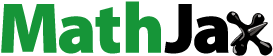
Abstract
Preservation of banana through drying is a recommended method for improving the shelf life. In this study, an experimental and theoretical evaluation of a developed forced-convection indirect solar drying system with a double-pass solar collector (DPSC) was undertaken. Experimentation using banana slices of 4 mm thickness was carried out. The DPSC achieved an optimal peak outlet temperature of 72°C with a maximum operational efficiency of 72.5%. A computational fluid dynamic (CFD) model was developed for prediction of the dryer temperature and 3D airflow distribution within the dryer unit using ANSYS 18.2. The CFD model was validated using experimental data and good agreement was achieved. The developed dryer demonstrated improved efficiency over similar dryers, and this is attributable to the unique arrangement of component parts. Dried banana chips were evaluated for quality through taste, color and shape and the results indicated good quality products. This was attributed to the pretreatment of banana chips with lemon juice prior to drying.
Keywords:
PUBLIC INTEREST STATEMENT
This work presents a solar dryer that can process ripe and unripe banana to extend shelf life. The dryer does not require any form of electricity. Experiments using sliced banana indicated that the dryer has a performance efficiency of 72.5%. A computational analysis of the dryer carried out in ANSYS showed agreement with the experimental setup. The dryer can be used to process banana chips while preserving the taste, the attractive color and without significant shrinkage in shape. This can be achieved by dipping the sliced banana chips in lemon juice for about 10 minutes before introducing them into the dryer. Dried banana can either be consumed as dry chips, ground as flour or easily rehydrated for use in preparing other desired food. It can also be used for the preparation of animal feed. This dryer will help reduce or eliminate wastage of banana due to ripening.
1. Introduction
The consumption rate of fruits is very high in Botswana, like in many other countries worldwide. Fruits are naturally highly perishable hence requiring some form of preservation to prolong their shelf life. Botswana, located in Southern Africa, is largely dependent on the importation of fruits from neighboring countries amounting to millions of Pula annually (DAFF, South Africa, Citation2014; IMTS, Citation2016). Botswana has about 258,520 square kilometers of agricultural land, which represents 45.6% percent of the total land area. Botswana’s agricultural sector employs about 29.9% of its total labor force and accounts for about 3% of the country’s GDP (Statistics Botswana, Citation2015). Livestock production in Botswana dominates the whole of the agricultural sector while crop production is very low due to the agro-ecological conditions which are unsuitable for cropping.
The rate of consumption of banana is very high in Botswana, including in processed form. Most of the banana consumed in Botswana are imported from neighboring countries including South Africa and Mozambique, amounting to millions of Pula annually. Averagely, 64% of banana exported from South Africa ends up in Botswana (FAO Statistical Yearbook, Citation2013). In 2017, Botswana imported banana fruits and plantains from Mozambique amounting to about USD 81.32 thousands (FAO Statistical Yearbook, Citation2013). Physical injury to banana is common and is attributable to the long transit involved in the importation and the overstaying or failure of the market to consume the product within its shelf life. This results in very high post-harvest losses (Kumari & Kumar, Citation2018; Kuyu & Tola, Citation2018). Banana is highly fibrous and contains nutrients like carbohydrates, vitamins, and natural sugar. It is also free from cholesterol (Bello-Pérez & Agama-Acevedo, Citation2019; Kuyu & Tola, Citation2018; Omolola et al., Citation2015; Pappu et al., Citation2015).
Banana fruits have a very short shelf life, hence can be stored for only few days. Ripening is usually accelerated by elevated ambient temperatures (Du et al., Citation2016; Zhu et al., Citation2018), and this is a huge challenge to countries like Botswana that could receive very high ambient temperatures from solar radiations. However, this challenge can be used as an advantage towards preserving banana from spoilage through methods such as ozonation and biofilm coatings. Processed banana can be used in many various ways. Dried banana products can be consumed as banana flakes, milled to banana flour and/or used to make banana cake, bread, biscuits, baby food and many other products. In addition, banana fruit and its peels can be dried, milled and used as animal feed, particularly in Botswana where the agricultural sector is dominated by livestock farming, ultimately reducing biomass waste.
