Abstract
Photovoltaic energy-generating has attracted widespread attention, because of its efficiency and environmental benefits. As the number of buildings floors increases, the area of the façade grows substantially larger than the roof, which led to increasing the potential for a solar system installed on the vertical walls, although they receive less solar radiation than roof surfaces. As a result, it has become critical to assess the solar installation possibilities on buildings’ roofs as well as facades in the early design stage. Accordingly, the aim of this paper is to present a method to evaluate the suitable area on the building surfaces for photovoltaic installation, taking Apartment buildings in Amman, Jordan as a case study. The methodology is based on the assessment of the incident solar radiation on different surfaces, considering the shading effect from surrounding buildings in the most common residential urban zone in Jordan, and architectural suitable areas for PV installation. Different simulation software was used, Autodesk Ecotect simulation software was used to calculate the incident solar radiation on the building surfaces and IDA ICE 4.8 simulation software to predict the overshadowing area. The main findings of this study show that conducting a solar potential in the early design stage is critical to defining the most suitable surfaces for PV installation. Moreover, the highest potential envelope part of installing the solar photovoltaic technologies is the roof because it is unshaded and received the highest solar radiation, followed by the south façade with about 40% less received solar radiation. This study can contribute to supporting energy and urban planners in determining the best locations for solar photovoltaic installations on building surfaces.
PUBLIC INTEREST STATEMENT
Nowadays, photovoltaic energy-generating has attracted widespread attention, because of its efficiency and environmental benefits. As the number of building floors increases, the area of the façade grows substantially larger than the roof, which led to increasing the potential for a solar system installed on the vertical walls, although they receive less solar radiation than roof surfaces.
As a result, it has become critical to assess the solar installation possibilities on buildings’ roofs as well as facades in the early design stage. Accordingly, the aim of this paper is to present a method to evaluate the suitable area on the building surfaces for photovoltaic installation. The methodology is the use of a computer-simulations program. The main findings of this study show that conducting a solar potential in the early design stage is essential to defining the most suitable surfaces for PV installation. This study can contribute to supporting energy and urban planners in determining the best locations for solar photovoltaic installations on building surfaces.
1. Introduction
According to the International Energy Agency, the building industry consumes around 40% of global primary energy, with over half of this energy utilized for space heating, cooling, and hot water preparation. This energy is mostly produced by fossil fuels combustion (IEA, Citation2017), which contributes to CO2 emissions and has an impact on climate change (Koo et al., Citation2017). It is also expected that the global energy demand will rise in the future because of many factors such as population expansion, improved living standards, and increased demand for construction services (Fahad et al., Citation2019; Goudarzi et al., Citation2021).
Consequently, energy prices are rising and energy poverty is expanding (IEA, Citation2017; Prieto et al., Citation2017), and this highlights the importance of improving the energy efficiency of building’s envelope (Ruiz Valero et al., Citation2021). However, implementing energy-saving measures will not be enough to keep up with rising building energy consumption (Fernandes et al.,Citation2017). Consequently, ensuring a secure, inclusive, affordable, and sustainable supply from renewable energy sources is essential for future prosperity (Kariuki et al., Citation2020; Pinheiro, Citation2020; Qerimi et al., Citation2020;; Abu qadourah, Al-Falahat, Alrwashdeh, Citation2022; Ala’a M. Al-Falahat et al., Citation2022; Abu Qadourah, Al-Falahat, Alrwashdeh, Nytsch‐Geusen, Citation2022)
Among different energy sources, the most promising resource is solar energy, particularly in the Mediterranean region (Ala’a Al-Falahat et al., Citation2022), because of its high solar radiation intensity and a long period of sunshine, with more than 2,000 hours of sunlight every year, particularly along the southern and eastern shores (GTZ, Citation2009). The Global Horizontal Irradiance (GHI) ranges from 1,600 kWh/m2/year to 2,600 kWh/m2/year. While the possibility in the northern Mediterranean area is smaller, it is still quite fascinating, with a GHI ranging from roughly 1,000 kWh/m2/year to 1,600 kWh/m2/year, as depicted in .
Figure 1. Global horizontal Irradiance (GHI), world, Mediterranean area, and Jordan Maps. Source of pictures:(World bank group, Citation2019).
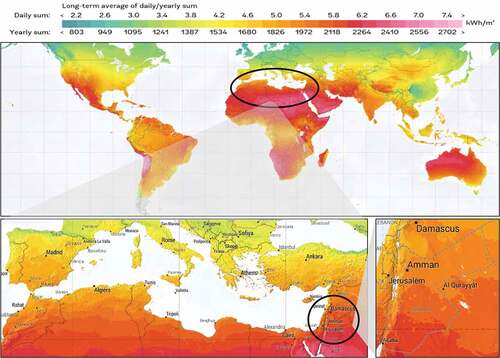
Jordan benefits from an abundance of solar energy due to its location in the “global sunbelt,” with average daily solar radiation ranging from 4 to 8 kWh/m2. This is equivalent to a total annual global horizontal radiation of 1,400–2,300 kWh/m2/year. The average length of sunshine about 300 days per year (Al-Addous et al., Citation2017). Because of these excellent conditions, solar technology development is particularly suited to the Mediterranean region. However, their contribution to the total supply of primary energy remains negligible (Attia & Al-Khuraissat, Citation2016; Krarti). In Jordan, solar energy technologies are not widely used except for solar water heaters, as it is a cost-effective technology, compared to other traditional water heating systems (Abdelhai, Citation2014; Al-Falahat, Abu Qadourah, etal., Citation2022; Alrwashdeh, Citation2022; AlShamaileh, Citation2010; Etier et al., Citation2010; Kiki et al., Citation2008; Sakhrieh & Al-Ghandoor, Citation2013). While photovoltaics is considered a relatively new technology that isn’t commonly used (Aberg et al., Citation2014; Knaack et al., Citation2014).
