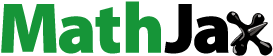
Abstract
The dimension of millimeter wave massive multiple input multiple output (mmWave mMIMO) and required number of radio frequency (RF) chains can be reduced without performance loss by Beamspace (BS) system using various beam selection algorithms. Nonetheless, beam selection requires precise information of BS channel and the number of supported users cannot be greater than number of RF chains. In this work, we analyze the performance of beamspace non-orthogonal multiple accesses (BS-NOMA) with estimating BS channel. Pilot-based channel estimation technique with a compressive sensing (CS) tool, few sample and low pilot overhead is used to estimate the BS channel by practicing orthogonal matching pursuit (OMP) algorithm. And also to support larger number of users than number of RF chains in the estimated BS channel, non-orthogonal multiple accesses (NOMA) is integrated with BS multi-input-multi-output (BS-MIMO) system. Minimum mean square error (MMSE) and maximum ratio transmission (MRT) and zero-forcing (ZF) precoders are implemented to improve the performance of BSNOMA with estimated BS channel and to mitigate inter-beam interference. Additionally, dynamic power allocation is applied to solve joint power optimization problem and to further maximize achievable sum rate of BS-NOMA with estimated BS channel. Results of this research work show that under the same BS channel estimation, spectral and energy efficiency performance of BS-NOMA is better than BS-MIMO system.
PUBLIC INTEREST STATEMENT
Millimetre wave communication has elevated increasing attentions from both academia and industry because of its outstanding advantage. It has been considered as a best candidate for 5 G and beyond 5 G communication systems to host very high data rate communication. However, a number of issues have to be addressed to make this communication more feasible. In this work, beam selection and channel estimation are considered to study the performance of beamspace based Non Orthogonal Multiple access in collaboration with lens antennas for millimetre wave communication. By doing this the performances (both energy and spectral efficiency) of millimetre wave communication systems are enhanced.
1. Introduction
The combination of millimeter wave (mmWave) technology with massive multiple input multiple output (mMIMO) can achieve higher orders of magnitude increase in system capacity with enhanced energy and spectral efficiencies, increased reliability, compactness and flexibility. However, unaffordable hardware cost and energy consumption caused by numerous RF chains as the number of antennas becomes very large is challenges to realize mmWave mMIMO in practice. Using antenna selection, many studies aim to reduce the hardware complexity of very high-dimensional MIMO system in order to exploit the favourable characteristics of mmWave frequency communications. But there is a high performance degradation compared to the full system (Busari et al., Citation2017). On the other hand in Ramanujam, Arumugam et al. (Citation2020), there is an enhancement of gain and bandwidth for 5 G mm-wave application by designing super compact parasitic patch loaded radiating system and four-element modified corporate feed array configuration. In Ramanujam, Arumugam et al. (Citation2020), the antenna exhibits good voltage standing wave ratio (VSWR), high gain and constant radiation efficiency over a wide range of frequencies but not give concern about signal processing and hardware complexity reduction using advanced antennas. In Ramanujam et al. (Citation2021), the authors design compact size and low complex Antipodal Vivaldi Antenna array with 1-to-8 wideband feeding network with enhanced gain, reduced mutual coupling and wide impedance bandwidth of 22.8–31.3 GHz is achieved for future 5 G mm-wave communication. In Pachaiyappan & Ramanujam (Citation2021), a coaxial feed, moon shaped parasitic patch loaded two layers stacked patch antenna is proposed to achieve wide bandwidth, high gain and improved radiation efficiency on the other hand in Ramanujam, Venkatesan et al. (Citation2020) also an electromagnetic interface is suppressed in stacked system by incorporating the circular complementary split ring resonator (CCSRR) in the ground plane and its application for radar target scanning, narrow band channel selector and low power narrow band wireless receivers. Due to orders-of-magnitude, larger bandwidth and smaller wavelength (10 –1 mm) at mmWave frequency can allow more antennas in a similar physical space however the authors in Ramanujam, Arumugam et al. (Citation2020),3,4; Ramanujam, Venkatesan et al. (Citation2020) design different types of antenna for different task with limited number of antennas but not deal about signal processing and hardware complexity in mmWave communication system. To lower transceiver complexity and energy consumption of RF chain in mmWave mMIMO utilizing lens antenna array in BS-MIMO have a vital role. A lens antenna array is an electromagnetic lens with feed antennas located on the focal surface of the lens, which allows concentrating the signal arriving from different directions on different antennas. The frequency range of usage of lens antenna starts at 1000 MHZ but its use is greater at 3000 MHZ and above. On the other hand, when it is compared with other antennas it has greater design tolerance, large amount of wave and its beam can be