Abstract
There are limited non-invasive treatment options in the home environment for children with cerebral palsy (CP); thus, evaluating such treatment options is needed. We aimed to evaluate the effect of an innovative full-body suit approach for non-invasive surface electrical stimulation ES, the electro-dress Mollii®. The full-body suit was primarily designed to reduce spasticity and improve motor function through the mechanism of reciprocal inhibition among children with CP. This study involved seven experimental single-case studies with an ABAB design. Seven studies were performed among children aged 4–17 years. The primary outcome (spasticity) was analyzed using graphed data with a visual inspection, and median values were analyzed for secondary outcomes (mobility, sitting, upper limb activity, sleep, pain, and adherence to treatment). The study protocol was recorded at clinicaltrials.gov (NCT04078321). Seven studies were analyzed, and the treatment with the electro-dress had little or no impact on the outcomes: spasticity, mobility, sitting, upper limb activity, sleep, and pain, in any of these seven studies. In conclusion, multiple ES treatment with the innovative electro-dress Mollii® revealed little or no observable changes on primary outcome (spasticity) in any of the seven studies. Further the possible impact on motor function is recommended to be further evaluated in future studies
PUBLIC INTEREST STATEMENT
More knowledge about non-invasive treatment options to reduce spasticity and increase physical function in the home environment for children with cerebral palsy (CP) is warranted. A suggested and feasible method to increase physical function is to use multiple electrical stimulation (ES) provided in a full body suit, the electro-dress Mollii®. This method requires optimal administration and dosing of electrical stimulation over time to potentially have an impact on physical function. The benefit of the electro-dress Mollii® needs to be further investigated.
1. Introduction
Cerebral palsy (CP) is caused by damage to the immature brain and is the most common cause of motor disability in children (Rosenbaum et al., Citation2007). The prevalence of CP varies among countries; however, it is reported to be approximately 2–4 per 1,000 live births (Boyle et al., Citation2011). There are different subtypes of CP, based on the extent of damage, which can be classified as bilateral or unilateral, according to the dominant symptoms, as follows: spastic, dyskinetic, or ataxic. Approximately 80% of patients have a spastic CP subtype (Westbom et al., Citation2007). Other common secondary symptoms resulting from CP include a restricted range of motion (ROM), pain, sleep, and difficulty performing movements, such as gait and upper limb activity, which is a common problem among patients with CP (Rosenbaum et al., Citation2007). Further, it has been suggested that treatments for the prevention of contractures should be initiated early considering that ROM seems to decrease over time, leading to movement restriction, which in turn results in a lower level of functioning and eventually a decreased quality of life (Casey et al., Citation2021; Jarl et al., Citation2019).
Some of the most used treatments for spasticity include botulinum neurotoxin (BoNT) in combination with therapies, such as baclofen, orthoses, and surgery (Hoare, Citation2014; Novak et al., Citation2013; Ryan et al.). Further, exercise (aerobic exercise, resistance training, and “mixed training”) as a treatment option for persons with CP to increase activity, participation, and quality of life have been suggested to be effective (Gimigliano, Citation2020; Ryan et al., Citation2017;). However, the evidence for such specific exercise intervention is still sparse (Gimigliano, Citation2020). Therefore, new ways to address both spasticity and activity aspects should be further investigated. One example of such interventions targeting both spasticity and activity outcomes is the use of transcutaneous electrical stimulation (TENS), a non-invasive modality, to reduce spasticity by placing surface electrodes on the antagonist muscles, inhibiting the overactivity in the spastic agonist muscle. The use of the TENS is based on the theory of reciprocal inhibition and may reduce hyperactive stretch reflexes by improving the inhibition of the H-reflex, which may lead to a reduction in the contraction of spastic muscles; this phenomenon may be reflected in a better balance between muscles (AA Alhusaini et al., Citation2019; IlliS, Citation1994). Reducing spasticity using TENS has previously been evaluated and is a promising therapeutic option (AA Alhusaini et al., Citation2019; Mahmood et al., Citation2019). Further, neuromuscular electrical stimulation (NMES) has been shown to strengthen muscles in patients with stroke and CP, and there is increasing evidence of NMES for increased muscle fiber diameter and muscle size as well as strength in children with CP (De Freitas Gr et al., Citation2018; Takeda et al., Citation2017). Additionally, single-channel NMES has been used to improve gait functions in patients with spinal cord injuries and stroke; however, the use of multi-channel NMES has been rarely investigated (Mooney & Rose, Citation2019).
Although studies have provided evidence for the use of electrical stimulation (ES) to reduce spasticity over the years, this treatment option has been difficult to apply in therapy when performing exercise due to the placement of the electrodes (Ertzgaard et al., Citation2018). One way to easily attach surface electrodes has been tested by the Mollii® method, multiple ES provided in a full body suit (Ertzgaard et al., Citation2018). By the Mollii® method, multi-channel or multiple ES involves the concurrent transfer of electrical signals by several electrodes to different antagonist muscles; however, evidence supporting such treatment remains unclear (Ertzgaard et al., Citation2018; Palmcrantz et al., Citation2020; Pennati et al., Citation2021).
