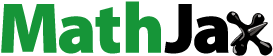
Abstract
This study assessed the aggregate properties that influence track ballast performance along selected Nigerian railway lines. Ballast materials from five selected rail tracks (RT1, RT2, RT3, RT4, and RT5) in Nigeria were collected and assessed in present conditions and after triple-fouling (subjected to degradation, clay, and diesel intrusion). Tests performed include gradation, strength, soundness, and drain-ability tests. Results obtained were compared with the requirements established in five recognized railway ballast standards,where applicable. Initial gradation results indicate that presently, ballast materials from the selected study locations can all be classified as “uniformly graded gravel”. However, upon triple-fouling, a gradation shift towards the “well graded” classification was observed. Based on EN 13,450, the gradations of RT1, RT2, and RT3 ballast materials were more satisfactory than gradations of RT4 and RT5. Strength test results show that ballast materials from tracks RT1, RT2, and RT3 performed satisfactorily based on the five ballast standards considered. RT4 and RT5 on the other hand, only performed suitably based on the AREMA No. 4 ballast standard. The least resistance to weathering was observed in the RT4 and RT5 samples. Initial drain-ability test showed that no ballast sample set achieved the standard drain-ability (3.0 cm/sec). However, RT2 ballast samples indicated the highest drainage capacity with a hydraulic conductivity (k) of 2.285 cm/sec. Furthermore, after triple-fouling, the drainage capacity of all ballast samples largely declined. However, the RT1, RT2, and RT3 samples indicated higher k values compared to RT4 and RT5 ballast samples. Outcomes from the study indicate the need for continuous monitoring of track sub-structure materials along Nigerian railways to ensure its sustainability via improved rail track performance, thereby increasing the safety and efficiency of the Nigerian railway network.
PUBLIC INTEREST STATEMENT
Track performance evaluation is essential in ensuring railway track efficiency. In Nigeria, maintenance of tracks is done with little considerations for the sub-structure components and the potential effects posed on serviceability. Track settlement and drainage problems are functions of the ballast performance. Hence, the need for proactive maintenance measures remains highly essential in Nigeria. The understanding of the present conditions of these materials aids in determining their performance under several fouling conditions. With increasing population comes the need for advancement in the transportation infrastructure. The transportation system of Nigeria can be said to be in a failed state as the roadways cannot conveniently deliver the level of service required to meet the mobility demands of its populace. This research assessed the present condition of ballast from selected Nigerian railways to proffer informed recommendations for improved track performance, thereby promoting the safety and efficiency of the Nigerian railway network.
1. Introduction
Track performance evaluation is essential in the determination of the present condition, necessary remedial action, and latent maintenance requirement, to ensure railway track efficiency. Commonly in practice, maintenance and replacement of rail track components are done without significant considerations for the components of the sub-structure, and the potential effects they pose on the serviceable life of the asset (Bassey et al., Citation2020; Brough et al., Citation2003). Several cost-intensive track rehabilitation works have been conducted in the past in Nigeria without eradicating the primary reason for the problem (Ayoola, Citation2016; Oshin, Citation1991). Ballasted railway tracks constitute the major transportation grids in many countries across the globe; they convey commuters, as well as freight and bulk cargoes between cities, mines, farmlands, and ports (Bassey et al., Citation2020; Indraratna, Citation2016). However, Nigerian railway tracks have suffered decades of neglect due to ineffective financial management, lack of adequate technical expertise, inadequate operational capacity, competition from the road transport mode, and decline in coal and agricultural exports, since the discovery of crude oil (Tolofari & Gubbins, Citation1984). The poor management of railway infrastructure has resulted in worn-out rails, sharp curves, steep grades, track settlement, surface erosion of track sub-structure materials, and poor sub-structure drainage. These challenges have consequently led to reduced train speeds, frequent derailments, non-functionality of rail tracks, the slow turn-around time for wagons, and even railway accidents (Ayoola, Citation2016; Chen, Citation2018). The lack of hands-on proactive and preventive railway track sub-structure maintenance has constituted a significant impediment to the present state of productivity and efficiency of the railway system. The impact of these problems on railway operations is seen in the decline in the number of operational locomotives, and the prolonged disruption of railway services. However, in order to keep pace with the rising mobility demands accompanying population and economic growth while boosting productivity, the rejuvenation of the Nigerian railway system has in recent years, gained priority. Several railway network expansion and rehabilitation projects commenced within the last decade, including the phased completion of the Lagos-Ibadan and Abuja-Kaduna lines, the upgrade of the Lagos-Kano line to standard gauge, the commencement of the Ibadan-Osogbo-Ilorin, and the Itakpe-Warri line, amongst others (Chen, Citation2018).
As weaker particles get crushed, and the sharp (angular) corners of aggregates deform under recurrent loadings, track differential settlement becomes virtually unavoidable (Indraratna, Citation2016; E. T. Selig & Sluz, Citation1978; Selig and Waters, Citation1994). As such, tracks laid on rigid formations like concrete bridge slabs, or rocky terrains, where massive dynamic stresses are experienced, are often subjected to intensified rates of these problems. Consequently, triggering the rapid fragmentation of the sub-structure’s ballast aggregates, and thereby reducing the mechanical and drainage performance of the rail track. Likewise, the ballast may be subjected to fouling via cyclic loadings (Qi, Cui, Chen et al., Citation2020; Wang et al., 2018; Wang et al., Citation2018a), upward movement of clayey fines from the subgrade and the migration of coal trickling down wagon trains (Ebrahimi et al., Citation2012; Giannakos, Citation2010; Huang et al., Citation2009; Indraratna, Navaratnarajah et al., Citation2014b; Tennakoon et al., Citation2015). All of these can adversely impact track drainage capacity. In critical cases, fouled ballast may require complete replacement or proper cleaning to maintain its resilience (stiffness), alignment, and bearing capacity requirements.
