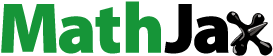
Abstract
This study investigated pozzolan from the co-firing of clay and rice husk. Rice husk was used to replace clay at 1.0%, 1.5% and 2.0% and calcined at 800°C. Pozzolanic strength activity index (PSAI) was performed on the calcined powder materials in accordance with the ASTM C311 standard. The calcined material that obtained the highest PSAI was selected and used to optimize the calcined pozzolan added to Portland cement. The optimum mixture proportions were used for 29Si and 27Al MAS NMR as well as water sorptivity and shrinkage studies which involved autogeneous and drying shrinkages. The results of the study showed that calcined clay that contained 2.0% rice husk had the optimum PSAI whereas 30 wt.% replacement content was the optimum cement replacement. The 29Si and 27Al MAS NMR showed that the superior strength of the optimum mortar mix to the control was due to a stable monosulphate phase formed at the octahedral environment and the formation of extra calcium aluminosilicate phases in the mortar mix. The initial and secondary sorptivity values of the pozzolan were 0.0055 and 0.0022 mm/sec1/2 respectively, lower than the values of the control , which were 0.0299 and 0.0083 mm/sec1/2 respectively. The shrinkage performances of the Portland cement-pozzolan mix were much enhanced than the unblended mortar mix. The inclusion of the co-fired materials reduced the embodied carbon by approximately 29%. The use of the co-fired material could be a sustainable means to dispose of waste rice husk and also reduce embodied carbon.
PUBLIC INTEREST STATEMENT
Waste management is problem for many developed and developing countries. In Ghana, the government has introduced a program called planting for food and jobs. Through this government program, foodstuff as well as waste from food cultivation have increased. A typical waste which has become a burden with their disposal is rice husk. Clay, a resource for producing pozzolan, a material used to reduce cement cost is achieved through heating of the clay. However, ashes of rice husk is also known to be a suitable pozzolan for construction. Due to the properties of the ashes of rice husk, this project added rice husk to clay to produce clay-rice husk pozzolan by heating. The properties of the ashes generated from burning rice husk enhanced the pozzolanic properties of the calcined clay making it a more suitable material to reduce cement usage for mortar and concrete works.
1. Introduction
The consumption natural resources of the earth by human beings and the demand for goods and services to meet their needs is a major contributor to the huge amount of waste generation coupled with its disposal into the environment (Sukholthaman & Shirahada, Citation2015). The quantities of waste generated annually has generally been rising progressively around the globe and this rise is attributed to rapid economic development and urbanization of societies. The generation of waste in developed and emerging countries in the world imposes great economic, social and environmental costs on society through waste collection, treatment and disposal. The increasing waste generation as well as the associated economic, social and environmental impacts have created the opportunity for development and adoption of techniques to reduce waste substantially.
Waste generation from the rice industry has been increasing annually in most West African countries including Ghana, Nigeria, and Togo due to increased consumption resulting from population growth (FAOSTAT Food and Agriculture data, Citation2015). In 2012, rice production in Ghana was about 0.48 million tonnes (MT), which increased to 0.57MT in 2013 and a further 0.6MT in 2014 (FAOSTAT, 2015). Zerbino et al. (Zerbino et al., Citation2011) have stated that on the average, a tonne of rice produced generates approximately 200 kg of rice husk. Currently, approximately 0.15 million tonnes of rice husk is produced in Ghana annually. Disposal of rice biomass in most Western African countries is commonly done by open burning (see Figure ). Uncontrolled or poorly managed burning of rice husk pollutes air quality thereby creating objectionable adverse impact on the environment and on public health and safety. An alternative way to dispose of the waste is for city authorities to collect the waste for proper disposal. The cost of collection of the waste is approximately 10 USD/tonne. Usually, town folks are unwilling to pay this amount thus leaving city authorities with financial stress. This consequently leaves the waste unattended to and hence posing environmental nuisance.
In some emerging and developing economies, waste from rice cultivation is utilized as a useful resource for the construction industry. Rice husk ash is usually produced and used as a pozzolan for the production of concrete and mortar (de Sensale, Citation2006; Zerbino et al., Citation2011). Rice husk has a calorific value between 16 and 17 MJ/kg and could therefore also be used as biofuel. The ashes contain amorphous silica which makes it one of the highly reactive pozzolans (Christopher et al., Citation2017). Clays can also be processed as a pozzolana (Avet et al., Citation2018). However, without heating at appropriate temperatures, pozzolanic properties cannot be activated. Using rice husk as a component in clay can serve as both a heating fuel and a source of elemental silica that can further enrich the pozzolanic nature of the clay. The Figure establishes that best pozzolanic activity is attained when calcined at 800°C (Fernandez et al., Citation2011).
Figure 2. Strength activity index of calcined pozzolan (Fernandez et al., Citation2011).
