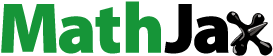
ABSTRACT
Corrosion resistance of untreated S40900, S43036, S44400 and S31635 stainless steels (S409, S430, S444 and S316) in 0.05 M H2SO4/3.5% NaCl solution was investigated and compared to their heat-treated counterparts after cyclic temperature variation between 1000°C and 37°C by potentiodynamic polarization, potentiostatic analysis, open circuit potential measurement and optical microscopy. Results showed untreated S409 exhibited the weakest resistance to corrosion at 8.406 mm/y while untreated S316 displayed the highest resistance at 1.581 mm/y. Cyclic heat treatment caused significant increase in corrosion rate of untreated S409, S430 and S444 by 21.92%, 38.46% and 94.76%. Corrosion rate of untreated S316 decreased by 69% to 0.490 mm/y. Untreated and heat-treated S316 exhibited the least metastable pitting tendency while heat-treated S409 and S430 exhibited the highest. Untreated S444 showed the highest tendency to passivate compared to heat-treated S409 and S430 with the lowest tendency. Heat treatment generally improved passivation rate, but not passivation resilience. Untreated S444 and heat-treated S430 exhibited the widest passivation range. Heat treatment increased the tendency for pit formation on the steels. Plots from open circuit potential measurement showed untreated S444 exhibited the lowest thermodynamic tendency to corrode with potential culminating at −0.201 V while among the heat-treated steels, S316 displayed the lowest corrosion tendency peaking at −0.288 V. Corrosion pits on heat-treated S409 were significantly larger compared to the untreated steel. Superficial corrosion pits visible on untreated S430 disappeared after heat treatment. Heat treatment decreased the occurrence of corrosion pits on S316 while miniature corrosion pits were present on heat-treated S444.
KEYWORDS:
PUBLIC INTEREST STATEMENT
The economic impact and problems resulting from corrosion have drawn strong attention from scientists and engineers worldwide. Corrosion in industrial environments is a major concern in chemical processing plants, oil and gas industry, manufacturing, automobile industry, marine operations, boiler plants and power generation plants due to the considerable cost involved in the replacement of metallic parts in their various applications. The consequence often leads to plant shutdowns, breakdown of industrial equipment, reduced efficiency, industrial downtime, high maintenance cost due to replacement of damaged part, wastage of valuable resources and expensive overdesign. Appropriate selection of engineering with desirable corrosion resistant properties significantly reduce corrosion damage thus prolonging the lifespan of the material and subsequently reducing cost of corrosion prevention.
1. Introduction
Automotive exhaust systems consist of piping connections that control the movement of heated reactive gases from the ignition chamber of a vehicular engine to the exhaust manifold and through the exhaust silencer to the pipe outlet (Ikpe & Abdulsamad, Citation2017). Failure due to corrosion of the exhaust system causes rupture; resulting in leakages within the internal components, noise issues due to acoustic changes and environmental pollution. The high temperature conditions of exhaust systems coupled with the presence of dissolved corrosive anions necessitate cautious material choice in the production of exhaust system components for optimum and long lasting performance. The exhaust muffler is a component of an automobile exhaust system whose major functionality is to reduce the rumble passed out by the exhaust gases of an internal ignition chamber. It has an exterior shell, inner plates, inner pipes, end plates and other components. The temperature of exhaust mufflers is around 300°C to 500°C, but it can also handle higher temperatures up to 1200°C. Stainless steels are utilized in the production of automobile exhaust silencers due to their resilience to corrosion, high temperature stability and exquisite quality. Corrosion is the major factor responsible for the limited lifespan of automobile silencers and other parts of the automotive exhaust system (Hoffmann & Gümpel, Citation2009). Corrosion reactions control the chemical characteristics of the steel and greatly alter their physical and mechanical attributes.
Functional and structural damage of exhaust mufflers leads to loss of operating performance. Deterioration occurs due to corrosive precipitates within the silencer, de-icing agents utilized on icy roads, material sensitization at temperatures up to 600ºC, static loading due to low cycle and high cycle fatigue, corrosivity of coastal environments and the aggravating effect of acid deposition (Piepho, Citation1991). Automobile exhaust systems can be classified into two zones, i.e. the hot end and the cold end. At the cold end, condensations of released combustion gases (CO2, H2O, O2, CO, HC, NOx and SO2, etc.) continually accumulate, producing sulphuric acid and low levels of HCl acid. The condensates, coupled with the presence of Cl− anion, produce a wet corrosive environment within the muffler leading to localized corrosion deterioration such as pitting (Heck & Farrauto, Citation2001; Nazir et al., Citation2016; Nockert et al., Citation2012). Resistance of stainless steel grades to pitting corrosion is heavily dependent on their alloy composition (Engstrom et al., Citation1999). Oxidation and spalling of the surface oxide layer dominate the corrosion reaction mechanism at the hot end of the exhaust system. As a result, stainless steel properties required for application therein must have high-temperature strength, thermal fatigue behaviour, vulnerability to oxidation and resistance to chloride corrosion. Thermodynamic and kinetic factors control the spontaneity and frequency of exhaust muffler corrosion in the form of general and localized deterioration of the surface (Shi et al., Citation2013).