Ballasted tracks have minimal support laterally; hence, lateral stability is controlled by increasing the lateral confining stresses (Indraratna, Nimbalkar et al., Citation2014; Indraratna et al., Citation2014b, Citation2014a; Lackenby et al., Citation2007). The behaviour of ballast is influenced by specific general properties of bulk aggregates masses for example, aggregate type, grain size gradation, relative density, and the ratio of voids (Bian et al., Citation2016; Indraratna et al., Citation2016; Qi, Cui, Dupla et al., Citation2020) whereas the characteristics of individual ballast particles like particle size, particle angularity, and particle shape determine its rate of deterioration under wheel loads. The magnitude of axle (wheel) load, the frequency (speed of trains), the number of recurrent loadings, and the impact loads, all also influence aggregate deformation (Qi Deng Sun et al., Citation2016; Indraratna, Nimbalkar & Neville, Citation2014a; Nimbalkar et al., Citation2012; Q.D. Sun et al., Citation2014; E. Selig & Waters, Citation1994). The impact load’s magnitude is dependent on the nature and form of surface irregularities on the rails and wheels, alongside the dynamic response offered by the rail track (Auersch, Citation2006; Correia, Citation2001; Correia & Cunha, Citation2014; Indraratna et al., Citation2014a; Jenkins et al., Citation1974; Li & Davis, Citation2005; Le Pen et al., Citation2014). These impact loads remain highly disadvantageous to all other railway track structure components since designing for deficiencies is not easily incorporated, which is the reason why the assessment of ballast material’s performance of under recurrent locomotive loadings is essential in railroad designs (Bergado et al., Citation2002; Indraratna et al., Citation2009; B Indraratna & Redana, Citation2000; Liu & Xiao, Citation2010; Preteseille et al., Citation2013). Angular crushed stones are examples of durable and hard ballast aggregates with proper shape characteristics that have been confirmed to be critical to the strength and stability of ballast. The in-situ sampling and testing of ballast, alongside degradation via particle size analysis generally offer insights into the composition and the possibilities for the accumulation of unwanted (fouling) materials. However, a more detailed appraisal of the efficiency and serviceability of ballast layers involves assessing the strength and deformation characteristics of ballast (De Bold et al., Citation2021; Sadeghi et al., Citation2018; Wnek et al., Citation2013).
Track settlement and poor sub-structure drainage problems are functions of the ballast, sub-ballast, and subgrade formation performances. As such, the need for proactive maintenance measures in track maintenance remains highly essential in Nigeria. The understanding of the present conditions of these granular materials can help in determining its performance after specified cyclic loadings and under several fouling conditions (Cui et al., Citation2013; Trinh et al., Citation2012). Also, with increasing population comes the need for advancement in the transportation infrastructure of a city. The transportation system of Nigeria can be said to be in a failed state as the presently dominant transportation mode (roadways) cannot conveniently deliver the level of service required to meet the mobility demands of its populace. As such, this research describes the present condition of track ballast along five selected railway tracks in Nigeria, a series of laboratory examinations were conducted to appraise the aggregate properties that influence the strength and durability performance of Nigerian railway track ballast. The experimental program constitutes of ballast gradation, strength, and durability analyses, including tests on ballast drain-ability, degradation, resistance to weathering, and susceptibility to fouling. Outcomes from the study are targeted towards making informed recommendations for improved rail track performance, thereby increasing the safety and efficiency of the Nigerian railway network.
2. Materials and methods
2.1. Materials
Materials used in this study include ballast aggregates, clayey soil, and diesel. The ballast used in this research were sourced locally from the five study areas discussed in Section 2.2.1. The track-crib-sampling method was adopted in the collection of ballast as similarly employed in the studies by Brough et al. (Citation2003), De Paiva et al. (Citation2017), Paiva et al. (Citation2015), and Tutumluer et al. (Citation2017). These studies sampled surface ballast from the cribs along studied rail tracks. In this method, the sampled ballast was considered clean and unfouled. shows a sample set of ballast used in this study.
The clay soil was collected from a clay deposit in Iju, Ota, Ogun State, Nigeria (Lat: 6.679246, Long: 3.137741). The clay soil served as one of the fouling agents in the triple-fouling process. The grain size distribution of the clay used is presented in . Diesel was also used as one of the agents of ballast contamination since the locomotives currently functional on Nigerian railways run on diesel as fuel. presents the index properties of the granular materials used.
Table 1. Index properties of selected ballast and the soil fouling agent
2.2. Methods
All laboratory experiments in this study were conducted at the Highway and Transportation and Geotechnical laboratories of the Department of Civil Engineering, Covenant University, Ota, Nigeria.
2.2.1. Study location and notations
This research focused on assessing track ballast materials collected from five (5) railway tracks in Nigeria; the Abuja light rail metro line connecting the central business district (CBD) to the Idu satellite town of Abuja; the Kaduna to Abuja standard gauge line; the Lagos to Ibadan line, the Ikeja to Agege line, and the Enugu to Makurdi rail line. The selection of rail tracks for this study was based on track age. The first three selected tracks were constructed within the last decade and open for commercial use in the years 2018, 2016, and 2020, respectively. The latter two selected tracks were constructed during the colonial era and have endured decades of poor maintenance (Ayoola, Citation2016). The three recently developed tracks were selected for assessment to validate the quality of ballast materials being utilized for the new phased-development of Nigeria railways. The two older tracks were selected basically for quality comparison. The magnitude of traffic (in Mt/year) along individual tracks in Nigeria have not been recorded in the past by the governing agency. However, gross reports indicate that in 2018, over 328 thousand tons of freight was conveyed via railways. All performance evaluations were based on Laboratory tests, whereas characterization was based on recognized ballast standards. The ballast aggregates assessed are grouped as presented in for ease of identification. The aggregates were sieved where necessary to achieve the specified gradation for each laboratory test. gives a spatial representation of the study locations across Nigeria.
Table 2. Notations representing proposed study areas
2.2.2. Ballast contamination design: the triple-fouling process
The triple-fouling process was conducted to simulate ballast contamination at its criticality. The ballast samples were subjected to three conditions of fouling: degradation from applied loads, clay, and fuel (diesel) intrusions. For all levels of ballast contamination, the diesel content was fixed at 10 percent of the sample’s mass. The clay content, on the other hand, was varied at the following percentages: 10%, 20%, and 30% by mass of the sample. The degradation of ballast was done with the use of an LAA machine at 1000 revolutions. Fines produced from the LAA degradation process were further incorporated into the respective ballast batches for further contamination. illustrates the experimental program adopted in this study.
