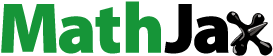
Abstract
Biosorption is an extensive technology applied for the removal of heavy metal ions and other pollutants from aqueous solutions. In the present study, the sorption of metal ions from the polluted huge drain (El Tapia drain) in Abu Qir Bay was determined using nano-magnetic iron oxide and nano-silver oxide, low cost and eco-friendly nano-sorbents, which shows a high potential for metal (Cd and Ni) uptake due to the small size of the nano-sorbents, which is favorable for the diffusion of metal ions from the solution onto the active sites of the adsorbent surface. Therefore, Fe3O4 and silver oxide Ag2O nanosorbents can be effective and economical adsorbents for the rapid removal and recovery of metal ions from aqueous media. In this study, we will synthesize magnetic active and silver oxide adsorbents and marine biomass-coated magnetic and silver composites and evaluate their effectiveness for the removal of toxic metals from one hot spot on the Alexandria coast.
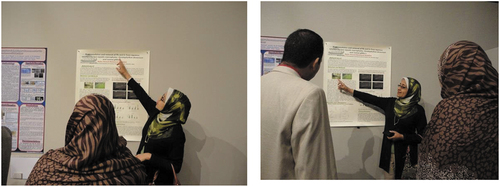
PUBLIC INTEREST STATEMENT
Biosorption is an extensive technology applied for the removal of heavy metal ions and other pollutants from aqueous solutions. This study focused on treating wastewater to be used directly or indirectly through reducing pollution in the end receptor, especially in Egypt in light of the present water crisis. Therefore, Fe3O4 and silver oxide Ag2O nanosorbents can be effective and economical adsorbents for the rapid removal and recovery of metal ions from aqueous media. In this study, we will synthesize magnetic active and silver oxide adsorbents and marine biomass as the aquatic macrophytes (Phragmites australis), which are natural materials that can be used as low-cost, safe, and effective adsorbents-coated magnetic and silver composites, and evaluate their effectiveness for the removal of Cd and Ni ions from polluted drain water; one hot spot on the Alexandria coast from one hot spot on the Alexandria coast.
1. Introduction
Indiscriminate disposal of heavy metals into water resources is considered the major reason for aquatic pollution worldwide. This problem caused international concern for the past few decades due to the non-degradable and harmful end products of all heavy metals. Heavy metal contamination is a great concern because of its toxic effect on plants, animals, and human beings, and its tendency for bioaccumulation even at relatively low concentrations (Abdallah, Citation2012). Heavy metals, such as Cd and Ni are common pollutants in the environment. The major sources of metal pollution are the effluents of industrial processing due to its wide manipulations and implementations in water pipes, storage batteries, food beverages, insecticides, and medicinal preparation for flavoring and sweetening (Abdallah, Citation2014; Abdallah & Badr El Din, Citation2020).
The toxicity of heavy metal compounds or species is mainly related to their effects on the nervous system and/or gastrointestinal track. It is also well known that heavy metals poisoning may cause mental retardation, reduction in hemoglobin formation, as well as damage to kidney and liver. Therefore, removing these pollutants from natural water has become an urgent necessity, especially after the issue of global water scarcity and in Egypt, in particular. Over the past decade, the concern has been about the effective and low-cost adsorbent materials derived from agricultural wastes that can be used for the effective removal and recovery of heavy metal ions from wastewater streams.
Intense research attention is now focused on cost-effective, ecofriendly, and easily available adsorbent particularly of biological origin natural materials that are available in large quantities, or certain waste products from industrial or agricultural operations, may have potential as inexpensive sorbents. However, the performance of a bio-sorbent depends on the characteristic properties of the biomass as well as the microenvironment of the target toxicant. The search for appropriate and inexpensive biomass is a continuing process.
In the present study, the biosorption of metal ions from polluted surface water in drain discharging in Abu Qir Bay on the Egyptian coast on the Mediterranean Sea was determined using nano-magnetic iron oxide and nano-silver oxide, low cost, and eco-friendly nano-sorbents, which shows a high potential for metal uptake due to the small size of both Fe3O4 and Ag2O nano-sorbents, which is favorable for the diffusion of metal ions from the solution onto the active sites of the adsorbent surface. In recent studies, nano-magnetic iron oxide and silver oxide show high potential for metal uptake due to the small size, which is favorable for the diffusion of metal ions from the solution onto the active sites of the adsorbent surface. These sorbents were treated chemically followed by surface loading of (macrophytes) biomass for increasing their biosorption capacity and the highest metal uptake values. Compared with centrifugation and filtration methods, the magnetic separation method is considered to be a rapid and effective technique for separating nanoparticles from aqueous solutions. Therefore, Fe3O4 and Ag2O nano-sorbent can be effective and economical adsorbents for the rapid removal and recovery of metal ions from aqueous media (Abdallah et al., Citation2017).
