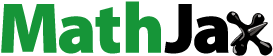
Abstract
It is very rare to have the ions of only one metal ion in wastewater. Hence, the removal of copper and lead ions from aqueous solution in single and binary systems using natural banana peels was investigated in a batch mode. Banana peels were characterised using FTIR, which gave the functional groups present. The spectra of banana peels loaded with Pb(II) had a higher percentage transmittance after adsorption. The point of zero charge pHpzc of banana peel was found to be 4.83 which makes it suitable for the adsorption of cations. Kinetic and isotherm studies were carried out at a pH of 5.5, concentration range of 10–100 mg/L, and adsorbent dosage range of 0.1–1 g. A pseudo-second order model is fitted to the kinetic data suggesting chemical adsorption. The Langmuir isotherm performed well with the experimental data which means adsorption occurred as a monolayer on the surface of banana peels. The maximum adsorption capacity of Pb(II) for Langmuir isotherm was 66.67 mg/g. This indicated that in the single system banana peels have more affinity for Pb(II) than for Cu(II). At higher concentrations, the experimental data for the isotherm study showed the effect of a competitive phenomenon which affected only Cu(II) in the binary system. Natural banana peels are efficient agricultural waste materials that can be used for the treatment of wastewater contaminated with copper and lead ions.
PUBLIC INTEREST STATEMENT
South Africa is a highly industrialized country with a lot of mining activities and chemical industries. Wastewater generated from these industrial activities contains heavy metals which contaminate waterbodies, thereby posing a risk to human life which results in various diseases.
Bio-sorbents derived from agricultural sources contain functional groups that enhance the adsorption of heavy metal ions in wastewater. South Africa produces 380, 000 tons of banana annually, some of which are exported and used to produce fruit juice. Banana waste constitutes a large fraction of food leftovers generated from households, market areas and restaurants. These wastes can be used as low-cost, readily available, and eco-friendly adsorbents for the removal of copper and lead from wastewater thereby reducing environmental pollution. This study investigated the capacity of natural banana peels to remove copper and lead ions from wastewater in a single and binary solute system.
1. Introduction
Pure and uncontaminated water has become a globally highly sought-after commodity in this 21st century. The earth surface contains more than 71% of water. However, according to the international standard, less than 1% is suitable for drinking (N.B Singh et al., Citation2018). This is as a result of various contaminants that get into the waterbodies. Wastewater effluents from industrial activities, sewage sludge, agricultural activities, and environmental changes are the main sources of these pollutants. These pollutants include toxic heavy metals, dyes, pesticides, organic compounds, and fertilizers. The presence of heavy metals in water poses a serious threat to human and aquatic life, as well as the environment. Toxic heavy metals include arsenic, cadmium, chromium, copper, lead, manganese, nickel and mercury (A. Singh et al., Citation2010; Nour T. Abdel-Ghani & El-Chaghaby, Citation2014; N.B Singh et al., Citation2018).
Among these pollutants, lead and copper are the most common heavy metals found in wastewater. They have severe environmental impacts on the waterbodies worldwide (Iqbal & Khera, Citation2015). These heavy metals are generated from industries, such as electroplating, electrolytic deposition, printing, pigments and explosives manufacturing, production of coatings, milling, and etching (Barakat, Citation2011). The presence of lead and copper ions in low concentrations of water is a risk to human life. This can result in various diseases (Gunatilake, Citation2015; Ahmed and Ahmaruzzaman 2016; N.B Singh et al., Citation2018). The World Health Organization has set permissible limits for lead and copper in drinking water at 0.01 and 2 ppm, respectively (WHO, Citation2003). The treatment of wastewater is therefore important as the international laws and regulatory agencies are becoming stricter on the implementation of effluent standards. However, most emerging industries might not be able to comply with these pollution control standards because of the high operational cost involve in removing the pollutants.
In order to remove the heavy metals, the wastewater is passed through treatment stages before being discharged into the environment, with each stage aimed at removing specific contaminants. This research work is focused on secondary effluent wastewater which has gone through treatment in the municipal plant and contains heavy metals in lower concentrations than when it entered the municipal treatment works, but needs to be treated further to conform to the environmental rules and regulations. It is therefore important to develop efficient and low-cost techniques for the removal of these toxic heavy metals at this treatment stage. There are various methods of removing heavy metals from wastewater, such as coagulation, oxidation—reduction, chemical and electrochemical precipitation, ion exchange, and membrane separation (Semerjian, Citation2018). These methods have drawbacks: high operational cost, high volumes of sludge generated, low selectivity, and imperfect removal of heavy metal ions, particularly when the metal ion concentration is low (Sadeek et al., Citation2015). Adsorption is an alternative technique for the removal of heavy metals from wastewater. It is more flexible, efficient and cost-effective, and guarantees complete removal at low concentrations.
