copolymers by ATRP
The synthesis of poly(tert-butyl acrylate) macroinitiators bromo-terminated (P(tBA)-Br) and PtBA-b-PN-PhMI block copolymers (denoted AP-1) was previously reported by our research group (Pizarro et al., Citation2010, Citation2013). The macroinitiator, 2-[(tert-butyldimethyl silyl)oxy] propylmethacrylate abbreviated as P(HPMA-TBDMS) and PHPMA-b-PN-PhMI block copolymer (denoted HPP-1) were synthesized according to the literature. (Mori et al., Citation1994) (see Table and Scheme 2). The block copolymers were prepared using the atom transfer radical polymerization (ATRP) to obtain well-defined amphiphilic block copolymers with controlled molecular weights and a narrow size distribution. The macroinitiator P(HPMA-TBDMS)-Br was polymerized with N-phenylmaleimide (N-PhMI) using the CuBr/PMDETA initiating system. The final block copolymer, abbreviated as P(HPMA)m-b-P(N-PhMI)n was obtained by the hydrolysis of the tert-butyl group by treatment with trifluoroacetic acid. The experimental data values by SEC showed the molecular weight distribution with narrow distribution (see Table in results and discussion section).
Table 1. Molecular weight and copolymer composition for the polymers
2.5. Preparation of polymer films
Breath figures (BF) method was used for the formation of porous materials or microspheres under the following experimental conditions. A series of block copolymer solutions were prepared in THF or CS2 at different concentrations (3.0 and 5.0 g L−1) maintaining humidity and temperature constant. After that, the block copolymer films were prepared using the solvent-assisted technique. Water (100 µL water/mL organic solvent) was added to the copolymer dissolved in THF or CS2. The films were obtained on glass substrate by spin-coating (250 rpm/18 s and 1500 rpm/60 s) at a relative humidity of 75%. This procedure was conducted in a Darwin Chamber model PH9-DA at 25°C.
3. Results and discussion
The diblock copolymers were characterized by 1 H-NMR, E.A, FTIR spectroscopy, and their thermal and optical properties were determined. The morphological properties were investigated by optical and SEM microscopy.
3.1. Copolymer composition
The copolymer molar fraction was calculated from the N/C ratio using elemental analysis data (content of N). Additionally, this result was corroborated by 1 H-NMR spectroscopy. According to the elemental analysis the following experimental data were obtained: Experimental values of nitrogen (N%), carbon (C%), hydrogen (H%) are the following for AP-1: N% = 2.554; C% = 67.603; H% = 8.992. For HPP-1 are: N% = 3.012; C% = 62.055; H% = 17.893 (see Table ). The copolymer composition determined by using 1 H-NMR spectroscopy was obtained from the proton signal attributed to tBA monomer unit [1.2–1.6 ppm, 9 H, -CH3, 3 groups] in AP-1, and for HPMA ([0.10 ppm, 9 H, -CH3, 3 groups]; at 0.91 ppm [9 H, -CH3, 3 groups] in HPP-1. The intensity ratio of these with the signals of aromatic ring protons of N-PhMI (5 H), from 6.7 to 7.7 ppm, were obtained see Table .
The FTIR spectrum for both AP-1 and HPP-1 block copolymers exhibited characteristic strong absorption bands at approximately 3500 cm−1 [υ(-OH) from -COOH], and 3438 cm−1 [υ(-OH from HPMA)]; at 2900 and 2850 cm−1 [υ(CH, CH2)]; at 1713.96 cm−1[υ(-C = O from N-PhMI)]; at 1633.7 and 1597.68 cm−1[υ(-C = O, ester from HPMA)]; 1389.8 cm−1 [υ(N-C, imide ring]; 846.31 and 625.26 cm−1 [υ(aromatic ring)] (see Figure ).