PV technologies are commonly mounted onto buildings’ roofs as pure technical elements. Generally, roofs have been determined to be effective for the installation of solar technologies (Jaugsch & Löwner, Citation2016; Alrwashdeh, Citation2018a, Citation2018b; CitationGoswami et al., Citation2021). Moreover, roofs are usually out of sight, especially in multi-story buildings, and by placing the PV on the roof, the designers tried to hide them to avoid negative effects on the building’s aesthetic. However, as urbanization grows, buildings with multiple stories and a small roof area have become the most common (Abdelhai, Citation2014; Aberg et al., Citation2014). Therefore, additional solar-accessible areas (such as external façades) are essential to provide the needed energy for building applications.
When PV technologies are incorporated into building facades, they function as multi-functional integral components (Buker & Riffat, Citation2015; Farkas et al., Citation2012; Probst & Roecker, Citation2011); it provides more than a technical energy purpose. Additionally, it contributes to the architectural and material fabric of the buildings (Hestnes, Citation1999; Probst et al., Citation2007). It may also affect the amount of heat transmitted through the building envelope. Consequently, the building’s energy demand (Lai & Hokoi, Citation2015). In addition, it can eliminate the need for lands to install the photovoltaic technologies and the additional support structure, which provides a further advantage of cost-saving (Basnet, Citation2012). The installation of PV technologies into the building’s facades poses a significant challenge to architects to accomplish a multifunctional role, as photovoltaic technology installation necessitates scientific, accurate, and numerical assessment. For example, to estimate the PV potential installation area on the building’s surfaces, different factors must be considered including photovoltaic module temperature, installation angle, orientation, shading, building form, aesthetic, incident solar radiation, etc. (Ádám Bognár et al., Citation2021; Azami & Sevinc, Citation2021; Kaan & Reijenga, Citation2004; Xiu et al., Citation2021).
Buildings facades, which are beneficial for solar energy production especially during the winter months (Desthieux et al., Citation2018; Jaugsch & Löwner, Citation2016), are becoming increasingly attractive as solar panel efficiency improves and innovative concepts such as Nearly Zero-Energy Buildings and Building Integrated Photovoltaics (BIPV) become available (Desthieux et al., Citation2018). However, when it comes to urban planning, solar radiation levels are rarely considered (Fernández-Ahumada et al., Citation2019).
It is critical for architects to take into account the level of solar irradiance on façades and roofs early in the design process (Brito et al., Citation2017; Fernández-Ahumada et al., Citation2019). Solar radiation levels can be used to determine which parts of a building are best suited for the installation of solar panels or thermal collectors in order to develop energy-saving measures in new constructions (Drif et al., Citation2008; Polo et al., Citation2018; Zhang & Wei, Citation2019). However, this analysis can be complicated in cities (Torres-Roldán et al., Citation2016), because of the many interactions that exist, including those with adjacent buildings (Salazar Trujillo & Jorge, Citation1998) and the requirement to study each neighborhood independently (Shao, Citation1990).
As a result, preliminary studies are necessary to ensure solar access and prevent the loss of solar availability in buildings (Sauchelli et al., Citation2013). Moreover, calculating the solar potential is becoming increasingly important for urban photovoltaic (PV) integration planning. Solar potential calculations in an urban area provide information about the solar potential of individual buildings. The solar potential of a building is determined by a variety of factors, including local irradiation, the solar system’s orientation and tilt, and the building’s operating conditions (Bouty et al., Citation2020).
In comparison to the roof, predicting the solar energy potential of the building’s facades is rare (Desthieux et al., Citation2018; Jaugsch & Löwner, Citation2016). Few studies have been conducted on how to optimize solar potential focusing on the PV positioning on building facades (Ádám Bognár et al., Citation2021; Garni et al., Citation2019; Redweik et al., Citation2013; Sun et al., Citation2021). According to Sun et al. installation of PV technologies into building facades requires careful planning to maximize renewable energy production while maintaining the aesthetic quality of the buildings in their urban context. Therefore, they developed a mapping tool to evaluate the viability of BIPV on building surfaces using visibility assessments and conventional energy yield estimations (Sun et al., Citation2021). Al Garni et al, argued that the ideal PV orientation boosts solar radiation by 4% when compared to fixed annual orientation (Garni et al., Citation2019). Although the irradiance reaching facades is smaller than that reaching roofs, when working with large areas, facades have a major effect on the solar potential received by buildings (Redweik et al., Citation2013).
A few simulation software are available to analyze the solar radiation distribution on the buildings surfaces in cities (Fernández-Ahumada et al., Citation2019). Benoit Beckers and Luc Masset developed software called Heliodon, with the help of this tool, the amount of sunlight reaches the buildings facades can be calculated. However, only the direct component of the solar radiation on clear sky days is considered in order to reduce computation time (Antaluca et al., Citation2010).
