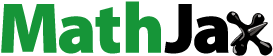
Abstract
The objective of this research paper is to analyze the materials which can be suggested for usage to solve the problem of structural defect caused by water absorption through penetration. Each of the concrete types was made in five concrete mix series of 0%, 0.45%, 0.9%, 1.2%, and 1.6% of chopped basalt fiber. Each of the concrete series was shared into two sets where the first set was tested for strength on the day 28 curing period while the second set was soaked in immersed in water at room temperature for additional 96 hours after the day 28 before testing for strength. The compressive strength of the expanded clay concrete cube specimen tested on day 28 curing period showed an increase in strength to 34.71 MPa lesser than the set with immersed in water for additional 96 hours to 37.38 MPa. Similarly, conventional concrete cube specimens at day 28 compressive strength to 29.97 MPa and to 29.97 MPa for conventional concrete after day 28. Expanded clay concrete showed more flexural strength of 2.921 MPa at day 28 and 2.895 MPa for concrete after day 28 with additional 96 hours in water. Similarly, on flexure, conventional concrete specimens showed flexural strength up to 1.917 MPa at day 28 while up to 1.733 MPa after day 28 with additional 96 hours in water. Concrete containing 1.6% basalt fiber gave more strength and improves the concrete capability.
PUBLIC INTEREST STATEMENT
The durability of a structure depends on many factors. These factors are linked to the properties of the materials used in the structure. Also, the environment where this structure is erected has its effect on the structure. Most constructions in the coastal regions of Sub-Saharan Africa face similar challenges on their ground and basement floors, which is linked to concrete water absorption. Based on this challenge, this paper suggests a solution to water penetrating the building floors. Extensive research and experiments were done to ascertain the effectiveness of the suggestion. This paper suggested the use of dispersed chopped basalt fiber and expanded clay aggregate as solution to the challenges.
1. Introduction
Rapid increment in construction works and urbanization brought greater demand for construction materials that have to do with the idea of sustainability, environmentally friendly, and low processing costs (Ash & Paste, Citation2020). Selection of building materials for construction is influenced by factors like climate, aesthetic, cost-effectiveness, availability, desired strength, hardness, etc. (Irwan et al., Citation2016). The influence of the above-listed factors is seen on the physical and chemical properties of the materials like compressive strength, flexural strength, absorption of water, durability, etc. (Alsayed & Amjad, Citation1996; Chahal et al., Citation2012; Kim et al., Citation2012; Kamseu et al., Citation2015; De Schutter & Audenaert, Citation2004).
Absorption of water value is one of the most important parameters of rocks that have an impact on physical and mechanical properties. This is mostly important for building stones, quality for hygiene, aesthetic view, and structural safety. Thus, water absorption of concrete is explained as the proportion of water, which can be absorbed by stone or other concrete coarse aggregate under specific immersion conditions which leads to degradation of strength, abrasion, and frost resistance of the concrete depending on the pore system (Balakrishna et al., Citation2019; Matarul et al., Citation2016; Md Noor et al., Citation2017; Shaikh, Citation2016).
Concrete durability is the ability of the concrete to last long without significant deterioration (Parthiban et al., Citation2017). The water absorption, volume of voids, and sorptivity are generally used to test concrete durability (Bogas & Sofia, Citation2019).
Compressive strength of concrete is the most common performance measure used by civil engineers in designing buildings while flexural strength of concrete material is essential to make sure the material is strong enough to be used in structures (Zhou et al., Citation2020).
Concrete has higher compressive strength and durability. But it has low tensile strength, poor impact resistance, poor toughness, and low tensile strength. Still, it can be improved by mixing concrete with basalt fiber as reinforcement material (Gamal & Chiadighikaobi, Citation2019) and with expanded Clay Aggregate, Granite, etc. (Chiadighikaobi et al., Citation2019). These materials have the potential to improve compressive strength as well as shearing and fracture resistance of concrete (Kim et al., Citation2011; High et al., Citation2015; Liu et al., Citation2006; Monaldo et al., Citation2019).
Basalt fiber derived from volcanic rocks has a wide range of raw material sources, good thermal stability (the end-use temperature range is −263°C to 900°C), thermal insulation (the thermal conductivity is approximately 0.04 W/(m·K)), good environmental compatibility, high tensile strength, and high elastic modulus (ASTM C1585–13, Citation2013; GOST 1Citation0180–2012, 2013; CitationLECA). Chopped Basalt Fiber is the basalt fiber composites according to research that is manufactured with and without the Gel-coating process and is immersed in a moisture absorption device at 80°C for more than 100 days (CitationLECA).
Lightweight expanded clay aggregate (LECA) is a lightweight aggregate. But the low volume weight, high water-absorbing capacity, and low strength are the main challenging during design, production, and construction. However, these can be modified by adding chopped basalt fiber. The chopped basalt fiber serves as an admixture or reinforcement. Both expanded clay aggregate (ECA) and basalt fiber (BF) are concrete materials with high-water absorption rate. These materials are excellent for use in areas that experience many waterlogs that penetrate the concrete walls and floor slaps (CitationLECA). ECA is an inert lightness substance that does not contain harmful materials, it has a natural pH value of about 7. It is not damaged by water and it is moisture impermeable, non-combustible, non-biodegradable, and non-decomposition against severe conditions (RILEM TC-196, Citation2007). LECA concrete or mortar has a lower E-modulus which leads to a lower crack tendency due to lower tensile stress induced by the same strain (Lopez et al., Citation2008).