Drying is commonly known as the process of removal of an amount of water in a product in order to reduce or stop microbial activities. There are various methods for drying agricultural produce including forced convection solar drying, which is one of the artificial drying methods. Due to its performance and proven record of drying different products, forced convection solar drying is an appropriate drying technology that can be adopted in Botswana, in-line with Botswana’s vision 2036 that directs the country towards the ultimate use of natural resources in transforming the economy (Botswana, Citation2016). Forced convection solar drying is fast as a large amount of products can be dried within a short time with satisfactory quality of end product (Babu et al., Citation2018). In addition, Botswana is endowed with abundant solar insolation potential to fulfil thermal energy demand.
The design and development of a solar dryer requires different evaluations, from mathematical and numerical points of view. During design, the solar collector area and its configurations, temperature and airflow recirculation, dryer dimensions and volume of drying chamber must be evaluated properly in order to achieve maximum heat transfer effects (Alam & Kim, Citation2017; Sanghi et al., Citation2018). Improvement of parameters during the design of solar dryer components is usually an attempt to optimize drying of agricultural produce.
Computational fluid dynamics (CFD) helps to carry out the study of heat transfer during the design stage of a dryer. A significant number of literature is available on experimental and CFD simulation of forced convective solar dryers (Arun, Srinivas et al., Citation2020; Chauhan et al., Citation2015; Malekjani & Jafari, Citation2018). A work on active drying of unripened banana using a multi-tray mixed-mode solar cabinet dryer with back-up energy storage highlighted a novel tray sequencing pattern for multi-tray mixed-mode solar cabinet drying. The multi-tray system achieved average pickup of 21.4%, a drying efficiency of 37.8% and approximate cost of drying of 0.35 USD/kg of unripened banana, while drying characteristic curves revealed that with tray sequencing the final moisture content was reduced below 16% (db) in all the trays from an initial moisture content of 180% (db) (Arun et al., Citation2019). Similarly, investigating drying uniformity and flexibility in product selection, Arun, Kunal et al. (Citation2020) proposed a tray sequencing approach that aided to achieve drying uniformity for banana flakes within 10 h and bitter gourd by 18 h, hence leading to energy utilization ratio of between 45.3% and 47.9%. In another work, Raj et al. (Citation2019) undertook a CFD odeling of a macro-encapsulated latent heat storage system towards addressing the impact of elementary heat transfer aiding to phase transition of phase change material (PCM) enclosed within a thin cylindrical macro-encapsulate. The study concluded that only 27% of the PCM portion from the bottom of the macro-encapsulate underwent complete melting for conduction assisted heat transfer, while over 70% of the PCM underwent complete melting within the stipulated time for a combined mode. However, aggregate information for researchers who wish to carry out studies in CFD modeling of a 3D forced-convective solar dryer, with a DPSC using ANSYS-CFD software is lacking.
This work presents experimental and numerical analyses of drying of banana using a forced convective solar dryer with a DPSC suitable for the climatic conditions of Botswana. A unique feature includes the simultaneous evaluation of the physical phenomena of both the double-pass solar collector and the drying chamber. Botswana is a sub-tropical country with low solar availability during the winter and very high solar availability during the summer. All year round solar drying of agricultural produce in this climatic zone could be challenging. Therefore, this study focuses on designing, developing and evaluating a forced convective solar dryer suitable for the climate of Botswana, simulating a 3D computational fluid dynamic model of the developed solar dryer as well as validating temperatures and airflow distributions using experimental data obtained.