moved angularly with respect to the axis. Lens antenna used as a wide band antenna both for mmWave and microwave frequency application and that is why this research work considered this antenna array. Lens antenna array is also used to approximately transform the antenna space signal to beamspace (BS), which has much lower dimension to significantly reduce the hardware complexity. Hence, the spatial model of the channel can be seen as a BS model. As the result of the BS channel has sparse characteristics: able to select a few dominant beams based on the beam selection algorithm to essentially reduce the dimension of MIMO system and required number of radio frequency (RF) chains without performance loss. The key challenging problems in BS-MIMO system are the accurate channel state information (CSI) must be obtained by the base station before beam selection and also number of served users cannot be larger than the required number of RF chains. In this work, we study and analyse the performance of BS-NOMA adopted in mmWave frequencies with estimated BS channel. To estimate the BS channel we consider pilot-based channel estimation technique with compressive sensing (CS) tool-based orthogonal matching pursuit (OMP) algorithm which is computationally proficient and estimate users channel in iterative way with few samples and low pilot overhead. After, estimating the BS channel to select the power dominate beams from the estimated BS channel and to serve larger number of users than required number of RF chain in the system we integrate BS-MIMO with power domain NOMA. The remaining portion of this work is organized as follows. In Section 2, review of related work is stated. In Section 3, system and channel model of BS-non orthogonal multiple accesses (NOMA) with estimated BS channel present. Mathematical and theoretical description of BS-NOMA with estimated BS channel, linear precoding techniques and dynamic power allocation present in Section 4. Simulation results and discussion are in Section 5 and conclusions are drawn in Section 6.
2. Related works
To offer near-optimal performances with a low hardware transceiver complexity, Amadori et al. (Citation2015); Gao et al. (Citation2016) proposed beam selection techniques in BS-MIMO system. The authors in Amadori et al. (Citation2015) evaluated the performance of beam selection techniques maximum magnitude (MM-S), maximization of the SINR selection (MS-S) and maximization of the capacity selection (MC-S) according to capacity computations, comparisons of number of RF chains and trade-off between spectral and power efficiency metrics. The MM-S has lower complexity than other techniques and MS-S achieved better performance for both spectral efficiency and required number of RF chain than others. The authors in Gao et al. (Citation2016) proposed modified near-optimal beam selection technique for BS mmWave mMIMO systems in single cell setup. In this paper an interference-Aware (IA) beam selection introduced in BS-MIMO system to address the basic challenges hold in conventional beam selection techniques: sufferance of serious multiuser interference and wastage of RF chain. IA beam selection guaranteed that all users can be served simultaneously with near-optimal sum rate performance and more energy efficient than other beam selection schemes. The main limitation of this work is each selected beams can serve only single user. The authors in Xie et al. (Citation2017) proposed power leakage problem in BS-MIMO system with lens antenna array in a perfect CSI. Using phase shifter network (PSN)-based precoding structure which employing multiple phase shifters for each RF chain is used to select multiple beams. Based on the precoding structure authors designed rotation based precoding algorithm to maximize signal to noise ratio (SNR) of each user in the system by rotating the channel gain of selected beams to the same direction. PSN-based precoding structure can efficiently solve power leakage problem in BS-MIMO system to achieve near-optimal sum rate and high energy efficiency than conventional precoding techniques. In Liu et al. (Citation2019); Wang et al. (Citation2017), BS-MIMO-NOMA proposed for mmWave communication using lens antenna arrays in perfect CSI. The authors disrupted the limit of BS-MIMO, which is number of users cannot exceed the number of RF chain. But by integrating power domain NOMA with BS-MIMO, they created an opportunity to serve more number of users than number of RF chains. The authors in Wang et al. (Citation2017) applied dynamic power allocation to solve joint power optimization by considering both intra & inter beam power. In addition to this to mitigate the existing inter-beam interference they implemented zero forcing (ZF) precoding. They showed as BS-MIMO-NOMA significantly reduces the dimension of MIMO system and also the required number of RF chains in the system. At the consequence of this their spectral and energy efficiency, it has better than BS-MIMO system. In Liu et al. (Citation2019), the authors considered a cluster based BS-NOMA and energy efficient power allocation problem formulation based on beam selection method as a non-convex fractional programming. By using sequential convex approximation (SCA) and second-order cone (SOC) transformation, the original non-convex fractional optimization problem is transformed into a convex optimization problem, which is solved through iterative optimization algorithm.
