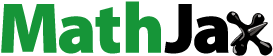
Abstract
South Africa is a water-scarce nation, and this has resulted to the use of groundwater as one of the alternative sources. Owing to the low socio-economic status of some communities in rural areas, where modern water purification equipment is not available, groundwater is consumed without prior treatment. Siloam Village, Limpopo Province, is one of such communities; however, the presence of excess fluoride in groundwater has led to the occurrence of fluorosis, which constitutes a health concern. Vermiculite which is a clay mineral is highly available in Limpopo Province and has not been exploited for defluoridation; thus, this study was aimed at the defluoridation of groundwater in Siloam Village using vermiculite modified with hexadecyltrimethylammonium, a cationic surfactant with antimicrobial properties. The clay mineral was activated with NaCl and subsequently modified by agitating with the surfactant in a 5%w/v solution. The pzc of the modified vermiculite was deduced to be 6.4 while that of the control vermiculite was 9.7. Using groundwater from sampling points GW 1 and GW 2 for defluoridation studies, the results show 22.27% and 20.72% removal while a maximum of 49.4% was obtained from a 5 mg/L laboratory simulated fluoride solution. The effect of competing anions especially CO32-, as well as the low electro-positivity on the surface of the modified vermiculite reduced the fluoride sorption potential of the adsorbent. Thus, the use of vermiculite modified with hexadecyltrimethylammonium is not a very suitable option for solving the problem of fluorosis in rural environments.
PUBLIC INTEREST STATEMENT
High fluoride in groundwater is a global phenomenon that has led to health complications such as dental and skeletal fluorosis. This occurs mostly in rural communities where groundwater is the only source of drinking water. Some of the existing methods are expensive, ineffective and are not applicable in rural communities. Hence, Siloam Village (with fluoride level of about 5.6 mg/L) in Limpopo Province of South Africa is a rural area, which relies on groundwater for household use due to inadequacy of alternative sources. The use of HDTMA-VMT for defluoridation of groundwater from Siloam Village showed a removal of 22% and 20%, respectively, while the Control-VMT showed 0% removal. The reduction in percentage removal was attributed to the alkaline pH of field water coupled with competition for adsorbent site by other anionic components. It can be deduced from this study that the use of HDTMA-VMT for defluoridation has shown potential which can be developed by additional research into a domestic defluoridation unit.
1. Introduction
The availability of clean potable water is a challenge that is faced by many nations of the world. This has led to the use of groundwater from wells and borehole sources as means of survival especially in environments with low socio-economic status. According to Blignaut and Van Heerden (Citation2009), South Africa is a water-stressed country. This has warranted the country’s reliance on alternative sources including untreated groundwater, which also generally contain elements such as (Fe), manganese (Mn), copper (Cu), zinc (Zn), calcium (Ca) and magnesium (Mg) as well as anions such as sulphate (SO42-), chloride (Cl−), fluoride (F−), nitrate (NO3−) and cyanide (CN−), in various concentrations (Cox, Citation1964). Fluoride, one of the inorganic components of groundwater has significant effects on various aspects of human health. Consumption of fluoride above 1.5 mg/L (World Health Organisation, Citation2004) and 1 mg/L (Department of Water Affairs and Forestry (DWAF), Citation1996) is considered harmful and could give rise to dental fluorosis. Dental fluorosis involves the destruction of enamel and discolouration of the teeth from white to yellow and to brown. Teeth commonly affected by fluorosis involve central incisors, lateral incisor and molars (Durowoju et al., Citation2015; Tewari & Dubey, Citation2009). This is usually characterised by symptoms such as dullness of teeth, loss of shine and yellow to brown streak on the dental enamel (Saksena & Narwaria, Citation2012). Cases of skeletal fluorosis have also been reported, where bones are weakened, causing pain and damage to the joint due to high fluoride (Ibrahim et al., Citation2011). According to World Health Organisation (Citation2004), consumption of water containing fluoride of 3 mg/L to 6 mg/L could be responsible for skeletal fluorosis.