Given that ES is a relatively safe intervention with an acceptable adverse event profile and because there are limited treatment options in the home environment for children with CP, there is a need for further evaluation of such treatment options for this target group. Therefore, this study aimed to evaluate the effect of ES treatment with the full-body suit (electro-dress) Mollii®, on spasticity, mobility, sitting, upper limb activity, sleep, pain, and adherence (frequency of use) to the treatment using experimental single-case studies with an ABAB design among children with CP (4–18 years of age).
2. Materials and methods
We used experimental single-case studies with an ABAB design (Kazdin, Citation2011; Onghena, Citation2005) to evaluate the individual effect of ES in a full-body suit, the electro-dress Mollii®. The ABAB design is a prospective design suited to study the effects of interventions in individual cases. The design was chosen because of significant variation in the population of individuals with CP in the geographical area. In an ABAB design, each participant is his or her control during alternating baseline (A) and intervention (B) phases. The possibility to establish the success of an intervention for a particular patient, as opposed to an average patient in group designs, is a strength of experimental single-case designs. Additionally, replication of results in several single-case studies strengthens generalizability. This study reports the results of seven experimental single-case studies. A study protocol was recorded at clinicaltrials.gov under NCT04078321.
2.1. Participants
Seven children who met the inclusion criteria and were available at two habilitation centers (HC) in Sweden from April 2019 to September 2019 accepted to participate in this study. Verbal information and written informed consent were collected from the participants and/or their guardians. The seven studies included four girls and three boys between 4 and 17 years of age (see, Table ). In five studies, participants used a walking aid in their daily lives (three girls and two boys). Two participants had a unilateral CP-subtype (study 3 and 5), and five had a bilateral CP-subtype.
Table 1. Characteristics, n = 7
The inclusion criteria include a) a diagnosis of CP, with spasticity as the main symptom according to information from the medical HC journal; b) Gross Motor Function Classification System (GMFCS) levels I–V. GMFCS describes gross movement ability at five levels. Level I represents the highest ability but with some difficulties in speed, balance, and coordination, while level V represents the lowest ability, with difficulties in controlling head and trunk posture in most positions (Palisano et al., Citation2008); c) Manual Ability Classification System (MACS) [levels 1–5] describes the use of hands to handle objects in daily activities at five levels; level 1 represents the highest function, and level 5 represents the lowest function. (Eliasson et al., Citation2006); d) age 4–18 years; and e) ability to communicate pain or discomfort.
The exclusion criteria were as follows: a) botulinum toxin treatment three months before participation in the study; and b) having a shunt or other medical pump, such as a baclofen and insulin pump, which may be affected by the ES provided by the suit.
2.2. Intervention
Each participant was scheduled for a first individual meeting to adapt to the suit in collaboration with a physiotherapist (PT) from the company delivering the suit. The company was responsible for the surface electrodes´ placements to influence the participants’ spasticity. An occupational therapist (OT) and/or physiotherapist (PT) from the HCs attended the initial meeting where the electrodes and the settings in the suit were tested before initiating ABAB phases but did not participate in decisions regarding the placement of the electrodes. The modified Ashworth Scale (Bohannon & Smith, Citation1987) was used to identify the appropriate ES placement at the initial meeting before the study started. For each participant, the three highest scores on the MAS were selected and included in this study.
The intervention consisted of a full-body suit with embedded electrodes providing ES. The suit consists of a pair of trousers and a jacket equipped with 58 electrodes that provide electrical signals inside the suit. A detachable control unit sends electrical signals and activates electrodes placed on individually selected antagonist muscles. The location of electrodes and intensity of stimulation were individually adapted simultaneously as the suit was tried on the participant by the company that distributed the suit. The number of electrodes used depends on the participants’ symptoms of spasticity and the muscles requiring inhibition. Stimulation parameters include pulse duration (0–30 microseconds), frequency 20 Hz, and voltage 20 V. The treatment effect is described by the company delivering the suit as individual and can last 48 hours or longer after 1 hour of use. The ES stimulation settings are saved in the control unit, making it simple for the device to be used at home or school.
The stimulation frequency and the placement of the electrodes were individually adjusted for each muscle group where the antagonist of each selected spastic muscle was stimulated to reduce the reflex mediated muscle overactivity of an antagonist muscle, promoting the balance between agonist and antagonist according to the theory of reciprocal inhibition.
Each participant started the four-week intervention period within two weeks after the initial meeting and adjustment of the suit. The treatment regimen was 1 hour every other day (3–4 times per week) in daily activities. Adverse events were recorded during the study period.