In Venugopal et al. (Citation2017), the authors considered CS-based solutions for a sparse wideband mmWave channel estimation problem in hybrid analog and digital precoding architecture. In this work, purely time, purely frequency and combined time frequency domains were considered in both single carrier frequency equalization and OFDM-based wideband mmWave systems. Authors showed that the algorithms required very few training frames to ensure low estimation error but for further reduction of training overhead and estimation error they employed multiple RF chains at the transceivers. The authors in Gao et al. (Citation2017) put forward reliable BS channel estimation for time division duplex mmWave mMIMO systems with lens antenna array. They Proposed support detection (SD)-based channel estimation scheme which can detect the supports of channel components by utilizing structural characteristics of mmWave BS channel and also the BS channel remains unchanged within such channel coherence time. The proposed channel estimation algorithm can estimate the BS channel in reliably way but still number of served users are equivalent to number of selected power dominate beams. X. Gao et al. (Gao et al., Citation2019) proposed a wideband BS channel estimation problem without common support assumption for mmWave MIMO systems relying on lens antenna arrays in which the widely used orthogonal pilot transmission strategy is adopted. The authors proved that each path component of the wideband BS channel exhibits a unique frequency-dependent sparse structure. In addition, successive support detection (SSD)-based BS channel estimation scheme that successively estimates each sparse path components were considered. As one can see the related works, most of the studies considered BS-MIMO system in perfect BS channel or estimating BS channel while the rest considered perfect BS channel for BS-NOMA. In this work we analyse the performance of BS-NOMA by estimating the BS channel in mmWave mMIMO system and its performance is analysed based on spectral and energy efficiency.
3. System and channel model
We consider time division duplex (TDD) mode mmWave mMIMO system, where the base station occupy N number of antennas and NRF RF chains to simultaneously serve K single antenna users in the single cell setup. Down link (DL) model is contemplated to clarify the fundamental principle of mmWave mMIMO with lens antenna array and to analyse the performance of BS-NOMA with estimated BS system but to estimate the DL BS channel UL scenario is considered.
The received signal can be represented as
Where and S are the spatial channel matrix and transmitted signal vector with normalized power
respectively. P is transmitted power for all k users that satisfies
. W is an N × K with ||Wk||2 = 1 and n is additive white Gaussian noise (AWGN) vector with zero mean and σ 2 variance.
shows system model of BS-NOMA with estimated BS channel. It consists dimension reduced precoder with reduced RF chain and a 1-bit phase shifter-based ASN. The ASN used to generate measurement matrix of BS channel to estimate BS channel and used as beam selector at the time of data transmission. Then after the power dominate beams selection from the estimated BS channel, each selected beams support multiple users.
In a channel model, the statistical fading distributions used in traditional MIMO analysis is inaccurate for mmWave channel modelling because of the hybrid of tightly packed arrays with in sparse scattering environments in mmWave system. Hence, Saleh-Valenzuela channel model shown in (2) plays a significant role to capture the mathematical structures of mmWave channels accurately (Gao et al., Citation2016; Wang et al., Citation2017).
Where is the line of sight (LOS) component of
with
presenting the complex gain and spatial direction
is the non-line-of-sight (NLOS) component of
for
is the Jth NLOS component of the Kth user, where J is the total number of NLOS components.
is the antenna array steering vector of size N × 1.