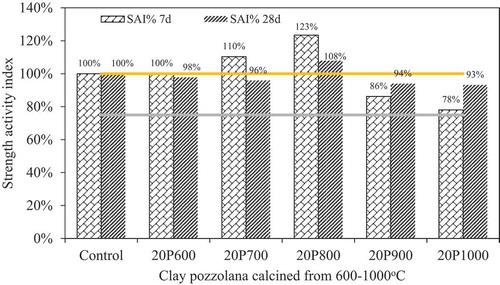
Modern construction has moved from the use of traditional Portland cement to alternative binders. Materials that are commonly used to form alternative binders for construction include fly ash, metakaolins, slag, silica fume and some agricultural waste ashes (Snelling, Citation2016). Assessment of the suitability of pozzolanic binders can be determined through a variety and several experimental techniques. Strength and durability characteristics of binders are important properties that influence the life cycle design of concrete structures.
The use of compressive strength as a mechanical performance to determine the performance of a pozzolan is described by Donatello et al. (Donatello et al., Citation2010) as an indirect method of assessment. Mamlouk and Zaniewski (Mamlouk & Zaniewski, Citation2006) explained that the mechanical behaviour is the material’s response to external load. Indirect methods of testing use parameters such as strength, electrical conductivity and heat evolution by conduction calorimetry to determine the influence of the pozzolan (McCarter & Tran, Citation1996; Paya et al., Citation2001; Shi et al., Citation2005). Luxan (Luxan et al., Citation1989) has indicated that the electrical conductivity and heat evolution methods are less time-consuming. However, the strength activity index determination is more preferable than the other methods. The reason is that the electrical conductivity and heat evolution methods are sensitive to only the pozzolanic effect of powder materials, however, excludes the filler effect whereas Strength Activity Index method is sensitive to both pozzolanic effect and packing effect. Authors including Donatello et al. (Donatello et al., Citation2010) and Tironi et al. (Tironi et al., Citation2013) have used strength activity index to explain pozzolanic activity of pozzolans.
Factors that lead to increased strength performance involve the complex transformation of cementitious systems during hydration. Si NMR provides valuable information relating CSH gel, the main hydrated phases of cement whilst Al NMR is useful to probe calcium aluminate hydrates (CAH) and aluminate hydrates such as ettringite and monosulphate hydrates (Mendess et al., Citation2011). The NMR indicates chemical shifts within ranges and this is a function of bond length and bond valence; all related to polymerization or crystal growth (Mendess et al., Citation2011).
Durability of a product is its ability to maintain the required performance over a given or long time, under the influence of foreseeable action. Foreseeable actions have been explained as potential degradation factors including temperature, humidity, moisture, UV radiation, abrasion, chemical attack, biological attack, weathering and in temperate environment frost, and freeze-thaw (Anon, Citation2015). The United States Department of Housing and Urban Planning has stated that using durable materials also means providing affordable, efficient and sustainable homes (J. Liu et al., Citation2014).
With cement-based materials, moisture penetration through the pores as well as shrinkage effects may prove to be among the significant challenges for concrete and mortar durability. Liu et al. (X. Liu et al., Citation2011) have indicated that the study of transport of water in cement-based materials such as concrete and mortar means a lot for the analysis of concrete durability. This is because usually aggressive substances are carried along in the pores by water. The ingress of moisture and the transport of ions including chlorides, sulphates and other aggressive chemicals have become the underlying source of many engineering problems such as steel corrosion, carbonation, frost and freeze- thaw actions.
Itim et al. (Itim et al., Citation2011) mentioned that shrinkage prediction is of very great essence for durability study and the inclination for long term functioning of concrete structures. Drying shrinkage is the loss of moisture (due to drying) from the concrete or mortar to the environment. Aside drying shrinkage, autogenous shrinkage is also a type of shrinkage that is widely gaining attention. Autogenous shrinkage is caused by a reduction in the pore relative humidity as a result of hydration products formation (Jensen & Hansen, Citation1996). Structural deformation due to shrinkage is well known to be the origin of cracks and even more rarely failure of concrete structures (Hua et al., Citation2017; Tam et al., Citation2012). Pozzolans are widely known as a material suitable for all cement-based products including concrete and mortar (Ballim & Graham, Citation2009; Bediako, Purohit et al., Citation2017; Fernandez et al., Citation2011). It has also been used successfully in refining pore structure and restraining shrinkage of cement-based products (Bao-guo et al., Citation2007; Khatib, Citation2004; Khatib & Hibbert, Citation2005).
This study investigated the mechanical and durability properties of co-fired clay and rice husk. The mechanical studies of the work were based on pozzolanic strength activity index (PSAI) prescribed by the ASTM C311 (ASTM, Citation2002). The PSAI helped in analysing pozzolanic reaction of the co-fired mixtures whereas compressive strength test was used to optimize the co-fired mixture. 29Si and 27Al MAS NMR were used to investigate the hydrates formed from the hydration process involving the Portland cement and the cementitious binder pastes. The durability of the cement system containing the calcined pozzolan was based on sorptivity and shrinkage which included autogenous and drying shrinkages. For sorptivity, the pore system of the cement paste in a matrix is the principal feature that relates to transport of water or sorptivity (Dhandapani et al., Citation2018). Therefore, mortar was formulated and used as a model to understand moisture movement (sorptivity) and shrinkage in concrete. The formulation of mortar was based on the optimum mixture proportion obtained from the results of compressive strength.