Low carbon steel was previously the most widely used steel for exhaust systems. However, it suffered from weak corrosion resistance resulting in very short life span. Aluminised low carbon steel is capable of withstanding pitting corrosion but is unsuitable for high-temperature applications, while high-performance stainless steels are more reliable but are expensive (Heck & Farrauto, Citation2001). 409 ferritic stainless steel with low coefficient of thermal expansion is one of the most commonly used steels for exhaust mufflers (Han et al., Citation2014). However, it is susceptible to intergranular corrosion, pitting corrosion and grain coarsening at welding temperatures. Ferritic stainless steel grades represent about 26% of the total steel production worldwide while austenitic stainless steels represent 60% thus providing a large pool for selection of stainless steels for application in corrosive and high-temperature conditions (Charles et al., Citation2009; Totten, Citation2006). While some grades of stainless steel are able to withstand the degradation effect of corrosive anions, their corrosion when subjected to high-temperature conditions is not specific as there are circumstances where the steel’s resilience to corrosion increases, declines or is steady (Loto, Citation2019a; Loto, Citation2019b; Loto et al., Citation2016, Citation2018). Past investigation on the resilience of the inert passive film of S40900 stainless steel dilute NaCl and chloride/sulphate electrolyte shows the inert oxide depicts an n-type semi conductive behavior. The concentration of chloride and sulphate species strongly influences corrosion resistance (Loto, Citation2019a; De Souza et al., Citation2017). Study of the influence of Mo on S43036 shows Mo increases the pitting resistance of the steel (Loto, Citation2017). The pitting corrosion resistance of alloy S44400 steel was evaluated in chloride and sulphate environment with results showing the steel is more resilient to localized corrosion in chloride solution (Zatkalíková et al., Citation2010). Results from research on S31635 steel showed that the alloy performed better than the 304 L counterpart in the presence of chlorides (AlHazaa et al., Citation2015). Surface finishing negatively influences the pitting resistance of S31635 steel in FeCl3 electrolyte in the temperature range 30–80°C (Mahvash et al.,). In line with appropriate material selection of stainless steels for application in corrosive environments, this investigation targets the influence of cyclic high temperature fluctuations on the resilience of S43036 ferritic, S44400 ferritic and S31635 austenitic stainless steels to corrosion in sulphate contaminated seawater and comparing it to the resistance of the conventional S40900 ferritic stainless steels.
2. Materials and methods
S40900 ferritic stainless steel (S409) was retrieved from an automobile exhaust silencer. S43036 ferritic stainless steel (S430), S31635 austenitic stainless steel (S316) and S44400 ferritic stainless steel (S444) were obtained from Vienna University of Technology, Austria. The elemental constituents of the steels are shown in . They were determined with PhenomWorld scanning electron microscope equipped with energy dispersive spectrometer at the Materials Characterization Laboratory in Department of Mechanical Engineering, Covenant University, Ota, Ogun State, Nigeria. The steel specimens (one test piece each for each steel) were cut to required average dimensions (1 cm2) and encased in hardened acrylic paste. The exposed part of the steels uncovered by the acrylic paste were subjected to repetitive grinding and smoothening with coarse emery papers before burnishing with 6 µm liquid diamond. They were subsequently washed with deionized water and acetone, and preserved within a desiccator for potentiodynamic polarization according to (ASTM G1-03, Citation2011). 800 mL of 0.05 M H2SO4 solution plus 3.5% NaCl concentration were concocted from standard quality reagent of the acid and salt in deionized H2O. A second group of prepared steel specimens were heat treated three times in a muffle furnace up to 1000°C, maintained for 30 mins, chilled to ambient temperature before encasing in harden acrylic paste. The furnace temperature was sustained with a control switch (± 10°C accuracy) connected to K-Type thermocouple. Electrochemical assessment of S409, S430, S316 and S444 stainless steels were conducted with Digi-Ivy potentiostat. The equipment apparatus consists of three electrode configuration (encased S409, S430, S316 or S444), Ag/AgCl reference electrode and platinum counter electrode. The electrodes were inundated in 200 mL of the electrolyte inside a lucid container and attached to the potentiostat/computer system through the electrodes. Electrochemical plots were produced at scanning speed of 0.0015 V/s between −1.5 V to +1.5 V according to ASTM G59–97Citation2014). Corrosion current density, Icorr (A/cm2), and corrosion potential (V) were estimated from the polarization plots (Holland, Citation1991). Corrosion rate CR (mm/y) was computed from the formula below according to (ASTM G102–89 Citation2015e1);
Table 1. Elemental constituents (%) of S409, S430, S444 and S316 stainless steel
EQ represents equivalent weight (g) of each steel, 0.00327 represents corrosion rate constant and D represents steel density (g/cm3) (Kahyarian et al., Citation2017). Polarization resistance, Rp (Ω), was determined from Equationequation (2)(2)
(2) where Ba is the anodic Tafel slope (V/dec) and Bc is the cathodic Tafel slope (V/dec).