Bio-sorption is the use of natural adsorbents for the removal of adsorbates from solution. These natural adsorbents are referred to as the bio-sorbent. They are derived from agricultural waste, industrial by-products, and biopolymers. Agricultural waste has been proven over the years to be efficient for the removal of heavy metals from wastewater as they contain functional groups, which can achieve maximum removal of the metal ions. Some of these agricultural wastes are pre-treated or modified to increase the adsorption capacity. These agriculture-based adsorbents are low-cost, readily available, easy to process, and can be regenerated (Iqbal & Khera, Citation2015; Zamani Beidokhti et al., Citation2019). Several bio-sorbents have been used for the removal of heavy metals, such as cabbage (M. A. Hossain et al., Citation2014), orange peel (Lugo-Lugo et al., Citation2012), moringa oleifera seeds (Araujo et al., Citation2013), potato peels (Guechi et al., Citation2016), corn cob (Mahmoud, Citation2016) and palm tree leaves (Soliman et al., Citation2016).
Bio-sorption of single metal ions using agricultural waste has been studied extensively, and the isotherm models for mono-metal ion bio-sorption have been used to characterize the adsorption process. Less focus has been given to multi-metal ion bio-sorption systems. It is very rare to have just a single metal ion in wastewater; hence, research studies have recently focused on systems containing two or more metal ions (Iqbal & Khera, Citation2015; Martín-Lara et al., Citation2016; Medellin-Castillo et al., Citation2017). These studies have explained the effects of coexistence of metal ions relating to the adsorption capacity of the metal ions involved and the affinity of the bio-sorbent used for each of the metal ions. Therefore, the modelling, interpretation and presentation of the multi-metal ion system adsorption mechanism is more complex than that of the single metal ion system due to the competitive affinity of the metal ions for the active-binding sites (Walton & Sholl, Citation2015).
The present study aims to investigate the equilibrium characteristics and kinetics of bio-sorption of copper and lead in both single and binary systems using banana peels. Banana is a fruit that is mostly consumed in large quantities worldwide. However, the discarded unwanted peels also contribute significantly to the waste generated from domestic, market and food industry garbage. Banana peels consist of cellulose, hemicellulose, pectin, and lignin which are made up of functional groups such as the carboxyl, hydroxyl and amine groups. These functional groups are responsible for the performance of this bio-sorbent in the removal of metal ions from aqueous solutions. Although works have been reported on the bio-sorption of metal ions using banana peels (Arunakumara et al., Citation2013; Deshmukh et al., Citation2017; Mondal & Roy, Citation2018), those works have not dealt with the coexistence of metal ions in solution using this bio-sorbent is not significant, hence the focus of this study. Equilibrium and kinetic studies are important for industrial-scale design to determine the reaction times and the size of the columns.
2. Materials and method
2.1. Preparation of bio-sorbent and synthetic solution
The bio-sorbent used in this study was prepared as reported by (Afolabi et al., Citation2021). All the chemicals used were of analytical grade purchased from Laboratory Analytical Supplies Limited, South Africa. Stock solutions (1000 mg/L) of Pb(NO3)2 and Cu(NO3)2.3H2O were prepared. These stock solutions were also used for the mixture of the two metals for binary competitive adsorption. Different initial concentrations of the metal solutions were prepared by serial dilution of the stock solution. The solution pH was determined using a digital pH meter (edgepH HI 2002, USA) with the aid of a digital pH electrode with an integrated temperature sensor calibrated with a standard buffer solution. The pH of the solution was adjusted by adding drops of 0.1 M H2SO4 or 0.1 M NaOH.
2.2. Analytical instruments
Fourier transform infrared spectroscopy (FTIR) was used to determine the functional groups present in the banana peels before and after adsorption. The FTIR wavenumber and intensity were obtained from spectrum 10 software (Perkin Elmer). The batch experiments were conducted using the jar test equipment (JLT 6 jar test, VELP Scientifica Srl) while the composition of Pb(II) and Cu(II) in the aqueous solution after adsorption was analysed using a micro-plasma atomic emission spectrophotometer (MP-AES, MY 18,379,001, Agilent).