Studies from different continents of the world show the presence of groundwater fluoride at medium to high concentrations. In Mexico (South America), fluoride has been discovered at 5.9 mg/L in Meoqui city, North of Mexico City (Armienta & Segovia, Citation2008). In Ohio State (North America), fluoride is present between 2 and 4 mg/L (Ohio EPA., Citation2012). In Ethiopia (Africa), fluoride range in groundwater from 7.8 to 18 mg/L (Rango et al., Citation2012). In India (Asia) fluoride is available in groundwater up to 30 mg/L (Sharma et al., Citation2011). In Australia, fluoride has been discovered in the range of 3 mg/L to 9 mg/L while in Polland (Europe) fluoride ranges from 0.8 to 1.2 mg/L in Środa Wielkopolska (Czajka-Jakubowska, Citation2009). Issues of fluorosis in South Africa have been recorded, debated and discussed since 1935 (Louw & Chikte, Citation1997) and in 1941, three incidents of human fluorosis were reported in various regions in the former Union of South Africa (McCaffrey & Willis, Citation2001). In more recent times, fluoride has been reported in North West (8 mg/L), Kwazulu-Natal (8 mg/L) and Western Cape Provinces (4 mg/L) (Ncube & Schutte, Citation2005). These concentrations are above the WHO and DWAF’s standard values of 1.5 and 1 mg/L respectively and can be responsible for fluorosis (Ncube & Schutte, Citation2005).
Siloam Village, located in Makhado Municipality, Limpopo Province of South Africa, is a community with low economic status that uses groundwater as its major source of drinking without prior treatment. A study by Odiyo and Makungo (Citation2012) showed that out of the 87% of households that use groundwater, 85% have members with mottled teeth which is a major symptom of fluorosis. The study also revealed that children within the ages of 11 to 14 years in Siloam primary school had mottled teeth while the concentration of fluoride in groundwater was found to be 5.6 mg/L. This relatively high amount of fluoride in water above the DWAF standard of 1 mg/L necessitates its reduction to permissible limits, as constant consumption of fluoride-rich water can cause health complications.
The prevalence of this health defect in South Africa has led to the exploration of adsorption technique as an effective and economic defluoridation option. Examples of materials (clay minerals) used as adsorbents include kaolinite, bauxite, palygoskite, bentonite and laterite (Coetzee et al., Citation2003). Further study by Coetzee et al. (Citation2003) showed that South African clays containing high amount of gibbsite or aluminum oxide have the best fluoride removal potential; thus, from the study, the highest fluoride removal was recorded using bauxite.
Vermiculite, a clay mineral with natural deposit at Phalaborwa, Limpopo Province South Africa, has, however, not been actively exploited for its defluoridation properties. This fact coupled with the morbidity of fluorosis in Siloam Village, and other rural communities in South Africa motivated this research. Vermiculite is a clay mineral originating from the mica group. It is generally composed of hydrous magnesium aluminum layers interpolated by layers of H2O (França et al., Citation2005). The structure is composed of two tetrahedral sheets attached to a central octahedral sheet; hence, this structure is called a 2:1 phyllo-silicate (Dizadji et al., Citation2013). It is expressed as dioctahedral Mx(Al2−yFe43+)(Si4−xAlx)O10(OH)2•nH2O or trioctahedral Mx(Mg3+)(Si4−xAlx)O10(OH)2•nH2O vermiculite (Shichi & Takagi, Citation2000). Al3+ substitution for Si4+ in the tetrahedral layers and Mg2+ or Fe2+ substitutions for Al3+ in the octahedral layers are responsible for the overall negative charge of the structure (Hongo et al., Citation2012). Thus, the clay mineral requires adequate modification to enhance anionic attraction.