I represent corrosion current. Open circuit potential analysis was done at step potential of 0.1 V/s for 5400s in the acid/chloride electrolyte with Digi-Ivy potentiostat. Optical representations of the untreated and heat treated steel surfaces were obtained prior to and after corrosion tests with Omax metallurgical microscope for comparison.
3. Result and discussion
3.1. Potentiodynamic polarization studies
Potentiodynamic polarization plots of untreated and heat treated S409, S430, S316 and S444 in 0.05 M H2SO4/3.5% NaCl solution are laid out in ) and (b). depicts the data gotten from the polarization plots of the steels. S409 shows the greatest corrosion rate value of 8.406 mm/y among the untreated steels which corresponds to Icorr of 7.82 x 104A/cm2 and polarization resistance of 32.8 Ω in the presence of Cl− and SO42- anions. Inspection of the polarization plots in ) indicates the plots for S409 had the highest cathodic-anodic Tafel slopes, signifying the degree of H2 formation, O2 reduction and surface deterioration mechanisms are much higher and corroborates the corrosion rate result obtained (Gupta et al., Citation2013). S316 exhibits the lowest corrosion rate value (1.581 mm/y) while the corrosion rates of S430 and S444 are comparable at 3.504 mm/y and 3.130 mm/y. The degree of cathodic reaction mechanisms for S316 is quite similar to S409 despite the very low anodic slope which indicates the selective adsorption of H2 might lead to H2 embrittlement. S444 and S430 were less vulnerable to H2 embrittlement from inspection of its decreased cathodic Tafel slope. However, the high anodic Tafel slope of S430 shows it is vulnerable to surface oxidation, hence it corrosion rate value. The cathodic Tafel slopes data for the untreated steel show the cathodic process of the corrosion reaction mechanism is under activation control, playing limited role in the overall general corrosion of the steels. The anodic reaction dominates the corrosion reaction mechanism as shown from the anodic Tafel slope values.
Table 2. Potentiodynamic polarization data for untreated and heat treated S409, S430, S316 and S444 corrosion in 0.05 M H2SO4/3.5% NaCl solution
Figure 1. Potentiodynamic polarization plots of S409, S430, S316 and S444 corrosion in 0.05 M H2SO4/3.5% NaCl solution (a) untreated steels and (b) heat-treated steels
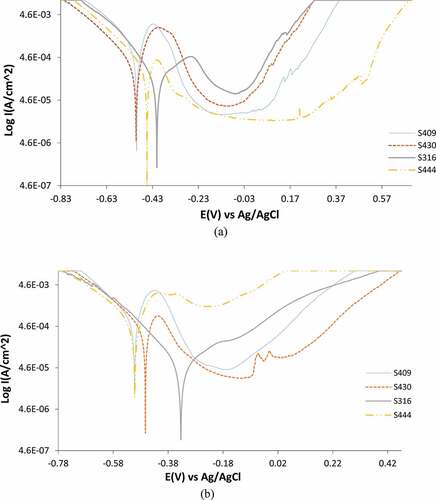
Cyclic temperature variation substantially impacts the corrosion susceptibility of the tested alloys (Seikh, Citation2013; Sheu et al., Citation2018). Corrosion rate of S409, S430 and S444 significantly increased while S409 with its broad utilization in the production of automobile exhaust mufflers remained the most vulnerable with heat treatment weakening its resilience to corrosion at 10.766 mm/y. Under this condition, the polarization resistance of the steel has decreased further to 25.6 Ω at Icorr of 1.00 x 10−3A/cm2. The corrosion rate of S430 and S444 increased from 3.504 mm/y and 3.130 mm/y to 5.694 mm/y and 6.096 mm/y while their polarization resistance decreased to 48.5 Ω and 44.7 Ω. The change in corrosion potential value between the untreated and heat treated S430 (from −0.502 V to −0.463 V) coupled with the increase in corrosion rate show heat treatment generally degrades the steel due to increase in oxidation reaction mechanisms. This assertion is confirmed from the anodic Tafel slope value at 1.050 V/dec. Variation of corrosion potential between the untreated and heat treated S409 and S444 from −0.499 V and −0.454 V to cathodic values of −0.502 V, coupled with increase in corrosion rates shows heat treatment in addition to general surface deterioration, increases the likelihood of localized corrosion reactions due to the electrochemical action of Cl− and SO42- species. The corrosion resistance of S316 increased significantly after cyclic heat treatment from 1.581 mm/y to 0.490 mm/y. The steel’s corrosion potential transited from −0.411 V to −0.323 V, signifying decreased vulnerability to localized corrosion in addition to general surface deterioration. The corrosion potential plots in ) show the cathodic reaction process is under activation control at a greater degree than the untreated steels. However, variation in their corrosion resistance after heat treatment is strongly influenced by the anodic reaction processes as confirmed on the anodic plot in ). This is further corroborated from the anodic Tafel slope values in where S316 with the lowest corrosion rate had the highest anodic Tafel slope value of 11.070 V/dec due to its greater anodic exchange Icorr.