2.3. Batch adsorption
All the adsorption experiments were conducted at room temperature. Kinetic experiments were carried out with single and binary solutions of Cu(II) and Pb(II) at pH 5.5. For kinetic studies, the actual dosage of 1 g of the processed banana peels was added to 1 L solution containing either Cu(II), Pb(II) or a mixture of both solutes. The initial concentrations of Cu(II) and Pb(II) in each of the solutions were the same (10, 55 and 100 mg/L). The beakers containing the solutions were put in the jar test equipment which rotates at 180 rpm, and liquid samples were taken at intervals (0.25, 0.5, 1, 2, 3, 4, 5, 6, and 24 h). The samples were filtered using Whatman grade 1 filter paper and then 0.25 µm syringe filters.
The single and binary adsorption isotherm experiments were carried out at a pH of 5.5. For the single solute, the experiments were conducted with 0.5 g of the processed banana peel in a solution containing either Cu(II) or Pb(II) with an initial concentration of 10, 25, 50, 60, 80, 100, 125, 150, 180 and 200 mg/L. For the binary solute, the same amount of adsorbent dosage was used with the solution containing the mixture of Cu(II) and Pb(II), each having the same initial concentration as in the single solutes. The beakers were covered with foil paper and shaken for 2 h at 180 rpm, after which the supernatant portion was filtered using Whatman grade 1 filter paper and 0.25 µm syringe filters. All the isotherm experiments were conducted in triplicate, while the average of the values was reported. The samples were then analyzed to determine the amount of Cu(II) and Pb(II) remaining after adsorption. The quantity adsorbed was calculated using Equationequation (1)(1)
(1) :
where qe (mg/g) is the quantity adsorbed at equilibrium, Co and Ce (mg/L) are the initial and final concentrations, respectively, V (L) is the volume of the solution and m (g) is the mass of the adsorbent used .
2.4. Adsorption models
2.4.1. Adsorption kinetic studies
The kinetic study is used to determine the rate of the adsorption process. It explains the adsorption process with time. Therefore, for industrial scale, the reaction efficiency is a function of the rate of adsorption and the sorption capacity. Hence, a fast kinetic will require smaller columns while a slow rate of adsorption will necessitate a long column to achieve maximum adsorption uptake. The pseudo-first- and pseudo-second-order kinetic models were employed to describe the adsorption mechanism of Cu(II) and Pb(II) onto banana peels. The pseudo-first order model is given in Equationequation (2)(2)
(2) :
The pseudo-second order model (Equationequation (3)(3)
(3) ) is expressed as;
where qe and qt are the quantities adsorbed at equilibrium (mg/g) and at time t (h) respectively, K1 is the pseudo-first order reaction rate constant (h) and K2 is the reaction rate constant of the pseudo-second order model (g mg−1 h−1).
2.4.2. Adsorption isotherm studies
Langmuir and Freundlich isotherm models are mostly used in adsorption studies to characterize the equilibrium of the adsorption process. The Langmuir isotherm model assumes a monolayer adsorption and an adsorption energy that is the same for all sites. The model equation is stated below:
where qmax is the maximum adsorption capacity of the metal ion (mg/g); b is the Langmuir constant (L/mg); qe is the equilibrium adsorption capacity (mg/g) and Ce is the final metal concentration at equilibrium (mg/L). qmax and b can be obtained from the linear form of the plot of Ce/qe versus Ce as the intercept and slope, respectively. The Freundlich isotherm model assumes multilayer adsorption and the adsorption energy being distributed exponentially on the active sites. The model equation is as stated below (Equationequation (5)(5)
(5) ).
where qe is the equilibrium adsorption capacity (mg/g) and Ce is the equilibrium concentration (mg/L). Kf and n are the Freundlich constants (L/g).
3. Results and discussion
3.1. Characterization of bio-sorbent
3.1.1. Physical properties of banana peels
The point of zero charge pHpzc of an adsorbent is the point at which the charge on the adsorbent becomes neutral. shows that the pHpzc of banana peel is 4.83, which implies that the surface of banana peel is acidic and therefore, favors the adsorption of cations. However, reports have also shown that banana peels can be used for the adsorption of both cations and anions when the pHpzc is close to neutral (Pathak et al., Citation2017).