2.3. Assessments
OTs and PTs with working experience at the HCs took all the measurements. Original instructions and manuals were provided in booklets and delivered at the beginning of the study. The therapist taking the measurements was not involved in the treatment, which was home-based.
All participants were evaluated on 12 occasions during a 4-week period, three times each week. The first week included three measurements during an inactive period (baseline: A phase). Meanwhile, the second week included three measurements during an active period (first B phase), and the third week (second A phase) included three measurements wherein the suit should not be used for 48 hours before the first measurement. The fourth week included three measurements during an active period (second B phase). In addition, the participants and their guardians received a diary to record sleep, pain, and adherence to the treatment.
Spasticity was measured using the modified Ashworth Scale (MAS). MAS consists of a 6-point scale (0, 1, 1+, 2, 3, and 4), where 0 indicates normal muscle tone and 4 indicates high spasticity or increased resistance to passive movement (Mutlu et al., Citation2008).
Mobility, sitting, and upper limb activity were measured using the following three methods:
1) Timed Up and Go test (TUG): This test assesses mobility and requires both static and dynamic balance. The time required to rise from a chair, walk 3 meters, turn around, walk back to the chair, and sit down is recorded (Dhote et al., Citation2012).
2) Level of Sitting Scale (LSS): This evaluates the ability to sit on an 8-point scale, where 1 indicates an inability to attain a sitting position, and 8 indicates the ability to lean backward and back to the starting position again, without using the hands. LSS is considered to have moderate-to-good reliability (Fife et al., Citation1991).
3) Box and Block Test: This is used to measure the capacity of the timed upper limb motor dexterity. The test materials include a box divided into two equal compartments, with one compartment filled with colored cubes. The cubes are moved once with each hand. The Box and Block Test is valid and reliable for measuring upper limb manual dexterity at a young age (3–19 years; Araneda et al., Citation2019; Mathiowetz et al., Citation1985).
Data on pain, sleep, and adherence to using the suit were collected using a diary completed by the participant or their guardians. Pain was measured by a rating based on six facial expressions. The facial expressions ranged from a happy face at 0, representing no pain, to a crying face at 6, representing the worst imaginable pain (Westbom et al., Citation2017).
Sleep was rated via two questions about the quality of sleep as follows: 1) Do you wake up often at night? 2) Do you wake up rested or exhausted? The response format ranged from 1 = “worst quality” to 3 = “best quality of sleep.”
Adherence was rated by identifying the time the participants used the suit and the frequency of use in a home diary. All PTs and OTs were familiar with the measurements commonly used in daily practice for children with CP.
2.4. Ethics
The subjects received no compensation for participation. The study was approved by the Regional Ethics Committee in Stockholm DNR: 2019–01564. We followed the 1964 Helsinki Declaration with respect to human rights, informed consent, and correct procedures concerning the treatment in research involving human participants.
2.5. Statistical analysis
The primary outcome in each case was spasticity, as measured using the MAS, and was presented in graphs for the three most spastic measures at baseline. Owing to the limited amount of data points in each phase and the ordinal nature of the scores, we examined graphed data using visual inspection, considering changes in magnitude, i.e., level and median (Kazdin, Citation2011). The rating level 1+ on the MAS was converted to 1.5 in the graphs. The secondary outcomes are presented with their median values across the ABAB phases in each study.
3. Results
Seven experimental single-case studies with an ABAB design were performed. Regarding adherence, the frequency of using the suit varied from 2 to 5 (120–300 minutes) times in each of the two B phases. The total number of electrodes applied varied among the seven studies. It varied in intensity (pulse duration) from 2–16 microseconds with a set frequency of 20 Hz, and 20 V voltage. Since all participants were judged to have low scores on MAS, this study only included and evaluated the three most spastic agonists muscles according to the results from MAS (). The results are presented for the primary outcome for each study in . Secondary outcomes are presented in Table . All participants used the suit during their normal daily activities in their homes. Missing data resulted from impatience and/or measures being too easy or difficult to take and were, therefore, excluded.
Table 2. Secondary measures and their median values across experimental phases
Figure 1. Graph displaying measurement timepoints during the ABAB phases for experimental single- case study 1.
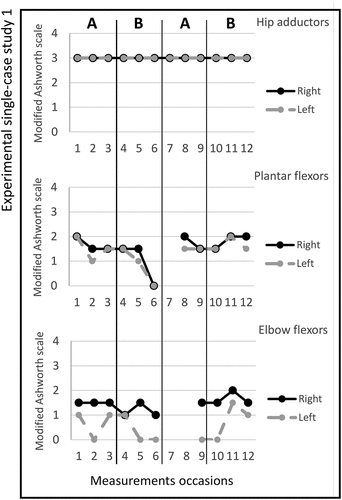
Figure 2. Graph displaying measurement timepoints during the ABAB phases for experimental single-case study 2.