The main objective of this study is to assess the solar PV systems potential installation area on the building’s surfaces including the building roof and facades using different simulation software, taking the multi-family buildings in the typical neighborhood context of Amman, Jordan as a case study. To achieve the research objective, the study investigates the following main question: “Where in the building envelope should the solar PV technologies be installed?”. The study’s findings are likely to encourage energy scholars, urban planners, and architects to advocate for the architectural integration of PV systems into building envelope in urban context. In addition, since BIPV is becoming more widely accepted, the proposed methodology could be used as a standard tool for making early design decisions in urban areas, which would help further promote the use of BIPV.
In the next section, the main steps and methods used to answer the research question are presented.
In Section 3, the study results are presented including solar radiation analysis, solar exposure and overshadowing analysis, and architectural suitable area. Finally, the conclusion of this study is presented in section 4.
2. Methodology
The objective of this study is to estimate the solar potential of building in a typical neighborhood context in Jordan, taking into account the vertical surfaces. The assessment of the potential solar PV installation area into the buildings facades and roof was carried out in different steps, as illustrated in .
Figure 2. Study framework overview to assess the potential of solar energy installation into building envelope.
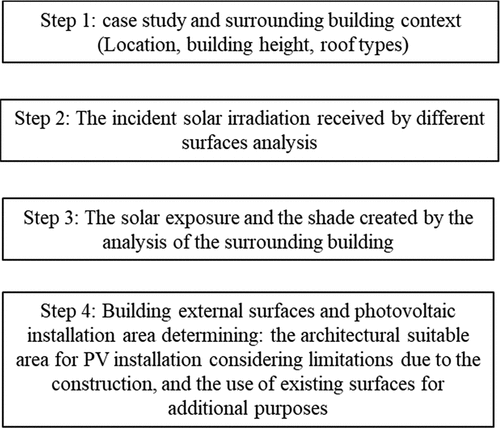
The first step is to define the case study and the surrounding building’s location, height, setbacks, etc. Second, is the accurate estimation of the incident solar irradiation received by the building in order to identify the solar potential of the facades and roof. Third, calculate the solar access and the shadow occlusion created by surrounding buildings, as the shadows from the surrounding buildings can have a negative effect on the amount of solar radiation reached on the PV system. Finally, the architectural suitable area for integration considering limitations due to the constructions, also the use of existing surfaces for additional purposes.
In this study, different simulations software has been utilised, the building model geometry within the typical urban context was created in “SketchUp”, which was then imported into Ecotect.
IDA ICE 4.8 software was used to predict the shaded area from adjacent buildings on the target building by considering the relevant information on location (i.e., latitude, altitude), orientation, and weather data. The incident solar radiation on the building surfaces and the solar exposure analysis was estimated using Ecotect software. Meteonorm software was used to allow the simulation software to make a realistic simulation of the surrounding environment for every hour of the year. “AMMAN JO” weather data file for Amman city was employed as input in all simulations.
This paper focuses on the integration of PV technologies into the multi-family building’s roof and facades, in the Mediterranean region, taking Amman the capital of Jordan as a case study. Amman accounts for 45% of Jordan’s new construction, while multi-family apartment buildings constitute approximately 75% of the overall housing stock and about 80% of all buildings (Younis, Citation2017). So basically, this study deals with the apartment buildings as a studying model. This makes sense for covering most of the construction and ensuring reliability. The typical studied multi-family buildings in Amman, Jordan are defined below.
2.1. Case study description
The studied multi-family building is located in the city of Amman in Jordan. The latitude is 31°95’ North and the longitude is 35°93’ East. The elevation is 788 m above sea level. All simulation models use the “AMMAN JO” weather data file provided by Meteonorm 7 software for Amman city. The studied apartment building represents the typical middle-class apartment building that is found in most Jordanian residential building blocks. The floor layout is rectangular, with 6 floors (including the basement floor), contains 10 residential apartments as each floor accommodates two facing apartments, so one flat has three exterior facades.
The studied multi-family building is considered to be in the most prevalent residential zone, classified as C class urbanized context which obliges 6 m side setback, and 8 m back setback (Awadallah & Awadallah, Citation2015; Johansson et al., Citation2009). In this zone, the maximum allowable height for all neighbouring structures is 15 meters, illustrates the building model within its urban context. However, in IDA ICE only the buildings next to the simulated case are considered, see, , due to the fact that the other building lacks a notable shadowing impact on the simulated building energy demand, accordingly, requires less time in the simulation process.
3. Results and discussion
In this section, results and discussion of study are presented. There is a high degree of consistency between the current findings and those of earlier studies on the solar potential installation area (Redweik et al., Citation2013; Brito et al., Citation2017; Á. Ádám Bognár et al., Citation2021; Middelhauve et al., Citation2021).
3.1. Solar radiation analysis
In this part, the solar radiation is estimated firstly without considering the shading effect from the surrounding buildings, the reason for this is to estimate its actual full potential for solar energy generating. Further analysis is done to estimate the solar radiation of the studied building envelope within a typical neighborhood context in Amman, Jordan.
illustrates the available annual solar radiation in kWh/m2/year for the tilted and vertical surfaces in different directions, and the percentage of annual radiation received by each surface related to the available global horizontal radiation (2,054 kWh/m2/year) in Amman, Jordan without considering the effect of the surrounding buildings. The south 30° tilted surface received higher annual solar radiation with 2,296 kWh/m2/year. It is also obvious that the vertical surfaces received lower solar radiation than the horizontal surface with about 33%, 40%, 43%, and 75% in the south, east, west, and north directions, respectively. Accordingly, the south-facing facades has a higher solar potential than other directions. However, there is a solar potential for east and west facades, especially when the south elevation is shaded from the adjacent buildings. The north direction façade receive very low solar potential, so it is excluded.