In this study, we synthesized magnetic active adsorbent and a biomass coated magnetic composite and evaluated their effectiveness for the removal of toxic metals from the water of some polluted drains. Accordingly, the aim of this work is to produce two low cost and eco-friendly sorbents (Fe3O4 nanosorbent and nano-silver oxide Ag2O nanosorbent) and test them in the removal of the same metals Cd and Ni from the huge drain containing mainly industrial wastes (El Tapia Drain in Abu Qir Bay, ) to know its efficiency in removal. In order to solve one of the serious and considered problems of some drains act as hot spots contributed to pollution along the Alexandria coast.
2. Materials and methods
2.1. Study area
Abu Qir Bay receives about 2 × 106 m3 /day of a mixture of untreated sewage and industrial wastes discharged from El Tapia drain and dumped into the Bay through the “Tabia Pump Station”; these industrial wastes include metals, fertilizers industry, textiles manufacturing, chemicals, dyes, food processing, and canning wastes, as well as paper mill effluents (about 101,850 m3/ day). In addition, about 2 × 106 m3 d−1 of agricultural drainage waters were discharged into the Bay through the opening of Lake Edku (brackish water) directly to the Bay and finally at the far east side of Abu Qir Bay. The industrial wastewaters for the present study were collected from this drain ().
2.2. Synthesis of Fe3O4 andAgO2 nano-sorbents -immobilized macrophytes sorbents
Amounts of the biomass (Phragmites australis) were collected from nearby wetlands around Alexandria City, washed with potable water to eliminate solid particles, and then air-dried at room temperature. Finally, the washed air—dried macrophytes were—dried in the oven at 65 °C during 24 h, crushed, and sieved through a 0.5-mm sieve. The crushed materials were then stored at room temperature in an airtight pack and used for further metal removal experiments.
The synthesis of low cost, eco-friendly, and highly efficient sorbent in the removal of toxic metals as nano-magnetic iron oxide (Fe3O4 nano-sorbents) and silver oxide nano-sorbent on the surface of biomass were according to Mahmoud et al. (Citation2015) and Solomon et al. (Citation2007). A normal weight of (Fe3O4 and Ag2O) sorbent was added to the biomass and the reaction mixture was mixed well and dried in an oven.
2.3. Synthesis of spiked metals solutions
All chemicals used in this study were supplied by Sigma–Aldrich, India. A spiking standard of Cd and Ni were prepared by dissolving an accurately weighed quantity in double distilled water to prepare the stock solutions (500 mg L−1) of the two metals separately and employed in the preparation of working standards. All working samples of wastewater were spiked with both studied metals stock solutions that were separately prepared. One strip of drain wastewater was analyzed without additional metals. Serial spiking dilutions were made by diluting two metals with industrial wastewater. After the adsorption of the two metals, the water sample was analyzed for dissolved metal characterization (American Public Health Association (APHA), Citation2005) with an inductively coupled plasma/ mass spectrometry (ICP/MS—Agilent 7500, USA). For analysis, calibration solutions were analyzed followed by an initial calibration blank to control carry-over. The percent recovery of the initial calibration verification standard should be 90–110% for each element being determined.
2.4. Biosorption studies
The metal capacity of nano-biomass sorbents was tested through effective parameter, such as an effect of pH, effect of contact time, effect of mass dose of sorbents on the process of removing toxic metals and effect of metal concentration on the process of removing toxic metals.
The removal experiments were processed parameters, such as the metal’s initial concentration was estimated by contacting 0.1 g of each nano-sorbent separately with 100 mL of metal’s solutions of different initial concentrations ranging from 5 to 30 mg/L for Cd and Ni and agitation speed of 250 rpm were maintained. The effect of the initial pH on the adsorption of the two metals adsorbed was estimated by containing 100 mL of spiked wastewater solution of optimum initial concentrations for both Cd and Ni at different pH from 2.0 to 10.0 by using diluted solutions of HCl and NaOH.
The effect of the amount of nano-sorbent used on the equilibrium uptake was estimated by agitating the metals spiked wastewater solution of optimum initial concentrations and pH, with the weighed amounts of nano-biosorbent ranging from 0.05 to 0.35 g. The effect of time on the adsorption of the two metals was obtained by agitating 0.1 g of the nano-sorbent with the same volume of spiked wastewater solution (100 ml) at different times from 30 to 180 min with temperature maintained at 25°C agitation speed of 250 rpm. Samples pipetted out at different time intervals were filtered through a 0.45-mm-pore-size cellulose acetate membrane filter and then analyzed for the concentration of the metals. The concentrations of Cd and Ni were measured with an inductively coupled plasma/ mass spectrometry (ICP/MS—Agilent 7500, USA). The amount of metal adsorbed onto the nano-sorbent at equilibrium was calculated from the mass balance of EquationEq. (1)(1)
(1) as given below
where C0 and Ce are the initial and equilibrium concentrations of metals (mg/L), respectively, qe is equilibrium metals concentration on nano-sorbent (mg/g), V is the volume of the metals solution (L), and W is the mass of the nano-sorbent used (in grams).