Several clay minerals have been modified using inorganic agents such as H2SO4 (Gogoi & Baruah, Citation2008), Titanium (Das et al., Citation2005) and aluminum (Oladoja & Helmreich, Citation2014; Vhahangwele et al., Citation2014). Recently organic cations such as hexadecyltrimethylammonium bromide (HDTMABr), 3-aminopropyltriethoxysilane (silane), octadecylamine (ODA) and dodecylamine have been used to prepare organoclays (Motawie et al., Citation2014) which can be used to adsorb anions from solution. The use of hexadecyltrimethylammonium in this study was on the basis that it has successfully been used for the removal of anions such as chromates, nitrates and oxyanions from solution (Aroke et al., Citation2014; Li et al., Citation2003; Onyango et al., Citation2010). Subsequently, antimicrobial effects, such as cellular lysis for Escherichia choli (Ishikawa et al., Citation2002), growth inhibition for Staphylococcus aureus (El Haddad et al., Citation2012) and change in cell surface charge from negative to positive for Candida albicans (Vieira & Carmona-Ribeiro, Citation2006) have been reported for HDTMA, thus suggesting its use as a decontaminant. This study was therefore aimed at using vermiculite modified with hexadecyltrimethylammonium for fluoride removal from groundwater.
2. Materials and methods
2.1. Groundwater sampling and analysis
Groundwater samples were taken from two publicly accessible borehole sites from Siloam Village (Figure ). At the point of sampling, physicochemical properties of the water such as pH, temperature and electrical conductivity were measured. Polyethylene bottles, which had been pre-washed with detergent, dilute HNO3 and deionised water, were rinsed with the groundwater from the source to avoid cross contamination before sampling and transporting to the laboratory for analysis. The samples were analysed for their ionic composition using ICP-MS (Agilent 7700 series, United States of America) while defluoridation studies (batch adsorption) were carried out on the samples using controlled and modified vermiculite. The amount of fluoride in each test solution was measured using the fluoride meter of an ion-selective electrode.
2.2. Preparation of the adsorbent
Superfine grade of vermiculite used in this study was supplied by Mandoval vermiculite, Johannesburg. All reagents used in this study was of analytical grade and were prepared using deionised water. The vermiculite was milled to ≤250 µm and was activated with NaCl by agitating 5 g of clay mineral with 50 mL (1:10) of 1 M NaCl solution for 1 hour at room temperature (Motawie et al., Citation2014). The excess chloride was washed by centrifuging with deionised water until chloride was confirmed absent when the washings were tested with AgNO3; subsequently, the activated vermiculite was dried at 105°C for 6 hours. And, 0.2 M of HDTMABr was prepared by dissolving 1.4578 g of the salt in 20 mL of deionised water. A weighed quantity of the activated clay and deionised water was prepared in 5 %w/v solution into which 20 ml of 0.2 M HDTMABr was added. The solution was agitated in a temperature-controlled shaker at 40°C for 24 hours, after which the modified clay was washed of any bromide with deionised water by centrifuging. The pH of the modified adsorbent was further reduced to make the surface more electropositive by continuous addition of 0.1 M HCl to the solution of the bromide washed adsorbent, until the pH of the solution reached the desired value. The adsorbent was thus dried for 6 hours at 105°C and labelled as HDTMA-VMT while the unmodified adsorbent was labelled Control-VMT
2.3. Point of zero charge (pHpzc)
The point of zero charge (pHpzc) of the HDTMA-VMT was carried out using the method described in Hu et al. (Citation2014). Aliquots of 45 mL of 0.01 M NaCl were measured into seven 50 mL bottles. The pH was adjusted from 4 to 10 (using 0.1 M HCl/NaOH) and the remaining volume made up to 50 mL with the 0.01 M NaCl solution. A mass of 0.15 g of the samples (HDTMA-VMT) was added to each solution, shaken and left to stand for 24 hours after which the equilibrium (final) pH was measured. The change in pH (ΔpH) was calculated by subtracting the equilibrium pH from the initial pH.