The important property of LECA is water absorption, which plays an important role in the proportioning of concrete mixtures. Expanded clay (EC) shows high-water absorption with conventional aggregates. This high-water absorption is related to the porous structure of its grains (ASTM C127–15, Citation2015; Azhani et al., Citation2018). By contrast, ordinary aggregates generally absorb less than 2% of moisture. This characteristic is in good agreement with performed research on the absorption properties of expanded shale and clay aggregates where 24 h water absorption recorded from 6.0% to 30.5% (ASTM C128–15, Citation2015; Moravia et al., Citation2006).
Comparing ECA with granite, granite is qualified as impervious to water and is less absorbent than some solid surface (plastic) products. Granite is widely used as a building material for high-rise buildings due to its ability to withstand gale-force winds and rain. It is known generally for its natural ability to resist moisture. It has a natural water resistance that helps protect it. Since it has pores, it will still absorb bits of moisture leading to damage (Granite fabricator, Citation0000).
One of the challenges in construction is the effect of water penetration into the walls and ground floor slabs that leads to structural failure and reduces structural durability (). Water absorption by immersion and total pore volume are considered better indicators in evaluating the potential durability of concrete than capillary absorption (Levy & Helene, Citation2004). Despite the advances in studies and utilizing construction, gap like durability still needs more to be covered on the characteristics of expanded clay and basalt fiber (Guo et al., Citation2018; Monaldo et al., Citation2019; Sim et al., Citation2005). Water penetration and inability of some concrete walls and floors deteriorate () the building faster and affect its functionality, and it causes musty odors or mildew growth in the space (Maruthupandian, Citation2018).
Figure 1. Effect of water penetration on walls and slabs.https://theconstructor.org/building/prevention-dampness-building/4577/
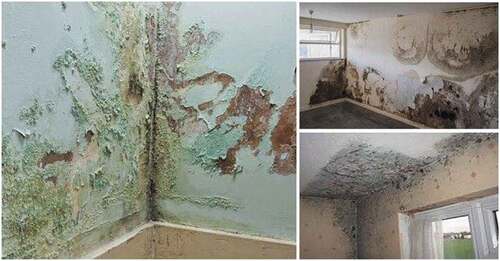
Thus, this paper investigates and suggests a better material through laboratory experiments to solve the problems of water penetration on walls and slabs. The experimental study uses ECA and dispersed chopped BF materials, to investigate the compressive strength, flexural strength, water absorptions, and durability (hydrological properties of the compressive and flexural specimens).
2. Experimental setup
The following procedures and materials were used for the preparation of concrete specimens for experiments and analysis.
2.1. Materials
The experimental studies were carried out under the CIS Interstate Standard GOST 10180–2012 (GOST 1Citation0180–2012, 2013).
The characteristics associated with materials used in the experiments are stated in this section:
Concrete Coarse Aggregate EC of 5–8 mm fraction for Lightweight Concrete ().
The clay is dried, heated, and burned in rotary kilns at 1100–1300°C inhabiting essential properties like lightweight, insulating, strong, non-combustible, and fire-resistant, extremely stable and durable, natural material for sustainable construction, versatility, and high drainage capacity (Md. et al., Citation2016; Sepehr et al., Citation2014; Slater et al., Citation2012). The chemical composition of LECA according to the analysis of different authors is illustrated in .
Concrete coarse aggregate crushed granite of 5–20 mm fraction for conventional concrete ().
Fine aggregate quartz sand of 0.4–0.6 mm fraction 0.4–0.6 mm.
Table 1. Chemical composition of LECA (CitationLECA)
A feature of the proposed quartz is the presence of coarse-grained sand, with a large modulus of fineness up to M3.5, and quartz sand has a rounded part with a low content of clay inclusions and inclusions of soft rocks. The resulting quartz sand undergoes additional enrichment and drying. The moisture content is up to 0.2% (CitationFractionated quartz sand). The chemical composition of quartz sand is illustrated in .
Binder Holcim Portland cement M500 D20 CEM II 42.5 N.
Table 2. Chemical composition of quartz sand (Fractionated quartz sand)
The characteristics of Holcim Portland cement M500 D20 CEM II 42.5 N. M—brand, 500 is a figure showing the average compressive strength for 28 days in kg/cm2, D—additives, 20—allowable number of additives in % (up to 20%), CEM II—cement containing additives, and the content of additives is 6–20%, I-type additives, limestone, 42.5-class compressive strength for 28 days, must be at least this value, and B-quick hardening. presents the physical and chemical properties of the Holcim Portland cement.
Mineral filler Silverbond Quartz flour of 50 µm.
Table 3. Physical and chemical properties of Holcim Portland cement
Unique Properties of Silverbond Quartz Flour among Other fillers are hardness and abrasion resistance, high chemical resistance, anticorrosion, low oil absorption, and low coefficient of thermal expansion. Quartz flour is produced by grinding chemically pure, natural quartz sand to a finely divided state. The technology used guarantees the stability of the chemical composition during grinding and allows you to get a constant particle size distribution of quartz flour. The crushed quartz flour is represented by rounded particles with uneven, broken edges. Quartz differs from other mineral fillers in hardness, abrasion and chemical resistance, anti-corrosion, and low coefficient of thermal expansion. Quartz is a chemically stable mineral; it is soluble only in hydrofluoric acid. With a low oil absorption and a small surface area of the particles, the use of quartz flour will allow you to get a system with a high degree of filling. illustrates the compositions and properties found in quartz flour.
The basalt fiber for this experiment has a diameter of 15 µm and a length of 20 mm ().