Surface functionality of sorbent is characterized by its responsibility for all activity and capability for all adsorption properties and processes. In the present study, the sorbent was imaged by the use of scanning electron microscope (JSM-5300, JEOL Ltd.). An ion sputtering coating device (JEOL-JFC-1100E) was used to coat the SEM specimens with gold to increase the conductivity.
3. Results and discussion
3.1. Surface characterization of the prepared nano-sorbent
Scanning electron microscopy (SEM) is considered as an efficient technique for the investigation and evaluation of the surface morphology of the sorbents. represents the SEM image of the biosorbent before treatment by nano-magnetic and nano-silver particles and after treatment. The surface morphology of the natural plant (Phragmites australis) as biosorbent has retained its original shape () and after treated by the two nanoparticles its distribution leading to noticeable changes with a lot of active sites as shown in . In other evidence, the treatment of the chemically modified biosorbent with magnetic and silver nanoparticles has resulted in pronounced surface changes of this sorbent as represented by . Furthermore, the mean pore diameter of nano-magnetic and nanosilver biosorbents were 7.081and 96.52 nm, respectively, which is larger compared with the new biosorbent of active carbon treated by immobilized by baker’s yeast (4.7455 nm) recorded by Abdallah et al. (Citation2017), which owns a much richer porous structure.
Figure 2. Scanning electron microscope of (a) raw biosorbent, (b, C) after treatment by nano-magnetic and nano silver particles, (D and E) after adsorption by Cd on nano-magnetic and nano silver particles and (F and G) after adsorption by Ni on nano-magnetic and nano silver particles.
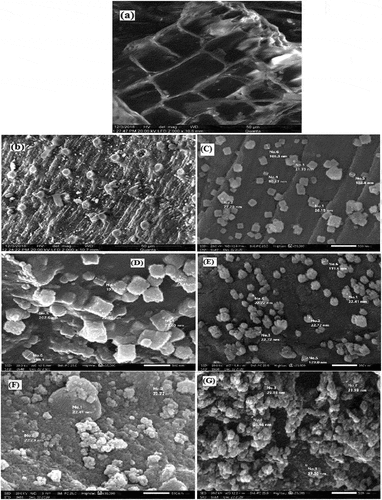
As seen in , after adsorption of Cd and Ni on the two biosorbents a smoother configuration, regular surface, and expanded pores were formed, after the uptake of metals onto the nano biosorbents. The expansion of the pores can be because of the uptake of Cd and Ni onto the sorbent surface that filled these pores.
3.2. Effect of initial concentrations of Cd and Ni
The initial metals concentration provides an important driving force to overcome all mass transfer limitations of metals between the aqueous and solid phases. Thus, higher initial metals concentrations will enhance the biosorption process. The equilibrium uptake capacity (qe) for the two modified nano-sorbents increased with an increase in Cd concentration. Except for Ni, the equilibrium uptake capacity increased to the maximum value at concentration 20 and 25 mg/L, respectively (). Thus, the effect of initial metals concentration plays a significant role in determining the maximum uptake capacity of the nano-sorbents for studied metals. This may indicate the ability of nano-biosorbent (nano-magnetic iron oxide and silver oxide) in absorbing high concentrations of Cd and Ni from contaminated waters. The present result reveals that the two modified nano-sorbents are more effective for the removal of Cd from wastewater than Ni.
3.3. Effect of pH
Removal of a pollutant from an aqueous medium by biosorbent is dependent on the solution pH, which affects the surface charge of the biosorbent and the degree of ionization of the pollutant. The effect of initial pH in the removal of Cd and Ni onto nano-sorbents at the given experimental conditions was showed in . The maximum uptake capacity of both Ni and Cd on nano-silver and nano-magnetic iron oxide were shown at pH of 10 with percentage sorption capacity of 98% and 97%, respectively. These observations indicate that neutral/un dissociated Cd and Ni compounds get preferably adsorbed on the surface of the biosorbent in its molecular form. Also, the two biosorbents work well in an alkaline medium; the results show evidence that pH acts exclusively in the chemistry of metals in aqueous solution, but not in the chemical state of the surface of the adsorbent. These results are in complete agreement with the results reported by (Navarro et al., Citation2008) for brown marine seaweeds biosorbents.
3.4. Effect adsorbent dosage
The effect of adsorbent dosage was investigated by varying the dose between 0.05 and 0.3 g/100 ml (). The results reveal that the adsorption of metals increases gradually with an increase in the amount of silver oxide Ag2O-nono-biosorbent () for both two metals due to the greater availability of metal binding sites of the surface area at higher amount of the adsorbent, which increases the adsorption percentage. On the other hand, the adsorption capacity (qe) decreased with the increase in dosage, because increased adsorbent quantity decreased the saturation of active sites. This falls the number of covered sites per unit mass of adsorbent, which leads to a fall in capacity, that is, it affects the adsorption capacity negatively (Vieira et al., Citation2010).
Figure 5. Effect of various doses on the uptake of Cd and Ni by nano-silver oxide and nano-magnetic iron oxide biomass.