The batch adsorption technique was carried out on the groundwater samples using the optimum parameters determined when HDTMA-VMT was used for fluoride adsorption from laboratory simulated solution. The conditions for optimum performance using HDTMA-VMT included 2 g adsorbent dosage, 70 minutes contact time, pH 6.5, 200 rpm and 25°C which had been reported in a previous study by Ologundudu et al. (Citation2016). These parameters were used for defluoridation of the groundwater samples.
3. Quality assurance
This study was carried out by maintaining a high level of sensitivity while weighing and cleanliness while using glassware. Deionised water was used to prepare all reagents, thus preventing contamination. TISAB III buffer was used to prevent complexing of metal ions such as iron and aluminium with fluoride, which could affect fluoride measurement using fluoride ion-selective electrode. However, due to the rigorous nature of the experimental procedure, the following steps and indicators (in the subsections that follow) were also carefully observed during analysis to ensure the quality of this study. Each step of the analysis using HDTMA-VMT (which is the test adsorbent) was carried out using a control. This enabled the determination of the actual performance of HDTMA-VMT, thus emphasising credible conclusions.
4. Results and discussion
The characteristics of the absorbent were fully described by Ologundudu et al. (Citation2016). The study reported that the FTIR analysis shows that HDTMA-VMT reveals the adsorption sites and reduction in transmittance especially after defluoridation. Also, the SEM of HDTMA-VMT after defluoridation generally reveals that the surface appears to be more pre-occupied than those of the Control-VMT (Ologundudu et al., Citation2016). The pzc of both Control-VMT and HDTMA-VMT are described in Figures and . The pHpzc was plotted as ΔpH against initial pHo (El Haddad et al., Citation2012; Hu et al., Citation2014).
For the Control-VMT, the pzc follows a straight line until it intersects the horizontal axis at 9.7 which gives the pzc for the Control-VMT (Figure ). This suggests that the Control-VMT would weakly attract anions because the pzc is in the alkaline region (Smith, Citation1999). Consequently, analysis for HDTMA-VMT showed a pzc of 6.4 (Figure ); this implies a reduction of 3.3 units, from the pzc of the Control-VMT. The pzc of 6.4 would thus support fluoride removal because the surface of the clay is acidic enough to attract fluoride via electrostatic attraction.
A study by Ologundudu et al. (Citation2016) reported that optimised conditions and kinetic of adsorption for fluoride sorption efficiency of vermiculite modified with HDTMA which is 2 g adsorbent dosage, 70 min contact time, solution pH of 4, and room temperature (25°C) guaranteed 51.13% fluoride removal from an 8 mg/L fluoride solution with a maximum adsorption capacity of 2.36 mg/g.
Laboratory synthesised fluoride solution was also used as test water samples and the result presented in Table . The table presents percentage fluoride removal, which lies within the range of 46–49.4% for 2–7 mg/L. Above 5 mg/L, fluoride removal began to reduce (Figure ); therefore, HDTMA-VMT was more effective for concentrations within the range of 2–5 mg/L.
Table 1. Adsorption using HDTMA-VMT with laboratory fluoride solution
The results of defluoridation of the groundwater samples using HDTMA-VMT and Control-VMT are presented in Table . The results of groundwater defluoridation for sampling points GW1 and GW2 show adsorptions of 22.27% and 20.72%, respectively, for HDTMA-VMT. The Control-VMT, however, yielded 0% adsorption.
Table 2. Defluoridation of GW I and GW2
This non-adsorption observed in the case of the Control-VMT can be attributed to the absence of the modifying agent which is responsible for the defluoridation effect of the HDTMA-VMT. No adsorption was also observed for the control because the pzc was in the alkaline region where there would be no attraction between fluoride and the surface of the adsorbent.
Compared to the laboratory fluoride solution which yielded as high as 49.4% removal (using HDTMA-VMT) the reduction in adsorption to 22.27% (GW 1) and 20.72% (GW 2), using groundwater is not abnormal since it contains several anions which compete with fluoride for adsorbent sites. Table shows physicochemical parameters of GW 1 and GW 2 before and after defluoridation. Besides the presence of competing anions in groundwater, the initial pH of the medium especially GW 2 was alkaline and not suitable for defluoridation. The reduction in defluoridation efficiency of the adsorbents due to high pH and competing anions was also observed by Kamble et al. (Citation2007).