3.2. Pitting corrosion and passivation studies
Corrosion pits initiate due to the adsorption of Cl− ions on the surface of the protective oxide on the steel surfaces (Baryshnikova et al., Citation2009; McCafferty, Citation2003; Song et al., Citation2017). Previous research on Cl− anionic adsorption on metallic surfaces signifies that the metal-chloride bond on the surface is polar and involves charge transfer (Samin & Taylor, Citation2018). Theories that discussed the evolution of pitting corrosion have been proposed (Schmuki, Citation2002). Pit initiation occurred at regions of discontinuity, flaws, inclusions and impurities where passivity has been broken. These initiation points are anodic to the adjacent regions. Pitting corrosion in stainless steels occurs in two consequent steps: (a) nucleation which involves the disintegration of the passive film resulting in oxidation and dissolution of the steel and (b) pit propagating due to acidification and chloride concentration of the solution within the pit holes (Burstein et al., Citation2004; Kobayashi et al., Citation2000). Potentiostatic data depicting the pitting potential, metastable pitting potential, passivation potential, passivation range, potential range to metastable pitting and potential range to passivation for the untreated and heat-treated steels are shown in . The values for these parameters are relative to the corrosion potential. Metastable pitting potential depicts the potential at which metastable pitting activity initiates following anodic polarization (Pistorius & Burstein, Citation1992). This phenomenon occurs with the formation of transient corrosion pits on the steel surface which nucleates and grows during potential scanning before collapsing at the passivation potential. Though transient in nature, it depicts the capacity of the steel to withstand evolution of corrosion pits and resistance to their growth. The metastable pitting values for the untreated steel ranged between −0.25 V and −0.43 V while the corresponding values for the heat-treated steels ranged between 0 V and −0.42 V. Observation of the potential range to passivation for untreated S316 shows extensive anodic polarization and acidification of the steel surface prior to the onset of metastable pits. The highest potential range to metastable pitting for the untreated steels occurred on S316 at 0.16 V while the heat-treated counterpart for S316 is 0.33 V. This phenomenon confirms the comparatively low corrosion or high corrosion resistance of S316 compared to other steels. S409 exhibited the shortest range for the untreated steels which agrees with its corrosion rate results while S430 exhibited the shortest range to metastable pits for the heat-treated steels. The potential range to passivation depicts the capacity of the steels to passivate following anodic polarization in response to displacement of metallic cations. Untreated S444 exhibited the shortest range to passivation at 0.18 V due to peculiarities of its metallurgical structure compared to other steels indicating the ability of the steel to quickly adsorb dissolved O2 and form an impenetrable protective oxide. Untreated S409 and S430 exhibited the widest potential range to passivation at 0.31 V respectively. Heat treatment generally improved the capacity of the steels to passivate as shown in the generally relative decrease in potential to passivate values. However, the corresponding passivation range values shows such observation do not translate to strong resilient passive film. The passivation range values show the resilience of the passive film to anionic attack and its ability to sustain its resilience over wide potential range during potentiostatic scanning. Untreated S444 exhibited the widest passivation range value at 0.57 V followed by S409 at 0.27 V. However, after heat-treatment S444 and S316 exhibited the lowest passivation range values at 0.09 V and 0.06 V while S409 and S430 exhibited the passivation range values at 0.13 V and 0.25 V. This signifies heat-treatment has detrimental effect of the localized corrosion resistance of the steels with the exception of S430. Comparison of the pitting potential values for the untreated and heat-treated stainless steel alloys shows heat-treatment exposes the alloys to the initiation and growth of stable pits. Observation of the pitting potential variation shows the extent to which resistance to pitting corrosion of the steels declined. S444 exhibited the greatest decrease in resistance to localized corrosion followed by S409 while S316 exhibited the lowest decrease in resistance.