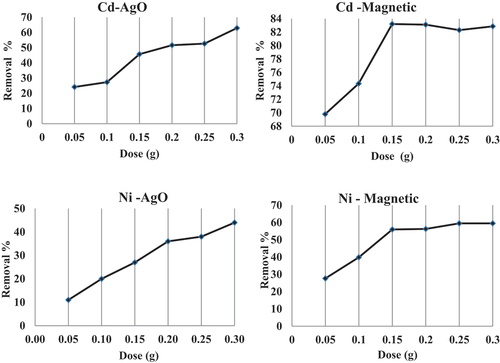
In case of Fe3O4 nanosorbents, the maximum percentage removal recorded at dosage of 0.15 g/ 100 ml for both Cd and Ni solutions, after that, the percentage removal of the two metals curves was smooth and continues leading to saturation (). This can be attributed to the increased adsorbent surface area and availability of more adsorption sites resulting from the increase dosage of the adsorbent.
3.5. Effect of contact time
The effect of contact time on the adsorption of Cd and Ni ions from wastewater was studied at various intervals between 30 and 180 min. The results are summarized in ; it is clear that the trend is similar in case of removal of Cd by nano-magnetic and Ni by nano silver, respectively; the percent removal of the two metals was increased by increasing the contact time. In case of adsorption of Cd by nano silver, it is evident that the adsorption is fast initially and becomes slower at the equilibrium at 150 min. This may be due to the availability external surface area of adsorbent during initial stages, which gets exhausted as adsorption proceeds (Ahamad et al., Citation2019). The adsorption of Ni by nano-magnetic particles is fast initially and becomes slower (after 90 min) and become constant to150 min as it attains equilibrium then very slow after that. Such behavior is typical from the presence of several adsorption sites on the biosorbent surface in the initial stage of reaction, which gradually gets saturated with the Ni at increasing contact times.
Figure 6. Effect of contact time on the uptake of Cd and Ni by nano-silver oxide and nano-magnetic iron oxide biomass.
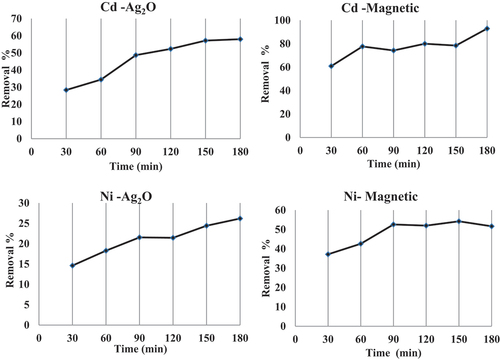
The sorption capacity for both Cd and Ni ions in the present study by nano-magnetic iron oxide and nano-silver oxide in Phragmites australis from Polluted Huge Drain was higher than that recorded by Arecoa et al, (Citation2012) and Selatnia et al. (Citation2004) on the removal of Cd by Green alga Ulva lactuca and Streptomyces rimosus biomass as bio-sorbents from aqueous Solution. In the same context, the sorption capacity of the present study is also higher than that measured by Danila et al. (Citation2018) and Genç-Fuhrman et al. (Citation2008) of the removal of Cd and Ni using iron-based sorbent and Bacterium planococcus sp from polluted water and the aqueous solution, respectively. This means that the two modified nano-sorbents were more effective in removing Cd and Ni than the previously mentioned sorbents by other authors (). On the other hand, the sorption capacity of the two bio-sorbent in the present study was in agreement with that measured by Siswoyo et al. (Citation2014) and Guo et al. (Citation2018) for Cd and with Hoseini et al. (Citation2020) for Cd and Ni.
Table 1. Comparison of nickel and cadmium biosorption by different bio-sorbent
3.6. Kinetics of metals biosorption
The kinetics of adsorbate uptake is important for choosing optimum operating conditions for design purposes. The rate of sorption of metal onto modified nano-sorbent was evaluated using different kinetic models: the pseudo-first-order, pseudo-second-order kinetics and intraparticle diffusion models and their regression coefficients were compared. The best fit model was selected based on the linear regression correlation coefficient (R2), which is a measure of how well the predicted values from a forecast model match with the experimental data.
3.6.1. Pseudo-first-order kinetic model
In order to determine the uptake rate of Cd and Ni on the nano-synthesized biosorbent and get access to the equilibrium time, studies on adsorption kinetics were carried out. The pseudo-first-order Lagergren model (Lagergren, Citation1898) assumes that the rate of occupation of sorption sites is proportional to the number of unoccupied sites and expressed by EquationEq. (2(2)
(2) ),
where qe and qt (mg/g) are the amount of metal adsorbed at equilibrium time and time t (min), respectively. Values of the Lagergren rate constant, k1, equilibrium adsorption capacity, qe, and the correlation coefficient, R2, were calculated from the plots of log (qe −qt) versus t ().
Figure 7. Lagergren pseudo first- and second-order kinetics biosorption for Cd and Ni onto two nano-sorbents.