Table 3. Physicochemical parameters of GW 1 and GW 2 before and after defluoridation with HDTMA-VMT
The concentration of CO32- before defluoridation in Table was shown to reduce after defluoridation thus highlighting CO32- as the major ion preventing fluoride adsorption in this study. CO32- has harmful effect on fluoride uptake and this behaviour can be explained by the high affinity of carbonate ions on the absorbent surface. Interestingly, there is an increase in some competing anions such as NO3−, Cl−, SO42- and PO43- after defluoridation. It could be attributed to dissolution of the vermiculite minerals under alkaline pH resulting to release of free radicals into the water. These competing anions (NO3−, Cl−, SO42- and PO43-) do not impact on fluoride removal in the groundwater and a similar result was obtained by Nouria et al. (Citation2019). However, CO32- greatly compete (Table ) with fluoride adsorption resulting to lower fluoride removal in the groundwater. The percentage removal using HDTMA-VMT did not exceed 50% because the adsorbent did not possess enough electropositivity. This could be attributed to the pzc of HDTMA-VMT which was slightly below the neutral point (weakly electropositive) as well as the overall charge of the organic cation (equivalent to 1).
Comparing HDTMA-VMT with other used adsorbents from literature such as Kanaujia et al. (Citation2015), Hanumantharao et al. (Citation2011), Salim et al. (2014), Rayappan et al. (Citation2014), and Rani et al. (Citation2012) among others, it has low efficiency in fluoride removal in groundwater. Table shows some of the reduction effects of competing anions on defluoridation from literature. This table presents the effects of competing anions in the groundwater; hence, this accounts for the reduction in the percentage fluoride removal in groundwater compared to the laboratory fluoride solution.
Table 4. Comparison of defluoridation between laboratory fluoride solution and field groundwater
5. Conclusions
Only fluoride removal percentages of 22.27% and 20.72% were obtained using GW 1 and GW 2 while a maximum of 49.4% was obtained from a 5 mg/L laboratory fluoride solution. This brings into question the effectiveness of the use of HDTMA-VMT as a viable adsorbent for solving the problem of fluorosis in Siloam Village. Besides offering antimicrobial properties, the single (+1) charge of HDTMA does not impart enough electro-positivity to the surface of the clay mineral, thus weakening its fluoride sorption potential. The presence of competing anions especially CO32- also greatly compromised adsorption. Thus, further study should focus on identifying better organic/inorganic modifying agents compactible with vermiculite which would improve on fluoride removal from groundwater in Siloam Village. The effect of competing ions should also be considered in such studies and steps should be taken to annul their negative effect. Also, the use of HDTMA-VMT can be developed into a domestic defluoridation unit but will require further research on how to increase its efficiency as well as regeneration after use.
Conflicts of interest
The authors declare no conflict of interest.
Acknowledgements
The authors would like to appreciate the University of Venda, Research and Publications Committee (RPC) for funding this research.
Additional information
Funding
Notes on contributors
Tayo O. Ologundudu
Ologundudu Tayo Oladipo, MSc, holds MSc. in Environmental Sciences. His research interests focus on groundwater including Hydrochemistry, Water Quality and Remediation among others.
Olatunde S. Durowoju
Durowoju Olatunde Samod*, PhD, holds PhD in Environmental Sciences with specialisation in Environmental geochemistry and Health. His research focus includes Hydrogeochemistry and Isotope hydrology, Environmental geochemistry and Health, Biogeochemistry and Ecohydrology, Water Quality and Remediation within environmental media.
John O. Odiyo
Odiyo John Ogony, PhD, holds PhD in Civil Engineering with specialisation in Water Engineering. His research focus includes hydrology, hydrogeology, environmental media including soils, air and water and wastewater resources and remediation, water quality and water treatment, water resources management and open channels hydraulics.