3.1. BS channel estimation
To estimate the BS channel, the UL CSI can be more easily acquired at the BS due to a few number of single antenna users. Where, the estimation accuracy and overhead in the UL is independent of number of base station antenna N (Bj¨ornson et al., Citation2017). Under this consideration firstly the UL BS channel is estimated at the base station and then this estimation is used for the DL system to transmit data from the base station to the user, which means the base station uses the estimated BS channel to detect the UL data and generate beamforming vectors for DL data transmission (Khandaker & Wong, Citation2018).
To estimate the BS channel, in the UL of TDD systems, all K users should send known pilot sequences to the base station over Q instants (each user send one pilot symbol in each instant) for channel estimation, and we assumed that the BS channel remains unchanged within such channel coherence time (Gao et al., Citation2017, Citation2019). In addition, our work considered block type pilot transmission approach, where Q instants are divided into M blocks and each block consists of K instants, that is, Q = MK. The Pilot matrix Ψ of size k × k for the mth block which contains K mutually orthogonal pilot sequences transmitted by k users over K instants (Gao et al., Citation2017, Citation2019). Obviously, we have and
to normalize the UL pilot power to unit. The received UL signal matrix
of size N × K at the base station in the mth block can be expressed as
Where Nm is the noise matrix in the mth block of size N × K whose entries are independent and identically distributed (i.i.d) complex Gaussian random variables with zero mean and σ2 UL (UL noise power). The UL SNR can be considered as for UL pilot power normalized to 1. During pilot transmission, combiner Dm of size K × N is used at the base station to combine the received UL signal
expressed in Equationequation 3
(3)
(3) . Then, the sparse channel estimation problem can be expressed as
Where and
are the kth column of
and
respectively.
The sparse signal recovery problem expressed in Equationequation 4(4)
(4) is solved by classical CS algorithm called OMP because OMP is one of the greedy approaches which is simple in implementation and computationally efficient. The detail operation of OMP algorithm is discussed on algorithm 1. Next, the power dominate beams is selected from the estimated BS channel to serve more number of users than required number of RF chains in the system and the bean selection algorithm is shown in algorithm 2.
Table
Table
After beam selection process the received signal vector be modified as
Where of size
is the dimension reduced BS channel matrix including selected beams and B is the index set to selected beams.
is dimension reduced precoding matrix of size
. The row dimensions of
is much lesser than N (the row dimension of the original precoding matrix
) and also the required number of RF chain is significantly reduced and equal to the number of selected beams in the system.
4. BS-NOMA with estimated BS channel for mmWave mMIMO system
In BS-NOMA with estimated BS channel number of users served in beam which is expressed as
for
where
for
. Consequently, each beam contains a distinct users and a single beam can serve multiple users. Number of selected beams
is equal to the number of RF chain
but numbers of served users are greater than number of RF chain. Thus, the received signal of
user in
beam can be presented as
Where the transmitted signal and transmitted power for the user in
beam are represented by
and
,respectively, and
is the additive noise with zero mean and
variance. The
user interference (for all
) in
can be cancelled by the SIC operation according to increasing order of equivalent channel gains,
. Then, after SIC operation the received signal of
user in
beam can be rewritten as shown in Equationequation 7
(7)
(7) .
Finally, for interference plus noise term the SINR and achievable sum rate
user in
beam are expressed in Equationequation 8
(8)
(8) and Equation9
(9)
(9) , respectively.
Where
OMP algorithm can achieve better achievable sum rate at higher UL-SNR than lower UL-SNR value (Gao et al., Citation2017). To improve its performance further we combine BS-MIMO with power domain NOMA. However, direct combination of NOMA technology with BS-MIMO cannot give a guarantee for efficient performance in BS-NOMA with estimated BS channel. So, to nullify the interference and improve achievable sum rate of BS-NOMA with estimated BS channel linear precoding techniques (ZF, MMSE, MRT) and power allocation are considered. The power allocation for different users in different beams is optimized by using dynamic power allocation based on the constraints shown in Equationequation 10(10)
(10) .
5. Simulation results and analysis
5.1. Simulation parameters
shows the simulation parameters used to generate the results that will be discussed in the consecutive sections.