Figure 5. Influence of tilt and orientation on the percent of total solar radiation received annually in kWh/m2 in Amman, Jordan. Source of weather data: Meteonorm 7.
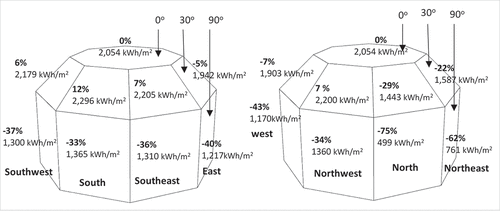
, illustrate the cumulative and hourly incident solar radiation on different surfaces for the studied building during different periods, including the whole year, cooling period, and heating period, considering the effect of surrounding buildings on the received solar radiation, for the location of Amman, Jordan.
Figure 6. Accumulative incident solar radiation through the whole year, and average daily incident radiation through heating and cooling periods, on studied multi-family building envelope.
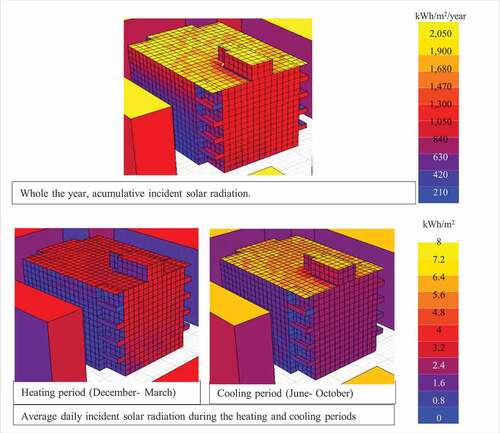
Figure 7. Hourly incident solar radiation received by the roof and south façade of the studied building. Source: Autodesk Ecotect analysis 2011 simulation software.
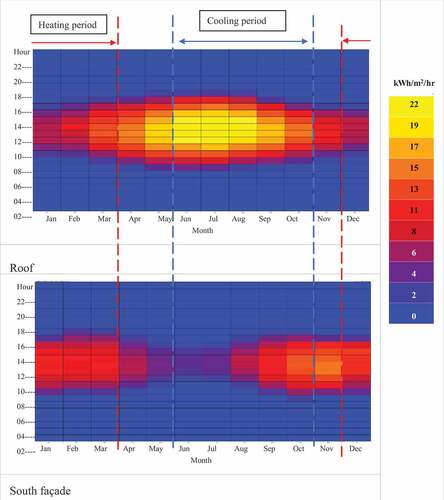
The highest amount of solar radiation is received by the roof with 2,050 kWh/m2/year which is also the case in scenes without shading scenario, while the roof area around the staircase received less amount of solar radiation varies between 1,050–1,680 kWh/m2/year, because it is shaded area. Moreover, the vertical south surfaces, receive approximately 1,300 kWh/m2/year, except the lower part of the facade with around 1,050 kWh/m2/year as it is the most shaded area, which means a reduction of 22% in solar radiation potential. While the east and west facades received the lowest amount of solar ranging from around 840 kWh/m2/year on the upper part to 210 kWh/m2/year on the lower part, this means that the solar potential reduced by 30% in the upper part and 80% in the lower part because of the obstruction.
In the cooling period, the roof receives more than 1,060 kWh/m2 /month as an average, and the peak hours are at noon. During the same period, the south wall does not receive much by comparison, due to the high sun position. Despite this, the south wall in the heating period can receive around 840 kWh/m2. This difference between the roof and the south wall is obvious in . However, the south-facing facade had great potential and is considered the best facade to install the PV panels because it received solar radiation nearly all day.
In general, after analyzing the solar incident solar radiation received by the building façade and roof, it was found that the roof is the best surface to install the PV panels as it receives the highest solar radiation. Moreover, out of all façades, only the south façade is considered suitable for solar PV integration.
3.2. Solar exposure and overshadowing analysis
below, illustrates the solar exposure percentage of the roof and south façade, as these are the most envelope surfaces that have the potential for solar PV installation. It is obvious that during the mid-day throughout all year the sun is quite high, and the roof and south facade has no shading from the surrounding buildings. However, in the early morning and late afternoon, for a few hours, around 10% (ground floor) to 40% (ground and first floor) of the south facade is shaded. Also, part of the roof is shaded, especially during summer periods, which is resulting from the building’s projections (elevator and staircase).
Figure 8. Hourly solar exposure analysis in percentage received by the roof and south façade of the studied building.
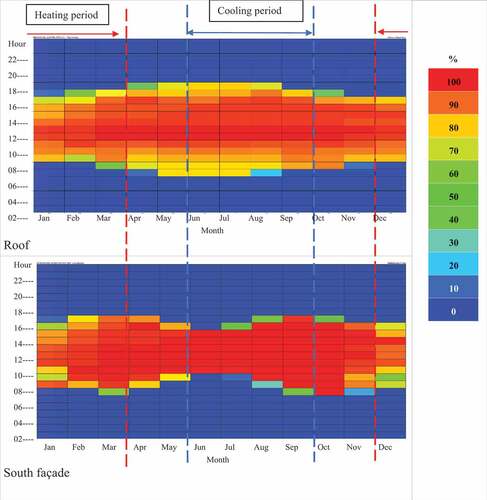
Shadow analysis is also done with IDA ICE, illustrates the shadow on the studied building during the different times for three days of the year: winter solstice (21st December), spring equinox (21st March), and summer solstice (21st June). It is clear that part of the south façade is shaded during the summer period.