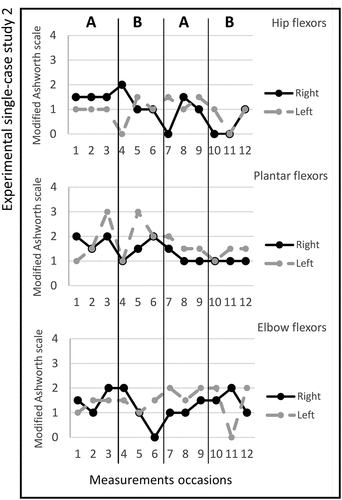
Figure 3. Graph displaying measurement timepoints during the ABAB phases for experimental single-case study 3.
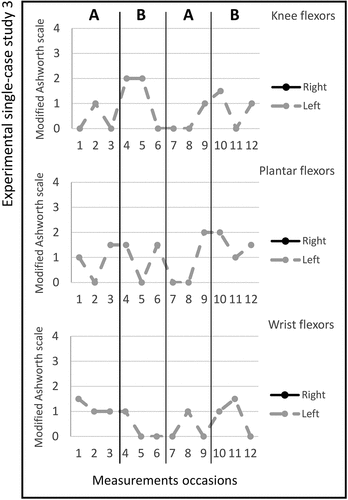
Figure 4. Graph displaying measurement timepoints during the ABAB phases for experimental single-case study 4.
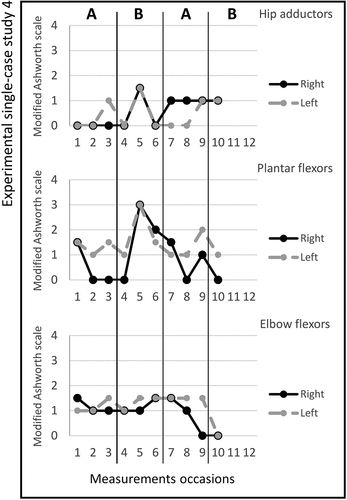
Figure 5. Graph displaying measurement timepoints during the ABAB phases for experimental single-case study 5.
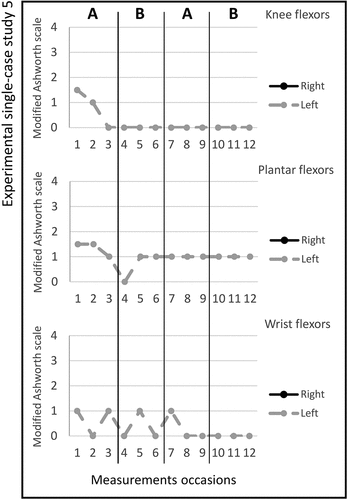
Figure 6. Graph displaying measurement timepoints during the ABAB phases for experimental single-case study 6.
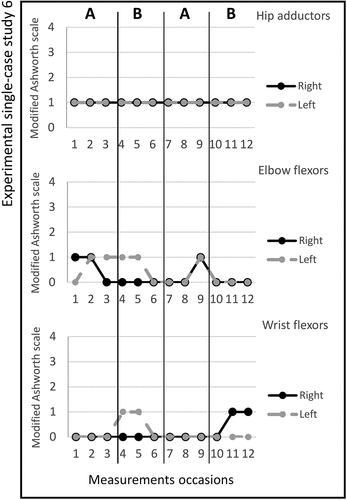
Figure 7. Graph displaying measurement timepoints during the ABAB phases for experimental single-case study 7.
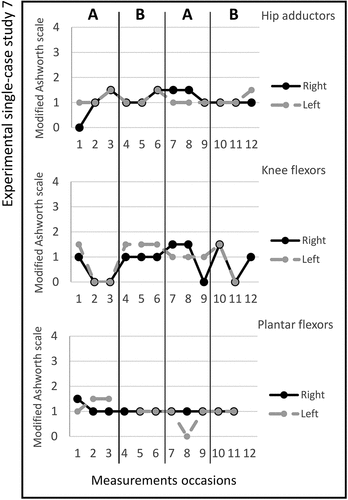
3.1. Results in each experimental single-case study
Study 1. The total number of stimulated muscles in study 1 was six, three on the left and three on the right side; Gluteus medius bilateral, tibialis anterior bilateral, and triceps brachii bilateral. The pulse duration was 2–12 microseconds, and no visual changes in magnitude were observed; the MAS scores across the experimental phases are shown in figure . The median scores for the secondary outcomes are shown in Table . The adherence rate during the two active periods was six times (4/2).
Study 2. The total number of stimulated muscles in study 2 was six, three on the left and three on the right side; Gluteus maximus bilateral, tibialis anterior bilateral, and triceps brachii bilateral. The pulse duration was 12–16 microseconds, and no visual changes in magnitude were observed; the MAS scores across the experimental phases are shown in figure . The median scores for the secondary outcomes are shown in Table . The adherence rate during the two active periods was five times (3/2).