3.1.2. FTIR spectroscopy analysis
The FTIR spectra of the banana peel before and after adsorption under both single and binary conditions were measured between 500 and 4000 cm −1 as shown in . The FTIR spectroscopy is mostly used to identify the functional groups present on the surface of an adsorbent. The peaks close to 3400 cm−1 and 1500 cm−1 shown for all samples are identified as OH stretching, peaks near 2950 cm−1 are observed to be CH stretching, the peak near 1750 cm−1 is C = O while the peak at 1615 cm−1 is assigned to C = C stretching band as similarly reported (Bhaumik & Mondal, Citation2014; Deshmukh et al., Citation2017). There are similarities in the shape and band positions of the spectra of banana peel (), after the single adsorption of the metal ions as well as the binary adsorption. The FTIR spectra of banana peels after adsorption of Cu2+ and Pb2+ have similar features to the natural banana peels; however, there are significant changes in the percentage transmittance of the peaks. The following peaks: 3325, 2950, 2850, 1750, 1615, 1587, 1484, 1020 and 802 cm−1 on natural banana peels shifted to 3340, 2932, 2845, 1732, 1616, 1584, 1487, 1024 and 904 cm−1 respectively after adsorption of Cu and Pb ions. This reveals that the functional groups interact with the metal ions in solution during the adsorption process through electrostatic attraction. The functional group identification is very important because the metal-binding capacity of a bio-sorbent depends on the presence of functional groups such as carboxylic and hydroxylic groups.
3.2. Single and binary adsorption isotherms
The isotherm studies were carried out with varying initial heavy metal ion concentrations and a fixed adsorbent dosage. The experimental data and the linear fit of the isotherm models of Cu(II) and Pb(II) ions under single and binary conditions are presented in .
Figure 4. Adsorption isotherms of Cu and Pb on banana peel in single and binary systems at pH 5.5. The symbols are the experimental results while the solid lines are the linear fittings of (a) Cu Langmuir adsorption, (b) Pb Langmuir adsorption, (c) Cu Freundlich adsorption and (d) Pb Freundlich adsorption models
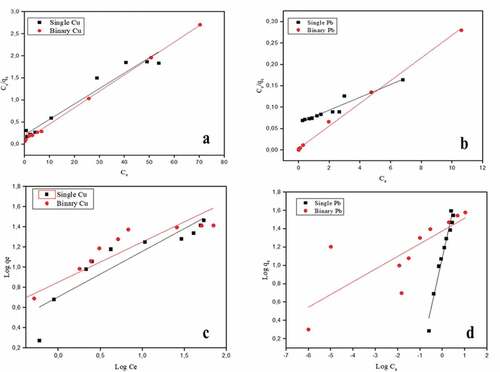
The adsorption increased with increasing initial concentration for both single and binary systems, the maximum adsorption capacity (qmax) was 25.23, 29.96, 37.32, and 39.32 mg/g for single Cu, binary Cu, single Pb, and binary Pb, respectively. The adsorption capacity reduced for both metals in the binary system. The separation factor “RL” which describes the type of adsorption isotherm for the Langmuir isotherm is stated below.
where b is the Langmuir constant and Ce is the equilibrium concentration for each initial concentration.
For all the systems of Cu(II) and Pb(II), the separation factor “RL” was calculated and found in the range of 0.9 to 0.01 which lies within 0 and 1, suggesting that the adsorption is favourable (Deshmukh et al., Citation2017). As shown in , the Langmuir constant value “n” for Pb(II) was higher than that for Cu(II) in both systems, suggesting that Pb(II) had a higher affinity for the active sites on the banana peel surface than Cu(II). The Freundlich isotherm constant “n” which measures the adsorption intensity of both metals was observed to be greater than 1 which suggests that the adsorption process is physical, except for Pb(II) single system which was lower than 1 indicating the adsorption is a chemical process. From , comparing the correlation values (R2) for Langmuir and Freundlich isotherms, the Langmuir model fitted the experimental data well.
Table 1. Langmuir and Freundlich isotherm parameters
In the binary adsorption, Pb(II) was adsorbed more on the surface sites than Cu(II), similar to the observation in the single system. Also, the presence of Cu(II) had no significant effect on Pb(II) adsorption, while Cu(II) adsorption was influenced at higher concentrations by the coexistence of Pb(II). The quantity adsorbed for Cu(II) increased at the initial concentration of 125 mg/L from 19.22 mg/L to 23.64 mg/L. This could be because the concentration of Pb(II) in the solution had reduced and there was less competition for active sites.
3.3. Adsorption kinetics
The percentage removal curves of Pb(II) and Cu(II) at varying initial concentration for both single and binary systems are shown in . The adsorption of Pb(II) and Cu(II) progressed rapidly in both systems, while equilibrium was reached within the first 5 h. However, certain differences can be observed in the adsorption curves of the two metals as regards the varying initial concentrations. The percentage removal of single Pb(II) at 10 ppm reached 100% within 4 h which suggest that there was complete adsorption at equilibrium whereas single Cu(II) at the same concentration was 95% adsorbed at equilibrium. Similarly, the percentage removal of single Pb(II) at 55 ppm initial concentration reached 98% while the percentage removal of single Pb(II) at 100 ppm reduced significantly with approximately 43% at equilibrium. This means that at 55 ppm, the active sites on the surface of banana peels have reached saturation for single Pb(II). Many researchers have reported that the percentage removal of metal ions decreases as the initial metal ion concentration increases. Iqbal and Khera (Citation2015) and Semerjian (Citation2018) reported that the percentage removal of Cu(II) and Pb(II) decreased as the initial metal ions increased. The percentage removal of single Cu(II) at initial concentrations 55 ppm and 100 ppm reached equilibrium at 18% which suggest that the active sites are saturated and banana peels have less affinity for Cu(II).