Table 4. Compositions and properties of quartz flour
The mechanical properties of concrete enhanced meaningfully when using basalt fiber with a length between 12 mm and 24 mm, and content between 0.1% and 0.5% of total volume (Sami Elshafie, Citation2015). It has 89 GPa and 4840 MPa elastic modulus and tensile strength, respectively. The elastic modulus measures the stiffness of the material and is related to atomic bonds and does not depend on strength. For quality purposes, generally tensile strength can be used. For this experimental work, tensile strength and elastic modulus of basalt fiber were obtained from the manufacturer. BF possessing higher tensile strength generally produces higher flexural strength (Fractionated quartz sand, Citation0000; Sami Elshafie, Citation2015; SILICA FUME MK-85, Citation0000; Thomas, Citation2007). The percentages of BF for this research are 0.45%, 0.9%, 1.2%, 1.6%. shows the used BF.
Chopped basalt fiber serves as a dispersed reinforcement in the concrete. The chemical composition and properties are shown in , respectively.
Organic mineral-based additives: silica fume (microsilica) = 62.5 kg/m3 and fly ash = 62.5 kg/m3.
Table 5. Chemical composition of basalt fiber chopped strands
Table 6. Properties of chopped basalt fiber
The chemical compositions of silica fume and fly ash in percentage (%) are outlined in . Silica fume is a product of Ferroalloy production and is formed during the smelting of ferrosilicon and its alloys. By granulometric composition, the average particle size of silica fume is approximately 100 times smaller than the average grain size of cement.
Table 7. Chemical compositions of silica fume (microsilica) and fly ash in percentage (%)
When using silica fume for the manufacture of especially strong concrete, thousands of spherical microparticles surround each cement grain, compacting the cement mortar, filling the voids with strong hydration products, and improving adhesion to aggregates, much more effectively than other mineral additives, such as zeolite tuff, blast furnace, and boiler slag. It is used as a highly active additive to concrete. It is intended for the preparation of special high-grade concrete for strength and waterproofness, foam concrete, dry building mixes, rubber, ceramics, tiles, tiles, and refractory masses (Sami Elshafie, Citation2015). The addition of silica fume helps reduce cement consumption (up to 200–450 kg/m3). As a result of physical and chemical effects, a favorable change in the microstructure of the test occurs, associated with a significant decrease in porosity in the zone of capillary pores. A change in the structure of pores in concrete is considered by many researchers as the main factor in the influence of silica fume on the mechanical properties and strength of concrete. These changes are reflected in the decrease in concrete permeability. Reduced water permeability enhances the resistance of concrete to aggressive environments. High properties of silica fume improve concrete characteristics such as compressive strength, adhesion and wear resistance, frost resistance, chemical resistance, and significantly reduce permeability.
Fly ash. The use of fly ash can lead to significant retardation of the setting time, which means that finishing operations may have to be delayed. At normal temperatures, the rate of the pozzolanic reaction is slower than the rate of cement hydration, and fly ash concrete needs to be properly cured if the full benefits of its incorporation are to be realized. When high levels of fly ash are used, it is generally recommended that the concrete is moist cured for a minimum period of 7 days. It has been recommended that the duration of curing is extended further (for example, to 14 days) where possible, or that a curing membrane is placed after 7 days of moist curing. If adequate curing cannot be provided in practice, the amount of fly ash used in the concrete should be limited. The finishing and curing requirements for high-volume fly ash concrete exposed to cyclic freezing and thawing in the presence of deicing salts is discussed in the section Effect of Fly Ash on the Durability of Concrete (SILICA FUME MK-85, Citation0000).
Super plasticizing and water-reducing additive Sika Plast concrete in liquid form = 8 l/m3.
Tap water at room temperature, which is suitable for drinking, is generally considered satisfactory for mixing and curing of concrete. Hence, potable water was used for the preparation of all concrete specimens.
2.2. Experimental concrete mix design and specimen preparation
The experimental tests were conducted on three specimens from each series on day 28 of the curing period. Another three specimens from each series were immersed in distilled water for 96 hours. Water absorption tests of the concretes were carried out according to ASTM C1585–13 (Citation2013) and British Standard Method of Testing Hardened Concrete for Other than Strength, British Standard Institute, London (Citation1970). The average value from each set of specimens was estimated and used for the analysis. The concrete cube specimens for the comprehensive tests were produced with dimensions of 100x100x100 mm while concrete rectangular prism specimens for the flexural test were produced with dimensions 40 × 40 x 160 mm. The usual 7–14-day analysis done on concrete to check the strength growth was not done in this research paper as it has been established that the strength of lightweight expanded clay concrete (LECC) and conventional concrete increases during the periods of 7 and 14 days and more with the addition of basalt fiber in the concrete (Chiadighikaobi, Citation2020).
The materials used in the concrete mix of this experiment are outlined below.
LECA with fractions of 5–8 mm = 200 kg/m3.
Granite of 5–20 mm fraction.
Quartz sand with fineness modulus of 2.7 = 585 kg/m3.
Quartz flour of 50 µm = 100 kg/m3.
Portland cement CEM I 42.5 N = 500 kg/m3.
Microsilica = 62.5 kg/m3 and fly ash = 62.5 kg/m3.
SikaPlast®Concrete in liquid form = 8 l/m3.
Tap water = 255 l/m3 for mixing.
Chopped basalt fiber.