Table 3. Potentiostatic data for pitting corrosion and passivation behaviour for S409, S430, S316 and S444 stainless steel alloys
3.3. Open circuit potential measurement
The open circuit potential plots for untreated and heat treated S409, S430, S316 and S444 for 5400s of exposure time are shown in ) and ). Untreated S409 and S430 exhibited the highest thermodynamic tendency to corrode in ) while in ), heat-treated S409 and S444 exhibited the highest thermodynamic tendency to corrode (Revie and Uhlig, Citation2008). Untreated S409 and S430 exhibited similar open circuit potential behaviour without applied potential in sulphate-chloride media. The OCP plot of S430 initiated at −0.493 V (0 s) and sharply declined to −0.520 V at 13.9s. At this point its OCP plot aligns with the OCP plot for S409 which initiated at −0.520 V after which they both progressed to higher (positive) potentials and culminated at −0.490 V and −0.488 V (5400s). The gradual increase in potential occurs from the evolution of inert oxide layer responsible for corrosion protection (Bacelis et al., Citation2021; Choudhary et al., Citation2016). However, the relatively lower potential plots of S409 and S430 compared to S316 and S444 shows the extent of protection against the electrochemical action of SO42- and Cl− anions is significantly lower. Untreated S444 exhibited the lowest thermodynamic impulse to corrode from observation of ). Its OCP plot initiated at −0.340 V and progressed sharply to −0.321 V at 100s before gradually progressing to −0.201 V at 5400s. The OCP plot of untreated S444 shows it is the most resistant to corrosion at rest potentials with resilient protective oxide film. S316 also displayed sufficient corrosion resistance compared to S409 and S430 with potential initiating at −0.434 V (0 s) and peaked at −0.391 V (5400s).
Figure 2. OCP plot for S409, S430, S316 and S444 in 0.05 M H2SO4/3.5% NaCl solution (a) untreated steels and (b) heat-treated steels
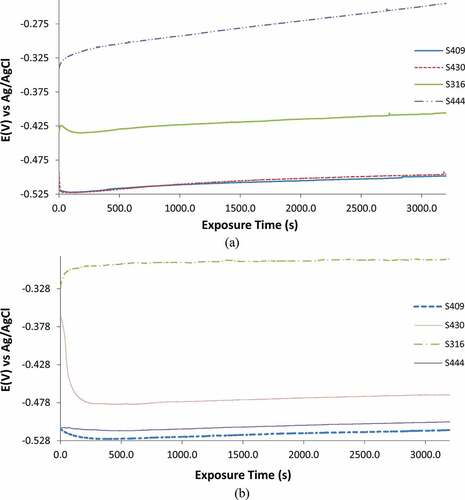
The OCP plots of ) substantially counter the plots in ) due to the effect of repetitive heat treatment replicating the conditions of automobile mufflers. Very little change occurred on the OCP plot of heat-treated S409 which initiated at −0.513 V (compared to −0.520 V for untreated S409) and sharply declined −0.526 V at 300s before progressively increasing to −0.509 V at 5400s. The change represents increased vulnerability to corrosion after heat treatment. The performance of heat treated S444 was quite poor compared to its untreated counterpart. The OCP plot of heat treated S444 showed significant decrease in potential from −0.340 V (untreated S444) to −0.512 V at 0 s due to microstructural changes of the steels constituents resulting in increased vulnerability to corrosive anions. At 5400s the OCP value of the untreated and heat treated S444 are −0.201 V and −0.497 V.
While S444 demonstrates sufficient corrosion resistance for application in corrosive environments, its application in very high temperature conditions must be considered. The OCP plot of heat treated S430 showed slight improvement in corrosion resistance. However, there are concerns on its thermodynamic stability as the steel initiating at −0.365 V (0 s) (compared to −0.493 V for untreated S430) sharply decreased significantly to lower potentials, peaking at −0.477 V (211.70s) and later progressed to −0.462 V at 5400s. The final potential of −0.462 V for heat treated S430 is slightly higher than the value of −0.488 V obtained for the untreated steel. Heat treated S316 performed better than the other steels from observation of its OCP plot compared to the untreated steel. The plots of untreated S316 demonstrated sufficient corrosion resistance; however, the heat treated steel performed better with corrosion potential initiating at −0.328 V (compared to −0.434 V for untreated S316), progressed sharply to −0.306 V at 50.10s before peaking at −0.288 V (5400s). The untreated steel peaked at −0.391 V. However, the plots of heat treated S316 displayed minimal thermodynamic instability.