Study 3. The total number of stimulated muscles in this study was five; Quadriceps femoris, tibialis anterior, extensor digitorum, extensor carpi radialis longus, and extensor carpi radialis brevis on the left side. The pulse duration was 8–12 microseconds, and no visual changes in magnitude were observed; the MAS scores across the experimental phases are shown in figure . A change in the Box and Block Test scores between ABAB phases were noted and presented in Table . All median scores for the secondary outcomes are shown in Table . The adherence rate during the two active periods was seven times (4/3).
Study 4. The total number of stimulated muscles in study 4 was six, three muscles on the left and three muscles on the right side; Tibialis anterior bilateral, triceps brachi bilateral and gluteus medius bilateral. The pulse duration was 2–12 microseconds, and no visual changes in magnitude were observed; the MAS scores across the experimental phases are shown in figure . Changes in the Box and Block Test scores between the ABAB phases were noted and presented in Table . All median scores for the secondary outcomes are shown in Table . The adherence rate during the two active periods was six times (3/3).
Study 5. The total number of stimulated muscles in study 5 was five; Tibialis anterior, extensor digitorum, extensor carpi radialis longus, extensor carpi radialis brevis, and quadriceps femoris on the left side. The pulse duration was 2–15 microseconds, and no visual changes in magnitude were observed; the MAS scores across the experimental phases are shown in figure . The median scores for the secondary outcomes are shown in Table . The adherence rate during the two active periods was six times (4/2).
Study 6. The total number of stimulated muscles in study 6 was ten, five on the left and five on the right side; Gluteus medius, triceps brachii, extensor digitorum communis bilateral, extensor carpi radialis longus bilateral, and extensor carpi radialis brevis bilateral. The pulse duration was 5–15 microseconds, and no visual changes in magnitude were observed; the MAS scores across the experimental phases are shown in figure . Changes in the Box and Block Test scores between the ABAB phases were noted and presented in Table . All median scores for the secondary outcomes are shown in Table . The adherence rate during the two active periods was nine times (4/5).
Study 7. The total number of stimulated muscles in study 7 was six, three on the left and three on the right side; Gluteus medius bilateral, quadriceps femoris bilateral, and tibialis anterior bilateral. The pulse duration was 12–15 microseconds, and no visual changes in magnitude were observed; the MAS scores across the experimental phases are shown in figure . Changes in the TUG test score between the ABAB phases was noted and presented in Table . The median scores for the secondary outcomes are shown in Table . The adherence rate during the two active periods was five times (2/3).
4. Discussion
We evaluated the effect of multiple ES stimulation with the electro-dress on spasticity, motor function, sleep, pain, and adherence to treatment in seven experimental single-case studies, with an ABAB design, in children aged 4–17 years with CP. The results suggest that the multiple ES treatment had little or no impact on the primary outcome—spasticity—in any of the studies.
Since reciprocal inhibition plays a fundamental role in the normal performance of movements, this was an underlying theory in this study. This theory has also been confirmed in previous studies, which reported ES as effective in increasing muscle strength, reducing spasticity, and increasing the passive ROM (Palmcrantz et al., Citation2020; Schuhfried et al., Citation2012). However, this study could not provide evidence to support the result of decreased spasticity.
In three of the studies, 3,4, and 6, the results showed a change individually between the A and B testing periods for the Box and Block Test, in both the right and left sides. Further, a change was observed between the A and B periods in the TUG test in study 7; however, these minimal changes were judged to have low clinical relevance; therefore, they were recommended to be further evaluated in multiple session of treatment for a longer time than in our study. Inconsistent results have been reported in the use of ES to improve activity measures, such as gait, activity, and participation levels, among children and adolescents with CP. However, studies have suggested that ES may offer unique benefits for gait rehabilitation (Moll et al., Citation2017; Mooney & Rose, Citation2019) and to improve foot drop in CP and stroke. Due to the inconsistency in the results obtained with the use of ES to improve activity measures, similar to the study by Pennati et al., our study supports the fact that future studies should consider the potential dose-response and involve multiple sessions of treatment over time (Pennati et al., Citation2021).
Our research team discussed the most appropriate time points for measurement following the treatment, and in our study, measurements were performed within approximately 48 hours. In a recently published study, the authors presented promising results after using the electro-dress Mollii® in 16 children with CP; however, the measures in that study were performed within 24 hours after treatment (Hedin et al., Citation2020). The optimal timing of measurements after multiple ES treatments needs to be further discussed, especially considering the clinical implications of such short-term effects.
The variation in the frequency of use, which ranged from 2–5 (120–300 minutes) times in each of the two B phases, appears to have a minimal systematic impact on the results. Adherence to using the suit was low in a previous study (Ertzgaard et al., Citation2018); however, a different pattern was observed in our study. Four of the participants used the suit only twice during one of their B periods (recommended at least three times in each B phase); however, according to the ABAB phases results, this was judged to have no impact. We also investigated sleep quality and pain; however, these measures indicated no changes across the ABAB phases. A relationship between pain and sleep and sleeping problems has been reported in 23–46% of children and adolescents with CP (Dutt et al., Citation2015).