2.2.3. Test methods
2.2.3.1. Gradation analysis
: The gradation analysis was conducted per ASTM C136/C136M-19. The required sieves with openings suitable for the sampled materials were selected, the sieves were nested in an order of increasing sizes from bottom to top as shown in . For each test batch, a 5 kg sampled ballast was placed on the topmost sieve, and the sieve set was placed on a mechanical sieve shaker for agitation. After the agitation process, the mass of ballast retained on each sieve was recorded.
2.2.3.2. Strength tests
: LAA tests in this study was performed per ASTM C535 (1000 revolutions at 33 rpm) with the equipment shown in . Dry sampled ballast was loaded into the rotating drum with the specified set of steel balls. The shelf (a crossbar inside the drum) interposes the movement of the steel balls, ensuring that they make a crushing course, and do not simply move around the drum with the rotation. The drum was made to rotate for the specified number of revolutions. The quantity of fines produced from the process was determined as a fraction of the initial mass of the ballast samples and was recorded as the LAA value (AS Citation2758.1, 2009; ASTM C535, Citation2016).
Aggregate Impact test was also performed to further assess the capacity of the selected ballast materials to withstand sudden impact loadings. This test was done per IS 2386–4 providing at least two tests per sample. shows the apparatus setup for impact testing. Ballast aggregates finer than 12.5 mm but larger than 10 mm were oven-dried between 100°C and 110°C for about 4 hours. Afterwards, samples were placed and tamped in three layers into the cylindrical mould, and weighed before being placed into the firmly-positioned base cup. The hammer was raised and allowed to drop freely, impacting 15 steady blows on the test sample. The crushed ballast material was then unloaded from the cup and sieved. The fraction finer than the 2.36 mm sieve was weighed and recorded. The aggregate impact value was computed as the weight ratio of fines produced to the initial weight of each test sample and was expressed in percentage.
2.2.3.3. Durability tests
: The soundness test was conducted to assess the weathering resistance capacity of all selected ballast samples. The Nigerian tropical climate subjects these aggregates to severe conditions of heating both daily and seasonally. This test was performed in line with the ASTM C88 procedure which simulates weathering by immersing the ballast samples into a saturated solution of crystalline heptahydrate magnesium sulphate (Epsom salt) (MgSO4∙7H2O) form. The aggregates were made to undergo 5 cycles of 16-hour soaking and 4-hour drying to simulate freezing and thawing actions, respectively. Afterwards, the ballast particles were cleaned, dried, then weighed. The weighted mean percentage loss for each test sample was determined and compared to standard allowable thresholds. shows the sulphate and solution used for the soundness test.
Figure 6. [a] Magnesium sulphate, [b] Solutions prepared for soundness test, and [c] Constant head permeability test set-up used
![Figure 6. [a] Magnesium sulphate, [b] Solutions prepared for soundness test, and [c] Constant head permeability test set-up used](/cms/asset/3632834f-9f77-447f-b883-2ee110cdf5bb/oaen_a_1932240_f0006_oc.jpg)
The constant head permeability test was further performed on all ballast batches under clean and varying levels of triple-fouling with the set-up shown in . The test was conducted as per ASTM D2434:2019. For each test batch, 12,000 cm3 of abraded ballast was prepared and weighted. The ballast was then split into three sub-batches of 4,000 cm3 each. Clay contamination was done in proportions: 10%, 20%, and 30% by weight of the respective sub-batches. Diesel and the fines produced from abrasion were then added in a fixed proportion: 10% by the weight of the ballast sub-batch before clay contamination. The materials were then mixed and allowed to air-dry for 24 hours before drain-ability testing. The sampled ballast was placed up to a height of 300 mm inside the permeameter. An outlet positioned at the top region of the container, above the sample was used to maintain the constant head. Also, an inlet/outlet pipe and valve of 12 mm in diameter was positioned beneath the sample to provide for the ascending flow of water through the ballast samples during testing, and the discharge of water after the test. Additionally, a water tank was used to control the inlet water head.
3. Results and discussions
3.1. Gradation analysis
The gradation of ballast has always been a key indicator for ballast suitability (Bian et al., Citation2016; Qian et al., Citation2017). Successive train loadings accompanied by the effects of weathering and temperature variations may over time affect the initial gradation of track ballast formations (Bassey et al., Citation2020). The results from the initial gradation analysis on the selected ballast samples are presented in . The ballast samples collected fall under the non-fouled category since all collected particles were larger than the 4.75 mm sieve, as a result of the crib sampling method adopted. It is seen that all selected ballast samples are generally uniformly graded. However, RT1, RT2, and RT3 possessed fractions of larger particles (up to 90 mm). RT4 and RT5, on the other hand, possessed smaller-sized particles (65 mm maximum). The initial (current) gradation curves for the ballast samples indicate that presently, ballast materials from the selected study locations can be classified as “uniformly graded”, which is generally suitable based on four ballast standard specifications under consideration (AREMA: Manual for Railway Engineering, Citation2010; EN 13,450: Aggregate for Railway Ballast, 2013; AS Citation2758.1, 2009: Aggregate for Railway Ballast, Citation2009; Indian Railway Standards, Citation2004).
The gradation curves obtained after the triple-fouling exercise which was conducted to determine the ballast performance when subjected to the worst case of degradation by abrasion and infiltration of contaminants, indicated the presence of finer-sized particles as illustrated by the broad-graded gradation curves. The new positions of the curves indicate a particle size gradation shift towards the red regions of unacceptability based on the ERRI and CEN ballast cleaning criteria, respectively (Sussmann et al., Citation2012). However, the RT1, RT2, and RT3 samples indicated better performance (in terms of gradation) even under the triple-fouled condition. The curves for the RT4 and RT5 samples tended more towards the region of unacceptability, indicating their inadequacy under adverse conditions of contamination. Based on the fouling index (FI) established by E. Selig and Waters (Citation1994), FI is derived as the summation of the percentages by mass of the ballast sample finer than sieves No. 4 (4.75 mm) and No. 200 (0.075 mm). further categorizes each ballast sample set at their initial conditions and after the triple-fouling exercise.
Table 3. Categorization of selected ballast materials based on fouling index
All sampled ballast materials can be further classified as “clean” since 100 percent of the material is coarser than 10 mm. The initial FI value of 0 obtained across all ballast samples could be attributed to the sampling method adopted: crib ballast sampling. Crib ballast endures minimal vertical loading while providing drainage alongside longitudinal and lateral stability to the track (Paiva et al., Citation2015). As such, crib ballast suffers less contamination compared to ballast lying beneath the sleepers. After the triple-fouling exercise, RT4 and RT5 ballast samples were adjudged to be “highly contaminated” whereas samples from RT1, RT2, and RT3 were less degraded and as such adjudged “contaminated” based on the E. Selig and Waters (Citation1994) fouling index.