Figure 5. Percentage removal of (a) Pb(II) and (b) Cu(II) onto banana peels at different initial concentrations and pH 5.5 in both single and binary systems
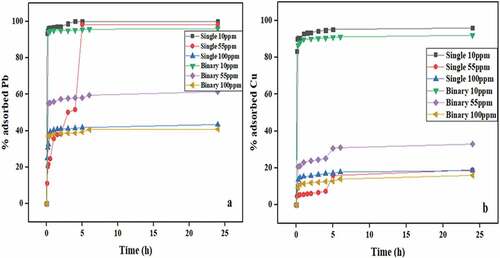
In the case of the binary solutes, the adsorption of binary Pb(II) and Cu(II) at initial concentration 10 ppm was very rapid and equilibrium was reached at approximately 96% and 92%, respectively. This indicates that both metals competed well for the active sites; however, the adsorption of Pb(II) was faster than Cu(II). The percentage removal of binary Pb(II) at initial concentrations 55 ppm and 100 ppm was higher than for Cu(II) at the same initial concentrations. The percentage adsorbed for the binary system was in the order Pb(II) 10 ppm > Cu(II) 10 ppm > Pb(II) 55 ppm > Pb(II) 100 ppm > Cu(II) 55 ppm > Cu(II) 100 ppm with values of 96%, 92%, 61%, 41%, 33%, and 16%, respectively. It indicates that Pb(II) has a greater affinity for banana peels than Cu(II). It is obvious that the percentage removal of Pb(II) was higher than Cu(II) for all the initial concentrations. In addition, the quantity adsorbed increased as the initial concentration increased. However, the adsorption efficiency decreased as the initial concentration increased since the amount of the adsorbent in solution remains the same. At higher initial concentration, the amount of metal ions in solution is higher compared to the available active sites on the surface of the bio-sorbent, hence decreasing the percentage removal of the metal ions.
The linear form of the pseudo-first-order and pseudo-second-order kinetic models was used to analyze the kinetic adsorption experimental data, as depicted in . The model parameters for the two kinetic models are listed in . The kinetic study for Pb(II) and Cu(II) was carried out for both single and binary systems at the initial concentrations of 10, 55, and 100 mg/L to explain the sensitivity of the adsorption process of the metal ions in coexistence. The adsorption kinetics of Pb(II) and Cu(II) in both systems at various initial concentrations fitted the pseudo-second order model well with correlation coefficient (R2) very close to 1, which suggests that the adsorption rate is controlled by a chemical adsorption process. The equilibrium adsorption capacity of Pb(II) increased with increasing initial concentration for both single and binary systems. The lower value of K2 for Cu(II) in the binary system as compared to Pb(II) suggests that the adsorption rate of Cu(II) is negatively affected by the presence of Pb(II).
Figure 6. Kinetic model plots of Pb(II) and Cu(II) at different initial concentrations; (a) pseudo-first order single, (b) pseudo-first order binary, (c) pseudo-second order single, (d) pseudo-second order binary
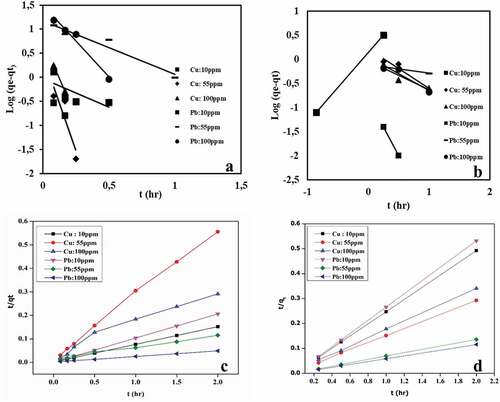
Table 2. Kinetic model parameters obtained from pseudo-first order model and pseudo-second order model
3.4. Comparison of Pb2+ and Cu2+ uptake using banana peels and other bio-sorbents
The adsorption uptakes of Pb2+ and Cu2+ onto banana peels in single and binary systems obtained were 66.67 mg/g (single), 37.69 mg/g (binary) for Pb2+ and 28.56 mg/g (single), 26.89 mg/g (binary) for Cu2+. The adsorption of Pb2+ was higher than Cu2+ in both systems. The maximum adsorption uptake of Pb2+ and Cu2+ using banana peels in this study is also compared with other bio-sorbent uptake data available in literature ().