Figure 9. Shadow analysis on the studied apartment building in the typical urban context in Amman, Jordan during the different times for three days of the year: winter solstice (21st December), spring equinox (21st March), and summer solstice (21st June). Shadow simulation results are obtained from IDA ICE 4.8 simulation software.
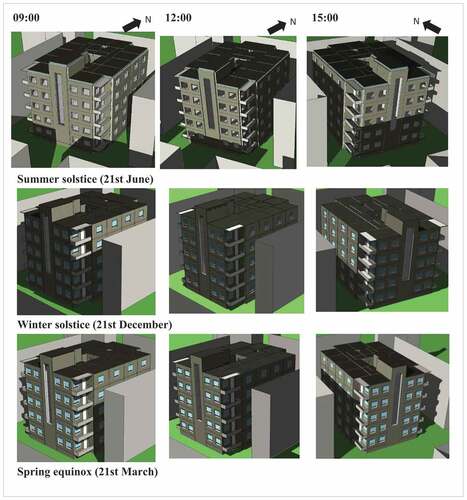
3.3. Architectural suitable area
Based on the above discussion the most solar suitable surface area for PV installation is the roof, followed by the south façade, as they received the highest amount of solar radiation. The total roof area is about 300 m2, however, it is assumed that part of the roof area is used for the building services installation such as water tanks, dishes, etc. The assumed area on the roof used to install the building services is near the staircase to avoid the shade into the PV modules. Accordingly, the total available area for solar PV installation above the roof is about 200 m2, and 25 m2 above the staircase roof.
Regarding the south elevation, the vertical total available south façade area, including wall and windows is about 240 m2. As mentioned before, the lower part of the south façade (ground floor) is the most shaded part, which is around 50 m2 façade area, therefore, the PV installation in this part of façade must be avoided. Accordingly, the available architectural suitable area for solar PV installation on the south façade is about 190 m2, including 57 m2 transparent parts (window and staircase glazing), as illustrated in .
Figure 10. Architectural suitable surfaces area for solar PV installations into multifamily buildings in typical urban context in Amman, Jordan.
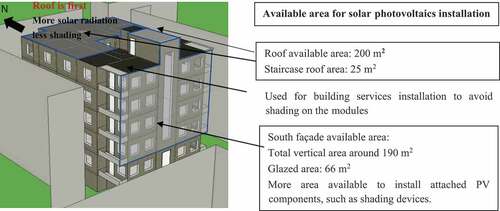
Another possibility for the PV installation into the façade is attached elements such as an overhang shading device, which increased the potentially available area for solar PV installation. This will be considered more in the future studies considering also different tilts angles.
According to the above analysis, for the studied case the roof has the greatest solar potential for PV technology installation. Far behind comes the south wall with a 40% less received solar radiation and more shaded area.
4. Conclusion
In this study, the potential envelope surfaces for the solar photovoltaic installation in the typical neighborhood context of Amman, Jordan was investigated. This was done by determining the suitable surface areas for the PV installation considering the incident solar radiation on different surfaces, shading analysis as well as the architectural suitable area for integration considering limitations from the constructions, also the use of the existing roof for other purposes. The study was performed by using different simulation software.
Generally, the outcomes confirmed that the highest potential envelope part of installing the solar systems is the roof, as most of its area is unshaded and received the highest solar radiation with about 2,000 kWh/m2/year. For the façades, the south facing façade has higher potential for installing PV panels than facades with other orientation. The south façade received about 40% (1,300 kWh/m2/year) less received solar radiation and more shaded area compared to the roof, especially in the lower part of the facade. However, it is also noticed that without considering the effect of the surrounding building on the incident solar radiation, east and west facades were not that far behind the south façade in their solar potentials, with 7% (1,210 kWh/m2/year) and 10% (1,170 kWh/m2/year) less received solar radiation for east and west facades, respectively. This means under different neighborhood contexts where the east and west are not obstructed from the surrounding building, installation for active solar technology on these façades can be beneficial. Or when the south façade is shaded, together east and west façade can be used for solar PV installation.
The most common urban zone was considered in this study, however, the urban layout’s characteristics, such as the width of the streets with different directions, buildings height, setbacks, etc., could have a significant impact on the building envelope’s solar adaptability. Therefore, future work should include more neighborhoods context. Moreover, only the potential of installing PV on building facades and roof was analyzed without estimate the electricity production of the PV system which can be also estimated in the future studies, considering different technologies, tilts and azimuth angles, etc.
The methodology approach presented in this study could be used in different urban context, in order to provide guidelines for architects, engineers, city planners to make informed decisions in the early design phases of a project to assist technical decisions and limit the impact of urban shadowing on solar potential. Moreover, to optimize PV panel installation decisions in existing urban areas.
Disclosure statement
No potential conflict of interest was reported by the author(s).
Additional information
Funding
Notes on contributors
Jenan Abu Qadourah
Jenan Abu Qadourah is an assistant professor in the Architecture Engineering Department at the University of Mutah, Jordan. Her research interest includes but is not limited to low-carbon and renewable technologies, energy efficiency, thermal comfort, and environmentally sustainable design for the built environment.
Ala’a M. AL-Falahat
Ala’a M. AL-Falahat is an assistant professor in the Mechanical Engineering Department at Mutah University, his research interest includes rotordynamics, automatic control, and mechanical vibration.