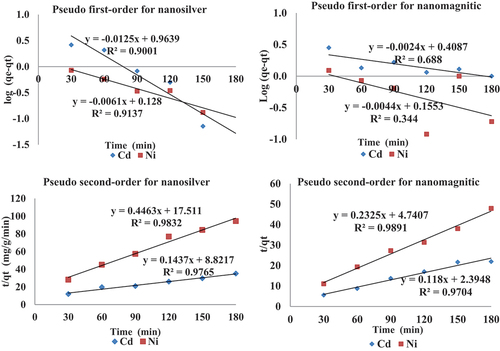
The straight-line plot of log (qe − qt) against t gives log (qe) as slope and intercept equal to k1/2.303. Hence, the amount of metal sorbed per gram of sorbent at equilibrium (qe) and the first-order sorption rate constant (k1) can be evaluated from the slope and the intercept. The equilibrium rate constant of the pseudo-first-order sorption (k1) was observed to be 2.219 and 0.941 min−1 for Cd and 0.295 and 0.357 min−1 for Ni for the two nanosorbents, respectively. It was observed from that the Lagergren model fits well for a contact time of 0–90 min for Ni at the two biosorbents the adsorption was rapid, and then slowed considerably, and thereafter the date deviates from the theory. On the contrary, in the case of Cd adsorption, the curve never takes a straight line in the two biosorbents, even at a high regression coefficient. Thus, the model represents the initial stages where rapid adsorption occurs well but cannot be applied for the entire adsorption process.
From , the qe (cal) values calculated from pseudo-first-order kinetic model differed appreciably from the experimental values. The regression coefficient values were recorded of 0.90 and 0.69 for Cd and 0.913 and 0.344 for Ni, respectively (), which shows that the model can be applied but is not appropriate to describe the entire process; this is probably due to some complex nature of adsorption.
Table 2. Comparison of the first- and second-order adsorption rate constants and calculated and experimental qe values for Cd and Ni and nano-biosorbent
3.6.2. Pseudo-second-order kinetic model
Adsorption kinetics were explained by the pseudo-second order that is based on the assumption that the rate-limiting step due to physicochemical interactions between the two phases, where the rate-limiting step is the surface adsorption (chemisorption) involving valence forces through sharing or exchange of electrons among metal ions and adsorbent. Equation of the pseudo-second order was given by Ho et al. (Citation2000) and was rearranged to obtain a linear form (EquationEq. 3)(3)
(3) :
where k2 (g mg−1 min−1) is the equilibrium rate constant of pseudo-second-order adsorption. The linear plot t/qt versus t shown in . The second-order rate constants were used to calculate the initial sorption rate, h (mg g−1 min−1) is expressed by the following EquationEq. (4)(4)
(4) :
The second-order rate constant, K2, and the equilibrium adsorption capacity, qe, were calculated from the intercept and slope of the plots of t/qt versus t. The calculated qe (cal) values from the pseudo-second-order kinetic model are very close to qe (exp) at two initial metal ions concentrations (). Further, the values of correlation coefficients (R2) of the pseudo-first-order model were less than the pseudo-second-order model. Over the above, a regression coefficient of above 0.970 of the pseudo-second-order model for the two metals by the two nano-sorbent shows that this model can be applied for the entire adsorption kinetic process and confirms the chemisorption of the nano-sorbents for Cd and Ni; also, it is better obeyed than the pseudo-first-order model.
3.6.3. Adsorption mechanism
3.6.3.1. Weber and Morris model
For a sorption mechanism, the solute transfer is usually characterized by external mass transfer (boundary surface layer diffusion), or intraparticle diffusion, or both. The adsorption of Cd and Ni onto two nano-biosorbent may be controlled due to surface diffusion at first stage and after that by the intraparticle diffusion. Over the whole time of plotting, the graphs are not linear indicating that more than one step is including in the adsorption of metal ions. The intra-particle diffusion model is used to investigate the adsorption behavior; the probability of the intra-particle diffusion was explored by using the equation suggested by Weber & Morris (Citation1964):
where qt (mg g−1) is the adsorption capacity at any time t(min), Kid is the intraparticle diffusion rate constant (mg g −1 min1/2), C is the value of intercept which gives an idea about the boundary layer thickness, i.e., the larger intercept; the greater is the contribution of the surface adsorption in the rate limiting step. The plots of qt vs. the square root of time obtained for the adsorption of Cd and Ni onto nano-sorbent at different time are shown in . The two regions in the qt vs t0.5 plot suggest that the sorption process proceeds by surface sorption and intraparticle diffusion. The initial curved portion of the plot indicates a boundary layer effect, while the second linear portion is due to intraparticle or pore diffusion. Although linear for a wide range of contact periods but not linear over the whole time range and not pass through the origin indicating that more than one step is contributory in the adsorption of metal ions. The rate constant of pore diffusion (Kid) for the solute was thus calculated from the slope of the linear portion of the plot (). From , in case of nano-magnetic iron oxide biosorbent for both Cd and Ni the intercept values are higher than that in nano-silver oxide Biosorbent, that is, the boundary layer thickness (film diffusion) or the surface adsorption in the rate controlling step in nano-magnetic. The calculated intraparticle diffusion coefficient Kid values are listed in .