3.2. Thermal and optical properties
The thermal stability of the block copolymers was studied by TGA (Figure (a,b)). The thermograms reveal degradation in two-steps for AP-1 and HPP-1. The first step with a TDT1 at 250°C, has a weight loss of about 10%, which was attributed at the mass-loss of tert-butyl groups that were not completely hydrolyzed. A second pronounced step TDT2 was observed at 350°C and was attributed to the decomposition process of the block copolymer (see Figure ).
The absorption spectra of the block copolymers exhibited similar electronic properties at room temperature, only one band in the UV region. This could be due to the effective interaction of the lateral functional groups (aromatic ring;—COOH; -OH). Further supporting this, it is necessary to point out that all the polymers showed a dark red-purple color in liquid and solid state, probably due to the electrons delocalization on its structure. The maximum absorption bands determined by UV-Vis, in THF was recorded at 238 nm and 245 nm for AP-1 and HPP-1, respectively (see Figure ). This band can be assigned to the π¯π* transition of the phenyl ring of N-PhMI block. It is possible to observe, for HPP-1 block copolymer, a red shift of the absorption band as compared with that of AP-1. This shift of the band could be attributed to the different domain particle size. The results of the luminescence spectra of the AP-1 and HPP-1 block copolymers showed a maximum emission band at λmax 480 nm and 500 nm respectively, at an excitation wavelength of 320 nm.
3.3. Morphological properties
The morphology of the polymeric films was examined by SEM. Typical micrograph images showed a porous morphology (see Figure ). Furthermore, the upper surface films layer displayed a porous structure with a spherical and straight cylinder-like morphology.
3.4. Formation of porous polymer films or polymer spheres: effect of the concentration and solvent
The formation of porous polymer films via BF method has been reported in the literature (Stenzel et al., Citation2006, Citation2003; Stenzel-Rosenbaum et al., Citation2001; Yunus et al., Citation2007). In this sense, and concerning to the applicability of porous structures, the control and design of the chemical functionality is crucial. The use of other polymers with different nature and functionalities can improve or introduce additional optical o thermal properties (Bolognesi et al., Citation2008; Jin et al., Citation2010; Ke et al., Citation2010; Stenzel et al., Citation2006, Citation2003; Stenzel-Rosenbaum et al., Citation2001; Yunus et al., Citation2007). The porous film formation using BF method based on the precipitation of the amphiphilic polymers around the water droplets implies the orientation of the hydrophilic groups preferably to the wall of the cavities (Bolognesi et al., Citation2008; Jin et al., Citation2010). An excess of water in the solution can produce microspheres instead of porous films, and a very high humidity in the polymer solution also promotes the coexistence of porous films and microspheres (Ke et al., Citation2010).
In this context, the compositions of acrylic derivate and N-PhMI monomers are referred as M1 = AA/HPMA and M2 = N-PhMI for the linear diblock copolymers. In this caseƒM1 is higher (75%) than ƒM2, (25%), then segment M1 takes up a larger volume than M2 domain, and the packing geometry can be regarded as cone-like, coming together to form a spherical micelle with the M2 phase dispersed in the M1 phase.
In this work, the surface morphological properties were determined by optical micrograph images, and SEM microscopy varying experimental conditions of concentrations (3.0 and 5.0 g L−1) and solvents (in CS2 and THF), at an evaporation time of 1 h, at a relative humidity of 75%, and 25°C.
The optical microscopic images in Figure (from a-l) displayed differences in the morphological properties due to self-assembling processes for AP-1 and HPP-1 systems. This was attributed to the different molecular structure of the hydrophilic co-domains which, due to the location of the polar functional groups within the pores, could interact in different ways with water molecules.
According to this procedure, two series of porous films using the BF method were prepared. In the first series, the polymer concentration effect (C = 3.0 and 5.0 g L−1 in THF) of both copolymer was studied, at the same temperature, time and humidity conditions. As results, the surface films showed circular and spherical shaped nanostructures for both block copolymers Figuress , for AP-1 and HPP-1, respectively, at C = 3 g L−1 and Figures (b,e), at 5 g L−1 (two-droplet of water/1 mL solvent) on glass substrate. SEM images were performed for AP-1 and HPP-1 at C = 5 g L−1 (Figure respectively) which exhibit similar behavior at optical microscopic images.