Saad S. Alrwashdeh
Saad S. Alrwashdeh is an associate professor at Mutah University, his research interest includes energy, nuclear energy, imaging techniques, renewable energy, thermal systems, mechanical power system analysis, mechanical machine, protection systems, transmission, and distribution system, power, and control systems and electrical installation.
References
- Abdelhai, A. (2014). Solar water heating market transformation and strengthening initiative (GSWH project). Jordan: United Nations Development Programme (UNDP) - International Copper Association (ICA).
- Aberg, E., Alison, M., Masuda, S., Zhao, C., Mysalieva, N., & Barghouth, A. (2014) (Regional Center for Renewable Energy and Energy Efficiency (RCREEE)). Up-scaling solar PV for self-consumption in the Jordanian market. To the future, 72.
- Abu Qadourah, J. , Al-Falahat, A., Alrwashdeh, S. S., Nytsch‐Geusen, C., & . (2022). Improving the energy performance of the typical multi-family buildings in Amman, Jordan. City, Territory and Architecture, 9(1), 6. https://doi.org/10.1186/s40410-022-00151-8
- Al-Addous, M., Dalala, Z., Class, C., Alawneh, F., & Al-Taani, H. Performance analysis of off-grid PV systems in the Jordan Valley. Renewable Energy, 113 1 ,930–15. (June 1, 2017). https://doi.org/10.1016/j.renene.2017.06.034
- Al-Falahat, A., Qadourah, J., & Alrwashdeh, S. (2022). Economic feasibility of heating source conversion of the swimming pools. Journal of Applied Engineering Science, 20(1), 230–238. https://doi.org/10.5937/jaes0-34474
- Al-Falahat, A. M., Abu Qadourah, J., Alrwashdeh, S. S., Khater, R., Qatlama, Z., Alddibs, E., & Noor, M. (2022). Energy performance and economics assessments of a photovoltaic-heat pump system. Results in Engineering, 13(December), 100324. https://doi.org/10.1016/j.rineng.2021.100324
- Alrwashdeh, S. S. (2018a). Assessment of the energy production from PV racks based on using different solar canopy form factors in Amman-Jordan. International Journal of Engineering Research and Technology, 11(10), 1595–1603 https://doi.org/10.20508/ijrer.v8i2.7337.g7368.
- Alrwashdeh, S. S. (2018b). Investigation of the energy output from pv racks based on using different tracking systems in Amman-Jordan. Int. J. Mech. Eng. Technol. International Journal of Mechanical Engineering and Technology, 9(10), 687–694.
- Alrwashdeh, S. S. (2021). Investigation of the energy output from pv panels based on using different orientation systems in Amman-Jordan. CSITE Case Studies in Thermal Engineering, 28 3 , 101580 doi:10.1016/j.csite.2021.101580
- Alrwashdeh, S. S. (2022). Energy sources assessment in Jordan. Results in Engineering, 13 3 , 100329 https://doi.org/10.1016/j.rineng.2021.100329.
- AlShamaileh, E. (2010). Testing of a new solar coating for solar water heating applications. Solar Energy, 84(9), 1637–1643. https://doi.org/10.1016/j.solener.2010.06.003
- Antaluca, E., Merino, L., & Beckers, B. Correlation between measured and calculated solar radiation data in Compiègne, France. WSEAS Transactions on Environment Anddevelopment, 6(6), 478–487 doi:10.1016/j.rineng.2021.100329. ((June 1, 2010)).
- Attia, S., & Al-Khuraissat, M. (2016). Life cycle costing for a near zero energy building in Jordan: initial study.” In The 5th Architectural Jordanian International Conference, http://www.jeaconf.org/UploadedFiles/Document/0695e0f8-6a90-47c5-b5b0-07f86dca48c8.pdf
- Awadallah, T., & Awadallah, T. (2015). Role of street-level outdoor thermal comfort in minimizing urban heat island effect by using simulation program, Envi-Met: Case of Amman, Jordan. Research Journal of Environmental and Earth Sciences, 7(3), 42–49. https://doi.org/10.19026/rjees.7.1694
- Azami, A., & Sevinc, H. The energy performance of building integrated photovoltaics (BIPV) by determination of optimal building envelope. Building and Environment, 199 4 ,107856. ((April 1, 2021)). https://doi.org/10.1016/j.buildenv.2021.107856
- Basnet, A. (2012). Sustainable architecture: architectural integration of photovoltaic and solar thermal collector systems into buildings. LAP LAMBERT Academic Publishing.
- Bognár, Á., Roel Loonen, and Jan Hensen. Calculating solar irradiance without shading geometry: A point cloud-based method. Journal of Building Performance Simulation, 14(5), 480–502. (September 3, 2021). https://doi.org/10.1080/19401493.2021.1971765
- Bouty, K., Gaillard, L., Thebault, M., Lokhat, I., Gallice, A., & Ménézo, C. (2020). Solar cadaster in urban area including verticality. Proceedings of the ISES Solar World Congress 2019 and IEA SHC International Conference on Solar Heating and Cooling for Buildings and Industry 2019, 2179–2188. https://doi.org/10.18086/swc.2019.45.01.