In benchmarking the gradation results obtained for the five ballast sample sets, the material fraction retained on certain specified sieves were further evaluated. The four different standards with established individual mesh retention requirements considered in this study are presented in .
Table 4. Performance of selected ballast under standard mesh retention benchmarks
From , it can be deduced that the European standard (EN 13,450) gives provision for relatively coarser ballast particles compared to the AREMA, AS, and IS standards. Based on EN 13,450, the gradations of RT1, RT2, and RT3 ballast materials were more satisfactory than gradations of RT4 and RT5. On the other hand, based on AREMA No. 4, AS 2758.7, and IRS-GE-1-2004, RT4 and RT5 ballast materials had more satisfactory gradations. Alemu (Citation2011) posited that the reasons for the larger gradation requirements of the European standard is due to variations in climatic conditions across nations in the region. Larger ballast particles offer higher resistance to freezing and thawing cycles (Buddhima Indraratna & Salim, Citation2005). The smaller sizes observed in the RT4 and RT5 ballast materials can be attributed the fact that both tracks have endured longer periods of loading cycles and exposure to weathering and fouling. Tracks RT1, RT2, and RT3 were all constructed within the last decade and as such, are relatively newer rail lines.
3.2. Strength performance
The Los Angeles abrasion and Aggregate impact tests were adopted in assessing the strength performance of the selected ballast aggregates. The results are presented in . The LAA value is an indicator of ballast hardness and maximum expectable damage during track operation and maintenance activities.
shows that RT1, RT3, and RT2 obtained the least abrasion values with 16.48%, 17.81%, and 20.42%, respectively. RT4 and RT5 indicated lower levels of hardness with abrasion values of 31.1% and 30.4%, respectively. As presented in , different recognized railway standards have established thresholds for ballast suitability based on the LAA value.
Table 5. Benchmarks for LA abrasion values across railway standards
shows that ballast materials from tracks RT1, RT2, and RT3 performed satisfactorily based on the five ballast standards considered. RT4 and RT5 on the other hand only performed suitably based on the AREMA No. 4 ballast standard. The satisfactory LAA results obtained for ballast samples from RT1, RT2, and RT3 can also be as a result of the track age, owing to the fact that these granular materials have endured lesser cycles of train loadings compared to those from RT4 and RT5. shows a set of ballast aggregates before and after the LAA test. The particle angularity properties which are key factors in the ballast-ballast inter-contact were observed to be abraded significantly, resulting in rounded particles.
Furthermore, the results from the aggregate impact tests were observed to follow a similar trend as those from the LAA tests. The results are presented in . The aggregate impact value remains one of the indicators of toughness in granular materials.
From , RT4 and RT5 ballast samples clearly offered the least resistance to direct impact loads with AIVs of 16.9% and 17.71%. On the other hand, RT1 and RT3 indicated the most satisfactory AIVs with 9.84% and 10.02%. Out of the five ballast standards considered in this study, only the IRS-GE-1-2004 and the EN 13,450 provide specifications for railway ballast based on the aggregate impact value (AIV). Both standards place their AIV threshold for ballast acceptability at a maximum of 20 %. Based on this criteria, AIV results shown in indicate that the five sampled ballast materials meet the AIV requirements for railway ballast; however, ballast from RT1, RT2, and RT3 performed more superior compared to those from RT4 and RT5 tracks.
Generally, the LAA test is considered to yield more representative results for degradation and fragmentation of granular materials, as such, it is more broadly adopted over the AIV tests (Koohmishi, Citation2019; Wnek et al., Citation2013). To further understand the correlation between the two strength parameters observed, a scatter plot is presented in .
indicates a linear trend of high statistical significance (R = 0.992, R2 = 0.9849) between the AIV and the LAA results.
3.3. Durability performance
3.3.1. Soundness tests
The soundness test was adopted in assessing the susceptibility of the selected ballast materials to damages from freezing and thawing actions. This often depends majorly on climatic conditions, material petrography, as well as the presence of pore spaces on the ballast particles. Unlike the cement/bitumen-bound aggregates used in highway pavements, railway ballast can be highly susceptible to weathering from freeze/thaw cycles because they remain unbound through their design life. The results of the soundness tests have been presented in two categories: the quantitative results and the qualitative results. gives the quantitative data obtained from the Magnesium sulphate-based ballast soundness tests.
Table 6. Ballast soundness test quantitative results
From the weighted percentage loss data presented in , the least extent of degradation due to the cyclic formation of salt crystals was recorded for the RT1, RT3, and RT2 ballast samples with 1.0%, 1.4%, and 2.5% weighted losses, respectively. RT4 and RT5 ballast samples endured the largest extents of degradation from the sulphate attack with 7.7% and 8.3% weighted losses, respectively. Notwithstanding, all ballast samples performed satisfactorily in terms of weighted percentage losses as per ASTM C88. further presents qualitative outcomes observed from the ballast soundness tests. The AREMA, 2010 standard for ballast permits the use of ballast with a maximum percentage weight loss of 5 %, whereas the CN 12–20 C permits a maximum of 7%. Based on these criteria, benchmarks the performance of the five selected set of ballast samples.
Table 7. Percentage weight loss benchmarks from soundness test across standards
Table 8. Ballast soundness test qualitative results
shows that RT4 and RT5 ballast samples performed unsuitably in terms of weighted percentage lost from the sulphate soundness test. RT1, RT2, and RT3 ballast samples on the other hand performed satisfactorily under the two criteria considered herein. As indicated in , the highest extent of crumbling observed was recorded for the RT4 and RT5 samples.
The qualitative data presented in shows that some ballast samples exhibited distresses such as splitting, crumbling, cracking, and flaking. However, the most common distress endured due to the cyclic attack of the sulphate was crumbling. In correspondence with the quantitative results in , RT4 and RT5 samples endured higher extents of distress compared to RT1, RT2, and RT3 samples. It was also noticed that most particles exhibited more than one form of distress as the number of cycles increased, with crumbling being the first observable defect endured, and then, in some cases, succeeded by cracking and splitting. shows some distresses observed in ballast particles during the sulphate soundness tests. Particles endured crumbling, cracking, splitting, and flaking after five cycles of immersion and drying.