In the second series, CS2 was used as solvent at the same polymer concentrations (3 and 5 g L−1), temperature, time and humidity on the formation of the porous polymer-structured films. It is known that the pore shape can be affected by solvent density (Wan et al., Citation2012). Comparing the density of the THF and CS2 with respect to water (d = 1.00 g mL−1), THF has a smaller density (d = 0.889 g mL−1) but exhibit a higher miscibility with the water than CS2. The CS2 has a higher density (d = 1.28 g mL−1) that the water and exhibit a lower miscibility that the THF. In view of the above, CS2 were successfully utilized for preparing the structured films on glass substrate. In this way, the second series was carried out for AP-1 (Figure ) and HPP-1 (Figure ) at C = 3 g L−1 and at 5 g L−1 for AP-1 (Figure ) and HPP-1 (Figure ), respectively.
The AP-1 and HPP-1 block copolymer in CS2 exhibited different morphologies which varies from aggregates, individual spherical micelles to porous structured films depending on the polymer concentration. For example, the AP-1 at 3.0 g L−1 in CS2 exhibited porous structure films (Figure ) and at higher concentration exhibited aggregates as well as large spherical micelles compounds (Figure ). SEM images for AP-1 and HPP-1 at 5 g L−1 in CS2 were performed, see Figure (i and l, respectively). Notably, the different solvents and concentration affected the structured morphology. Moreover, the porous structure film for HPP-1 block copolymer in CS2 displayed a higher pore definition, with size diameters between 500 and 900 nm, than in THF with diameters between 100 and 500 nm, see Figure (f,l), respectively.
Figure 4. Optical (first three columns) and scanning electron microscopy (last column) pictures of porous films prepared for AP-1 block copolymer at 3 g L−1 (4.a) and 5 g L−1 (4.b) in THF, and at 3 g L−1 (4.g) and 5 g L−1 (4.h), in CS2 and for HPP-1 block copolymer at C = 3 g L−1 (4.d) and 5 g L−1 (4.e) in THF and at 3 g L−1 (4.j) and 5 g L−1 (4.k), in CS2 on glass substrate at relative humidity 75%
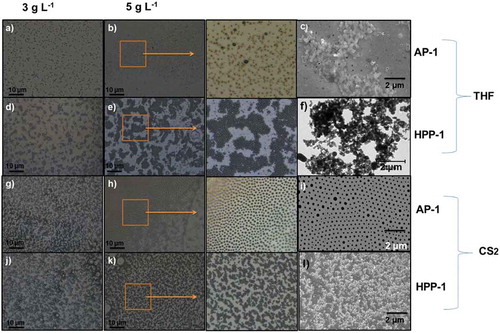
The SEM images of the AP-1 and HPP-1 diblock copolymers in THF, as solvent, at 3.0 g L−1, prepared by adding water (two-droplet/mL of organic solvent) showed large-compound spherical micelles with an average size between 300 and 800 nm (Figure (a–c))
3.5. Conclusions
The SEM microscopy reveal that the block copolymers tended to self-assemble, showing spherical structure as large spherical micelles compounds in THF and tended to form porous-structured films with an average pore diameter size ranging from 300 to 800 nm. On the other hand, these materials exhibited self-assembled organization with a size ranging from 100 to 500 nm in THF and from 500 to 900 nm in CS2 by optical microscopic images. The SEM image of the upper surface layer of the block copolymer films exhibited from large micelles-spherical aggregates (or individual micelle-like morphology), to porous-structured films. Moreover, the results of the photoluminescence spectra of the block copolymers showed single-photon absorption maxima at λmax 420 and 500 nm respectively, at the excitation wavelength of 310 nm. The absorption behavior of the block copolymer was indicative of the effective conjugation from the lateral groups of the polymer chains (N-PhMI and—COOH/-OH functional groups) influencing the self-assembly process.