- Brito, M. C., Freitas, S., Guimarães, S., Catita, C., & Redweik, P. (2017). The Importance of Facades for the Solar PV Potential of a Mediterranean City Using LiDAR Data. Renewable Energy, 111 10 , 85–94. https://doi.org/10.1016/j.renene.2017.03.085
- Buker, M. S., & Riffat, S. B. (2015). Building integrated solar thermal collectors – A review. Renewable and Sustainable Energy Reviews, 51 11 , 327–346. https://doi.org/10.1016/j.rser.2015.06.009
- Desthieux, G., Carneiro, C., Camponovo, R., Ineichen, P., Morello, E., Boulmier, A., Abdennadher, N., Dervey, S., & Ellert, C. (2018). Solar energy potential assessment on rooftops and facades in large built environments based on lidar data, image processing, and cloud computing. methodological background, application, and validation in Geneva (Solar cadaster). Frontiers in Built Environment, 4(March), 14. https://doi.org/10.3389/fbuil.2018.00014
- Drif, M., Perez-Higueras, P., Aguilera, J., & Aguilar, J. (2008). A new estimation method of irradiance on a partially shaded PV generator in grid-connected photovoltaic systems. Renewable Energy, 33(9), 2048–2056. https://doi.org/10.1016/j.renene.2007.12.010
- Etier, I., Anas, A., & Ababne, M. (2010). Analysis of solar radiation in Jordan. Jordan Journal of Mechanical and Industrial Engineering, 4(6), 733–738.
- Fahad, S., Ullah, N., Mahdi, A., Ibeas, A., & Goudarzi, A. (2019). An advanced two-stage grid connected PV system: A fractional-order controller. International Journal of Renewable Energy Research, 9(1), 504–514 https://www.ijrer.org/ijrer/index.php/ijrer/article/view/9031.
- Farkas, K., Andresen, I., & Grete Hestnes, A. (2012). Architectural integration of photovoltaic cells overview of materials and products from an architectural point of view. 3rd International Conference on Smart and Sustainable Built Environments (SASBE 2009). Delft (The reserch center on zero emission buildings).
- Fernandes, J., Santos, M., & Castro, R. (2021). Introductory review of energy efficiency in buildings retrofits. Energies, 14(23), 8100. https://doi.org/10.3390/en14238100
- Fernández-Ahumada, L. M., Ramírez-Faz, J., López-Luque, R., Márquez-García, A., & Varo-Martínez, M. (2019). A methodology for buildings access to solar radiation in sustainable cities. Sustainability (Switzerland), 11(23), 5–7. https://doi.org/10.3390/su11236596
- Garni, H. Z. A., Wright, A., & Al Garni, D. (2019). Optimal orientation angles for maximizing energy yield for solar PV in Saudi Arabia. Renew. Energy Renewable Energy, 133 4 , 538–550. https://doi.org/10.1016/j.renene.2018.10.048
- Goswami, A., Sadhu, P., & Sadhu, P 2012 . Development of a grid connected solar-wind hybrid system with reduction in levelized tariff for a remote Island in India. Journal of Solar Energy Engineering, 142(4), 1–15. https://doi.org/10.1115/1.4046147
- Goudarzi, A., Yanjun, L., & Xiang, J. (2021). Efficient energy management of renewable resources in microgrids Yang, Qiang. In Renewable energy microgeneration systems (pp. 285–321). Academic Press.
- GTZ. (2009). Solar thermal application in Egypt, Jordan, Lebanon, Palestinian territories, Syria and Tunisia: technical aspects, framework conditions and private sector needs. Solaterm
- Hestnes, A. G. (1999). Building integration of solar energy systems. Solar Energy, 67(4–6), 181–187. https://doi.org/10.1016/S0038-092X(00)00065-7
- IEA 2017. Energy access outlook 2017: From poverty to prosperity. International Energy Agency, 94 3 ,2017. https://doi.org/10.1787/9789264285569-en
- Jaugsch, F., & Löwner, M. O. (2016). Estimation of solar energy on vertical 3d building walls on city quarter scale. International Archives of the Photogrammetry, Remote Sensing and Spatial Information Sciences - ISPRS Archives, 42(2W2), 135–143. https://doi.org/10.5194/isprs-archives-XLII-2-W2-135-2016
- Johansson, E., Ouahrani, D., Shaker Al-Asir, H., Awadallah, T., Blomsterberg, Å., Håkansson, H., Hellström, B., & Kvist, H. (2009). Johansson, E. Climate Conscious Architecture and Urban Design in Jordan - Towards energy efficient buildings and improved urban microclimate, 12 (Housing Development & Management,)978-91-87866-33-3 .
- Kaan, H., & Reijenga, T. (2004). Photovoltaics in an architectural context. Progress in Photovoltaics: Research and Applications, 12(6), 395–408. https://doi.org/10.1002/pip.554
- Kariuki, S., Murage, D., Musau, P., & Saulo, M 2020. Comparative analysis of implementation of solar PV systems using the advanced SPECA modelling tool and HOMER software: Kenyan scenario. HighTech and Innovation Journal, 1(1), 8–20. https://doi.org/10.28991/HIJ-2020-01-01-02
- Kiki, P., Maria, K., Lampropoulou, L., & Kyritsis, A. (2008). Integration of solar technologies into buildings in Mediterranean communities electronic guide CRES. Pikermi.
- Knaack, J. M., Al Ghandoor, A., Grundner, C., & Najdawi, C. (2014). Enabling PV in the MENA region, the emerging PV market in Jordan. Deutsche Gesellschaft für Internationale Zusammenarbeit (GIZ) GmbH.