Georges-Ivo E. Ekosse
Ekosse Georges-Ivo Ekosse PhD, DTech, FAAS, holds PhD in Soil Mineralogy and Doctor of Technology in Environmental Health and is Senior Professor of Applied Clay Mineralogy and Environmental Geology. His research covers clays and clay minerals, human and enzootic geophagia, extraction metallurgy, environmental geochemistry, natural and artificial porous materials, and reconstruction of paleoenvironments.
References
- Armienta, M. A., & Segovia, N. (2008). Arsenic and fluoride in the groundwater in Mexico. Environmental Geochemistry and Health, 30(4), 345–10. https://doi.org/10.1007/s10653-008-9167-8
- Aroke, U. O., El-Nafaty, U. A., & Osha, O. A. (2014). Removal of oxyanion contaminants from wastewater by sorption onto HDTMA-Br surface modified organo-kaolinite clay. International Journal of Emerging Technology and Advanced Engineering, 4(1), 475–484.
- Blignaut, J., & Van Heerden, J. (2009). The impact of water scarcity on economic development initiatives. Water SA, 35(4), 415–420. https://doi.org/10.4314/wsa.v35i4.76800
- Coetzee, P. P., Coetzee, L. L., Puka, R., & Mubenga, S. (2003). Characterisation of selected South African clays for defluoridation of natural water. Water SA, 29(3), 331–338. http://doi.org/10.4314/wsa.v29i3.4935
- Cox, C. R. (1964). Operation and control of water treatment process. World health organization.
- Czajka-Jakubowska, A. (2009). Effect of the interaction of amelogenin and enamel crystal in clinical and experimental fluorosis. Wydawnictwo Naukowe Uniwersytet Medyczny im. Karola (In Polish).
- Das, N., Pattanaik, P., & Rita, D. (2005). Defluoridation of drinking water using activated titanium rich bauxite. Journal of Colloid and Interface Science, 292(1), 1–10. https://doi.org/10.1016/j.jcis.2005.06.045
- Department of Water Affairs and Forestry (DWAF). (1996). South African water quality guidelines (Vol. 8). Department of water affairs and forestry. field guide (2nd use).
- Dizadji, N., Rashtchi, M., Dehpouri, S., & Nouri, N. (2013). Experimental investigation of adsorption of copper from aqueous solution using vermiculite and clinoptilolite. International Journal of Environmental Research, 7(4), 887–894. https://doi.org/10.22059/IJER.2013.670
- Durowoju, O. S., Odiyo, J. O., & Ekosse, G. E. (2015). Hydro-geochemical setting of geothermal springs in Limpopo province, South Africa – A review. Research Journal of Chemistry and Environment, 19(1), 77–88.
- El Haddad, M., Mamouni, R., Saffaj, N., & Lazar, S. (2012). Removal of a cationic dye-basic Red 12- from aqueous solution by adsorption onto animal bone meal. Journal of the Association of Arab Universities for Basic and Applied Sciences, 12(1), 48–54. https://doi.org/10.1016/j.jaubas.2012.04.003
- França, S. C. A., Arruda, G. M., & Ugarte, J. F. O. (2005). Vermiculite utilization on the treatment of water contaminated with organic compounds. Enpromer- 2005, 2nd Mercosur Congress on Chemical Engineering.
- Gogoi, P. K., & Baruah, R. (2008). Fluoride removal from water: Adsorption on acid activated kaolinite clay. Indian Journal of Chemical Technology, 15(5), 500–503.
- Hanumantharao, Y., Kishore, M., & Ravindhranath, K. (2011). Preparation and development of adsorbent carbon from acacia farnesiana for defluoridation. International Journal of Plant, Animal and Environmental Sciences, 1(3), 209–223.