3.4. Optical microscopy characterization
Optical images of S409, S430, S316 and S444 before and after corrosion are shown from ) to 5(c). ) to (d) exhibits the optical images of the steels prior to the corrosion test. ) to (d) depicts the optical images of the untreated steels after corrosion test while ) to (d) depicts the optical images of the heat treated steels after corrosion test. Shallow corrosion pits with different size variation are observable on the exterior of untreated S409 [)]. However, the size, dimension and depth of the corrosion pits have significantly increased on the morphology of its heat treated counterpart [)] indicating greater vulnerability of the steel to corrosion after heat treatment as proven from the decrease in the passivated region of its polarization plots [) and (b)]. The corrosion pits on the morphology of untreated S430 [)] are quite few and superficial with limited localized deterioration of the steel surface which indicates higher corrosion resistance than S409. Upon heat treatment of S430 [)], corrosion pits are visibly absent due to modification of the steel’s microstructure resulting in enhanced corrosion resistance. This is responsible for the increased passivated region on the polarization plot of heat treated S430 [)]. The morphology of S316 and S444 exhibited very few corrosion pits, though the pits on S316 are slightly larger. However, heat treatment decreased the occurrence of corrosion pits on S316 while miniature corrosion pits were present on S444 at mag. x100 [].
4. Conclusion
The effect of repetitive temperature fluctuations on the resilience of S43036, S31635 and S44400 to corrosion in comparison to the resilience of conventional S40900 used for automobile exhaust system was studied in chloride-sulphate solution. Data outputs showed the corrosion rate of as-received S43036, S31635 and S44400 were substantially lower than the corrosion rate value obtained for as-received S40900 steel. Heat treatment significantly decreased the corrosion resistance of S40900, S43036 and S44400 with conventional S40900 having the highest corrosion rate value. The corrosion rate of heat treated S31635 significantly decreased. Before heat treatment, as-received S44400 displayed the highest pitting resistance while as-received S40900 depict the most substantive metastable pitting behaviour. Heat treatment substantially decreased the pitting corrosion resistance of S40900, S31635 and S44400 while heat treated S43036 depict substantial enhancement in pitting corrosion resistance. Heat-treatment decreases the metastable pitting resistance, accelerates the rate of passive film formation, decreases passivation range. Heat treated S31635 displayed the lowest tendency to corrode from OCP measurement. As-received S40900 displayed the largest corrosion pits which further increased upon heat treatment. However, occurrence of corrosion pits for the other steels were significantly lower and in some cases absent after heat treatment.
Acknowledgements
The authors appreciate Covenant University for the provision of facilities for the research.
Disclosure statement
Authors declare no conflict of interest.
Additional information
Funding
Notes on contributors

Roland Tolulope Loto
Prof. Roland Tolulope Loto is a lecturer and researcher at Covenant University. He is proven scholar in the field of metallic corrosion prevention and control. He has over one hundred and eighty (180) research publications. He has consistently served as reviewer in respectable journals due to his intensive knowledge and technical expertise. Roland has been undertaking a number of renowned engineering research in collaboration with research/educational institutions. His in-depth experience in research experimentation basically aimed at proffering solutions to the current depreciating effect of metallic degradation and failure in service in various engineering and industrial applications. He has a Doctor of Technology (DTech) in Metallurgical and Materials Engineering (2014) from Tshwane University of Technology, Pretoria, South Africa, Master’s Degree (MSc) in Metallurgical and Materials Engineering (2007) from University of Lagos, Lagos, Nigeria, and a Bachelor of Technology in Mechanical Engineering (2002) from Ladoke Akintola University of Technology, Oyo State, Nigeria.
References
- AlHazaa, A. N., El-Sayed, M. S., & Hany, S. A. (2015). Galvanic corrosion in 3.5 wt% NaCl solutions of magnesium alloy AZ31 coupled with Ni after different bonding periods of time. International Journal of Electrochemical Science, 10, 5420–14. electrochemsci.org/papers/vol10/100705420.pdf
- ASTM G102–89. (2015). e1 standard practice for calculation of corrosion rates and related information from electrochemical measurements. Accessed 30 May 2020. http://www.astm.org/Standards/G31.