2. Materials and methods
2.1. Materials
The materials that were used for the study included Portland cement, clay, water reducer, rice husk, sand and potable water. The Portland cement was an ASTM type 1/11 and was obtained from Ash Grove Cement Company, United States. The clay was obtained from a village, Nyamebekyere in the Ashanti Region of Ghana. Rice husk was obtained from a rice milling plant at Konongo also in Ashanti Region of Ghana. Grade sand that conformed to ASTM C778 (ASTM, Citation2006) was used. A polycarboxylate high range water reducer (HRWR) obtained from BASF, United States was also used. Table shows the chemical and mineralogical compositions of the Portland cement.
Table 1. Properties of Portland cement
2.2. Methods
2.2.1. Preparation and calcination of clay-rice husk mixtures
The clay was preconditioned by drying and milling in a hammer mill to sizes ranging between 75 microns and 2 mm. The rice husk was used to replace the powder clay at 2, 1.5 and 1% by weight and mixture used to formulate pellets of sizes ranging between 1 and 3 mm. The pellets containing rice husk content of 2, 1.5 and 1% were labelled as RH1, RH2 and RH3, respectively. The pellets were placed in a ceramic bowl having a diameter of 120 mm and depth of 90 mm and calcined in a laboratory furnace (Barnstead Thermolyne 6000 furnace) at 800°C for 3 h. The 800°C temperature, was used because the clay obtained from this area of Ghana attained maximum pozzolanic reactivity index at this temperature (Bediako, Kevern et al., Citation2017). After the 3hrs, the ceramic bowl was removed from the furnace and left to cool on a metallic mesh under room temperature. The calcined materials were milled in a laboratory mill and sieved through 75 microns sieve size using a mechanical sieve shaker. The chemical composition of the calcined material (Clay-RH) is shown in Table . Figure shows samples of the calcined clay-rice husk pellets.
2.2.2. Compressive strength and pozzolanic strength activity index
Pozzolanic strength activity index (PSAI) was determined by preparing mortar specimens in accordance with ASTM C109 (ASTM, Citation2007a) standard. The Pozzolanic Strength Activity Index (PSAI) determination was also guided by the ASTM C311 where replacement of Portland cement is specified at 20% by weight. The standard, ASTM C109 (ASTM, Citation2007a) specifies the ratio of cementitious binder to sand at 1:2.75 and a water to cementitious binder ratio at 0.485. Mixing of materials was performed in a Hobart mixer in accordance with ASTM C305 (ASTM, Citation2014a). Flow measurements were performed using the mortar flow table and measurement of the mortar spread by the digital caliper in accordance with ASTM C1437 (ASTM, Citation2007b). The polycarboxylate-based HRWR was used to achieve the flow of the mortars that contained the calcined materials. Mortar specimens that attained the desired flow were cast in a 50 mm cube three gang metallic mould. The mould and content were cured under a plastic sheet and a wet burlap cover for 24hrs. After the 24hrs, the mortar specimens were demoulded and cured in water containing lime. PSAI was performed on mortar specimens cured at 7 and 28 days whereas the compressive strength on mortar specimens was measured at 3, 7 and 28 days. Strength determination was taken as the average of three specimens.
2.2.3. Si and 27Al MAS NMR
Tecmag Apollo Console (Houston, TX) with 8.45 T magnet and homebuilt, single channel, 4 mm wide-bore NMR probe was used to determine 27Al and 29Si spectra. About 90 mg of a sample was taken for each analysis and signal represented as chemical shift value; δ: ppm. The 27Al and 29Si Larmor frequencies were 93.074 MHz and 70.958 MHz, respectively. 27Al spectra were acquired with MAS spinning frequency, last delay and 90º pulse length of 8 KHz, 1s and 2.5 μs, respectively. 29Si spectra were acquired with MAS spinning frequency, last delay and 60º pulse length of 8 kHz, 20s and 5.5 μs, respectively. Aluminum nitrate [Al(NO3)3] and Tetramethyl silane (TMS) were used as reference compounds for 27Al and 29Si spectra, respectively. All experiments were performed at ambient temperature without any corrections for sample heating.
2.2.4. Sorptivity
Water sorptivity determination was performed in accordance with ASTM C1585 (ASTM, Citation2014b). The principle behind sorptivity states that if a mortar or concrete surface is exposed to wetting by water, then during the initial absorption period (S), the cumulative water absorption (i) is proportional to the square root of elapsed wetting time (t) (see EquationEquation 1)(1)
(1) . An average of three mortar specimens were used for the absorption calculations. The ratio of gain in mass per unit area to the density of water was plotted versus the square root of the elapsed time (t). The slope of the line of best fit of these points was taken as the sorptivity value.