Table 3. Values of the parameters of kinetic models for Cd and Ni onto nano-silver and nano-magnitic Biosorbent
3.6.3.2. Boyd model
From the intra-particle diffusion study, it becomes evident that sorption of Cd and Ni ions on nano-magnetic and nano-silver particles is a complex and multi-stage process. But the process which controls the overall rate of sorption process remains uncertain which needs to be addressed. In order to determine the actual rate limiting step a complex mathematical expression given by Boyd et al. (Citation1947) have been applied to the sorption kinetics data. The Boyd expression is represented as in Equationeq (6)(6)
(6) :
Where, qe is the amount of metal ions adsorbed at equilibrium (mg g1), qt is the amount of ions adsorbed at any time t (min) and Bt is a mathematical function of F. EquationEquation (6)(6)
(6) can be rearranged by taking the natural logarithm to obtain EquationEq (8)
(8)
(8) :
According to Boyd model, if the plot of Bt against time () produces a straight line that passes through the origin, the intraparticle diffusion is the adsorption rate-controlling step, in the present study, all the plots for the two nano-sorbent and metals provided linear relationship but not pass through the origin. Thus, the external mass transfer governs the overall rate of the removal process. In another word, film diffusion or the chemical reaction seems to be controlling the overall rate of the Cd and Ni sorption on both nano-sorbents. The effective diffusion coefficients (Di, cm2/s) for different nano-sorbents, found from the slope of the Bt vs t plot, are listed in .
The present results on removal of Cd and Ni by nano-magnetic iron oxide and silver oxide nano-sorbent were better fit with Mahmoud et al. (Citation2018) who use Starch-coated TiO2 nanoparticles for removal Cd from spiked tap-water. The present study agrees with Li et al. (Citation2003), who carried out the oxidation of carbon nano-tubes (CNTs) with HNO3, H2O2, and KMnO4 () and received increased adsorption capacities for adsorption of Cd (II) ions, compared to the adsorption capacity of the untreated carbon nanotubes, which was 1.1 mg/g. Also, our results for the removal of Cd agree with the those for Yan et al. (Citation2003; Ping et al. (Citation2015), who gave good results and showed that MWCNTs treated with nitric acid, and the Graphene oxide successfully removed Cd ions from the aqueous solution. On the other hand, our results for removing Ni agreed with Mahdavi et al. (Citation2013), which investigated the different adsorption effects of nanosized TiO2, toward Ni (II), from aqueous solutions.
Table 4. Adsorption capacities of different adsorbents for the removal of Cd and Ni
4. Conclusion
The pollution of drains water with heavy metals is a major issue worldwide, so, the development of suitable technology to remove heavy metals from this wastewater is necessary. Accordingly, in many parts of the world, industrial wastewater and sewage are discharged directly to waterways such as canals and drains, which, in turn, discharge their waters to lakes or the sea (larger water bodies). This study focused on treating this water in order to be used directly or indirectly through reduce pollution in the end receptor, especially in Egypt, in light of the present water crisis. Therefore, this study found that nanoparticles have a good sorption capacity for different pollutants, especially heavy metals and organics. The aquatic macrophytes (Phragmites australis) was natural materials that can be used as low cost, safe, and effective adsorbents was prepared as nano-magnetic iron oxide (Fe3O4 nano-sorbents) and silver oxide nanoparticles and used for the removal of Cd and Ni ions from polluted drain water. Generally, the sorption efficiency was dependent on the pH of the solution, reaction time, temperature, and initial metal concentration in the solution. The decrease in particle size enhanced sorption properties due to increases in the specific surface area. Pseudo-second-order model can be applied for the entire adsorption kinetic process and confirms the chemisorption of the nano-sorbents for Cd and Ni, also it is better obeyed than the pseudo-first-order model. In case of Weber and Morris model in nano-magnetic iron oxide biosorbent for both Cd and Ni, the intercept values are higher than that in nano-silver oxide biosorbent, that is, the boundary layer thickness (film diffusion) or the surface adsorption in the rate controlling step in nano-magnetic. About Boyd model, the external mass transfer governs the overall rate of the removal process. In other words, film diffusion or the chemical reaction seems to be controlling the overall rate of the Cd and Ni sorption on both nano-sorbents.
Availability of Data and Materials
Our data citations were include a persistent identifier (DOI) and were ideally included in the reference list, and all DOIs were expressed as full URLs.
Authors’ contributions
“Maha Abdallah drew up the work plan and carried out the practical part of the research and was a major contributor in writing the manuscript. The author read and approved the final manuscript.”