4.1. Strength and limitations
There were several limitations associated with this study. First, the seven studies showed that treatment evaluation in a heterogeneous group of children with CP is complex. Different levels of functioning among these children and adolescents imply that large samples should be accommodated for potentially large within-group variations. Therefore, choosing a study design that can be used to investigate causality in individual cases was judged to be appropriate.
Furthermore, the secondary measurements revealed difficulties in performing the required tasks for most children, which warrants further investigation and consideration when planning future studies. This also strengthens the case for the use of a single-case study design and an individual approach. We are also aware of reports about the limitations of MAS for measuring spasticity and passive resistance to motion because the MAS scores a single value and does not discriminate between neural and non‐neural contributions (Damiano et al., Citation2007; Van den Noort Jc et al., Citation2017). It has been reported that measures, such as the MAS scale, lack precision and sensitivity for measuring and detecting smaller changes in spasticity. The assessment results may also relate to the therapist’s experience and subjective ability to identify signs and levels (Adel. A Alhusaini et al., Citation2010; Pandyan et al., Citation1999). Although MAS provides limited information, it is the most commonly used scale in clinical practice for measuring spasticity within the context of HC (Alriksson-Schmidt et al., Citation2017).
In conclusion, the results indicate that multiple ES stimulation with the electro-dress Mollii® had little or no effect on the primary outcome measure of spasticity in all the seven studies included in this study. Further, the possible impact of the electro-dress on motor function is recommended to be further evaluated considering potential dose-response and the involvement of multiple sessions of treatment over time.
Acknowledgements
This study was supported by the Centre for Clinical Research Sormland, Uppsala University, Sweden and the Social Contract, Mälardalen Competence Center for Health and Social Welfare.
Disclosure statement
No potential conflict of interest was reported by the author(s). https://doi.org/10.23736/S1973-9087.17.04791-8
Additional information
Funding
Notes on contributors
Marina Arkkukangas
Marina Arkkukangas more focus on how electrical stimulation such as the electro-dress Mollii® may affect movement performance in daily activities, i.e., provide real benefits for patients who use the method as a treatment alternative, is needed. Limitations in measuring and evaluating symptoms of spasticity need further attention in future studies evaluating the electro-dress Mollii® which is mainly aimed at affecting spasticity and secondary effects of spasticity. This project is related to other research interests in the research group, focusing on evidence-based practice and the use of appropriate measurements in research activities.
References
- Alhusaini, A., Dean, C. M., Crosbie, J., Shepherd, R. B., & Lewis, J. (2010). Evaluation of spasticity in children with cerebral palsy using Ashworth and Tardieu Scales compared with laboratory measures. Journal of Child Neurology, 25(10), 1242–16. https://doi.org/10.1177/0883073810362266
- Alhusaini, A. A., Fallatah, S., Melam, G. R., & Buragadda, S. (2019). Efficacy of transcutaneous electrical nerve stimulation combined with therapeutic exercise on hand function in children with hemiplegic cerebral palsy. Somatosensory & Motor Research, 36(1), 49–55. https://doi.org/10.1080/08990220.2019.1584555
- Alriksson-Schmidt, A. I., Arner, M., Westbom, L., Krumlinde-Sundholm, L., Nordmark, E., Rodby-Bousquet, E., & Hägglund, G. (2017). A combined surveillance program and quality register improves management of childhood disability. Disability and Rehabilitation, 39(8), 830–836. https://doi.org/10.3109/09638288.2016.1161843