The flexural test on concrete was conducted using a three-point load test (ASTM C78/C78M—18, Citation2018) or center-point load test (ASTM C293/C293M—16, Citation2016). The test method described in this article is according to ASTM C78/C78M—18 (Citation2018). The compressive test on concrete was conducted on surface area pressure (ASTM C1585–13, Citation2013). The maximum load applied to the specimens were recorded. Average values were taken as the representative of the batches. All expanded clay concrete specimens were cured in a digital concrete curing box for sample curing at temperature 19–22°C and relative air humidity (95 ± 5)%.
The experimental study of concrete specimens was carried out on a MATEX hydraulic press of up to 1500KN at a compression test and 150 KN at flexural test in the laboratory of civil engineering, academy of engineering of the Peoples Friendship University of Russia, Moscow.
3. Results analysis
Based on experimental test results basalt fiber was chosen. The basalt fiber with the concentrations 0.45%, 0.9%, 1.2%, and 1.6% was added to the expanded clay concrete (ECC). After casting, the compressive strength tests were done on day 7.
The compressive strength of expanded clay concrete reinforced with dispersed chopped basalt fiber () shows a gradual decrease in the compressive strength of 1.6% basalt fiber though retains the best basalt fiber percentage for the concentration when compared to the addition of 1.2% basalt fiber.
Figure 5. The cube specimens of expanded clay concrete and 1.6% basalt fiber on compressive strength tests
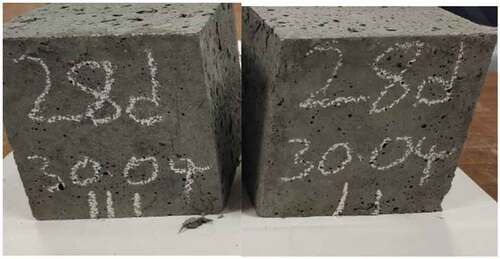
Figure 6. The cube specimens of expanded clay concrete with 1.6% basalt fiber for compressive strength on the additional 96 hours in water
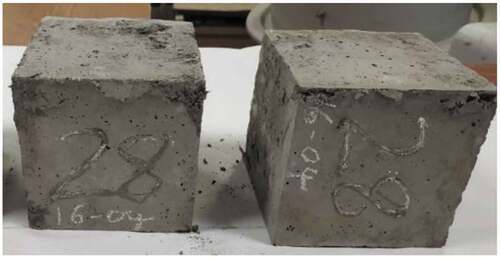
Figure 7. The rectangular prism specimens of expanded clay concrete with 1.6% of basalt fiber for flexural strength tests
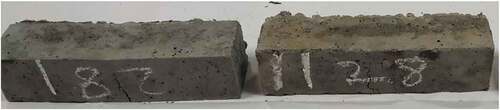
Figure 8. The rectangular prism specimens of expanded clay concrete with 1.6% on flexural strength on the additional 96 hours in water

Table 8. Results of the laboratory tests of ECC specimens of 100 x 100 x 100 mm on the compressive strength
show the cube specimens while show rectangular prism specimens.
The experimental results below are strength comparisons of ECC with conventional concrete with and without dispersed chopped basalt fiber. present specimens’ test results conducted on day 28 curing period while present specimens’ test results conducted on after immersion in water for 96 hours additional from the day 28 curing period. Compressive strengths of both expanded clay concretes and conventional concretes increased with the addition of chopped basalt fiber in the mix. Compressive strength of ECC cube specimens on day 28 increased by 71% while conventional concrete increased by 77%. Nevertheless, the weight and density increased for both expanded clay concrete and conventional concrete which is attributed to the increment chopped basalt fiber percentage added in the concrete. The flexural strength increased while the basalt fiber amount is increased for both expanded clay concrete & conventional concrete.
Table 9. RS test results of expanded clay, concrete cube specimens on day 28
Table 10. RS results of conventional concrete cube specimens on day 28
Table 11. Rf results of expanded clay concrete rectangular prism specimens on day 28
Table 12. Rf results of conventional concrete rectangular prism specimens on day 28
Table 13. Rs results of expanded clay concrete cube specimens immersed in water for 96 hours after day 28 curing period
Table 14. Rs results of conventional concrete cube specimens immersed in water for 96 hours after day 28 curing period
Table 15. Rf results of expanded clay concrete rectangular prism specimens immersed in water for 96 hours after day 28 curing period
Table 16. Rf results of conventional concrete rectangular prism specimens immersed in water for 96 hours after day 28 curing period
The expanded clay concrete rectangular prism specimens immersed in water for 96 hours after day 28 curing period had the weight increased when immersed in water. Similarly, for conventional concrete cube specimens immersed in water 96 hours after day 28.
where RS is compressive strength, Rf is flexural strength.
3.1. Compressive strength
The expanded clay concretes have more strength than conventional concretes. The cases are similar before and after the cube specimens were immersed in water. The quantity of water absorbed by expanded clay concrete is more than that of conventional concrete. The concrete specimens lose their compressive strength after immersion in water. Further, the densities of the cube specimens increased.
The conventional concrete cube specimens before and after impressed are showing a clear variation on the compressive strength while the cube specimens of expanded clay concrete did not show a big variation.
The compressive strength increased with an increase in the basalt fiber content (0–1.6%). For expanded clay concrete (not immersed), the compressive strength increased from 21.92 MPa to 34.71 MPa, with the basalt fiber content 0% and 1.6%, respectively. Similarly, for the conventional concrete immersed in water, the compressive strength increased from 15.37 MPa to 29.97 MPa, with the basalt fiber content 0% and 1.6%, respectively (see ).
For the case of immersed expanded clay concretes, less performance was observed when compared to the expanded clay concrete specimens not immersed in water. However, when the basalt fiber content is 1.6%, the compressive strengths are similar. It is almost like 37.38 and 37.41 MPa.