2. Evaluation of a double-pass solar collector (DPSC)
2.1. Energy balance
Using the principle of energy conservation, the energy balance and the thermal performance of a double-pass solar collector can be evaluated. It can be described by analyzing the airflow pattern between the upper and lower channels independently (Ho et al., Citation2018; Kareem, Habib, Ruslan et al., Citation2017; Ravi & Saini, Citation2016; Singh & Dhiman, Citation2016). Figure shows the energy balance of the top and bottom glasses, upper and lower channels and the absorber plate of a DPSC subjected to radiative and convective heat effects, and the resultant relationships are expressed in EquationEquations 1(1)
(1) –Equation4
(4)
(4) .
2.2. Thermal performance
The thermal performance of a solar collector is investigated by evaluating its efficiency, which is a measure of the available energy from the solar radiation that can be harnessed by the flowing air under steady-state conditions as shown in EquationEquations 5(5)
(5) –Equation7
(7)
(7) (Kareem, Habib, Sopian et al., Citation2017; Singh & Dhiman, Citation2016).
where FR is the collector heat removal factor and is the collector efficiency.
2.3. CFD model development
A 3D computational domain of both a DPSC and drying chamber units was modeled separately. This separation was decided in order to be computationally efficient and to simplify the complexity and application of different turbulence models to the units. The CFD modeling is implemented based on the well-known application of the laws of conservation of mass, momentum and energy.
2.4. Turbulence modeling
Forced convection systems usually encounter turbulences due to the use of forced mechanisms. The evaluation of turbulences was carried out through the use of turbulence models, to account for the viscous and inertia forces (Malekjani & Jafari, Citation2018). A number of turbulence models are currently available and have been used for different studies (Demissie et al., Citation2019; Gawande et al., Citation2016; Tegenaw et al., Citation2019). The choice of an appropriate turbulence model depends on various factors such as flow behavior—swirling or strained flows, complexity of the problem to be solved in terms of geometry and structure, computational cost and accuracy.
3. Developed DPSC and experimentation
3.1. System description
Figure illustrates the solar dryer developed at the University of Botswana while Figure shows the detailed component parts of the developed solar dryer. The DPSC consists of a top and bottom glass of 5 mm thickness each and 0.18 W/mK thermal conductivity, absorber plate aluminium sheet of 1.6 mm thickness with five fins and 210 W/mK thermal conductivity, a connector aluminium foil into the drying chamber, a recirculation fan powered by a 15 W solar panel, and a polyethylene foam insulation of 12 mm thickness and 0.02–0.027 W/mK thermal conductivity. The drying chamber is made of plywood insulated with a 16 mm polyethylene foam of 0.1–0.2 W/mK thermal conductivity. The collector dimensions are 1.7 m x 0.8 m x 0.188 m for length, width and height, respectively, and has a surface area of 1.36 m2. The drying chamber is the tray type having a total internal volume of 0.16 m3 with length, width and volume of 0.648 m x 0.468 m x 0.528 m, respectively. The system features a unique arrangement, as the drying chamber is underneath the double-pass solar collector, and the solar collector itself can be adjusted between an angle of 0⁰ and 35⁰ for ease of sun tracking and use in different latitudes.
3.2. Drying of banana fruits
Fresh ripe banana fruits were purchased from the local market in Gaborone a day before the experiment. The fruits were cleaned, manually peeled and sliced into chips of 4 mm thickness. Each slice of banana was pretreated by dipping it into lemon juice for 10 minutes before the introduction into the drying chamber. Pretreated banana slices achieve the best rehydration rate compared to other treatment options, hence enables the banana slices to retain color and taste (Abano & Sam-Amoah, Citation2011). Remote data collection using data loggers was carried out in-situ during drying experiments over the period of 8–10 hours each day. Initial moisture content was determined using samples of banana slices in a standard oven drying procedure (Reeb & Milota, Citation1999). Sliced banana fruits were then loaded to the drying chamber for drying experimentation (Figure ). To improve air circulation within the drying chamber, a 15 W solar panel powered fan was incorporated into the drying chamber. Air velocity increased as solar availability improves through the day. A digital anemometer was used to measure wind velocity variation within the drying chamber throughout the drying time.