Table 3. Comparison of single solute adsorption capacity (qmax) of Pb2+ and Cu2+ with other bio-sorbents
4. Conclusions
This study was carried out to explore the capacity of banana peels for the removal of Pb(II) and Cu(II) in both single and binary systems to determine the effect of the coexistence of these metal ions in the adsorption process. The adsorbent characterization was done by pHpzc and FTIR analysis. The surface of the banana peels was found to be acidic, hence an increase in the solution pH led to a significant increase in the adsorption capacity of the bio-sorbent. Also, the FTIR measurements showed the presence of acid groups (-OH and -COOH) on the surface of banana peels. These functional groups are deprotonated in solution; hence, the bio-sorbent surface becomes negatively charged thereby attracting Pb(II) and Cu(II) ions in solution by electrostatic interaction.
In both single and binary metal ion systems, the adsorption uptake of Pb(II) was higher than Cu(II), suggesting that banana peels have a greater affinity for Pb(II). This may be due to the fact that the ionic radius of Pb(II) is smaller than Cu(II), which makes it easier to be adsorbed on the active sites. The adsorption equilibrium was well described by Langmuir isotherm models for Pb(II) and Cu(II) in both single and binary systems. The adsorption of Cu(II) was sensitive to the presence of Pb(II) in the binary systems; the adsorption of Cu(II) was similar to that in the single system at lower concentrations until Pb(II) adsorption reached equilibrium, while the presence of Cu(II) had no influence on the adsorption of Pb(II). Hence, the existence of a competitive phenomenon was observed at higher initial concentration that affected only the adsorption of Cu(II).
The kinetic model followed pseudo-second order for both single and binary systems. Hence, the mechanism of adsorption is chemisorption. The kinetic process was rapid which suggests that a smaller column is required for the bio-sorption in an industrial treatment plant. Therefore, it can be concluded that banana peels are a suitable agricultural waste for the removal of Pb(II) and Cu(II) from water.
List of Nomenclature
b Langmuir isotherm constant (L/mg)
C0 Initial metal ion concentration (mg/L)
Ct Concentration at a time t (mg/L)
Ce Concentration at equilibrium (mg/L)
Kf Freundlich equilibrium constant (L/mg)
K1 Pseudo-first-order rate constant (h)
K2 Pseudo-second-order rate constant (g mg−1 h−1).
M Mass of adsorbent (g)
n Reciprocal of the reaction order
qt Adsorption capacity at time t (mg/g)
qe Adsorption capacity at equilibrium (mg/g)
qmax Maximum adsorption capacity (mg/g)
R2 Correlation coefficient
V Volume of solution (mL)
Acknowledgements
The authors appreciated the financial support of the National Research Foundation (Grant Nu: 105235) and the Institute of Wastewater Treatment, Durban University of Technology for the MP – AES analysis.
Disclosure statement
The authors declare no conflict of interest.
Additional information
Funding
Notes on contributors
Felicia O Afolabi
This research is part of a larger project funded by the South African National Research Foundation (NRF) and has been running for the past 3 years. Research activities seek to find sustainable and eco-friendly solutions for the removal of heavy metals commonly found in South African waters and detrimental to life. To this end, a selected number of readily available and low-cost bio-waste adsorbents such as sugarcane bagasse, orange peel, eggshell, mango peel, banana peel etc, are being explored. The study covers their characterization, batch experiments to study the adsorption mechanisms and kinetics, as well as pilot-scale column studies for scale-up and design of household filters and industrial-scale adsorption columns. The designed equipment should be capable of handling multi-solute systems.