3.2. Flexural strength
Flexural strength or modulus of rupture of concrete is determined by applying the failure load on the prismatic specimen after 28 days (). The flexural strength of expanded clay concrete is more than that of conventional concrete. show the result of the specimens after undergoing flexural text for expanded clay concrete reinforced with dispersed chopped basalt fiber not soaked in water and expanded clay concrete reinforced with dispersed chopped basalt fiber that was soaked in water. show that though the water absorbed by expanded clay concrete is more than that absorbed by conventional concrete, the expanded clay concrete still retained its high strength. The concrete specimens lose their flexural strength with an increase in their densities after immersion in water.
In general, the flexural strength increased with an increase in the basalt fiber content (0–1.6%). For all concrete rectangular prism specimens showed an increase trend when the basalt fiber content increased. For the expanded clay concrete rectangular prism specimens which were not immersed, the flexural strength increased from 0.571 MPa to 2.921 MPa for the basalt fiber content 0% and 1.6%, respectively. Similar to the conventional concrete rectangular prism specimens, the flexural strength increased from 0.279 to 1.733 for the basalt fiber content 0% and 1.6%, respectively. The flexural strength results of concrete rectangular prism specimens with basalt fiber content from 0 to 1.6%.
Figure 10. The flexural strength results of concrete rectangular prism specimens with basalt fiber content from 0 to 1%
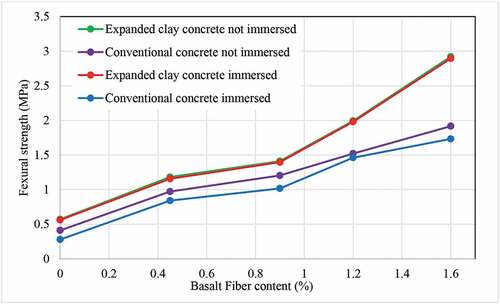
The immersed expanded clay concrete rectangular prisms show very small variations from the not immersed. But, for both cases, the flexural strength increased from 0.562 MPa to 2.895 MPa. The results show that the flexural strength of the concrete increased as the basalt fiber content increased. The expanded clay concrete shows a better performance than conventional concrete.
3.3. Water absorption
The concrete was immersed in water for 96 hours after day 28 curing period and water absorption was measured. Water absorption is considered as the quantity of water absorbed by the concrete when immersed totally in water. The water absorption is estimated by using the following EquationEquation (1)(1)
(1) .
where is water absorption,
is the weight of the concrete cube specimens before immersed, and
is the weight of the concrete cube specimens after immersed.
The water absorption for all concrete cube specimens is showing a decreasing trend when the basalt fiber content was increased. For the expanded clay concrete used for the compressive strength test. In expanded clay concrete cube (a) used for the compressive strength test, the water absorption graphs did not significantly change from 0.45% to 0.44% for 0% and 1.6% the basalt fiber content, respectively. But, for the conventional concrete cube (a) used for the compressive strength test, the water absorption decreases from 0.2% to 0.12% for the basalt fiber content 0% and 1.6% respectively.
In general, from the results in , it is seen that expanded clay concrete absorbs more water than conventional concrete because of the high-water absorption capacity of expanded clay and basalt fiber. It is seen that the concretes without basalt fiber absorb lesser water.
3.4. Durability
The basalt fiber significantly improved the toughness and crack resistance performance of concrete. The enhancing effect of the basalt fiber on the compressive strength of concrete is lower than that of tensile strength and flexural strength.
The density highly influenced the mechanical properties of it. illustrate the weight and density of the concrete cube specimens after water immersion. A denser concert cube will have small voids and higher strength. The lower voids it contains, less permeable to water and soluble elements it becomes (Carolina, Citation2015). Hence, it improves its durability (Afroz et al., Citation2017; Reddy et al., Citation2013) Besides, the void spaces have effects on the penetration of water and sorptivity. The depth of penetration of water should not be more than 25 mm if not the specimen will fail in the permeability test (Parthiban et al., Citation2017). The rate of absorption can be determined by the sorptivity test in the capillary suction.
where ECC means expanded clay concrete and CC means conventional concrete.
The lower the percentage of basalt fiber added in the concrete mixture, the lower the water-resistance of the concrete, and vice-versa.
Figure 13. The density of the cube specimens immersed in distilled water for 96 hours after day 28 curing period
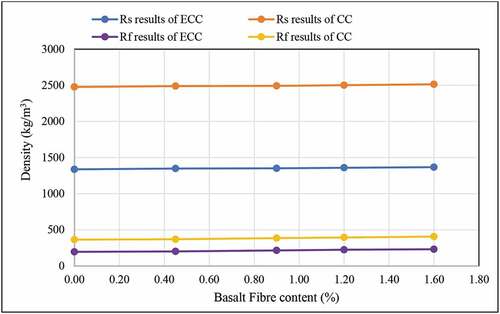
The concrete densities differ because of the percentage of BF added to the concrete mix for each specimen preparation. Thus, the density of the fiber added has an impact on the density of the concrete.
4. Conclusion
Based on this experimental work, the mechanical properties: compressive strength, flexural strength, water absorption, and durability of concrete improved significantly when used basalt fibers of 20 mm length, diameter 15 µm, and content between 0% and 1.6% by total volume.
The compressive strength of the ECC cube specimens (not immersed) increased from 21.92 MPa to 34.71 MPa. And, for the immersed, it is increased from 21.77 MPa to 37.38 MPa. Similarly, conventional concrete cube specimens (not immersed) increased from 15.37 MPa to 29.97 MPa. also, for the immersed, increased from 15.13 MPa to 29.97 MPa.