2.2.5. Drying and autogenous shrinkage
The shrinkage tests were determined in accordance with ASTM C596 (ASTM, Citation2017) standard test on mortar specimen. Mortar samples were prepared to achieve a flow of 110 ± 5%. For the cement-blended mortars, the desired flow was achieved with the aid of HRWR (Glenium 7500). Six mortar samples were cast in 25 by 25 by 285 mm prismatic moulds. The specimens were covered with a transparent plastic sheet and a moist burlap to prevent evaporation. After 24 hours of moist curing, samples were demoulded and cured in a water bath for 48 h. Mortar specimens designated for the drying shrinkage test were placed under curing water for 72 hours after which their dimensions were recorded. On the other hand, specimens designated for autogenous shrinkage were sealed with wax and placed in an environmental chamber maintained at 23°C and 50% relative humidity. The unsealed samples after lime water curing were also exposed to the conditions in the environmental chamber. Length measurement of both the sealed and unsealed mortar prisms were recorded at 4, 7, 11, 18 and 25 days of exposure in the chamber.
2.2.6. Estimation of embodied carbon
The estimation of the embodied carbon emissions considers all the carbon output of the mortar constituents per cubic meter of the control and optimum mortar mix (30RH1). The computation of the embodied carbon was based on EquationEquation (2)(2)
(2) .
Where EC = Embodied energy; CMQ = mass of the ith type material; EEC = Embodied carbon coefficient of the ith material.
This method of computing embodied energy of materials has been used by many researchers including Kumanayake et al. (Kumanayake et al., Citation2018) and Chau et al. (Chau et al., Citation2015).
3. Results and discussions
3.1. Pozzolanic strength activity index (PSAI)
Figure shows the strength activity indices of the control and the co-fired pozzolanic incorporated mortars. ASTM C618 (ASTM, Citation2012) specifies that the activity of mortars containing pozzolans at 7 and 28 days must not fall below 75% represented by the line labelled as minimum (min). The activity of the calcined clay and rice husk were normalized against the control mortar represented by the line labelled as maximum (max). All the pozzolan mortars met the ASTM standard as indicated by the grey line. From the figure, all the co-fired materials attained mortar strength activity indices higher than the control. The maximum strength activity for all the mortars was recorded by RH1 (98% clay and 2% rice husk). The improvement of the strength activity index of RH1 could be attributed to the extra pozzolanic effect imparted on the calcined clay from the rice husk ash.
3.2. Compressive strength
Table presents the mix proportions of the control and the blended mortars. The labelling of the cement and pozzolan mixes were 10RH1, 20RH1, 30RH1 and 40RH1 which represented the replacement of cement with clay by 10 to 40%. The table shows that as the pozzolan content increased from 0 to 40%, the content of the chemical admixture (HRWR) required to achieve the appropriate flow of the mortar also increased. The inclusion of the calcined materials altered the specific gravities of the various mixes. The specific gravities of the control, 10RH1, 20RH1, 30RH1 and 40RH1 of the co-fired material were 3.15, 3.08, 3.01, 2.94 and 2.84, respectively.
Table 2. Mortar mix proportions of control and blended cement mortars
Figure shows the results of the compressive strength of mortars; control and the mixtures- 10RH1, 20RH1, 30RH1 and 40RH1. The early age strength at 3 and 7 days of 10RH1, 20RH1 and 30RH1 were all higher than the control mortar except 40RH1. However, at 28 days, all the mortar mixture proportions attained compressive strengths which were higher than the control mortar. From Figure it is observed that the 20RH1 and 30RH1 have similar compressive strength at the various curing periods. The student t-test performed between 20RH1 and 30RH1 show a statistically insignificant predictive value (p = 0.67). The preferred replacement content for Portland cement is 30RH1. The reason is that at higher supplementary cementitious content utilization, there are reductions in embodied energy and cost of the binder (ASTM, Citation2012).
3.3. Al and 29Si MAS NMR
Figure shows the 27Al MAS NMR spectra analysis of the control and the clay-RH binders at 3, 7 and 28 days. The figures show two distinct chemical shift which occurred around 68 ppm and 7.8 ppm. Chemical shift around 68 ppm shows Al substitution in the calcium silicate hydrate (CSH) in the tetrahedral environment of Al, a metastable form of hydrogarnet or calcium aluminosilicate hydrate (CASH) (Andersen et al., Citation2003; Marceau et al., Citation2007). The chemical shift around 7.8 ppm corresponds to thermodynamically stable monosulphates (AFm) which occur in the Al(vi) environment (Chenguang et al., Citation2014; Skibsted et al., Citation1993). The intensities at the octahedral environment were 94% at 3 days and 99% at both 7 and 28 days. This shows that the hydration progressed from 3 days to 7 and 28 days with an increased aluminium phase in the tetrahedral environment that dissolved in solution into the octahedral environment due to the presence of the pozzolanic material. The dissolution from the unstable tetrahedral to stable octahedral environment indicates the occurrence of pozzolanic reaction. Anderson et al. (Andersen et al., Citation2006) have indicated that usually the stable phase formed in the octahedral environment is stratlingite (C2AH8), a known stable monosulphate phase. The formation of the monosulphate phase in that environment also indicates the substitution of Al3+ for Si4+ to form calcium aluminosilicate hydrates. Chenguang et al. (Andersen et al., Citation2003) mentioned that Al3+ substitution for Si4+ promotes the stability of calcium silicate hydrates. The increase in stable compound in the aluminate phases in the CSH structure explained the reason for the higher strength of clay-RH pozzolan more than the control.