Table 1. Simulation parameters
5.2. Spectral efficiency versus DL-SNR
shows spectral efficiency performance analysis of BS-NOMA with estimated BS channel and compared with other MIMO architecture. Fully digital MIMO in perfect CSI achieve highest spectral efficiency than other MIMO architectures. On the other hand, BS-NOMA with estimated BS channel achieves better spectral efficiency than BS-MIMO estimate for the same estimation techniques and also close to the BS-NOMA with perfect BS channel. Because the dynamic power allocation and linear precoders are used to maximize system-achievable sum rate by mitigate the existing interferences.
Figure 2. Spectral efficiency versus DL-SNR with different MIMO architecture at K = 32, N = 400 and UL-SNR = 20 dB.
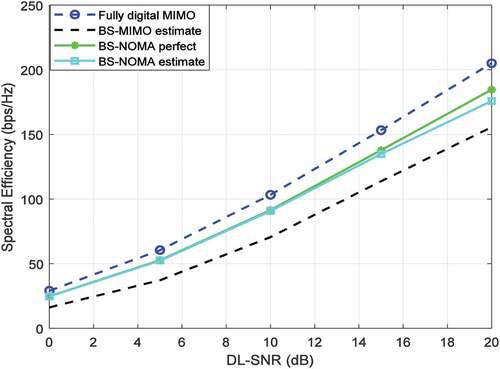
Similarly, presents spectral efficiency versus DL-SNR of BS-NOMA with estimated BS channel for K = 32, N = 400, UL-SNR = 20 dB and different linear precoding techniques. As one can observe from the result, the spectral efficiency of MMSE slightly outperforms both ZF and MRT at lower power regime but for higher power regime, since ZF approaches an optimal it outperforms the MMSE and MRT precoding techniques.
5.3. Spectral efficiency versus number of users
discusses spectral efficiency versus number of user of BS-NOMA with estimated BS channel and compared the obtained result with other MIMO architecture. The result reveals that the performance of fully digital MIMO is better than both BS-NOMA and BS-MIMO with estimated BS channel. As the number of users increase BS-NOMA with estimated BS channel gives better performance than BS-MIMO estimate. This is true because as the number of users increase in BS-MIMO system the probability of different users select the same beam will be increased and this leads existence of multi-user interference but for the case of BS-NOMA estimate the dynamic power allocation mitigate the effect of interference.
Figure 4. Spectral efficiency versus number of users with different MIMO architecture at DL-SNR = 10 dB, N = 400 and UL-SNR = 20 dB.
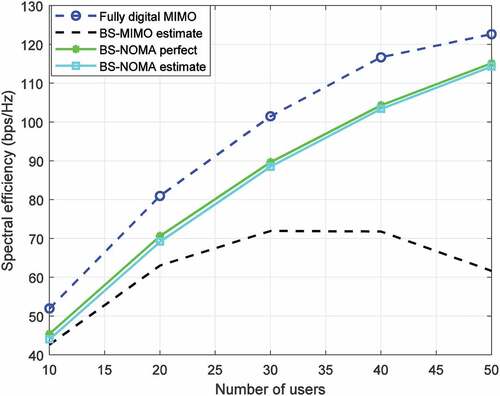
The impact of linear precoding on the performance of spectral efficiency for variable number of users is shown in . As it can be seen from the result BS-MONA with estimated BS channel with MMSE out performs ZF and MRT till number of users K = 30. Although the MMSE is able to minimize inter-beam interference, but as a number of served users are getting large ZF gives better spectral efficiency.
5.4. Energy efficiency versus DL-SNR
show the energy efficiency for variable DL-SNR values of BSNOMA with estimated BS channel and other MIMO architecture without and with linear precoding, respectively. As one can see from , energy efficiency of BS-NOMA with estimated BS channel has comparable performance with perfect BS channel but gives better performance than BS-MIMO and fully digital MIMO system because BS-NOMA able to serve multiple users in each beam and the required number of RF chain is equivalent to number of selected beam. Whereas the number of RF chain in BS-MIMO fully digital MIMO system are equivalent to number of served user and number of base station antennas respectively. Similarly as shown in , the same linear precoders performance comparison are made for energy efficiency as that of spectral efficiency discussed in the above section.