References
- Abdel-Ghani, N. T., & El-Chaghaby, G. A. (2014). Biosorption for metal ions removal from aqueous solutions: A review of recent studeis. International Journal of Latest Research in Science and Technology, 3(1), 24–14. https://scholar.google.com/
- Afolabi, F. O., Musonge, P., & Bakare, B. F. (2021). Evaluation of lead (II) removal from wastewater using banana peels: Optimization study. Polish Journal of Environmental Studies, 30(2), 1–10. https://doi.org/10.15244/pjoes/122449
- Anwar, J., Shafique, U., Waheed Uz, Z., Salman, M., Dar, A., & Anwar, S. (2010). Removal of Pb(II) and Cd(II) from water by adsorption on peels of banana. Bioresource Technology, 101(6), 1752–1755. http://dx.doi.org/10.1016/j.biortech.2009.10.021
- Araujo, C. S. T., Carvalho, D. C., Rezende, H. C., Almeida, I. L. S., Coelho, L. M., Coelho, N. M. M., Marques, T. L., & Alves, V. N. (2013). Bioremediation of waters contaminated with heavy metals using moringa oleifera seeds as biosorbent. INTECH. 227-255. https://doi.org/10.5772/56157
- Arunakumara, K., Walpola, B. C., & Yoon, M.-H. (2013). Banana peel: A green solution for metal removal from contaminated waters. Korean Journal of Environmental Agriculture, 32(2), 108–116. http://dx.doi.org/10.5338/KJEA.2013.32.2.108
- Barakat, M. A. (2011). New trends in removing heavy metals from industrial wastewater. Arabian Journal of Chemistry, 4(4), 361–377. http://dx.doi.org/10.1016/j.arabjc.2010.07.019
- Bhaumik, R., & Mondal, N. K. (2014). Optimizing adsorption of fluoride from water by modified banana peel dust using response surface modelling approach. Applied Water Science, 6(2), 115–135. http://dx.doi.org/10.1007/s13201-014-0211-9
- Da Silva Correia, I. K., Santos, P. F., Santana, C. S., Neris, J. B., Luzardo, F. H. M., & Velasco, F. G. (2018). Application of coconut shell, banana peel, spent coffee grounds, eucalyptus bark, piassava (Attalea funifera) and water hyacinth (Eichornia crassipes) in the adsorption of Pb2+ and Ni2+ ions in water. Journal of Environmental Chemical Engineering, 6(2), 2319–2334. https://doi.org/10.1016/j.jece.2018.03.033
- Deshmukh, P. D., Khadse, G. K., Shinde, V. M., & Labhasetwar, P. (2017). Cadmium removal from aqueous solutions using dried banana peels as an adsorbent: Kinetics and equilibrium modeling. Journal of Bioremediation & Biodegradation, 08(3), 395. https://doi.org/10.4172/2155-6199.1000395
- Guechi, E.-K., Hamdaoui,, & Hamdaoui, O. (2016). Evaluation of potato peel as a novel adsorbent for the removal of Cu (II) from aqueous solutions: Equilibrium, kinetic, and thermodynamic studies. Journal of Desalination and Water Treatment, 57(23), 10677–10688. https://doi.org/10.1080/19443994.2015.1038739
- Guiza, S. (2017). Biosorption of heavy metal from aqueous solution using cellulosic waste orange peel. Ecological Engineering, 99, 134–140. http://dx.doi.org/10.1016/j.ecoleng.2016.11.043
- Gunatilake, S. (2015). Methods of removing heavy metals from industrial wastewater. Journal of Methods, 1(1), 12-18. https://scholar.google.com/scholar
- Hossain, M., Ngo, H. H., Guo, W., & Nguyen, T. (2012). Removal of copper from water by adsorption onto banana peel as bioadsorbent. International Journal of Geomate, 2(2), 227–234. https://www.geomatejournal.com
- Hossain, M. A., Ngo, H. H., Guo, W. S., Nghiem, L. D., Hai, F. I., Vigneswaran, S., & Nguyen, T. V. (2014). Competitive asorption of metals on cabbage waste from multi-metal solutions. Bioresource Technology, 160, 79–88. http://dx.doi.org/10.1016/j.biortech.2013.12.107
- Iqbal, M., & Khera, R. A. (2015). Adsorption of copper and lead in single and binary metal system onto fumaria indica biomass. Chem. Int, 1(3), 157b–163b. https://d1wqtxts1xzle7.cloudfront.net
- Kumar, K., Patavardhan, S. S., Lobo, S., & Gonsalves, R. (2018). Equilibrium study of dried orange peel for its efficiency in removal of cupric ions from water. International Journal of Phytoremediation, 20(6), 593–598. https://doi.org/10.1080/15226514.2017.1405379
- Lasheen, M. R., Ammar, N. S., & Ibrahim, H. S. (2012). Adsorption/desorption of Cd(II), Cu(II) and Pb(II) using chemically modified orange peel: Equilibrium and kinetic studies. Solid State Sciences, 14(2), 202–210. https://doi.org/10.1016/j.solidstatesciences.2011.11.029