3.3.2. Drain-ability tests
The drain-ability of the selected ballast materials was assessed prior to and after the triple-fouling exercise. Drainage remains a core quality of proper ballasted tracks and is essential for sustained track functionality. presents drain-ability results obtained from tests on clean ballast samples before fouling.
Table 9. Initial drain-ability test results on clean ballast samples
The clean ballast drain-ability test results indicate that presently, RT2 ballast samples offer the highest drainage capacity with a hydraulic conductivity (k) of 2.285 cm/sec. RT1 and RT3 ballast samples also showed high drainage capacities with k values of 1.914 and 1.903 cm/sec. The least drainage capacity was observed with the RT4 ballast samples with a k value 1.008 cm/sec. From past studies, the hydraulic conductivity of clean ballast can be up to 3.0 cm/sec (Indraratna et al., Citation2010; Paiva et al., Citation2015). However, the results for the selected ballast materials in their clean state showed that no sampled ballast set achieved such hydraulic conductivity. RT2 obtained the highest hydraulic conductivity across sampled ballast batches with a k value of 2.285 cm/sec, being just 23.82% shy of the standard k value for clean ballast as shown in . This can be explained by the presence of finer-sized aggregates as indicated in the initial gradation curves shown in . This implies that at the present conditions of these tracks, the drainage capacity of RT4’s ballast bed is about 42.58% less efficient than that of RT2.
Figure 13. Performance of selected ballast initial drain-ability in comparison with standard clean ballast
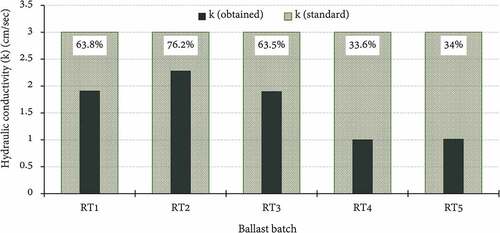
further presents the drain-ability test results after the triple-fouling exercise on all ballast samples.
Table 10. Drain-ability test results on triple-fouled ballast samples
Results in show that the drainage capacity of all ballast samples largely declined upon contamination. Notwithstanding, the RT1, RT2, and RT3 samples still indicated higher k values compared to RT4 and RT5 ballast samples. Furthermore, it indicates that after triple-fouling, the hydraulic conductivity of the selected ballast samples declined drastically by up to 191.4 times the initial hydraulic conductivity without the inclusion of clay. Upon increasing inclusions of clay (10%, 20%, and 30%) the hydraulic conductivity saw further decline up to 382 times that of the initial hydraulic conductivity. Similar to the initial drain-ability results, RT4 and RT5 ballast samples obtained lower k values after the triple fouling exercise compared to RT2, RT1, and RT3 ballast samples. shows the relationship between clay contamination and the hydraulic conductivity of ballast. The drain-ability of these materials reduces significantly with increasing clay content.
As illustrated in , an exponential variation was seen between ballast drain-ability and increasing clay percentages under triple fouling. A relatively similar exponential function was established in a study by Paiva et al. (Citation2015) on Brazilian railway ballast, although fouling by degradation and diesel intrusion was not considered. With an R2 value of 0.6875, the relationship established can be said to be statistically significant. These results demonstrate the challenge of drainage, which can be encountered along railways in Nigeria if contamination is not adequately monitored and controlled.
4. Conclusions
This study aimed to investigate the aggregate properties that influence track ballast performance under normal conditions of loading encountered along selected Nigerian railway lines. Ballast materials from five selected rail tracks across the country were collected and assessed both in their present conditions and after being triple-fouled (subjected to degradation, clay, and diesel intrusion). Laboratory tests performed include: gradation analyses, strength tests, soundness tests and drain-ability tests. Results obtained were compared with the requirements established in five recognized railway ballast standards: (AREMA: Manual for Railway Engineering, Citation2010; BS EN 13450, Citation2013; CN 12–20 C; AS Citation2758.1, 2009;; Indian Railway Standards, Citation2004), where applicable. The following conclusions were drawn:
(i) The initial gradation analyses indicate that presently, ballast materials from the selected study locations can all be classified as “uniformly graded gravel”, which is generally suitable based on the four ballast specifications considered. Gradation analyses after triple-fouling saw a shift in gradation for all ballast samples towards the “well graded” classification. Based on EN 13,450, the gradations of RT1, RT2, and RT3 ballast materials were more satisfactory than gradations of RT4 and RT5. On the other hand, based on AREMA No. 4, AS 2758.7, and IRS-GE-1-2004, RT4 and RT5 ballast materials had satisfactory gradations.
(ii) LAA results show that ballast materials from tracks RT1, RT2, and RT3 performed satisfactorily based on the five ballast standards considered. RT4 and RT5, on the other hand, only performed suitably based on the AREMA No. 4 ballast standard. Furthermore, AIV results indicate that the five sampled ballast materials meet the AIV requirements for railway ballast; however, ballast from RT1, RT2, and RT3 tracks performed more superior compared to those from RT4 and RT5 tracks.
(iii) RT4 and RT5 ballast samples performed unsuitably in terms of weighted percentage lost from the sulphate soundness test. RT1, RT2, and RT3 ballast samples on the other hand, performed satisfactorily under the two criteria considered herein. The highest extent of particle crumbling observed was recorded for the RT4 and RT5 samples.
(iv) Initial drain-ability test indicates that no ballast sample set achieved the standard drain-ability (3.0 cm/sec). RT2 ballast samples indicated the highest drainage capacity with a hydraulic conductivity (k) of 2.285 cm/sec. RT1 and RT3 ballast samples also showed high drainage capacities with k values of 1.914 and 1.903 cm/sec. The least drainage capacity was observed with the RT4 ballast samples with a k value 1.008 cm/sec. Furthermore, after triple-fouling, the drainage capacity of all ballast samples largely declined. However, the RT1, RT2, and RT3 samples still indicated higher k values compared to RT4 and RT5 ballast samples. The results further showed that the drain-ability of these materials reduces significantly with increasing clay fouling extents.