Figure presents the 29Si MAS NMR of the control and 30RH1 hydrated pastes at 3, 7 and 28 days. The figures (A, B and C) show two spectra representing the hydrated samples. At 3 days, both spectra showed a chemical shift at −89.7 ppm and −109.1 ppm. The shift at −89.7 ppm could indicate partly a Q2 and Q3 (1Al) unit similar to the shift found in the studies of Xiaoming et al. (Andersen et al., Citation2003). At this chemical shift Al3+ enters the C-S-H structure forming an aluminium rich compound called gehlenite (Xiamong et al., Citation2011). The incorporation of 30RH1 in the Portland cement partially responded to pozzolanic reaction after 3 days, which is indicated as a slightly intensed peak on the 30RH1 spectra. At 7 days (Figure B), the two spectra of the control and 30RH1 show a chemical shift at −90.7 ppm and −109.1 ppm. The figure also shows a slight chemical shift from −89.7 ppm at 3 days to −90.1 ppm at 7 days, which is indicative of an increase in polymerization with time. The 30RH1 spectrum shows the formation of an additional peak at −109.1 ppm which could be attributed to the effect of pozzolanic action (C-S-H formation). After 28 days of hydration, there was a slight shift from −90.1 ppm at 7 days back to −89.7 ppm (see Figure C). This indicates a decrease in the crystallinity of the C-S-H content in the Q2 and Q3 units. The increase in polymerization was confirmed with the slight shift from −109.1 ppm at 7 days to −109.5 ppm at 28 days on the control spectrum. The chemical shift from −109.1 ppm at 7 days to −112.3 ppm at 28 days as well as the formation of an additional chemical shift at −122.8 ppm (Q4(01Al)) unit confirmed that the addition of calcined clay to cement caused a significantly enhanced polymerized material. This also shows that pozzolanic reaction occurred and caused an increased strength of 30RH1 more than the control.
3.4. Sorptivity
Figure presents the rate of water absorption of the Portland cement (Control) and the clay-rice husk pozzolan (30RH1) mortars. The initial (Si) and the secondary sorptivity (Ss) coefficients of the control mortar were 0.0299 and 0.0083 mm/s1/2 respectively. For 30RH1 pozzolan mortars, the Si and Ss are 0.0055 and 0.0022 mm/s1/2 respectively. The coefficient of determination (R2) of the linear regression model namely Si (CON), Si (Clay-RH), Ss (CON), Ss (Clay-RH) were approximately 0.96, 0.87, 0.93 and 0.97, respectively. The r-squared values indicate a strong relation between the dependent and independent variables.
The results from Figure show that both the initial and the secondary coefficient values of the 30RH1 mortars were lower than the control mortars. Low sorptivity values of the 30RH1 pozzolan indicate that the binder slows down the rate of ingress of moisture into the mortar and therefore could be used to produce good concrete. This result falls in line with the investigations of Khatib (Khatib, Citation2004) and Dhandapani et al. (Dhandapani et al., Citation2018) who also recorded lower sorptivity and absorption on their studies on pozzolans.
3.5. Drying and autogenous shrinkage
Figure shows the results of the drying shrinkage characteristics of the Control and the 30RH1 mortars. It is a plot of strain (%) versus exposure period in days. A student t-test performed between the two mortar mixes gave a predictive value (p) of 0.33 meaning the difference between the shrinkage values is insignificant. This shows that the inclusion of the pozzolanic material will behave the same way like the Portland cement to restrain shrinkage.
Figure shows the results of the autogenous shrinkage of the Portland cement and blended cement mortars. A student t-test was performed between the values of the control (CON) and the blended (Clay-RH) mortars at a critical value of 0.05. The predictive (p) value was 0.99 meaning the difference between the average values of the two mortar mixes was also insignificant. The results of the autogenous shrinkage performance indicated that the inclusion of the calcined pozzolan could be used in place of Portland cement.
The autogenous and the drying shrinkage performance of the clay-RH pozzolan mortar could be attributed to the formation of adequate hydrates. Gleiz et al. (Gleize Philippe et al., Citation2007) and Itim et al. (Itim et al., Citation2011) have stated that the formation of extra hydrates usually makes mortars less deformable and hence restrain shrinkage.
3.6. Embodied carbon estimation
Table shows the embodied carbon of the materials contained in the mortars. The bill of activities to produce a cubic meter of the mortar is also shown in Table . The table shows that the inclusion of co-fired material in a cubic meter of mortar-reduced cement content by 31% and both water and sand content by 2%.