- Lugo-Lugo, V., Barrera-Diaz, C., Urena-Nunez, F., Bilyeu, B., & Linares-Hernandez, I. (2012). Biosorption of Cr(III) and Fe(III) in single and binary systems onto pretreated orange peel. Journal of Environmental Management, 112, 120–127. http://dx.doi.org/10.1016/j.jenvman.2012.07.009
- Mahmoud, M. A. (2016). Kinetics studies of uranium sorption by powdered corn cob in batch and fixed bed system. J Adv Res, 7(1), 79–87. http://dx.doi.org/10.1016/j.jare.2015.02.004
- Martín-Lara, M. A., Blázquez, G., Calero, M., Almendros, A. I., & Ronda, A. (2016). Binary biosorption of copper and lead onto pine cone shell in batch reactors and in fixed bed columns. International Journal of Mineral Processing, 148, 72–82. http://dx.doi.org/10.1016/j.minpro.2016.01.017
- Medellin-Castillo, N. A., Padilla-Ortega, E., Regules-Martínez, M. C., Leyva-Ramos, R., Ocampo-Pérez, R., & Carranza-Alvarez, C. (2017). Single and competitive adsorption of Cd(II) and Pb(II) ions from aqueous solutions onto industrial chili seeds (C apsicum annuum) waste. Sustainable Environment Research, 27(2), 61–69. http://dx.doi.org/10.1016/j.serj.2017.01.004
- Mondal, N. K., & Roy, A. (2018). Potentiality of a fruit peel (banana peel) toward abatement of fluoride from synthetic and underground water samples collected from fluoride affected villages of Birbhum district. Applied Water Science, 8(3), 3. https://doi.org/10.1007/s13201-018-0729-3
- Obike, A. I., Igwe, J. C., Emeruwa, C. N., & Uwakwe, K. J. (2018). Equilibrium and kinetic studies of Cu (II), Cd (II), Pb (II) and Fe (II) adsorption from aqueous solution using cocoa (Theobroma cacao) pod husk. Journal of Applied Sciences and Environmental Management, 22(2), 2. https://dx.doi.org/10.4314/jasem.v22i2.5
- Pathak, P. D., Mandavgane, S. A., & Kulkarni, B. D. (2017). Fruit peel waste: Characterization and its potential uses. Curr. Sci, 113(3), 444–454. https://doi.org/10.18520/cs/v113/i03/444-454
- Sadeek, S. A., Negm, N. A., Hefni, H. H., & Wahab, M. M. (2015). Metal adsorption by agricultural biosorbents: Adsorption isotherm, kinetic and biosorbents chemical structures. International Journal Biol Macromol, 81, 400–409. http://dx.doi.org/10.1016/j.ijbiomac.2015.08.031
- Semerjian, L. (2018). Removal of heavy metals (Cu, Pb) from aqueous solutions using pine (Pinus halepensis) sawdust: Equilibrium, kinetic, and thermodynamic studies. Journal of Environmental Technology Innovation, 12, 91–103. https://doi.org/10.1016/j.eti.2018.08.005
- Singh, A., Sharma, R. K., Agrawal, M., & Marshall, F. M. (2010). Health risk assessment of heavy metals via dietary intake of foodstuffs from the wastewater irrigated site of a dry tropical area of India. Food and Chemical Toxicology: An International Journal Published for the British Industrial Biological Research Association, 48(2), 611–619. https://doi.org/10.1016/j.fct.2009.11.041
- Singh, N. B., Garima, N., Sonal, A., & Rachna. (2018). Water purification by using adsorbents: A review. Environmental Technology and Innovation, 11, 187–240. https://doi.org/10.1016/j.eti.2018.05.006
- Soliman, A. M., Elwy, H. M., Thiemann, T., Majedi, Y., Labata, F. T., & Al-Rawashdeh, N. A. F. (2016). Removal of Pb(II) ions from aqueous solutions by sulphuric acid-treated palm tree leaves. Journal of the Taiwan Institute of Chemical Engineers, 58, 264–273. https://doi.org/10.1016/j.jtice.2015.05.035
- Walton, K. S., & Sholl, D. S. J. A. J. (2015). Predicting multicomponent adsorption: 50 years of the ideal adsorbed solution theory. Separations: Materials, devices and processes, 61(9), 2757–2762. https://doi.org/10.1002/aic.14878
- WHO, W. H. O. (2003). Lead in drinking-water: Background document for development of WHO guidelines for drinking-water quality. World Health Organization.
- Zamani Beidokhti, M., Naeeni, S. T., & AbdiGhahroudi, M. S. (2019). Biosorption of nickel (II) from aqueous solutions onto pistachio hull waste as a low-cost biosorbent. Civil Engineering Journal, 5(2), 2. http://dx.doi.org/10.28991/cej-2019-03091259