3.3. CFD modeling of a DPSC and drying chamber flow domain
CFD models were developed using ANSYS 18.2 workbench. A 3D flow domain of a DPSC and drying chamber was modeled with actual dimensions as in Figures and , while Table shows the mesh parameters and properties of the DPSC. Based on the mesh quality assessment and grid independency test, the DPSC flow domain was discretized into small grid elements having skewness of less than 0.57 which was assumed to be suitable for the simulation.
Table 1. Properties and mesh metric parameters of a DPSC and drying chamber
3.4. Numerical modeling of developed double-pass solar collector
The collector is the main source of heat for solar drying of agricultural produce, including banana slices. The collector consists of three main parts—two glasses, absorber plate and the wooden cabinet. The fluid domain of the solar collector was modeled in the ANSYS design model following exactly the internal wall geometry of the developed solar collector. The model catered for hot air blowing through the system from the inlet to the outlet holes.
3.4.1. The geometry and airflow of solar collector
Following the experimental model of the solar collector, a 3D geometry of a solar collector having an air inlet hole, top & bottom glasses, absorber plate together with outlet hole as shown in Figure was modeled using ANSYS 18.2 academic version software. Airflow started from the inlet holes, passed through the top channel and then entered the bottom channel with a U-turn motion downwards to the surface of the solar absorber. Airstream entering the bottom channel from the top channel was heated up by the absorber plate.
3.4.2. Meshing of solar collector
Meshing is the discretization of flow domain into a number of small elements and is shown in Figure ) for the solar collector. Using body and edge meshing techniques, the mesh refinement was applied to the critical zones and sharp corners of the collector flow domain, Figure ). Mesh quality was monitored through the element quality, skewness and the aspect ratio, as in Figure . The element size of 0.001 mm, having a tetrahedral shape for the individual edges and body meshing, was created while the inlet and outlet element size of 0.002 mm was used. The total number of elements of 3,816,337, with nodes of 746,255, was generated in order to enhance the convergence of the simulation. The sizes and number of elements were chosen to be more desirable in order to account for the laminar sub-layers along and near the walls of the solar absorber, therefore having better prediction of the internal flow. Fine meshes of high density were also applied to all boundaries such as the inlet and outlet, the region on top of the absorber plate and the corners of the solar collector geometry.
3.4.3. Fluent setup and boundary conditions of a solar collector
To account for viscous flow and momentum turbulences, the RNG k-ε turbulence model was used. The RNG k-ε turbulence model incorporates a model that calculates the effect of sunlight entering the computational domain of the solar collector. This utility helped to model the location of the sun in a specific day of the year by providing the global location, date, sunshine factor and domain orientation. During experimentation, the solar collector was subjected to solar heat from solar radiations. Therefore, in CFD Fluent settings, the energy equation and the Discrete Transfer radiation Model (DTRM) were energized for simulating the heat transfer due to the solar radiations. The solar load calculator was energized for the geographical location of the University of Botswana (24.67 S and longitude 25.92°E), the experimental site. Due to the position of the collector in ANSYS modeler, the grid orientation for North was set in +y axis and East in -x axis and default fair weather condition was selected. Given that the developed solar dryer is the forced convection type with an axial fan powered by a 15 W solar panel, the heat equations were energized in transient state to cater for turbulence. The physical system includes a solar collector consisting of a top and bottom glazing glass, therefore the material properties for both glasses were set as semi-transparent materials of 0.85 transmissivity and 0.96 absorptivity for the CFD setup. The same applied to the absorber plate, where all properties of aluminum were selected from the database of the Fluent. Simulation input parameters are as in Table .
Table 2. Some input parameters for the solar collector simulation
The convergence criteria for the turbulence k-ε, x, y & z velocity components, continuity and energy equations were monitored by setting the residuals as in Table .
Table 3. Convergence criteria of the collector CFD simulation
4. Results and discussion
4.1. Experimental results
From an initial average moisture content of 75–82% (w.b), dried banana chips achieved moisture removal up to an average of 20% final moisture content. Figure ) shows the inlet and outlet temperatures of the DPSC. The maximum outlet temperature of 345 K occurred at 13:00, 14:00, 15:00 hours Botswana time-zone, while the absorber plate temperatures at the respective times were 375 K, 368 K and 367 K. The graph in Figure ) shows the absorber plate temperature, top and bottom glass temperatures around the DPSC.