The flexural strength of the ECC rectangular prism specimens (not immersed) increased from 0.571 MPa to 2.921 MPa. And for immersed, it increased from 0.562 MPa to 2.895 MPa. Similarly, for conventional concrete rectangular prism specimens (not immersed) is increased from 0.411 MPa to 1.917 MPa. also, for the immersed, it is increased from 0.279 MPa to 1.733 MPa.
Based on experimental test observations, it can be concluded that expanded clay can absorb and retain much water and still give good strength better than granite as both are concrete aggregates.
Basalt fiber is a good material that is used as reinforcement or an aggregate which can retain water and still retain its strength or with very little loss in strength.
The combination of expanded clay concrete and basalt fiber gives the concrete more strength than the combination of conventional concrete and basalt fiber.
According to Dhand et al. (Dhand et al., Citation2014), basalt fiber is non-reactive toward the water and does not cause air pollution, it has a good heat-insulating capability, also being an ecologically pure substance, has a wide spectrum of applications. Unlike the conventional asbestos/glass fibers, which can cause health hazards, basalt fibers are non-hazardous as they are spun with a diameter higher than 6 lm. It is eco-friendly, non-toxic, and green (García et al., Citation2013; Hollaway, Citation1993; Paivaa et al., Citation2009; Taketa et al., Citation2010). Those researches and experimental works support our findings.
Further research
Further studies in the case of an alkali-aggregate reaction, chloride penetration, and acid attack are needed in process of this topic. Also, the economic perspectives.
Additional information
Funding
Notes on contributors
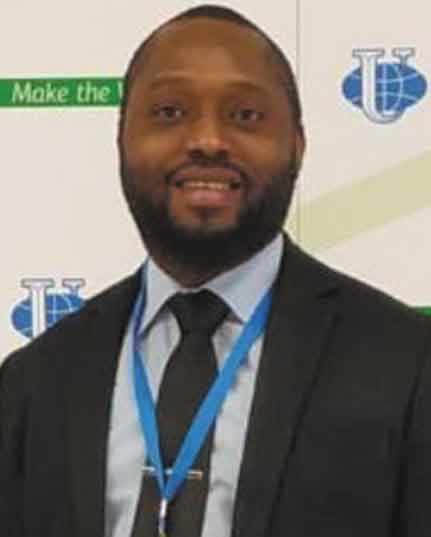
Paschal Chimeremeze Chiadighikaobi
Chiadighikaobi Paschal Chimeremeze is a Nigerian. He is a Civil Engineering Ph.D. postgraduate student at Peoples Friendship University of Russia (RUDN University). He holds His research interests are in; Structural materials, composite materials, structural design and analysis, computational civil engineering, steel frame stability analysis, computational mechanics of structural systems, concrete reinforcement, Nano-concrete, fiber dispersed concrete reinforcement, lightweight concrete, basalt fiber, finite element analysis, green city. Due to the challenges of affordable with high strength and durability, the author has been conducting research and experiments on the effects and uses of basalt fiber in concrete structures.
References
- Afroz, M., Patnaikuni, I., & Venkatesan, S. (2017). Chemical durability and performance of modified basalt fiber in concrete medium. Construction and Building Materials, 154(15), 191–21. https://doi.org/10.1016/j.conbuildmat.2017.07.153
- Alsayed, S. H., & Amjad, M. A. (1996). Strength, water absorption and porosity of concrete incorporating natural and crushed aggregate. Journal of King Saud University - Engineering Science, 8(1), 109–119. https://doi.org/10.1016/S1018-3639(18)30642-1
- Ash, B., & Paste, C. (2020). Engineering properties of basalt fiber reinforced. Materials, 13(1952), 1–15. https://doi.org/10.3390/ma13081952
- ASTM C127 – 15. (2015). Standard test method for relative density (specific gravity) and absorption of coarse aggregate. ASTM International, West Conshohocken, PA. https://doi.org/10.1520/C0127-15
- ASTM C128 – 15. (2015). Standard test method for relative density (specific gravity) and absorption of fine aggregate. , ASTM International, West Conshohocken, PA. www.astm.org
- ASTM C1585–13, (2013). standard test method for measurement of rate of absorption of water by hydraulic-cement concretes, ASTM International, West Conshohocken, PA
- ASTM C293/C293M – 16. (2016). Standard test method for flexural strength of concrete (using simple beam with center-point loading), ASTM International, West Conshohocken, PA. www.astm.org
- ASTM C78/C78M – 18. (2018). Standard test method for flexural strength of concrete (using simple beam with third-point loading)
- Azhani, Z., Ramli, N., Khairun, N., Mat, S., & Hossein, M. (2018). SEPKA-ISEED 2018. MATEC Web of Conf, 250(1016), 1-18. https://doi.org/10.1051/matecconf/201825001016
- Balakrishna, M. N., Mohamad, F., Evans, R., & Rahman, M. M. (2019). Assessment of water absorption of concrete by Initial surface absorption test Assessment of water absorption of concrete by Initial surface absorption test.Indian Journal of Engineering, 15, 57-65. http://www.discoveryjournals.org/engineering/current_issue/2018/A6.pdf
- Bogas, J. A., & Sofia, R. (2019). A review on the carbonation and chloride penetration resistance of structural lightweight aggregate concrete. Materials (Basel), 12(20), 3456. https://doi.org/10.3390/ma12203456
- British Standard Method of Testing Hardened Concrete for Other than Strength, British Standard Institute, London (1970) BS 1881: Part 5. British Standards Institution,London W4 4AL, UK.