- Araneda, R., Ebner‐Karestinos, D., Paradis, J., Saussez, G., Friel, K. M., Gordon, A. M., & Bleyenheuft, Y. (2019). Reliability and responsiveness of the Jebsen‐Taylor Test of Hand Function and the Box and Block Test for children with cerebral palsy. Developmental Medicine and Child Neurology, 61(10), 1182–1188. https://doi.org/10.1111/dmcn.14184
- Bohannon, R. W., & Smith, M. B. (1987). Interrater reliability of a modified Ashworth scale of muscle spasticity. Physical therapy, 67(2), 206–207. https://doi.org/10.1093/ptj/67.2.206
- Boyle, C. A., Boulet, S., Schieve, L. A., Cohen, R. A., Blumberg, S. J., Yeargin-Allsopp, M., Visser, S., & Kogan, M. D. (2011). Trends in the prevalence of developmental disabilities in US children, 1997-2008. Pediatrics, 127(6), 1034–1042. https://doi.org/10.1542/peds.2010-2989
- Casey, J., Agustsson, A., Rosenblad, A., & Rodby-Bousquet, E. (2021). Relationship between scoliosis, windswept hips and contractures with pain and asymmetries in sitting and supine in 2450 children with cerebral palsy. Disability and Rehabilitation, 1–6. https://doi.org/10.1080/09638288.2021.1971308
- Damiano, D. L., Quinlivan, J. M., Owen, B. F., Payne, P., Nelson, K. C., & Abel, M. F. (2007). Abel MF 2002 What does the Ashworth scale really measure and are instrumented measures more valid and precise? Developmental Medicine and Child Neurology, 44(2), 112. https://doi.org/10.1111/j.1469-8749.2002.tb00296.x
- de Freitas Gr, Szpoganicz, C., Ilha, J., & de Freitas, G. R. (2018). Does neuromuscular electrical stimulation therapy increase voluntary muscle strength after spinal cord injury? A systematic review. Topics in Spinal Cord Injury Rehabilitation, 24(1), 6–17. https://doi.org/10.1310/sci16-00048
- Dhote, S., Khatri, P., & Ganvir, S. (2012). Reliability of “Modified timed up and go” test in children with cerebral palsy. Journal of Pediatric Neurosciences, 7(2), 96. https://doi.org/10.4103/1817-1745.102564
- Dutt, R., Roduta-Roberts, M., & Brown, C. (2015). Sleep and children with cerebral palsy: A review of current evidence and environmental non-pharmacological interventions. Children, 2(1), 78–88. https://doi.org/10.3390/children2010078
- Eliasson, A. C., Krumlinde-Sundholm, L., Rösblad, B., Beckung, E., Arner, M., Öhrvall, A. M., & Rosenbaum, P. (2006). The Manual Ability Classification System (MACS) for children with cerebral palsy: Scale development and evidence of validity and reliability. Developmental Medicine and Child Neurology, 48(7), 549. https://doi.org/10.1017/S0012162206001162
- Ertzgaard, P., Alwin, J., Sörbo, A., Lindgren, M., & Sandsjö, L. 2018 Evaluation of a self-administered transcutaneous electrical stimulation concept for the treatment of spasticity: A randomized placebo-controlled trial. European Journal of Physical Rehabilitation and Medicine, 54:507–517 https://doi.org/10.23736/S1973-9087.17.04791-8.
- Fife, S. E., Roxborough, L. A., Armstrong, R. W., Harris, S. R., Gregson, J. L., & Field, D. (1991). Development of a clinical measure of postural control for assessment of adaptive seating in children with neuromotor disabilities. Physical Therapy, 71(12), 981–993. https://doi.org/10.1093/ptj/71.12.981
- Gimigliano, F. (2020). Are exercise interventions effective in patients with cerebral palsy? A Cochrane Review summary with commentary. Developmental Medicine and Child Neurology, 62(1), 18–20. https://doi.org/10.1111/dmcn.14400
- Hedin, H., Wong, C., & Sjödén, A. (2020). The effects of using an electrodress (Mollii®) to reduce spasticity and enhance functioning in children with cerebral palsy: A pilot study. European Journal of Physiotherapy, 1–10. https://doi.org/10.1080/21679169.2020.1807602
- Hoare, B. (2014). Rationale for using botulinum toxin A as an adjunct to upper limb rehabilitation in children with cerebral palsy. Journal of Child Neurology, 29(8), 1066–1076. https://doi.org/10.1177/0883073814533196
- Jarl, J., Alriksson-Schmidt, A., & Rodby-Bousquet, E. (2019). Health-related quality of life in adults with cerebral palsy living in Sweden and relation to demographic and disability-specific factors. Disability and Health Journal, 12(3), 460–466. https://doi.org/10.1016/j.dhjo.2019.02.002
- Kazdin, A. E. (2011). Single-case research designs: Methods for clinical and applied settings (2nd ed.). Oxford University Press.