The efficiency of a solar collector depends on the mass flow rate, inlet temperature and outlet temperature. From the experiments, the efficiency of the system increased with an increase in mass flow rates (Figure )). The maximum efficiency attained by the DPSC was 72.5%. Figure ) illustrates the percentage of the useful heat gained by the moving air against the heat collected from the sun. In 7 hours of drying out of 8−10 hours in a day, a minimum of 50% and maximum of 69% of the heat collected by the DPSC were absorbed by the air stream within the dryer for the drying process.
Figure 11. (a) Effect of mass flow rate on collector efficiency. (b) Percentage of useful heat gained.
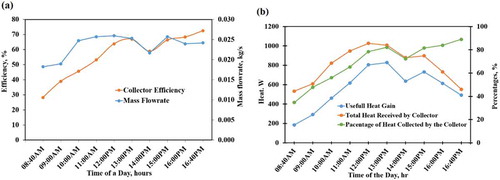
The achieved maximum instantaneous efficiency of the double-pass solar collector is 72.5% which is 3.5% and 10% high than that reported by (Mahmood et al., Citation2015; Pramanik et al., Citation2017), recorded to be 69% and 62.5%, respectively. The developed DPSC also showed better efficiency compared to (Raj et al., Citation2019) and (Ravi & Saini, Citation2016) which achieved 64.08% and 58.73%, respectively. However, the achieved efficiency of the DPSC was within the values as reported by (Fudholi et al., Citation2013), which was recorded to be between 70−80%.
4.2. CFD results and model validation
A study of the temperature profile and evaluation of Nusselt number were used to compare the experimental and theoretical results of the DPSC.
4.2.1. Temperature and velocity profiles of a DPSC
Figure ) shows the temperature contour of the top of the absorber plate while Figure ) shows the consistent temperature distribution on the top glass of the solar collector. Table shows a comparison and variation of temperatures at various points (A to F) on the absorber plate and the top glass of the DPSC.
Figure 12. (a). Temperature pattern on the absorber plate surface. (b). Temperature pattern on the top glass.
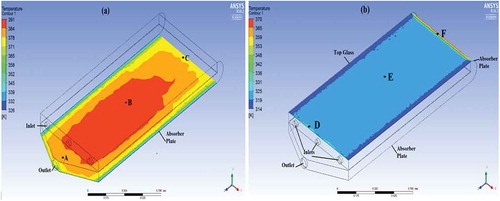
Table 4. Comparative temperature distributions on absorber plate and top glass from the CFD modeling and experiment results
Table shows that there is a very close agreement between the experimental results and the CFD analysis. This validates the theoretical model (Karim & Hawlader, Citation2005; Khalloufi et al., Citation2009; Rahman et al., Citation2016).
4.2.2. Nusselt and Reynolds numbers characteristics
The values of Nusselt number at different Reynolds numbers for the CFD simulation correlated with experimental data obtained using the Dittus-Boelter equation (Figure ). This presentation further validates the experimental approach.
4.3. Quality assessment of dried banana
The quality assessment of the dried banana chips was determined using the color and taste. While changes in dried agricultural products are inevitable, the extent of change of parameters like size, shape, color, aroma or taste determines the quality of the final food product (Kumar et al., Citation2014).
4.3.1. Color and taste of banana flakes
Physical observation of the dried banana chips showed that they maintained radiant color close to the original color of the chips before drying (Figure ). This is attributable to the pretreatment with lemon juice. Similarly, a consumption taste check performed on the chips indicated excellent taste. Dried chips were also checked for the extent of preservation when sealed. Sealed bags had lifespan beyond 3 months and retained a radiant color and taste (Figure ).