Table 3. Embodied carbon of concrete constituents
Table 4. Mass content per cubic meter of control and blended mortars
Table presents the estimated embodied carbon values per cubic meter of the control and the blended cement mortars. The embodied carbon (EC) content of the control mortar was approximately 516.00 kgCO2eq whereas the blended mortar (30RH1) was also approximately 369.23 kgCO2eq. The incorporation of 30% content of pozzolan reduced the greenhouse gases by approximately 29%. In essence, the inclusion of the pozzolan could be a means of decarbonizing mortar which could be very beneficial to the environment since it is already known that greenhouse gases are the major cause of global warming.
Table 5. Embodied carbon of control and blended mortars
4. Conclusions
The studies determined the mechanical and durability properties of co-fired clay and rice husk pozzolan. Pozzolanic strength activity index and compressive strength test were used to determine the pozzolanic reactivity and optimum-calcined material, respectively. 29Si and 27Al MAS NMR were used to determine the silicate and aluminate environments of the optimum hydrated paste and compared with the control. The durability studies used in this study were water sorptivity and drying shrinkage. From the results of the study the following conclusions were made:
Co-fired material that contained 2% of rice husk content and labelled as RH1 gave an optimum pozzolanic strength activity index of 118% and 116% at 7 and 28 days respectively. The recommended ASTM C618 value at 7 and 28 days is a value greater than 75%.
The optimum Portland cement replacement using the co-fired material was at 30%. This could be attributed to the reactivity of the calcined material with Portland cement forming extra cementitious hydrates that enhanced strength properties.
The formation of secondary calcium silicate/aluminate hydrate confirmed from the 29Si and 27Al MAS NMR studies was the major contributing factor to the higher strength performance of the 30RH1 mortar than the control.
For the clay pozzolan industry, the rice husk biomass could be treated as useful resource since it does not compromise the pozzolanic nature of calcined clay.
The sorptivity studies showed that clay-rice husk pozzolan recorded initial and secondary sorptivity values of 0.0055 and 0.0022 mm/sec1/2, which are lower than the Portland cement values of 0.0299 and 0.0083 mm/sec1/2. This shows that the binders prepared using clay-rice husk pozzolan could produce good and an enhanced durable concrete.
The shrinkage properties, both drying and autogeneous of the two binders, Portland cement and clay-rice husk pozzolan indicated that there is significant difference between the two binders.
The inclusion of the co-fired pozzolan reduced the estimated carbon equivalence from approximately 516.12 kgCO2eq to 369.23 kgCO2eq which represented about 29% reduction from the control mortar.
Additional information
Funding
Notes on contributors
Mark Bediako
Mark Bediako is a senior research scientist and the head of division of the Advanced material science division at CSIR-Building and Road Research Institute. He obtained his PhD in material science engineering. His research interest includes supplementary cementitious materials, concrete sustainability and durability, geomaterials and waste management.
Albert Adjaottor is an Associate Professor at the department of materials engineering, KNUST. His research area includes supplementary cementitious materials, waste management and ceramics
Simon Gawu is an Associate Professor in the Geological Engineering Department at KNUST. His research interests include construction materials, tailing dams, lithomargic clays, and other geomaterials.
Eric Opoku Amankwah is the Acting Deputy Director at the Office of Works and Physical Development of University of Education, Winneba in charge of Structural Engineering and Maintenance. He holds MPhil in Civil Engineering and specialized in structural and civil engineering materials.
References
- Andersen, M. D., Jakobsen, H. J., & Skibsted, J. (2003). Incorporation of Aluminum in the Calcium Silicate Hydrate (C−S−H) of hydrated portland cements: A High-Field 27 Al and 29 Si MAS NMR investigation. Inorganic Chemistry, 42(7), 2280–17. https://doi.org/10.1021/ic020607b
- Andersen, M. D., Jakobsen, H. J., & Skibsted, J. (2006). A new aluminium-hydrate species in hydrated Portland cements characterized by 27Al and 29Si MAS NMR spectroscopy. Cement and Concrete Research, 36(1), 3–17. https://doi.org/10.1016/j.cemconres.2005.04.010
- Anon. (2015). A professional’s guide to durable home design durability by design. U.S. Department of Housing and Urban Development.
- ASTM. (2002). Standard test methods for sampling and testing fly ash or natural pozzolans for use in portland-cement concrete (ASTM C311–02)
- ASTM. (2006). Standard specification for standard Sand (C778-13).
- ASTM. (2007a). Standard test method for compressive strength of hydraulic cement mortars (2-in or [50 mm] cube specimen (C109-03).
- ASTM. (2007b). Standard test method for flow of hydraulic cement mortar (C1437).
- ASTM. (2012). Standard specification for coal fly ash and raw or calcined natural pozzolana for use in concrete (C618).
- ASTM. (2014a). Standard practice for mechanical mixing of hydraulic cement pastes and mortars of plastic consistency (C305-14).