Maha Ahmed Mohamed Abdallah
Professor of Marine Pollution and Environmental Chemistry
National Institute of Oceanography & Fisheries
Mediterranean Sea Branch, Alexandria, Egypt
Egyptian
The current activities in the field include monitoring and assessing marine pollutants, especially toxic metals and organic pollutants in water and sediment, that is, using the sequential chemical fractionation of trace elements and heavy metals in the surface sediment to identify the picture that there is a component of the mineral deposits and the potential to address, and also enriching the accumulation of heavy metals in the sediments. In addition, it includes the preparation of nanoparticles from natural marine materials and using it in the removal of some pollutants from industrial wastes and contaminated drinking water.
correction
This article has been republished with minor changes. These changes do not impact the academic content of the article.
Acknowledgments
The author would like to thank the National Institute of Oceanography and Fisheries (NIOF); this research was part of NIOF’s search strategy for 2019. Also, the author would like to thank colleagues in the Marine pollution lab in the National Institute of Oceanography and Fisheries (NIOF) for their support and help during the practical section.
Author Contributions: M.A designed and supervised the project; the experiments, analyzed the data, and wrote this paper, submitted comments and corrected it.
Disclosure statement
No potential conflict of interest was reported by the author(s).
Additional information
Funding
Notes on contributors
Maha Ahmed Mohamed Abdallah
Dr. Maha Abdallah is professor at the Marine Pollution department and leader of the heavy metals group at the National Institute of Oceanography & Fisheries, Mediterranean Sea Branch in Egypt. She earned her B.Sc. in the Chemistry Department at the Faculty of Science of Alexandria University in Alexandria and her M.Sc. in Environmental Studies at the Institute of Higher Studies and Research at Alexandria University. She earned her Ph.D. in Marine Chemistry from the General Oceanography Department at the Faculty of Science of Alexandria University. Since then, she was a lecturer in Marine Pollution lab at the Marine Environment Department. She supervised and headed several national projects, postgraduate students and has several activities.
References
- Abdallah, M. A. M. (2012). Phytoremediation of heavy metals from aqueous solutions by two aquatic macrophytes, Ceratophyllum demersum and Lemna gibba L. Environmental Technology, 33(14), 1609–19. https://doi.org/10.1080/09593330.2011.640354
- Abdallah, M. A. M. (2014). Chromium geochemistry in coastal environment of the Western Harbour, Egypt: Water column, suspended matter and sediments. Journal of Coastal Conservation, Planning and Management, 18(1), 1–10. https://doi.org/10.1007/s11852-013-0288-6
- Abdallah, M. A. M., & Badr El Din, A. (2020). Ecological risk assessment by heavy metals from the surficial sediment of a submerged archaeology harbour, Egypt. ACTA Geochemica, 39(2), 226–235. https://doi.org/10.1007/s11631-019-00340-2
- Abdallah, M. A. M., Mohamed, E. M., Osman, M. M., & Ahmed, S. B. M. (2017). New biosorbent in removing some metals from industrial wastewater in El Mex Bay, Egypt. Applied Water Science, 7(4), 1931–1942. https://doi.org/10.1007/s13201-015-0371-2
- Ahamad, T., Naushad, M., & Alshehri, R. S. M. (2019). Ultra-fast spill oil recovery using a mesoporous lignin based nanocomposite prepared from date palm pits (Phoenix dactylifera L). Int J Biol Macromol, 130, 139–147. https://doi.org/10.1016/j.ijbiomac.2019.02.038
- American Public Health Association (APHA). (2005). Standard methods for the examination of water and wastewater. Washington DC.
- Arecoa, M. M., Hanela, S., Duranb, J., & Afonso, M. S. (2012). Biosorption of Cu(II), Zn(II), Cd(II) and Pb(II) by dead biomasses of green alga Ulva lactuca and the development of a sustainable matrix for adsorption implementation. Journal of Hazardous Materials, 213–214(2012), 123–132. https://doi.org/10.1016/j.jhazmat.2012.01.073
- Boyd, G. E., Adamson, A. W., & Myers, L. S. (1947). The Exchange Adsorption of Ions from Aqueous Solutions by Organic Zeolites. II. Kinetics1. Journal of the American Chemical Society, 69(11), 2836–2848. https://doi.org/10.1021/ja01203a066