- ASTM G1–03. (2011). Standard practice for preparing, cleaning, and evaluating corrosion test specimens. Accessed 4 April 2020. http://www.astm.org/Standards/G1.
- ASTM G59–97. (2014). Standard test method for conducting potentiodynamic polarization resistance measurements. Accessed 30 May 2020. http://www.astm.org/Standards/G31.
- Bacelis, A., Veleva, L., Sebastián Feliu, S., Jr., Cabrini, M., & Lorenz, S. (2021). Corrosion activity of carbon steel B450C and low chromium ferritic stainless steel 430 in cement extract solution. Buildings, 11, 220. https://doi.org/10.3390/buildings11060220
- Baryshnikova, E. A., Batrakov, V. V., & Egorov, V. V. (2009). Adsorption of Cl− ions on γ-Fe2O3 oxide from nitrate solutions. Russian Journal of Electrochemistry, 45(7), 765–771. https://doi.org/10.1134/S102319350907009X
- Burstein, G. T., Liu, C., Souto, R. M., & Vines, S. P. (2004). Origins of pitting corrosion. Corrosion Engineering, Science and Technology, 39(1), 25–33. https://doi.org/10.1179/147842204225016859
- Charles, J., Mithieux, J.-D., Santacreu, P.-O., & Peguet, L. (2009). The ferritic stainless family: the appropriate answer to nickel volatility?. Revue De Métallurgie, 106(3), 124–139. https://doi.org/10.1051/metal/2009024
- Choudhary, S., Garg, A., & Mondal, K. (2016). Relation between open circuit potential and polarization resistance with rust and corrosion monitoring of mild steel. Journal of Materials Engineering and Performance, 25(7), 2969–2976. https://doi.org/10.1007/s11665-016-2112-6
- De Souza, J. S., De Oliveira, L. A., Sayeg, I. J., & Antunes, R. A. (2017). Electrochemical study of the AISI 409 ferritic stainless steel: passive film stability and pitting nucleation and growth. Materials Research, 20(6). https://doi.org/10.1016/j.rinp.2018.12.031
- Engstrom, P., Amberntsson, A., Skoglundh, M., Fridell, E., & Smedler, G. (1999). Sulphur dioxide interaction with NOx storage catalysts. Applied Catalysis. B, Environmental, 22(4), L241–L248. https://doi.org/10.1016/S0926-3373(99)00060-0
- Gupta, R. K., Raman, S., Koch, C. C., & Murty, B. S., 2013. Effect of Nanocrystalline Structure on the Corrosion of a Fe20Cr Alloy. International Journal of Electrochemical Science, 8, 6791–6806.
- Han, P. H., Xu, Z. H., Wang, C. P., Li, M. C., & Bi, H. Y. (2014). Condensate corrosion behavior of type 409 stainless steel in simulated automotive muffler environments. International Journal of Electrochemical Science, 9, 3784–3794. electrochemsci.org/papers/vol9/90703784.pdf
- Heck, R. M., & Farrauto, R. J. (2001). Automobile exhaust catalysts. Applied Catalysis. A, General, 221(1–2), 443–457. https://doi.org/10.1016/S0926-860X(01)00818-3
- Hoffmann, C., & Gümpel, P. (2009). Pitting corrosion in the wet section of the automotive exhaust systems. Journal of Achievements in Materials Manufacturing and Engineering, 34(2), 115–121. jamme.acmsse.h2.pl/papers_vol34_2/3421.pdf
- Holland, R. I. (1991). Use of potentiodynamic polarization technique for corrosion testing of dental alloys. European Journal of Oral Science, 99(1), 75–85. https://doi.org/10.1111/j.1600-0722.1991.tb01026.x
- Ikpe, A. E., & Abdulsamad, G. (2017). Engineering material selection for automotive exhaust systems using CES software. International Journal of Engineering, Science and Technology, 3(2), 500–560. http://doi.org/10.19072/IJET.282847
- Kahyarian, A., Brown, B., & Nesic, S. (2017). Electrochemistry of CO2 corrosion of mild steel: effect of CO2 on iron dissolution reaction. Corrosion Science, 129, 146–151. https://doi.org/10.1016/j.corsci.2017.10.005
- Kobayashi, Y., Virtanen, S., & Böhni, H. (2000). Microelectrochemical studies on the influence of Cr and Mo on nucleation events of pitting corrosion. Journal of Electrochemical Society, 147, 155. https://doi.org/10.1149/1.1393169
- Loto, R. T. (2017). Study of the corrosion resistance of type 304L and 316 austenitic stainless steels in acid chloride solution. Oriental Journal of Chemistry, 33(3), 1090–1096. https://doi.org/10.13005/ojc/330304