- Carolina, S. (2015). Relation between density and compressive strength of hardened concrete. Concrete Research Letter, 6(4), 9. http://www.challengejournal.com/index.php/cjcrl/article/view/197
- Chahal, N., Siddique, R., & Rajor, A. (2012). Influence of bacteria on the compressive strength, water absorption and rapid chloride permeability of fly ash concrete. Construction and Building Materials, 28(1), 351–356. https://doi.org/10.1016/j.conbuildmat.2011.07.042
- Chiadighikaobi, P. C. (2020). Improving the compressive strength of lightweight cylindrical concrete column with basalt fiber reinforced polymer acting under imposed load. Structural Mechanics of Engineering Constructions and Buildings, 16(5), 424–434. https://doi.org/10.22363/1815-5235-2020-16-5-424-434
- Chiadighikaobi, P. C., Galishnikova, V. V., Kharun, M., Koroteev., D. D., & Dkhar, P. (2019). Compressive strength of basalt fiber reinforced concrete (BFRC) comparing on expanded clay and gravel as BFRC aggregate. IOP Conference Series: Materials Science and Engineering, 675, 012003, RUDN University, Moscow, Russian Federation. https://doi.org/10.1088/1757-899X/675/1/012003
- De Schutter, G., & Audenaert, K. (2004). Evaluation of water absorption of concrete as a measure for resistance against carbonation and chloride migration. Materials and Structures, 37(9), 591–596. https://doi.org/10.1617/14045
- Dhand, V., Mittal, G., Yop, K., Park, S., & Hui, D. (2014). A short review on basalt fiber reinforced polymer composites. Composites Part B, Elsevier Ltd, 73(December), 166–180. https://doi.org/10.1016/j.compositesb.2014.12.011
- Fractionated quartz sand. http://www.batolit.ru/93_p.shtml
- Gamal, T. S. F., & Chiadighikaobi, P. C. (2019). Comparative analysis of reliability of non-destructive methods of strength control of concrete impregnated with vegetable oil: Basalt fiber for increasing the concrete strength. Materials Today: Proceedings, 19(5), 2479–2482. https://doi.org/10.1016/j.matpr.2019.08.113
- García, P. D. L. R., Escamilla, A. C., & García, M. N. G. (2013). Bending reinforcement of timber beams with composite carbon fiber and basalt fiber materials. Composite Part B – Engineering, 55, 528–536. https://doi.org/10.1016/j.compositesb.2013.07.016
- GOST 10180–2012. (2013). Concretes. Methods for strength determination using reference specimens (pp. 30).
- Granite fabricator. https://www.granitefabricatordirect.net/are-granite-countertops-water-resistant/
- Guo, Z., Wan, C., Xu, M., & Chen, J. (2018). Review of basalt fiber-reinforced concrete in china: Alkali resistance of fibers and static mechanical properties of composites. Advanced Material Science and Engineering, 2018. Article ID 9198656. 11. https://doi.org/10.1155/2018/9198656
- High, C., Seliem, H. M., El-safty, A., & Rizkalla, S. H. (2015). Use of basalt fibers for concrete structures. Construction and Building Materials, 96, 37–46. https://doi.org/10.1016/J.CONBUILDMAT.2015.07.138
- Hollaway, L. (1993). Polymer composites for civil and structural engineering. Springer.
- Irwan, J. M., Anneza, L. H., Othman, N., & Alshalif, A. F. (2016). Compressive strength and water penetration of concrete with enterococcus faecalis and calcium lactate. Engineering Materials, 705, 345–349. https://doi.org/10.4028/www.scientific.net/KEM.705.345
- Kamseu, E., Ponzoni, C., Tippayasam, C., Taurino, R., Chaysuwan, D., Bignozzi, M. C., Barbieri, L., & Leonelli, C. (2015). Influence of fine aggregates on the microstructure, porosity, and chemico-mechanical stability of inorganic polymer concretes. Constructions and Building Materials, 96(15), 473–483. https://doi.org/10.1016/j.conbuildmat.2015.08.090
- Kim, H. K., Jeon, J. H., & Lee, H. K. (2012). Flow, water absorption, and mechanical characteristics of normal- and high-strength mortar incorporating fine bottom ash aggregates. Construction and Building Materials, 26(1), 249–256. https://doi.org/10.1016/j.conbuildmat.2011.06.019
- Kim, Y. H., Park, J. M., Yoon, S. W., Lee, J. W., Murakami, R. I., & Jung, M. K. (2011). The effect of moisture absorption and gel-coating process on the mechanical properties of the basalt fiber reinforced composite. International Journal of Ocean System Engineering, 1(3), 148–154. https://doi.org/10.5574/IJOSE.2011.1.3.148
- LECA. https://www.leca.co.uk/drainage-properties-of-lecar-lwa/
- Levy, S. M., & Helene, P. (2004). Durability of recycled aggregates concrete: A safe way to sustainable development. Cement and Concrete Research, 34(11), 1975–1980. https://doi.org/10.1016/j.cemconres.2004.02.009
- Liu, Q., Shaw, M. T., Parnas, R. S., & McDonnell, A. M. (2006). Investigation of basalt fiber composite aging behavior for applications in transportation. Polymer Composites, 27(5), 475–483. https://doi.org/10.1002/pc.20215
- Lopez, M., Lawrence, F. K., & Kimberly., E. K. (2008). Effect of internally stored water on creep of high-performance concrete. ACI Materials Journal, 105(3), 265–273.