- Danila, V., Vasarevicius, S., & Valskys, V. (2018). Batch removal of Cd(II), Cu(II), Ni(II), and Pb(II) ions using stabilized zero-valent iron nanoparticles. Energy Procedia, 147, 214–219. https://doi.org/10.1016/j.egypro.2018.07.062
- Genç-Fuhrman, H., Wu, P., Zhou, Y., & Ledin, A. (2008). Removal of As, Cd, Cr, Cu, Ni and Zn from polluted water using an iron based sorbent. Desalination, 226(1–3), 357–370. https://doi.org/10.1016/j.desal.2007.02.117
- Guo, X., Tang, S., Song, Y., & Nan, J. (2018). Adsorptive removal of Ni+2 and Cd+2 from wastewater using a green longan hull adsorbent. Adsorption Science & Technology, 36(1–2), 762–773. https://doi.org/10.1177/0263617417722254
- Ho, Y. S., McKay, G., Wase, D. A. J., & Foster, C. (2000). Study of the sorption of divalent metal ions on to peat, Adsorp. Sci. Technol, 18, 639–650. https://doi.org/10.1260/2F0263617001493693
- Hoseini, A. A., Kaboosi, H., Ahmady-Asbchin, S., Ghorbanalinezhad, E., & Ghadikolaii, F. P. (2020). Binary biosorption of Cadmium (II) and Nickel (II) onto planococcus sp. isolated from wastewater: Kinetics, equilibrium and thermodynamic studies. Biomedical Research and Clinical Practice, 5(2), 1–7. https://doi.org/10.15761/BRCP.1000208
- Lagergren, S. (1898). Zur theorie der sogenannten adsorption geloster stoffe,Kungliga Svenska Vetenskapsakademiens. Handlingar, 24, 1–39. https://doi.org/10.1007/BF01501332
- Li, Y.-H., Wang, S., Luan, Z., Ding, J., Xu, C., & Wu, D. (2003). Adsorption of cadmium(II) from aqueous solution by surface oxidized carbon nanotubes. Carbon, 41(5), 1057–1062. https://doi.org/10.1016/S0008-6223(02)00440-2
- Mahdavi, S., Jalali, M., & Afkhami, A. (2013). Heavy metals removal from aqueous solutions using TiO2, MgO, and Al2O3 nanoparticles. Chemical Engineering Communications, 200(3), 448–470. https://doi.org/10.1080/00986445.2012.686939
- Mahmoud, M. E., Ahmed, S. B., Osman, M. M., & Abdel-Fattah, T. (2015). A novel composite of nanomagnetite-immobilized-baker’s yeast on the surface of activated carbon for magnetic solid phase extraction of Hg(II). Fuel, 139, 614–621. http://dx.doi.org/10.1016/j.fuel.2014.09.002
- Mahmoud, M. E., Ali, S. A. A. A., & Elweshahy, S. M. T. (2018). Microwave functionalization of titanium oxide nanoparticles with chitosan nanolayer for instantaneous microwave sorption of Cu(II) and Cd(II) from water. International Journal of Biological Macromolecules, 111, 393–399. PMID: 29309870. https://doi.org/10.1016/j.ijbiomac.2018.01.014.
- Navarro, A. E., Portales, R. F., Sun-Kou, M. R., & Lianos, B. P. (2008). Effect of pH on phenol biosorption by marine seaweeds. Journal of Hazardous Materials, 156(1–3), 405–411. PMID: 18249490. https://doi.org/10.1016/j.jhazmat.2007.12.039 .
- Ping, T., Jian, S., Yongyou, H., Zheng, F., Qi, B., Yuancai, C., & Jianhuan, C. (2015). Adsorption of Cu2+, Cd2+ and Ni2+ from aqueous single metal solutions on graphene oxide membranes. Journal of Hazardous Materials, 297, 251–260. PMID: 25978188. https://doi.org/10.1016/j.jhazmat.2015.04.068.
- Selatnia, A., Bakhti, M. Z., Madani, A., Kertous, L., & Mansouri, Y. (2004). Biosorption of Cd2+ from aqueous solution by a NaOH-treated bacterial dead Streptomyces rimosus biomass. Hydrometallurgy, 75(1–4), 11–24. https://doi.org/10.1016/j.hydromet.2004.06.005
- Siswoyo, E., Mihara, Y., & Tanaka, S. (2014). Determination of key components and adsorption capacity of a low cost adsorbent based on sludge of drinking water treatment plant to adsorb cadmium ion in water. Applied Clay Science, 97–98, 146–152. https://doi.org/10.1016/j.clay.2014.05.024
- Solomon, S. D., Mulfinger, L., Bahadory, M., Jeyarajasingam, A. V., Rutkowsky, S. A., & Boritz, C. (2007). Synthesis and study of silver nanoparticles. Journal of Chemical Education, 84(2), 322. https://doi.org/10.1021/ed084p322
- Vieira, M. G. A., Neto, A. F. A., Gimenes, M. L., & da Silva, M. G. C. (2010). Sorption kinetics and equilibrium for the removal of nickel ions from aqueous phase on calcined Bofe bentonite clay. Journal of Hazardous Materials, 177(1–3), 362–371. https://doi.org/10.1016/j.jhazmat.2009.12.040
- Weber, W. J., & Morris, J. C. (1964). Equilibria and capacities for adsorption on carbon. Journal of the Sanitary Engineering Division, 90(3), 79–91. https://doi.org/10.1061/JSEDAI.0000496
- Yan, H. L., Ding, J., Luan, Z., Di, J., Zhu, Y., Xu, C., Wu, D., & Wei, B. (2003). Competitive adsorption of Pb2+, Cu2+ and Cd2+ ions from aqueous solutions by multiwalled carbon nanotubes. Carbon, 41(14), 2787–2792. https://doi.org/10.1016/S0008-6223(03)00392-0