- Mahmood, A., Veluswamy, S. K., Hombali, A., Mullick, A. N. M., Solomon, J. M., & Solomon, J. M. (2019). Effect of transcutaneous electrical nerve stimulation on spasticity in adults with stroke: A systematic review and meta-analysis. Archives of Physical Medicine and Rehabilitation, 100(4), 751–768. https://doi.org/10.1016/j.apmr.2018.10.016
- Mathiowetz, V., Volland, G., Kashman, N., & Weber, K. (1985). Adult norms for the Box and Block Test of manual dexterity. The American Journal of Occupational Therapy, 39(6), 386–391. https://doi.org/10.5014/ajot.39.6.386
- Moll, I., Vles, J. S. H., Dlhm, S., Witlox, A. M. A., Staal, H. M., Lawm, S., Janssen-Potten, Y. J. M., Coenen, M., Koudijs, S. M., & Vermeulen, R. J. (2017). Functional electrical stimulation of the ankle dorsiflexors during walking in spastic cerebral palsy: A systematic review. Developmental Medicine and Child Neurology, 59(12), 1230–1236. https://doi.org/10.1111/dmcn.13501
- Mooney, J. A., & Rose, J. (2019). A scoping review of neuromuscular electrical stimulation to improve gait in cerebral palsy: The arc of progress and future strategies. Frontiers in Neurology, 10, 887. https://doi.org/10.3389/fneur.2019.00887
- Mutlu, A., Livanelioglu, A., & Gunel, M. K. (2008). Reliability of Ashworth and Modified Ashworth Scales in children with spastic cerebral palsy. BMC Musculoskeletal Disorders, 9(1), 44. https://doi.org/10.1186/1471-2474-9-44
- Novak, I., Mcintyre, S., Morgan, C., Campbell, L., Dark, L., Morton, N., Stumbles, E., Wilson, S. A., & Goldsmith, S. (2013). A systematic review of interventions for children with cerebral palsy: State of the evidence. Developmental Medicine and Child Neurology, 55(10), 885–910. https://doi.org/10.1111/dmcn.12246
- Onghena, P. (2005). Single-Case Designs, B. S. Everitt & D. C. Howell Eds., Encyclopedia of Statistics in Behavioral Science. bsa. 625. John Wiley & Sons, Ltd
- Palisano, R. J., Rosenbaum, P., Bartlett, D., & Livingston, M. H. (2008). Content validity of the expanded and revised Gross Motor Function Classification System. Developmental Medicine and Child Neurology, 50(10), 744–750. https://doi.org/10.1111/j.1469-8749.2008.03089.x
- Palmcrantz, S., Pennati, G. V., Bergling, H., & Borg, J. (2020). Feasibility and potential effects of using the electro-dress Mollii on spasticity and functioning in chronic stroke. Journal of Neuroengineering and Rehabilitation, 17(1), 109. https://doi.org/10.1186/s12984-020-00740-z
- Pandyan, A. D., Johnson, G. R., Price, C. I. M., Curless, R. H., Barnes, M. P., & Rodgers, H. (1999). A review of the properties and limitations of the Ashworth and modified Ashworth Scales as measures of spasticity. Clinical Rehabilitation, 13(5), 373–383. https://doi.org/10.1191/026921599677595404
- Pennati, G. V., Bergling, H., Carment, L., Borg, J., Lindberg, P. G., & Palmcrantz, S. (2021). Effects of 60 min electrostimulation with the EXOPULSE Mollii suit on objective signs of spasticity. Frontiers in Neurology, 12, 706610. https://doi.org/10.3389/fneur.2021.706610
- Rosenbaum, P. N., Leviton, A., Goldstein, M., Bax, M., Damiano, D., Dan, B., & Jacobsson, B. (2007). A report: The definition and classification of cerebral palsy. Developmental Medicine and Child Neurology, 109, 8–14.
- Ryan, J. M., Cassidy, E. E., Noorduyn, S. G, O’Connell, N. E. 2017 . Exercise interventions for cerebral palsy. Cochrane Database of Systematic Reviews 11(6) doi:10.1002/14651858.CD011660.pub2 .
- S, I. L. (Ed.). (1994). Neurological rehabilitation (2nd ed.).
- Schuhfried, O., Crevenna, R., Fialka-Moser, V., & Paternostro-Sluga, T. (2012). Non-invasive neuromuscular electrical stimulation in patients with central nervous system lesions: An educational review. Journal of Rehabilitation Medicine, 44(2), 99–105. https://doi.org/10.2340/16501977-0941
- Takeda, K., Tanino, G., & Miyasaka, H. (2017). Review of devices used in neuromuscular electrical stimulation for stroke rehabilitation. <![cdata[medical Devices: Evidence and Research]]>, 24(10) , 207–213. https://doi.org/10.2147/MDER.S123464
- van den Noort Jc, Bar-On, L., Aertbeliën, E., Bonikowski, M., Braendvik, S. M., Broström, E. W., Buizer, A. I., Burridge, J. H., van Campenhout A, van den Noort, J. C., van Campenhout, A., Dan, B., Fleuren, J. F., Grunt, S., Heinen, F., Horemans, H. L., Jansen, C., Kranzl, A., Krautwurst, B. K., … Harlaar, J. (2017). European consensus on the concepts and measurement of the pathophysiological neuromuscular responses to passive muscle stretch. European Journal of Neurology, 24(7), 981–e38. https://doi.org/10.1111/ene.13322
- Westbom, L., Hagglund, G., & Nordmark, E. (2007). Cerebral palsy in a total population of 4–11 year olds in southern Sweden. Prevalence and distribution according to different CP classification systems. BMC Pediatrics, 7(1), 41. https://doi.org/10.1186/1471-2431-7-41
- Westbom, L., Rimstedt, A., & Nordmar, E. (2017). Assessments of pain in children and adolescents with cerebral palsy: A retrospective population-based registry study. Developmental Medicine and Child Neurology, 59(8), 858–863. https://doi.org/10.1111/dmcn.13459