Acknowledgements
The authors acknowledge the financial assistance of this work by VRAC grant number L217-15 and L217-21 of the Universidad Tecnológica Metropolitana, JS thanks FONDECYT 1191336 Project. D. Oyarzun thanks Plan de Mejoramiento Institucional– CD InES of UAB, Iniciativa Científica Milenio (ICM) del Ministerio de Economía.
Additional information
Funding
Notes on contributors
Guadalupe del C. Pizarro
Prof. Guadalupe del C. Pizarro, PhD, graduated as Chemist, Universidad de Chile, Santiago (Chile), and she obtained her Ph.D. in Macromolecular Chemistry (1994) from University of Concepción (Chile) with the Prof. Dr. Bernabé L. Rivas (University of Concepción-Chile) and Prof. Dr. K.E. Geckeler (University of Tübingen- Germany). Her main research areas are synthesis and characterization of polymeric materials with environment applications and hybrid structured films with nanoparticles for smart applications. Since 1998 she got a permanent position as full professor and researcher at the Faculty of Natural Sciences, Mathematics and Environment of Technological Metropolitan University (Santiago, Chile).
Pizarro, G del C. Since 1998 she got a permanent position at Technological Metropolitan University as full professor, where she is heading the nanostructured material research’s group. Her research interest include synthesis, characterization, design and application of new functional materials and polymeric materials. Also, her research covers hybrid structured optical materials.
References
- Beers, K. L., Boo, S., Gaynor, S. G., & Matyjaszewski, K. (1999). Atom transfer radical polymerization of 2-hydroxyethyl methacrylate. Macromolecules, 32(18), 5772. https://doi.org/10.1021/ma990176p
- Bolognesi, A., DiGlanvincenzo, P., Giovanella, U., Mendechi, R., & Schieroni, A. G. (2008). Polystyrene functionalized with EDOT oligomers. European Polymer Journal, 44(3), 793.https://doi.org/10.1016/j.eurpolymj.2007.12.002
- Cava, M., Deano, H., & Muth, K. (1961). Organic Syntheses, 41, 93.
- Coca, S. J., Jasieczek, C. B., Beers, K. L., & Matyjaszewski, K. (1998). Polymerization of acrylates by atom transfer radical polymerization. Homopolymerization of 2-hydroxyethyl acrylate. Journal of Polymer Science A, 36(9), 1417. https://doi.org/10.1002/()1099-0518
- Davis, K. A., & Matyjaszewski, K. (2000). Atom transfer radical polymerization of tert -butyl acrylate and preparation of block copolymers. Macromolecules, 33(11), 4039. https://doi.org/10.1021/ma991826s
- Davis, K. A., Paik, H.-J., & Matyjaszewski, K. (1999). Kinetic investigation of the atom transfer radical polymerization of methyl acrylate. Macromolecules, 32(6), 1767. https://doi.org/10.1021/ma9815051
- De León, A. S., Muñoz-Bonilla, A., Fernández-García, M., & Rodríguez-Hernádez, J. (2012). Breath figures method to control the topography and the functionality of polymeric surfaces in porous films and microspheres. Journal of Polymer Science A, 50(5), 851. https://doi.org/10.1002/pola.v50.5
- Jin, J., Wan, L. S., Ke, B. B., & Xu, Z. K. (2010). Progress in Chemistry, 22, 2173.