Ballast from tracks RT4 and RT5 performed poorly compared to those from tracks RT1, RT2, and RT3, based on all assessments performed in this study. Hence the need for preservation of the aged rail tracks within the country is of utmost urgency. Similarly, with the poor transportation asset maintenance culture predominant in Nigeria, it is clear that the satisfactory performances of RT1, RT2, and RT3 ballast materials result solely because they are relatively new tracks. As such, the provision of adequate proactive maintenance practices is essential to sustain the serviceability of these assets for future generations.
Disclosure of potential conflicts of interest
The authors hereby declare that there are no relevant financial or non-financial competing interests to report.
Acknowledgements
The authors hereby acknowledge the Management of Covenant University for the enabling environment provided for this study and the open access publication support.
Additional information
Funding
Notes on contributors
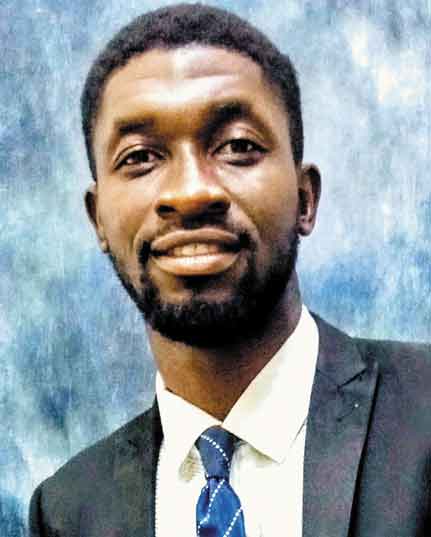
Daniel E. Bassey
Daniel E. Bassey is a Graduate Student in the Department of Civil Engineering, Covenant University, Ota, Nigeria. His research focuses on sustainability in relation to Transportation Infrastructure and Materials.
David O. Olukanni is a Professor in Covenant University’s Civil Engineering Department and the Pioneer Director of Covenant University Central Instrumentation Research Facility (CUCIRF). He has published several scholarly works in reputed journals on Infrastructural and Environmental Sustainability.
Ben U. Ngene is a Associate Professor in the Department of Civil Engineering, Michael Okpara University of Agriculture, Umudike, Nigeria, His research interest is in the area of Resilient Infrastructure and Resource Management
Gideon O. Bamigboye is a Senior Lecturer in Covenant University’s Civil Engineering Department. His research focuses on environmental and infrastructural Sustainability.
References
- Aggregate for Railway Ballast. (2009). Technical Note 75 1. Cement Concrete & Aggregate Australia.
- Alemu, A. Y. (2011). Survey of railway ballast selection and aspects of modelling techniques [Royal Institute of Technology]. (Dissertation). Retrieved from http://urn.kb.se/resolve?urn=urn:nbn:se:kth:diva-87466
- AREMA: Manual for Railway Engineering. (2010). Vol. 1: Track, Ch. 1: Roadway and Ballast, American Railway Engineering and Maintenance of Way Association (AREMA).
- AS 2758.1. (2009). The requirements of Concrete aggregates, Technical Note 73 1. Cement Concrete & Aggregate Australia.
- ASTM C136/C136M-19. (2019). Standard test method for sieve analysis of fine and coarse aggregates. ASTM International, West Conshohocken, PA, https://doi.org/10.1520/C0136_C0136M-19
- ASTM C535. (2016). Standard test method for resistance to degradation of large-size coarse aggregate by abrasion and impact in the los angeles machine. ASTM StandardsASTM International, West Conshohocken, PA, https://www.astm.org/Standards/C535.htm
- ASTM D2434. (2019). Standard test method for permeability of granular soils (constant head). ASTM International, West Conshohocken, PA, https://www.astm.org/Standards/D2434
- Auersch, L. (2006). Dynamic axle loads on tracks with and without ballast mats: Numerical results of three-dimensional vehicle-track-soil models. Proceedings of the Institution of Mechanical Engineers, Part F: Journal of Rail and Rapid Transit, 220(2), 169–21. https://doi.org/10.1243/09544097F00105
- Ayoola, T. A. (2016). Establishment of the Nigerian Railway Corporation. Journal of Retracing Africa, 3(1), 21–42. https://encompass.eku.edu/jora/vol3/iss1/4
- Bassey, D., Ngene, B., Akinwumi, I., Akpan, V., & Bamigboye, G. (2020). Ballast contamination mechanisms: A criterial review of characterisation and performance indicators. Infrastructures, 5(11), 94. https://doi.org/10.3390/infrastructures5110094
- Bergado, D. T., Balasubramaniam, A. S., Fannin, R. J., & Holtz, R. D. (2002). Prefabricated vertical drains (PVDs) in soft Bangkok clay: A case study of the new Bangkok International Airport project. Canadian Geotechnical Journal, 39(2), 304–315. https://doi.org/10.1139/t01-100
- Bian, X., Huang, H., Tutumluer, E., & Gao, Y. (2016). “Critical particle size” and ballast gradation studied by Discrete Element Modeling. Transportation Geotechnics, 6, 38–44. https://doi.org/10.1016/j.trgeo.2016.01.002
- Brough, M., Stirling, A., Ghataora, G., & Madelin, K. (2003). Evaluation of railway trackbed and formation: A case study. NDT and E International, 36(3SPEC), 145–156. https://doi.org/10.1016/S0963-8695(02)00053-1
- BS EN 13450. (2013). Aggregate for railway ballast. British Standards Institute.