- ASTM. (2014b). Standard test method for measurement of rate of absorption of water by hydraulic-cement concretes (C1585).
- ASTM. (2017). Standard test method for drying shrinkage of mortar containing hydraulic cement (C596).
- Avet, F., Li, X., & Scrivener, K. (2018). Determination of the amount of reacted metakaolin in calcined clay blends. Cement and Concrete Research, 106, 40–48. https://doi.org/10.1016/j.cemconres.2018.01.009
- Ballim, Y., & Graham, P. C. (2009). The effects of supplementary cementing materials in modifying the heat of hydration of concrete. Materials and Structures, 42(6), 803–811. https://doi.org/10.1617/s11527-008-9425-3
- Bao-guo, M. A., Xiao-dong, W., Ming-yuan, W., Jia-jia, Y., & Xiao-jin, G. (2007). Drying shrinkage of cement-based materials under conditions of constant temperature and varying humidity. Journal of China University of Mining and Technology, 17(3), 0428–0431. https://doi.org/10.1016/S1006-1266(07)60119-9
- Barcelo, L., Kline, J., Walenta, G., & Gartner, E. (2014). Cement and carbon emissions. Materials and Structures, 47(6), 1055e1065. https://doi.org/10.1617/s11527-013-0114-5
- Bediako, M., Kevern, J. T., & Dodoo-Arhin, D. (2017). Co-fired Ghanaian clay-palm kernel shells pozzolan: Thermogravimetric, 29Si and 27Al MA NMR characteristics. Construction and Building Materials, 153, 430–435. https://doi.org/10.1016/j.conbuildmat.2017.07.042
- Bediako, M., Purohit, S. S., & Kevern, J. T. (2017). Investigation into Ghanaian calcined clay as supplementary cementitious material. ACI Materials, 114(6), 889–895.
- Chau, C. K., Leung, T. M., & Ng, W. Y. (2015). A review on life cycle assessment, life cycle energy assessment and life cycle carbon emissions assessment on buildings. Applied Energy, 143, 395–413. https://doi.org/10.1016/j.apenergy.2015.01.023
- Chenguang, H. U., Shuguang, H. U., Qingjun, D., Xiaoxin, F., & Xiulin, H. (2014). Effect of curing regime on degree of Al3+ Substituting for Si4+ in C-S-H gels of hardened portland cement pastes. Journal of Wuhan University of Technology-Mater, 546–552.
- Christopher, F., Bolatito, A., & Ahmed, S. (2017). Structure and properties of mortar and concrete with rice husk ash as partial replacement of ordinary Portland cement – A review. International Journal of Sustainable Built Environment, 6(2), 675–692. https://doi.org/10.1016/j.ijsbe.2017.07.004
- de Sensale, G. R. (2006). Strength development of concrete with rice-husk ash. Cement & Concrete Composites, 28(2), 158–160. https://doi.org/10.1016/j.cemconcomp.2005.09.005
- Dhandapani, Y., Sakthivel, T., Santhanam, M., Gettu, R., & Pillai, R. G. (2018). Mechanical properties and durability performance of concretes with Limestone Calcined Clay Cement (LC3). Cement and Concrete Research, 1–18.
- Donatello, S., Tyrer, M., & Cheeseman, C. R. (2010). Comparison of test methods to assess pozzolanic activity. Cement and Concrete Composites, 32(2), 121–127. https://doi.org/10.1016/j.cemconcomp.2009.10.008
- FAOSTAT Food and Agriculture data. (2015). Retrieved December 13, 2016, from http://www.fao.org
- Fernandez, R., Martirena, F., & Scrivener, K. L. (2011). The origin of the pozzolanic activity of calcined clay minerals: A comparison between kaolinite, illite and montmorillonite, Cem. Cement and Concrete Research, 41(1), 113–122. https://doi.org/10.1016/j.cemconres.2010.09.013
- Gleize Philippe, J. P., Martin, C., & Gilles, E. S. (2007). Effects of metakaolin on autogenous shrinkage of cement pastes. Cement and Concrete Composites, 29(2), 80–87. https://doi.org/10.1016/j.cemconcomp.2006.09.005
- Hammond, G. P., & Jones, C. I. (2008). Embodied energy and carbon in construction materials. Proceedings of the Institution of Civil Engineers - Energy, 161(2), 87–98. https://doi.org/10.1680/ener.2008.161.2.87
- Hua, X., Shi, Z., Shi, C., Wua, Z., Tong, B., Ou, Z., & de Schutter, G. (2017). Drying shrinkage and cracking resistance of concrete made with ternary cementitious components. Construction and Building Materials, 149, 406–415. https://doi.org/10.1016/j.conbuildmat.2017.05.113
- Itim, A., Ezziane, K., & Kadri, E.-H. (2011). Compressive strength and shrinkage of mortar containing various amounts of mineral additions. Construction and Building Materials, 25(8), 3603–3609. https://doi.org/10.1016/j.conbuildmat.2011.03.055
- Jensen, O. M., & Hansen, P. H. (1996). Autogenous deformation and change of relative humidity in silica-fume modified cement paste. ACI Material J, 93, 539–543.