- Ke, -B.-B., Wan, L.-S., & Xu, Z.-K. (2010). Controllable construction of carbohydrate microarrays by site-directed grafting on self-organized porous films. Langmuir, 26(11), 8946. https://doi.org/10.1021/la904729b
- Kim, S., Lee, J., Jeon, S.-M., Lee, H. H., Char, K., & Sohn, B.-H. (2008). Orientation of lamellar nanostructures in the patterned thin films of a diblock copolymer. Macromolecules, 41(10), 3401. https://doi.org/10.1021/ma800584t
- Li, C., Gu, C., Zhang, Y., & Lang, M. (2012). Synthesis and self-assembly of pH-responsive amphiphilic poly(ε-caprolactone)-block-poly(acrylic acid) copolymer. Polymer Bulletin, 68(1), 69. https://doi.org/10.1007/s00289-011-0520-1
- Liu, J.-H., & Chiu, Y.-H. (2010). Behaviors of self-assembled diblock copolymer with pendant photosensitive azobenzene segments. Journal of Polymer Science A, 48(5), 1142. https://doi.org/10.1002/pola.v48:5
- Lonsdale, D. E., Whittaker, M. R., & Monteiro, M. J. (2009). Self-assembly of well-defined amphiphilic polymeric miktoarm stars, dendrons, and dendrimers in water: The effect of architecture. Journal of Polymer Science A, 47(22), 6292. https://doi.org/10.1002/pola.v47:22
- Matyjaszewski, K., Wang, J.-L., Grimaud, T., & Shipp, D. A. (1998). Controlled/“Living” atom transfer radical polymerization of methyl methacrylate using various initiation systems. Macromolecules, 31(5), 1527. https://doi.org/10.1021/ma971298p
- Moineau, G., Minet, M., Dubois, P., Teyssié, P., Senninger, T., & Jérôme, R. (1999). Controlled radical polymerization of (Meth)acrylates by ATRP with NiBr2(PPh3)2as catalyst†. Macromolecules, 32(1), 27. https://doi.org/10.1021/ma980995u
- Mori, H., Wakisaka, O., Hirao, A., & Nakahama, S. (1994). Protection and polymerization of functional monomers, 23. Synthesis of well-defined poly(2-hydroxyethyl methacrylate) by means of anionic living polymerization of protected monomers. Macromolecular Chemistry and Physics, 195(9), 3213. https://doi.org/10.1002/macp.1994.021950918
- Mühlebach, A., Gaynor, S. G., & Matyjaszewski, K. (1998). Synthesis of amphiphilic block copolymers by Atom Transfer Radical Polymerization (ATRP). Macromolecules, 31(18), 6046. https://doi.org/10.1021/ma9804747
- Müller-Buschbaum, L., Schulz, E., Metwalli, J. F., & Moulin, R. C. Langmuir. 2009, 25,4235.
- Pizarro, G. D. C., Marambio, O. G., Jeria-Orell, M., Flores, M. E., & Rivas, B. L. (2010). Amphiphilic diblock copolymers poly(2-hydroxyethylmethacrylate)-b-(N-phenylmaleimide) and poly(2-hydroxyethylmethacrylate)-b-(styrene) using the macroinitiator poly(HEMA)-Cl by ATRP: Preparation, characterization, and thermal properties. Journal of Applied Polymer Science, 118(6), 3649. https://doi.org/10.1002/app.32353
- Pizarro, G. D. C., Marambio, O. G., Jeria-Orell, M., & Geckeler, K. E. (2013). Self-assembled nanostructures: Preparation, characterization, thermal, optical and morphological characteristics of amphiphilic diblock copolymers based on poly(2-hydroxyethyl methacrylate-block-N -phenylmaleimide). Polymer International, 62(10), 1528. https://doi.org/10.1002/pi.2013.62.issue-10
- Pizarro, G. D. C., Marambio, O. G., Jeria-Orell, M., Oyarzún, D. P., & Geckeler, K. E. (2016). Size, morphology and optical properties of ZnO nanoparticles prepared under the influence of honeycomb-porous poly[(2-hydroxyethylmethacrylate)m-block-poly(N-phenyl maleimide)n] copolymer films. Materials & Design, 111, 513. https://doi.org/10.1016/j.matdes.2016.09.036