- Hongo, T., Yoshino, S., Yamazaki, A., Yamasaki, A., & Satokawa, S. (2012). Mechanical treatment of vermiculite in vibration milling and its effects on lead (II) adsorption ability. Applied Clay Science, 70, 74–78. https://doi.org/10.1016/j.clay.2012.09.016
- Hu, D., Wang, P., Li, J., & Wang, L. (2014). Functionalization of microcrystalline cellulose with N, N-dimethyldodecylamine for the removal of congo red dye from an aqueous solution. Bioresources, 9(4), 5951–5962. https://doi.org/10.15376/biores.9.4.5951-5962
- Ibrahim, M., Asimrasheed, M., Sumalatha, M., & Prabhakar, P. (2011). Effects of fluoride contents in ground water: A review. International Journal of Pharmaceutical Applications, 2(2), 128–134.
- Ishikawa, S., Matsumura, Y., Katoh-Kubo, K., & Tsuchido, T. (2002). Antibacterial activity of surfactants against Escherichia coli cells as influenced by carbon source and anaerobiosis. Journal of Applied Microbiology, 93(2), 302–309. https://doi.org/10.1046/j.1365-2672.2002.01690.x
- Kamble, S. P., Japtap, S., Labhsetwar, N. K., Thakare, D., Godfrey, S., Devotta, S., & Rayau, S. S. (2007). Defluoridation of drinking water using chitin, chitosan and lanthanum modified chitosan. Chemical Engineering Journal, 129(1–3), 173–180. https://doi.org/10.1016/j.cej.2006.10.032
- Kanaujia, S., Singh, B., & Singh, S. (2015). Removal of fluoride from groundwater by carbonised punica granatum carbon (“CPGC”) Bio-Adsorbent. Journal of Geoscience and Environment Protection, 3(4), 1–9.
- Li, Z., Willms, C. A., & Kniolak, K. (2003). Removal of anionic contaminants using surfactants-modified palygorskite and sepiolite. Clay and Clay Minerals, 51(4), 445–451. https://doi.org/10.1346/CCMN.2003.0510411
- Louw, A. J., & Chikte, U. M. E. (1997). Fluoride and fluorosis: The status of research in South Africa. 2nd International workshop on fluorosis prevention and defluoridation of water. In Dahi E, Nielsen JM, (eds), Proceedings of the 2nd International Workshop on Fluorosis and Defluoridation of Water; 1997 Nov 19-25, Nazareth, Ethiopia. International Society for Fluoride Research, 15–22. Dunedin, New Zealand. http://www.defluoride.net/2ndproceedings/15-22.pdf
- McCaffrey, L. P., & Willis, J. P. (2001). Distribution of fluoride rich groundwater in eastern and Mogwase region of the North West provinces, WRC Report no 526/1/01.
- Motawie, A. M., Madany, M. M., El-Dakrory, A. Z., Osman, H. M., Ismail, E. A., Badr, M. M., El-Komy, D. A., & Abulyazied, D. E. (2014). Physico-chemical characteristics of nano-organo bentonite prepared using different organo-modifiers. Egyptian Journal of Petroleum, 23(3), 331–338. https://doi.org/10.1016/j.ejpe.2014.08.009
- Ncube, E. J., & Schutte, C. F. (2005). Relation of dental fluorosis to groundwater fluoride in South Africa. 4th International Workshop on Fluorosis Prevention and Defluoridation of Water, 23–32.
- Nouria, N., Meriem, B., Mustapha, B., Touhami, M., Driss, L., Yahia, H., Antonis, A., & Zorpas Mejdi, J. (2019). Removal of fluoride from groundwater using natural clay (kaolinite): Optimization of adsorption conditions. Comptes Rendus Chimie, 22(2–3), 105–112. https://doi.org/doi.10.1016/j.crci.2018.09.010
- Odiyo, J. O., & Makungo, R. (2012). Fluoride concentrations in groundwater and impact on human health in Siloam Village, Limpopo Province, South Africa. Water SA, 38(5), 731–736. https://doi.org/10.4314/wsa.v38i5.12
- Ohio EPA. (2012). Fluoride in Ohio’s groundwater, Ohio Environmental Protection Agency.