4.3.2. Shrinkage of banana flakes
During the drying of banana slices using the developed solar dryer, shrinkage behavior was also investigated. Physical observation of the dried banana chips showed slight changes in shape. It was observed that the dried slices had slight shrinkages, which are expected due to moisture removal. The DPSC drying temperature was maintained within levels appropriate for drying banana chips. It is important to dry agricultural produce within their tolerable temperature range, to ensure the quality of the end product.
5. Conclusion
A drying experiment on banana chips was carried out using the developed DPSC. A CFD simulation using ANSYS was carried out and results from both experimental and theoretical approaches compared for correlation. From the experiments conducted, the maximum efficiency of the solar collector was obtained to be 72%. This shows that the DPSC dryer can generate enough drying temperature for even other products with higher moisture content other than banana fruits. The heat storage gap underneath the absorber plate helped to improve the efficiency of the collector and prevented the rapid drop of the outlet temperature of the solar collector during periods of low sunshine. Similarly, the quality of dried banana fruits remained high and this is attributable to the pretreatment of the fruits with lemon juice before the commencement of the drying process.
Notations
Table
Acknowledgements
Authors acknowledge the Office of Research and Development (ORD), the University of Botswana for supporting this work through funding.
Additional information
Funding
Notes on contributors
Kevin N. Nwaigwe
Philemon X. Mutabilwa holds a master's degree in Mechanical Engineering from the University of Botswana. He is currently a faculty at Mbeya University of Science and Technology (MUST). This article is part of his research work, under the supervision of Dr Nwaigwe.
Kevin N. Nwaigwe holds a PhD in Mechanical Engineering, in the Energy and Power specialization. He is a researcher with the Clean Energy Research Centre, University of Botswana, and a Faculty in the Department of Mechanical Engineering, University of Botswana. He serves as the Botswana technical lead for SOLTRAIN IV project, a solar thermal initiative in Southern Africa. He has supervised over nine (9) graduate students, including the co-author. His research is focused on renewable energy technology and the current work is harnessing solar energy resource for food processing. He has authored over 50 scholarly publications, including a registered patent.
References
- Abano, E. E., & Sam-Amoah, L. K. (2011). Effects of different pretreatments on drying characteristics of banana slices. ARPN Journal of Engineering and Applied Sciences, 6(3), 121–18.
- Alam, T., & Kim, M.-H. (2017). Performance improvement of double-pass solar air heater—A state of art of review. Renewable and Sustainable Energy Reviews, 79, 779–793. https://doi.org/10.1016/j.rser.2017.05.087
- Arun, K. R., Kunal, G., Srinivas, M., SujithKumar, C. S., Mohanraj, M., & Jayaraj, S. (2020). Drying of untreated Musa nendra and Momordica charantia in a forced convection solar cabinet dryer with thermal storage. Energy, 192, 116697. https://doi.org/10.1016/j.energy.2019.116697
- Arun, K. R., Srinivas, M., Saleel, C. A., & Jayaraj, S. (2019). Active drying of unripened bananas (Musa nendra) in a multi-tray mixed-mode solar cabinet dryer with backup energy storage. Solar Energy, 188, 1002–1012. https://doi.org/10.1016/j.solener.2019.07.001
- Arun, K. R., Srinivas, M., Saleel, C. A., & Jayaraj, S. (2020). Influence of the location of discrete macro-encapsulated thermal energy storage on the performance of a double pass solar plate collector system. Renewable Energy, 146, 675–686. https://doi.org/10.1016/j.renene.2019.07.036
- Babu, A., Kumaresan, G., Raj, V. A. A., & Velraj, R. (2018). Review of leaf drying: Mechanism and influencing parameters, drying methods, nutrient preservation, and mathematical models. Renewable and Sustainable Energy Reviews, 90, 536–556. https://doi.org/10.1016/j.rser.2018.04.002
- Bello-Pérez, L. A., & Agama-Acevedo, E. (2019). Banana and mango flours. In Flour and breads and their fortification in health and disease prevention (pp. 153–164). Elsevier.