- Loto, R. T. (2019a). Effect of SO42− and Cl− anionic attack on the localized corrosion resistance and morphology of 409 ferritic stainless steel. Results in Physics, 12, 738–742. https://doi.org/10.1016/j.rinp.2018.12.031
- Loto, R. T. (2019b). Electrochemical data on the effect of extreme temperatures on 2101 duplex steel corrosion performance in chloride-sulphate environment. Oriental Journal of Chemistry, 35(2), 531–537. https://doi.org/10.13005/ojc/350204
- Loto, R. T., Aiguwurhuo, O., & Evana, U. (2016). Corrosion resistance study of heat treated 420 martensitic stainless steel and 316 austenitic stainless steel in dilute acid concentrations. Revista Técnica De La Facultad De Ingeniería Universidad Del Zulia, 39(7), 35–40. http://doi.org/10.21311/001.39.7.04
- Loto, R. T., Loto, C. A., & Ohijeagbon, I. (2018). Effect of heat treatment processes on the localized corrosion resistance of austenitic stainless steel type 301 in chloride/sulphate solution. Results in Physics, 11, 570–576. https://doi.org/10.1016/j.rinp.2018.09.056
- McCafferty, E. (2003). Sequence of steps in the pitting of aluminum by chloride ions. Corrosion Science, 45(7), 1421–1438. https://doi.org/10.1016/S0010-938X(02)00231-7
- Nazir, M. H., Khan, Z. A., Saeed, A., & Stokes, K. (2016). A predictive model for life assessment of automotive exhaust mufflers subject to internal corrosion failure due to exhaust gas condensation. Engineering Failure Analysis, 63, 43–60. https://doi.org/10.1016/j.engfailanal.2016.02.014
- Nockert, J., Nyborg, L., & Norell, M. (2012). Corrosion of stainless steels in simulated diesel exhaust environment with urea. Materials and Corrosion, 63(5), 388–395. https://doi.org/10.1002/maco.201005783
- Piepho, L. L. (1991). Advancements in automotive corrosion resistance. NACE conference, Cincinnati, Ohio.
- Pistorius, P. C., & Burstein, G. T. (1992). Metastable pitting corrosion of stainless steel and the transition to stability. Philosophical transactions of the royal society of London. Series A: Physical and Engineering Sciences, 341, 531–559. http://doi.org/10.1098/rsta.1992.0114
- Revie, R. W., & Uhlig, H. H., 2008. Chapter 3 Thermodynamics: Corrosion Tendency and Electrode Potentials. New Jersey: John Wiley and Sons. http://doi.org/10.1002/9780470277270.ch3
- Samin, A. J., & Taylor, C. D. (2018). A first principles and kinetic monte carlo study of the thermodynamics and kinetics of chlorine adsorption on Zr(0001) surface. Colloids and Surfaces. A, Physicochemical and Engineering Aspects, 539, 92–100. https://doi.org/10.1016/j.colsurfa.2017.11.075
- Schmuki, P. (2002). From bacon to barriers: A review on the passivity of metals and alloys. J. Solid State Electrochemistry, 6(3), 145–164. https://doi.org/10.1007/s100080100219
- Seikh, A. H. (2013). Influence of heat treatment on the corrosion of microalloyed steel in sodium chloride solution. Journal of Chemistry, 587514. https://doi.org/10.1155/2013/587514
- Sheu, -H.-H., Hong, T.-Y., Lin, -T.-T., & Ge, M.-D. (2018). The effect of heat treatment on the corrosion resistance, mechanical properties and wear resistance of Cr−C coatings and Cr−C/Al2O3 composite coatings electrodeposited on low carbon steel. International Journal of Electrochemical Science, 13, 9399. https://doi.org/10.20964/2018.10.39
- Shi, X., Li, Y., Jungwirth, S., Fang, Y., Seeley, N., & Jackson, E. (2013). Identification and laboratory assessment of best practices to protect dot equipment from the corrosive effect of chemical deicers (pp. 17).
- song, Y., Jiang, G., Chen, Y., Peng Zhao, P., & Tian, Y. (2017). Effects of chloride ions on corrosion of ductile iron and carbon steel in soil environments. Scientific Reports, 7, 6865. https://doi.org/10.1038/s41598-017-07245-1
- Totten, G. E. (2006). Steel heat treatment handbook (2nd ed). Taylor and Francis Group.
- Zatkalíková, V., Bukovina, M., Škorík, V., & Petreková, L. (2010). Pitting corrosion of AISI 316Ti stainless steel with polished surface. Materials Engineering, 17(2), 15–19.