- Oladoja, N. A., & Helmreich, B. (2014). Batch defluoridation appraisal of aluminium oxide infused diatomaceous earth. Chemical Engineering Journal, 258, 51–61. https://doi.org/10.1016/j.cej.2014.07.070
- Ologundudu, T. O., Odiyo, J. O., & Ekosse, G. E. (2016). Fluoride sorption efficiency of vermiculite functionalised with cationic surfactant: Isotherm and kinetics. Applied Sciences, 6(10), 277. https://doi.org/10.3390/app6100277
- Onyango, M. S., Masukume, M., Ochieng, A., & Otieno, F. (2010). Functionalised natural zeolite and its potential for treating drinking water containing excess amount of nitrate. Water SA, 36(5), 655–662. https://doi.org/10.4314/wsa.v36i5.61999
- Rango, T., Kravchenko, J., Atlaw, B., McCornick, P. G., Jeuland, M., Brittany, M., & Vengosh, A. (2012). Groundwater quality and its health impacts: An assessment of dental fluorosis in rural inhabitants of the main Ethiopian rift. Environment International, 43, 37–47. https://doi.org/10.1016/j.envint.2012.03.002
- Rani, B., Maheshwari, R., Chauhan, A. K., & Bhaskar, N. S. (2012). Defluoridation of contaminated water employing brick powder as an adsorbent. International Journal of Science and Nature, 3(1), 78–82.
- Rayappan, S., Jeyaprabha, B., & Prakash, P. (2014). A study on removal of fluoride ions from drinking water using a low- cost natural adsorbent. International Journal of Engineering Research & Technology, 3(4), 1340–1346.
- Saksena, D. N., & Narwaria, Y. S. (2012). Incidence of fluoride in groundwater and its potential health effects in ten villages of Karera block in Shivpuri district, Madhya Pradesh, India. International Journal of Environmental Sciences, 3(3), 1141–1149. doi:10.6088/ijes.2012030133021
- Salim, M. M., Malek, N. A. N. N., Ramli, N. I., Hanim, S. A. M., & Hamdan, S. (2014). Antibacterial activity of CTAB-modified zeolite NaY with different CTAB loading. Malaysian Journal of Fundamental and Applied Sciences, 10(3), 19–33. https://doi.org/10.11113/mjfas.v10n3.267
- Sharma, B. S., Agrawal, J., & Gupta, K. A. (2011). Emerging challenge: Fluoride contamination in groundwater in Agra district, Uttar Pradesh. Asian Journal of Experimental Biological Sciences, 2(1), 131–134.
- Shichi, T., & Takagi, K. (2000). Clay mineral as a photochemical reaction field. Journal of Photochem. Photobiology C: Photochemistry Reviews, 1(2), 113–130. https://doi.org/10.1016/S1389-5567(00)00008-3
- Smith, K. S. (1999). Reviews in economic geology volume 6A and 6B. Environmental geochemistry of mineral deposits, Chapter 7 metal sorption on mineral surfaces: An overview with examples relating to mineral deposits. Society of economic geologist Inc. 9(SEG).
- Tewari, A., & Dubey, A. (2009). Defluoridation of drinking water. Efficacy and need. Journal of Chemical and Pharmaceutical Research, 1(1), 31–37.
- Vhahangwele, M., Gitari, W. M., & Ngulube, T. (2014). Defluoridation of drinking water using Al3+-modified bentonite clay: Optimization of fluoride adsorption conditions. Toxicological and Environmental Chemistry, 96(9), 1294–1309. https://doi.org/10.1080/02772248.2014.977289
- Vieira, D. B., & Carmona-Ribeiro, A. M. (2006). Cationic lipids and surfactants as antifungal agents: Mode of action. Journal of Antimicrobial Chemotherapy, 58(4), 760–767. https://doi.org/10.1093/jac/dkl312
- World Health Organisation. (2004). Fluoride in drinking-water, Background document for development of WHO Guidelines for drinking-water quality.