- Botswana, G. O. (2016). Vision 2036 achieving prosperity for all. P. T. Team, Ed., First published 2016 ed. Lentswe la Lesedi (PTYY) Ltd. [email protected] or [email protected]
- Chauhan, P. S., Kumar, A., & Tekasakul, P. (2015). Applications of software in solar drying systems: A review. Renewable and Sustainable Energy Reviews, 51, 1326–1337. https://doi.org/10.1016/j.rser.2015.07.025
- DAFF, South Africa. (2014). A profile of the South African banana market value chain. Retrieved October 21, 2019, from http://www.nda.agric.za
- Demissie, P., Hayelom, M., Kassaye, A., Hailesilassie, A., Gebrehiwot, M., & Vanierschot, M. (2019). Design, development and CFD modeling of indirect solar food dryer. Energy Procedia, 158, 1128–1134. https://doi.org/10.1016/j.egypro.2019.01.278
- Du, L., Song, J., Forney, C., Palmer, L. C., Fillmore, S., & Zhang, Z. (2016). Proteome changes in banana fruit peel tissue in response to ethylene and high-temperature treatments. Horticulture Research, 3(1), 16012. https://doi.org/10.1038/hortres.2016.12
- FAO Statistical Yearbook. (2013). World food and agriculture. Retrieved October 21, 2019, from http://www.fao.org/3/i3107e/i3107e00.htm
- Fudholi, A., Sopian, K., Othman, M. Y., Ruslan, M. H., & Bakhtyar, B. (2013). Energy analysis and improvement potential of finned double-pass solar collector. Energy Conversion and Management, 75, 234–240. https://doi.org/10.1016/j.enconman.2013.06.021
- Gawande, V. B., Dhoble, A., Zodpe, D., & Chamoli, S. (2016). Experimental and CFD-based thermal performance prediction of solar air heater provided with right-angle triangular rib as artificial roughness. Journal of the Brazilian Society of Mechanical Sciences and Engineering, 38(2), 551–579. https://doi.org/10.1007/s40430-015-0391-8
- Ho, C.-D., Chang, H., Hsiao, C.-F., & Huang, -C.-C. (2018). Device performance improvement of recycling double-pass cross-corrugated solar air collectors. Energies, 11, 338.
- IMTS. 2016 March. International merchandise trade statistics monthly digest. Retrieved October 21, 2019, from http://www.statsbots.org.bw
- Kareem, M., Habib, K., Ruslan, M. H., & Saha, B. B. (2017). Thermal performance study of a multi-pass solar air heating collector system for drying of Roselle (Hibiscus sabdariffa). Renewable Energy, 113, 281–292. https://doi.org/10.1016/j.renene.2016.12.099
- Kareem, M., Habib, K., Sopian, K., & Ruslan, M. (2017). Multi-pass solar air heating collector system for drying of screw-pine leaf (Pandanus tectorius). Renewable Energy, 112, 413–424. https://doi.org/10.1016/j.renene.2017.04.069
- Karim, A., & Hawlader, M. (2005). Drying characteristics of banana: Theoretical modelling and experimental validation. Journal of Food Engineering, 70(1), 35–45. https://doi.org/10.1016/j.jfoodeng.2004.09.010
- Khalloufi, S., Almeida-Rivera, C., & Bongers, P. (2009). A theoretical model and its experimental validation to predict the porosity as a function of shrinkage and collapse phenomena during drying. Food Research International, 42(8), 1122–1130. https://doi.org/10.1016/j.foodres.2009.05.013
- Kumar, C., Karim, M., & Joardder, M. U. (2014). Intermittent drying of food products: A critical review. Journal of Food Engineering, 121, 48–57. https://doi.org/10.1016/j.jfoodeng.2013.08.014
- Kumari, P., & Kumar, S. (2018). A study on post-harvest losses and constraints in banana cultivation in Vaishali district (Bihar). The Pharma Innovation Journal, 7(6), 93–95.