- Koo, C., Hong, T., Jeong, K., Ban, C., & Jeongyoon, O. (2017). Development of the smart photovoltaic system blind and its impact on net-zero energy solar buildings using technical-economic-political analyses. In Energy (Vol. 124 4 pp. 382–396 https://doi.org/10.1016/j.energy.2017.02.088). https://doi.org/10.1016/j.energy.2017.02.088
- Krarti, Moncef 2019 . Evaluation of energy efficiency potential for the building sector in the Arab region. Energies, 12(22), 4279. https://doi.org/10.3390/en12224279
- Lai, C.-M., & Hokoi, S. (2015). Solar façades: A review. Building and Environment, 91 12 , 152–165. https://doi.org/10.1016/j.buildenv.2015.01.007
- Middelhauve, L., Girardin, L., Baldi, F., & Maréchal, F. 2021. Potential of photovoltaic panels on building envelopes for decentralized district energy systems. Frontiers in Energy Research, 9 10 . (October 15, 2021). https://doi.org/10.3389/fenrg.2021.689781
- Pinheiro, A 2020. Architectural rehabilitation and sustainability of green buildings in historic preservation. HighTech and Innovation Journal, 1(4), 172–178. https://doi.org/10.28991/HIJ-2020-01-04-04
- Polo, M.-E., Pozo, M., & Quirós Rosado, E. (2018). Directional statistics in solar potential of rooftops at three different neighborhoods of a medium size city. Proceedings. Vol. 2, https://doi.org/10.3390/proceedings2201275
- Prieto, A., Knaack, U., Auer, T., & Klein, T. (2017). Solar coolfacades: Framework for the integration of solar cooling technologies in the building envelope. Energy, 137 4 , 353–368. https://doi.org/10.1016/j.energy.2017.04.141
- Probst, M., MariaCristina, & Roecker, C. (2007). Towards an improved architectural quality of building integrated solar thermal systems (BIST). Solar Energy, 81(9), 1104–1116. https://doi.org/10.1016/j.solener.2007.02.009
- Probst, M. C. M., & Roecker, C. (2011). Architectural integration and design of solar thermal systems. Routledge Taylor and Francis Group.
- Qerimi, D., Dimitrieska, C., Vasilevska, S., & Alimehaj, A 2020. Modeling of the solar thermal energy use in urban areas. Civil Engineering Journal, 6(7), 1349–1367. (). https://doi.org/10.28991/cej-2020-03091553
- Redweik, P., Catita, C., & Brito, M. (2013). Solar energy potential on roofs and facades in an urban landscape. Solar Energy, 97 11 , 332–341. https://doi.org/10.1016/j.solener.2013.08.036
- Sakhrieh, A., & Al-Ghandoor, A. (2013). Experimental investigation of the performance of five types of solar collectors. Energy Conversion and Management, 65 1 , 715–720. https://doi.org/10.1016/j.enconman.2011.12.038
- Sauchelli, M., Lobaccaro, G., Masera, G., & Fiorito, F. (2013). Smart solutions for solar adaptive facades. preliminary studies for an innovative shading device. IAHS World Congress on Housing. Milan, Italy (IAHS), https://doi.org/10.13140/RG.2.1.1953.2647
- Shao, J 1990. Calculation of sunshine duration and saving of land use in urban building design. Energy and Buildings - ENERG BLDG, 15(3–4), 407–415. https://doi.org/10.1016/0378-7788(90)90015-B
- Sun, H., Kiang Heng, C., Tay, S., Chen, T., & Reindl, T 2021 . Comprehensive feasibility assessment of building integrated photovoltaics (BIPV) on building surfaces in high-density urban environments. Solar Energy, 225 9 ,734–746. (September 1, 2021). https://doi.org/10.1016/j.solener.2021.07.060
- Torres-Roldán, M., López-Luque, R., & Varo-Martínez, M 2016 . Assessment of the pointing error of heliostats with a single not polar rotation axis for urban applications. Solar Energy, 137 11 ,281–289. ((November 1, 2016)). https://doi.org/10.1016/j.solener.2016.08.013
- Trujillo, S., & Jorge 1998 . Solar performance and shadow behaviour in buildings. case study with computer modelling of a building in loranca, Spain. Building and Environment, 33(2–3), 117–130. https://doi.org/10.1016/S0360-1323(97)00027-9
- Valero, R., Letzai, J. F.-G., Ferreira, J., González, V., Guerrero, N., & Ramírez-Rivera, F. A 2021 . thermal performance of facades based on experimental monitoring of outdoor test cells in tropical climate. Civil Engineering Journal, 7(12), 1982–1997. https://doi.org/10.28991/cej-2021-03091773
- World bank group. Global horizontal irradiation. Global solar atlas, 2019. https://globalsolaratlas.info/downloads/world
- Xiu, L., Guannan, L., Wang, A., Xiong, Q., Lin, B., & Guonian, L. (2021). Estimating the photovoltaic potential of building facades and roofs using the industry foundation classes. ISPRS International Journal of Geo-Information, 10(12), 827. https://doi.org/10.3390/ijgi10120827
- Younis, A. Towards resilient low-middle income apartments in Amman, Jordan: a thermal performance investigation of heating load. CIBSE ASHRAE Technical Symposium. Loughborough, UK (DE MONTFORT), 2017.
- Zhang, & Wei 2019. A hybrid model based on principal component analysis, wavelet transform, and extreme learning machine optimized by bat algorithm for daily solar radiation forecasting. Sustainability, 11(15), 4138. https://doi.org/10.3390/su11154138