- Pizarro, G. D. C., Marambio, O. G., Jeria-Orell, M., Oyarzún, D. P., Martin-Trasanco, R., & Sanchez, J. (2017). Monitoring morphological and optical properties on hybrid porous polymer films. International Journal of Polymer Analysis and Characterization, 22(8), 741. https://doi.org/10.1080/1023666X.2017.1370804
- Pizarro, G. D. C., Marambio, O. G., Jeria-Orell, M., Oyarzún, D. P., & Sanchez, J. (2018). Hybrid polymer films based ZnS nanocomposites and its optical and morphological properties: Monitoring the role of the binding-site interaction. Materials Research Bulletin, 98, 15. https://doi.org/10.1016/j.materresbull.2017.10.002
- Stenzel, M. H., Barner-Kowollik, C., & Davis, T. P. (2006). Formation of honeycomb-structured, porous films via breath figures with different polymer architectures. Journal of Polymer Science A, 44(8), 2363. https://doi.org/10.1002/()1099-0518
- Stenzel, M. H., Davis, T. P., & Fane, A. G. (2003). Honeycomb structured porous films prepared from carbohydrate based polymers synthesized via the RAFT process. Journal of Materials Chemistry, 13(9), 2090. https://doi.org/10.1039/b304204a
- Stenzel-Rosenbaum, M. H., Davis, T. P., Fane, A. G., & Chen, V. (2001). Porous polymer films and honeycomb structures made by the self-organization of well-defined macromolecular structures created by living radical polymerization techniques. Angewandte Chemie International Edition, 40(18), 3428. https://doi.org/10.1002/()1521-3773
- Wan, L.-S., Li, J.-W., Ke, -B.-B., & Xu, Z.-K. (2012). Ordered microporous membranes templated by breath figures for size-selective separation. Journal of the American Chemical Society, 134(1), 95. https://doi.org/10.1021/ja2092745
- Wang, Y., Finlay, J., Callow, M. E., Betts, D. E., Callow, J. A., Merkel, T. J., Luft, J. C., & De Simone, J. M. (2011). Amphiphilic co-networks with moisture-induced surface segregation for high-performance nonfouling coatings. Langmuir, 27(17), 10365. https://doi.org/10.1021/la202427z
- Widawski, G., Rawiso, M., & Francois, B. (1994). Self-organized honeycomb morphology of star-polymer polystyrene films. Nature, 369(6479), 387. https://doi.org/10.1038/369387a0
- Xia, J., Zhang, X., & Matyjaszewski, K. (1999). Synthesis of star-shaped polystyrene by atom transfer radical polymerization using an “Arm first” approach. Macromolecules, 32(13), 4482. https://doi.org/10.1021/ma9900378
- Yunus, S., Delcote, A., Poleunis, C., Bertrand, P., Bolognesi, A., & Botta, C. (2007). A route to self-organized honeycomb microstructured polystyrene films and their chemical characterization by ToF-SIMS imaging. Advanced Functional Materials, 17(7), 1079. https://doi.org/10.1002/adfm.v17:7
- Zhang, W., He, J., Liu, Z., Ni, P., & Zhu, X. (2010). Biocompatible and pH-responsive triblock copolymer mPEG-b-PCL-b-PDMAEMA: Synthesis, self-assembly, and application. Journal of Polymer Science A, 48(5), 1079. https://doi.org/10.1002/pola.v48:5
- Zhang, W., Zhang, W., Zhou, N., Zhu, J., Cheng, Z., & Zhu, X. (2009). Synthesis of miktoarm star amphiphilic block copolymers via combination of NMRP and ATRP and investigation on self-assembly behaviors. Journal of Polymer Science Part A: Polymer Chemistry, 47(22), 6304. https://doi.org/10.1002/pola.v47:22
- Zhou, X. D., Zhang, S. C., Huebner, W., Ownby, P. D., & Gu, H. (2001). Journal of Materials Science, 36(15), 3759. https://doi.org/10.1023/A:1017982018651