- Chen, Y. (2018). China’s role in Nigerian Railway development and implications for security and development. In United States Institute of Peace: Special Report (Issue 423). United States Institute of Peace. www.usip.org
- Correia, G. A. (2001). Soils mechanics in routine and advanced pavement and rail track rational design. In Geotechnics for roads, rail tracks and earth structures (pp. 165–187). A. A. Balkema, Lisse. http://pascal-francis.inist.fr/vibad/index.php?action=getRecordDetail&idt=14573341
- Correia, G. A., & Cunha, J. (2014). Analysis of nonlinear soil modelling in the subgrade and rail track responses under HST. Transportation Geotechnics, 1(4), 147–156. https://doi.org/10.1016/j.trgeo.2014.07.003
- Cui, Y. J., Duong, T. V., Tang, A. M., Dupla, J. C., Calon, N., & Robinet, A. (2013). Investigation of the hydro-mechanical behaviour of fouled ballast. Journal of Zhejiang University: Science A, 14(4), 244–255. https://doi.org/10.1631/jzus.A1200337
- De Bold, R., Connolly, D. P., Patience, S., Lim, M., & Forde, M. C. (2021). Using impulse response testing to examine ballast fouling of a railway trackbed. Construction and Building Materials, 274, 121888. https://doi.org/10.1016/j.conbuildmat.2020.121888
- De Paiva, C. E. L., Pereira, M. L., & Pimentel, L. L. (2017). Study of railway ballast fouling by abrasion-originated particles. Railway Engineering, 2004. https://doi.org/10.25084/raileng.2017.0074
- Ebrahimi, A., Tinjum, J. M., & Edil, T. B. (2012). Protocol for testing fouled railway ballast in large-scale cyclic triaxial equipment. Geotechnical Testing Journal, 35(5), 5. https://doi.org/10.1520/GTJ103846
- Giannakos, K. (2010). Loads on Track, Ballast Fouling, and Life Cycle under Dynamic Loading in Railways. Journal of Transportation Engineering, 136(12), 1075–1084. https://doi.org/10.1061/(ASCE)TE.1943-5436.0000182
- Huang, H., Tutumluer, E., & Dombrow, W. (2009). Laboratory characterization of fouled railroad ballast behavior. Transportation Research Record, 2117(1), 93–101. https://doi.org/10.3141/2117-12
- Indian Railway Standards. (2004). IRS-GE-1: specifications for track ballast (Issue 1). https://ecr.indianrailways.gov.in/uploads/files/1366518435624-GE-IRS-1.pdf
- Indraratna, B., & Redana, I. W. (2000). Numerical modeling of vertical drains with smear and well resistance installed in soft clay. Canadian Geotechnical Journal, 37(1), 132–145. https://doi.org/10.1139/t99-115
- Indraratna, B. (2016). 1st Proctor Lecture of ISSMGE : Railroad performance with special reference to ballast and substructure characteristics. Transportation Geotechnics, 7, 74–114. https://doi.org/10.1016/j.trgeo.2016.05.002
- Indraratna, B., Attya, A., & Rujikiatkamjorn, C. (2009). Experimental Investigation on Effectiveness of a Vertical Drain under Cyclic Loads. Journal of Geotechnical and Geoenvironmental Engineering, 135(6), 835–839. https://doi.org/10.1061/(ASCE)GT.1943-5606.0000006
- Indraratna, B., Navaratnarajah, S., Nimbalkar, S., & Rujikiatkamjorn, C. (2014). Use of shock mats for enhanced stability of railroad track foundation. Australian Geomechanics Journal, 49(4), 101–110. https://ro.uow.edu.au/eispapers/3424
- Indraratna, B., Nimbalkar, S., Coop, M., & Sloan, S. W. (2014). A constitutive model for coal-fouled ballast capturing the effects of particle degradation. Computers and Geotechnics, 61, 96–107. https://doi.org/10.1016/j.compgeo.2014.05.003
- Indraratna, B., Nimbalkar, S., & Neville, T. (2014). Performance assessment of reinforced ballasted rail track. Proceedings of the Institution of Civil Engineers - Ground Improvement, 167(1), 24–34. https://doi.org/10.1680/grim.13.00018
- Indraratna, B., Nimbalkar, S., & Rujikiatkamjorn, C. (2014a). Enhancement of rail track performance through utilisation of geosynthetic inclusions. Geotechnical Engineering, 45(1), 17–27. https://ro.uow.edu.au/eispapers/2145
- Indraratna, B., Nimbalkar, S., & Rujikiatkamjorn, C. (2014b). From theory to practice in track geomechanics - Australian perspective for synthetic inclusions. Transportation Geotechnics, 1(4), 171–187. https://doi.org/10.1016/j.trgeo.2014.07.004
- Indraratna, B., Nimbalkar, S. S., Navaratnarajah, S. K., Rujikiatkamjorn, C., & Neville, T. (2014). Use of shock mats for mitigating degradation of railroad ballast. Sri Lankan Geotechnical Journal -special Issue on Ground Improvement, 6(1), 32–41. https://ro.uow.edu.au/eispapers/4943
- Indraratna, B., Nimbalkar, S. S., & Tennakoon, N. (2010). The behaviour of ballasted track foundations: Track drainage and geosynthetic reinforcement. GeoFlorida, (2010, 2378–2387. https://doi.org/10.1061/41095(365)241
- Indraratna, B., & Salim, W. (2005). Mechanics of ballasted rail tracks: A geotechnical perspective. Taylor & Francis.