- Maruthupandian, S. (2018). Durability studies on basalt fibre reinforced concrete. Indian Concrete Journal, 92(4). https://www.researchgate.net/publication/325284257_Durability_Studies_on_Basalt_Fibre_Reinforced_Concrete
- Matarul, J., Mannan, M. A., Mohammad Ibrahim Safawi, M. Z., Ibrahim, A., Jainudina, N. A., & Yusuh, N. A. (2016). Performance-based durability indicators of different concrete grades made by the local ready mixed company: Preliminary results. Procedia – Social and Behavioral Sciences, 224:, 620–625. https://doi.org/10.1016/j.sbspro.2016.05.452
- Md Noor, N., Xiang-Ong, J., Mohd Noh, H., Abdul Hamid, N. A., Kuzaiman, S., & Adiwijaya, A. (2017). Compressive strength, flexural strength, and water absorption of concrete containing palm oil kernel shell. IOP Conference Series Material Science and Engineering, 271, 012073. https://doi.org/10.1088/1757-899X/271/1/012073
- Md., I., Sharmin, N. S., Md., M., & Akhtar, U. S. U. (2016). Effect of soda lime silica glass waste on the basic properties of clay aggregate. International Journal of Science and Engineering Research, 7(4), 149–153. https://www.ijser.org/researchpaper/Effect-of-Soda-lime-Silica-Glass-waste-on-the-basic-properties-of-Clay-Aggregate.pdf
- Monaldo, E., Nerilli, F., & Vairo, G. (2019). Basalt-based fiber-reinforced materials and structural applications in civil engineering. Composite Structures, 214(15 April 2019), 246–263. https://doi.org/10.1016/j.compstruct.2019.02.002
- Moravia, W. G., Oliveira, C. A. S., Gumieri, A. G., & Vasconcelos, W. L. (2006). Concrete Structural Leve Cerâmica, 52(322), 193–199. https://doi.org/10.1590/S0366-69132006000200012
- Paivaa, J. M. F. D., Santos, A. D. N. D., & Rezende, M. C. (2009). Mechanical and morphological characterizations of carbon fiber fabric reinforced epoxy composites used in aeronautical field. Material Research, 12(3), 367–374. https://doi.org/10.1590/S1516-14392009000300019
- Parthiban, T., Pavithran, G., & Pradeep, C. (2017). Durability of concrete by adding basalt fibre. International Research Journal of Engineering Technology, 4(9), 1491–1494. https://www.irjet.net/archives/V4/i9/IRJET-V4I9281.pdf
- Reddy, M. V. S., Ramana, R., Madan,, M., Reddy, K., & Ravi Kumar, C. M. (2013). Durability aspects. International Journal of Structural and Civil Engineering Research, 2(1), 40–46. http://www.ijscer.com/uploadfile/2015/0427/20150427030157970.pdf
- RILEM TC-196. (2007). Internal curing of concrete. In K. Kovler & O. M. Jensen. (Eds.), State-of-the-art Report of RILEM Technical Committee 196-ICC. RILEM Publications S.A.R.I. Bagneux, France, 2007), 5-13.
- Sami Elshafie, G. W. (2015). A review of the effect of basalt fibre lengths and proportions on the mechanical properties of concrete. International Journal of Research in Engineering and Technology, 04(Jan 01), 458-465. http://usir.salford.ac.uk/id/eprint/51070/.
- Sepehr, M. N., Kazemian, H., Ghahramani, E., Amrane, A., Sivasankar, V., & Mansur, Z. (2014). Defluoridation of water via lightweight expanded clay aggregate (LECA): Adsorbent characterization, competing ions, chemical regeneration, equilibrium, and kinetic modeling. Journal of Taiwan Institute of Chemical Engineers, 45(4), 1821–1834. https://doi.org/10.1016/j.jtice.2014.02.009
- Shaikh, F. U. A. (2016). Mechanical and durability properties of fly ash geopolymer concrete containing recycled coarse aggregates. International Journal of Sustainable Built Environment, 5(2), 277–287. https://doi.org/10.1016/j.ijsbe.2016.05.009
- SILICA FUME MK-85. ADDITIVE IN CONCRETE http://www.geogips.ru/catalog/cement_i_dobavki/plasticizer-accelerator/microsilica-mk-85_10kg/
- Sim, J., Park, C., & Moon, D. Y. (2005). Characteristics of basalt fiber as a strengthening material for concrete structures. Materials, 36(6-7), 504–512. https://doi.org/10.1016/j.compositesb.2005.02.002
- Slater, E., Moni, M., & Alam, M. S. (2012). Predicting the shear strength of steel fiber reinforced concrete beams. Construction and Building Materials, 26(1), 423–436. https://doi.org/10.1016/j.conbuildmat.2011.06.042
- Taketa, I., Ustarroz, J., Gorbatikh, L., Lomov, S. V., & Verpoest, I. (2010). Interpolymer hybrid composites with carbon fiber reinforced polypropylene and self-reinforced polypropylene. Composite Part A – Applied Science, 41(8), 927–932. https://doi.org/10.1016/j.compositesa.2010.02.003
- Thomas, M. D. A. (2007). Optimizing the use of fly ash in concrete (pp. 24). Portland Cement. Association.
- Zhou, H., Jia, B., Huang, H., & Mou, Y. (2020). Experimental study on basic mechanical properties of basalt fiber- reinforced concrete. Materials, 13(6), 1362. https://doi.org/10.3390/ma13061362