- Kuyu, C. G., & Tola, Y. B. (2018). Assessment of banana fruit handling practices and associated fungal pathogens in Jimma town market, SouthWest Ethiopia. Food Science & Nutrition, 6(3), 609–616. https://doi.org/10.1002/fsn3.591
- Mahmood, A., Aldabbagh, L., & Egelioglu, F. (2015). Investigation of single and double pass solar air heater with transverse fins and a package wire mesh layer. Energy Conversion and Management, 89, 599–607. https://doi.org/10.1016/j.enconman.2014.10.028
- Malekjani, N., & Jafari, S. M. (2018). Simulation of food drying processes by computational fluid dynamics (CFD); recent advances and approaches. Trends in Food Science & Technology, 78, 206–223. https://doi.org/10.1016/j.tifs.2018.06.006
- Omolola, A. O., Jideani, A. I., & Kapila, P. F. (2015). Drying kinetics of banana (Musa spp.). Interciencia, 40, 374–380.
- Pappu, A., Patil, V., Jain, S., Mahindrakar, A., Haque, R., & Thakur, V. K. (2015). Advances in industrial prospective of cellulosic macromolecules enriched banana biofibre resources: A review. International Journal of Biological Macromolecules, 79, 449–458. https://doi.org/10.1016/j.ijbiomac.2015.05.013
- Pramanik, R. N., Sahoo, S. S., Swain, R. K., Mohapatra, T. P., & Srivastava, A. K. (2017). Performance analysis of double pass solar air heater with bottom extended surface. Energy Procedia, 109, 331–337. https://doi.org/10.1016/j.egypro.2017.03.077
- Rahman, M. M., Mekhilef, S., Saidur, R., Mustayen Billah, A. G. M., & Rahman, S. M. A. (2016). Mathematical modelling and experimental validation of solar drying of mushrooms. International Journal of Green Energy, 13(4), 344–351. https://doi.org/10.1080/15435075.2014.952425
- Raj, A. K., Srinivas, M., & Jayaraj, S. (2019). CFD modeling of macro-encapsulated latent heat storage system used for solar heating applications. International Journal of Thermal Sciences, 139, 88–104. https://doi.org/10.1016/j.ijthermalsci.2019.02.010
- Ravi, R. K., & Saini, R. P. (2016). A review on different techniques used for performance enhancement of double pass solar air heaters. Renewable and Sustainable Energy Reviews, 56, 941–952. https://doi.org/10.1016/j.rser.2015.12.004
- Reeb, J., & Milota, M. (1999). Moisture content by the oven-dry method for industrial testing. WDKA. Retrieved June 20, 2020, from https://ir.library.oregonstate.edu/downloads/jm214q048
- Sanghi, A., Ambrose, R. K., & Maier, D. (2018). CFD simulation of corn drying in a natural convection solar dryer. Drying Technology, 36(7), 859–870. https://doi.org/10.1080/07373937.2017.1359622
- Singh, S., & Dhiman, P. (2016). Thermal performance of double pass packed bed solar air heaters—A comprehensive review. Renewable and Sustainable Energy Reviews, 53, 1010–1031. https://doi.org/10.1016/j.rser.2015.09.058
- Statistics Botswana. (2015). Botswana population projections (2011 – 2026). Retrieved October 21, 2019, from http://www.statsbots.org.bw
- Tegenaw, P. D., Gebrehiwot, M. G., & Vanierschot, M. (2019). On the comparison between computational fluid dynamics (CFD) and lumped capacitance modeling for the simulation of transient heat transfer in solar dryers. Solar Energy, 184, 417–425. https://doi.org/10.1016/j.solener.2019.04.024
- Zhu, X., Luo, J., Li, Q., Li, J., Liu, T., Wang, R., Chen, W., & Li, X. (2018). Low temperature storage reduces aroma-related volatiles production during shelf-life of banana fruit mainly by regulating key genes involved in volatile biosynthetic pathways. Postharvest Biology and Technology, 146, 68–78. https://doi.org/10.1016/j.postharvbio.2018.08.015