- Indraratna, B., Sun, Y., & Nimbalkar, S. (2016). Laboratory assessment of the role of particle size distribution on the deformation and degradation of ballast under cyclic loading. Journal of Geotechnical and Geoenvironmental Engineering, 142(7), 04016016. https://doi.org/10.1061/(ASCE)GT.1943-5606.0001463
- Jenkins, H. H., Stephenson, J. E., Clayton, G. A., Morland, G. W., & Lyon, D. (1974). The effect of track and vehicle parameters on wheel/rail vertical dynamic forces. Railway Engineering Journal, 3(1), 2-16. https://www.scinapse.io/papers/133468534
- Koohmishi, M. (2019). Drainage potential of degraded railway ballast considering initial gradation and intrusion of external fine materials. Soils and Foundations, 59(6), 2265–2278. https://doi.org/10.1016/j.sandf.2019.12.011
- Lackenby, J., Indraratna, B., McDowell, G., & Christie, D. (2007). Effect of confining pressure on ballast degradation and deformation under cyclic triaxial loading. Géotechnique, 57(6), 527–536. https://doi.org/10.1680/geot.2007.57.6.527
- Le Pen, L., Watson, G., Powrie, W., Yeo, G., Weston, P., & Roberts, C. (2014). The behaviour of railway level crossings: Insights through field monitoring. Transportation Geotechnics, 1(4), 201–213. https://doi.org/10.1016/j.trgeo.2014.05.002
- Li, D., & Davis, D. (2005). Transition of Railroad Bridge Approaches. Journal of Geotechnical and Geoenvironmental Engineering, 131(11), 1392–1398. https://doi.org/10.1061/(ASCE)1090-0241(2005)131:11(1392)
- Liu, J., & Xiao, J. (2010). Experimental study on the stability of railroad silt subgrade with increasing train speed. Journal of Geotechnical and Geoenvironmental Engineering, 136(6), 833–841. https://doi.org/10.1061/(ASCE)GT.1943-5606.0000282
- Nimbalkar, S., Indraratna, B., Dash, S. K., & Christie, D. (2012). Improved performance of railway ballast under impact loads using shock mats. Journal of Geotechnical and Geoenvironmental Engineering, 138(3), 281–294. https://doi.org/10.1061/(ASCE)GT.1943-5606.0000598
- Oshin, O. (1991). Road transport and the declining fortunes of the Nigerian Railway, 1901-1950. Journal of Transport History, 12(1), 11–36. https://doi.org/10.1177/002252669101200102
- Paiva, C., Ferreira, M., & Ferreira, A. (2015). Ballast drainage in Brazilian railway infrastructures. Construction and Building Materials, 92, 58–63. https://doi.org/10.1016/j.conbuildmat.2014.06.006
- Preteseille, M., Lenoir, T., & Hornych, P. (2013). Sustainable upgrading of fine-grained soils present in the right-of-way of High Speed Rail projects. Construction and Building Materials, 44, 48–53. https://doi.org/10.1016/j.conbuildmat.2013.03.022
- Qi, S., Cui, Y.-J., Chen, R.-P., Wang, H.-L., Lamas-Lopez, F., Aimedieu, P., Dupla, J.-C., Canou, J., & Saussine, G. (2020). Influence of grain size distribution of inclusions on the mechanical behaviours of track-bed materials. Géotechnique, 70(3), 238–247. https://doi.org/10.1680/jgeot.18.P.047
- Qi, S., Cui, Y. J., Dupla, J. C., Chen, R. P., Wang, H. L., Su, Y., Lamas-Lopez, F., & Canou, J. (2020). Investigation of the parallel gradation method based on the response of track-bed materials under cyclic loadings. Transportation Geotechnics, 24, 100360. https://doi.org/10.1016/j.trgeo.2020.100360
- Qian, Y., Boler, H., Moaveni, M., Tutumluer, E., Hashash, Y. M. A., & Ghaboussi, J. (2017). Degradation-related changes in ballast gradation and aggregate particle morphology. Journal of Geotechnical and Geoenvironmental Engineering, 143(8), 04017032. https://doi.org/10.1061/(ASCE)GT.1943-5606.0001706
- Sadeghi, J., Motieyan-Najar, M. E., Zakeri, J. A., Yousefi, B., & Mollazadeh, M. (2018). Improvement of railway ballast maintenance approach, incorporating ballast geometry and fouling conditions. Journal of Applied Geophysics, 151, 263–273. https://doi.org/10.1016/j.jappgeo.2018.02.020
- Selig, E., & Waters, J. (1994). Track geotechnology and substructure management. Thomas Telford Services Ltd. https://books.google.com/books?hl=en&id=ZHxW4D6XT0MC&oi=fnd&pg=PA10&ots=jo1yGVtOiM&sig=xXTCBk5BPdD_chXKeobCpuS0yNQ
- Selig, E. T., & Sluz, A. (1978). Ballast and subgrade response to train loads. Transportation Research Record, 694, 53-60. https://trid.trb.org/view/141400
- Sun, Q. D., Indraratna, B., & Nimbalkar, S. (2014). Effect of cyclic loading frequency on the permanent deformation and degradation of railway ballast. Géotechnique, 64(9), 746–751. https://doi.org/10.1680/geot.14.T.015
- Sun, Q. D., Indraratna, B., & Nimbalkar, S. (2016). Deformation and Degradation mechanisms of railway ballast under high frequency cyclic loading. Journal of Geotechnical and Geoenvironmental Engineering, 142(1), 04015056. https://doi.org/10.1061/(ASCE)GT.1943-5606.0001375
- Sussmann, T. R., Ruel, M., & Chrismer, S. M. (2012). Source of ballast fouling and influence considerations for condition assessment Criteria. Transportation Research Record: Journal of the Transportation Research Board, 2289(1), 87–94. https://doi.org/10.3141/2289-12
- Tennakoon, N., Indraratna, B., Nimbalkar, S., & Sloan, S. W. (2015). Application of bounding surface plasticity concept for clay-fouled ballast under drained loading. Computers and Geotechnics, 70, 96–105. https://doi.org/10.1016/j.compgeo.2015.07.010
- Tolofari, S. R., & Gubbins, E. J. (1984). Nigerian railways: Problems and prospects. Transportation Planning and Technology, 8(4), 225–235. https://doi.org/10.1080/03081068408717255
- Trinh, V. N., Tang, A. M., Cui, Y. J., Dupla, J. C., Canou, J., Calon, N., Lambert, L., Robinet, A., & Schoen, O. (2012). Mechanical characterisation of the fouled ballast in ancient railway track substructure by large-scale triaxial tests. Soils and Foundations, 52(3), 511–523. https://doi.org/10.1016/j.sandf.2012.05.009
- Tutumluer, E., Ahuja, N., Hart, J. M., Moaveni, M., Huang, H., Zhao, Z., & Shah, S. (2017). Field evaluation of ballast fouling conditions using machine vision. Safety IDEA Program Project Final Report, 27. IDEA Program Transportation Research Board The National Academies
- Wang, H. L., Cui, Y. J., Lamas-Lopez, F., Calon, N., Saussine, G., Dupla, J. C., Canou, J., Aimedieu, P., & Chen, R. P. (2018). Investigation on the mechanical behavior of track-bed materials at various contents of coarse grains. Construction and Building Materials, 164, 228–237. https://doi.org/10.1016/j.conbuildmat.2017.12.209
- Wang, H.-L., Cui, Y.-J., Lamas-Lopez, F., Dupla, J.-C., Canou, J., Calon, N., Saussine, G., Aimedieu, P., & Chen, R.-P. (2018a). Permanent deformation of track-bed materials at various inclusion contents under large number of loading cycles. Journal of Geotechnical and Geoenvironmental Engineering, 144(8), 04018044. https://doi.org/10.1061/(ASCE)GT.1943-5606.0001911
- Wnek, M., Tutumluer, E., Moaveni, M., & Gehringer, E. (2013). Investigation of aggregate properties influencing railroad ballast performance. Transportation Research Record, 2374(1), 180–189. https://doi.org/10.3141/2374-21