- Khatib, J. M. (2004). Absorption characteristics of metakaolin concrete. Cement and Concrete Research, 34(1), 19–29. https://doi.org/10.1016/S0008-8846(03)00188-1
- Khatib, J. M., & Hibbert, J. J. (2005). Selected engineering properties of concrete incorporating slag and metakaolin. Construction and Building Materials, 19(6), 460–472. https://doi.org/10.1016/j.conbuildmat.2004.07.017
- Kumanayake, R., Luo, H., & Paulusz, N. (2018). Assessment of material related embodied carbon of an office building in Sri Lanka. Energy & Buildings, 166, 250–257. https://doi.org/10.1016/j.enbuild.2018.01.065
- Liu, J., Xing, F., Dong, B., Ma, H., & Pan, D. (2014). Study on water sorptivity of the surface layer of concrete. Materials and Structures, 47(11), 1941–1951. https://doi.org/10.1617/s11527-013-0162-x
- Liu, X., Zhang, N., Sun, H., Zhang, J., & Li, L. (2011). Structural investigation relating to the cementitious activity of bauxite residue — Red mud. Cement and Concrete Research, 41(8), 847–853. https://doi.org/10.1016/j.cemconres.2011.04.004
- Luxan, M. P., Madruga, F., & Saavedra, J. (1989). Rapid evaluation of pozzolanic activity of natural pits by conductivity measurement. Cement and Concrete Research, 19(1), 63–68. https://doi.org/10.1016/0008-8846(89)90066-5
- Mamlouk, M. S., & Zaniewski, J. P. (2006). Materials for civil and construction engineers. Prentice Hall Publishers.
- Marceau, M. L., Nisbet, M. A., & VanGeem, M. G. (2007). Life cycle inventory of Portland cement association (pp. 120)
- McCarter, W. J., & Tran, D. (1996). monitoring pozzolanic activity by direct activation with calcium hydroxide. Construction and Building Materials, 10(3), 179–184. https://doi.org/10.1016/0950-0618(95)00089-5
- Mendess, A., Gates, W. P., Sanjayan, J. G., & Collins, F. (2011). NMR, XRD, IR and synchrotron NEXAFS spectroscopic studies of OPC and OPC/slag cement paste hydrates. Materials and Structures, 44(10), 1773–1791. https://doi.org/10.1617/s11527-011-9737-6
- Paya, J., Borracheto, M. V., Monzo, J., Peris-Mora, E., & Amah-Jour, F. (2001). Enhanced conductivity measurement techniques for evaluation of fly ash pozzolanic activity. Cement and Concrete Research, 31(1), 179–184. https://doi.org/10.1016/S0008-8846(00)00434-8
- Shi, G., Wu, T., Reifler, C., & Wang, H. (2005). Characteristics and pozzolanic reactivity of glass powders. Cement and Concrete Res, 35(5), 987–993. https://doi.org/10.1016/j.cemconres.2004.05.015
- Skibsted, J., Henderson, E., & Jakobsen, H. J. (1993). Characterization of calcium aluminate phases in cements by 27AlMAS NMR spectroscopy. Inorganic Chemistry, 32(6), 1013–1027. https://doi.org/10.1021/ic00058a043
- Snelling, R. (2016). Assessing, understanding and unlocking supplementary cementitious materials. RILEM Technical Letters, 1, 50–55. https://doi.org/10.21809/rilemtechlett.2016.12
- Sukholthaman, P., & Shirahada, K. (2015). Technological challenges for effective development towards sustainable waste management in developing countries: Case study of Bangkok, Thailand. Technology in Society, 1–10.
- Tam, C. M., Tam, V. W. Y., & Ng, K. M. (2012). Assessing drying shrinkage and water permeability of reactive powder concrete produced in Hong Kong. 26(1), 79–89. https://doi.org/10.1016/j.conbuildmat.2011.05.006
- Tironi, A., Terezza, M. A., Scian, A. N., & Irassar, E. F. (2013). Assessment of Pozzolanic activity of different calcined clays. Cement & Concrete Composites, 37, 319–327. https://doi.org/10.1016/j.cemconcomp.2013.01.002
- Xiamong, L., Na, Z., Henghu, S., Jixlu, Z., & Longtu, L. (2011). Structural investigation relating to the cementitious activity of bauxite residue red mud. Cement and Concrete Research, 41(8), 847–853.
- Zerbino, R., Giaccio, G., & Isaia, G. C. (2011). Concrete incorporating rice-husk ash without processing. Construction and Building Materials, 25(1), 371–378. https://doi.org/10.1016/j.conbuildmat.2010.06.016
- Zhou, D., Wang, R., Tyrer, M., & Wong, H. (2017). Christopher Cheeseman, Sustainable infrastructure development through use of calcined excavated waste clay as a supplementary cementitious material. Journal of Cleaner Production, 168, 1180–1192. https://doi.org/10.1016/jclepro.2017.09.098