Figure 6. Energy efficiency versus DL-SNR with different MIMO architecture at K = 32, N = 400 and UL-SNR = 20 dB.
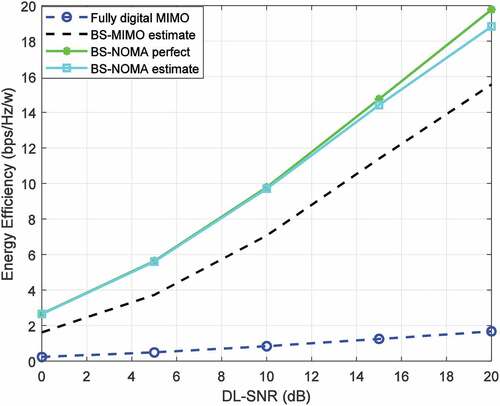
Figure 7. Energy efficiency versus DL-SNR with different precoding schemes at K = 32, N = 400 and UL-SNR = 20 dB.
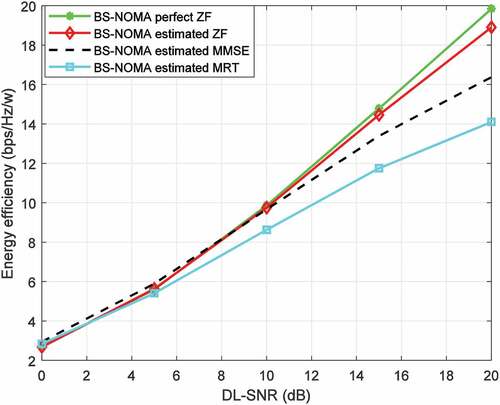
In , the energy efficiency performance of BS- NOMA with and without estimated BS channel, BS-MIMO with estimated BS channel and fully digital MIMO systems are compared for variable number users. As the numbers of served users are increased relatively, the required number of RF chain will increase for BS-MIMO system, whereas it is possible to serve large number of users with a limited number of RF chain. That is why the result claims that the performance of BS-NOMA is better than BS-MIMO and fully digital MIMO system.
Figure 8. Energy efficiency versus number of users with different MIMO architecture @ DL-SNR = 10 dB, N = 400 and UL-SNR = 20 dB.
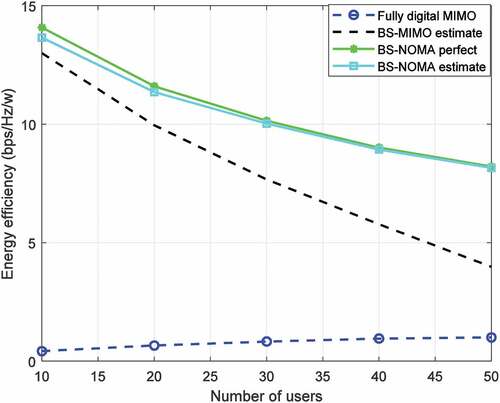
Finally, the comparison analysis of our work with imperfect BS channel is compared with other three related works as shown in . As one can see from the table the spectral and energy efficiency of the proposed work are approaching to the performance of the system that considered perfect channel.
Table 2. Comparison analysis of our work with related works
6. Conclusion
To reduce the dimension of mMIMO system using beam selection techniques, the base station must know accurate information of CSI before the beam selection and to serve more number of users than required number of RF chain in mmWave mMIMO system different technologies have to be considered. In this work, to estimate the BS channel pilot based channel estimation technique with CS sensing tool OMP algorithm is used and BS-MIMO with power domain NOMA are integrated to serve large number of users than RF chains. Based on the simulation results shown in the results and analysis section, the number of served user in BS-NOMA with estimated BS channel are larger than the required number RF chain. This means multiple users can be served in each selected beam and this increases both the spectral and energy efficiency of the proposed system. The energy efficiency with estimated BS channel outperforms BS-MIMO with estimated BS channel and fully digital system with perfect CSI. Finally, the spectral and energy efficiency of BS-NOMA with estimated BS channel can further be improved by using different linear precoding techniques.
Disclosure statement
No potential conflict of interest was reported by the author(s).
Additional information
Funding
Notes on contributors
Fikreselam Gared Mengistu
Fikreselam Gared Mengistu received his PhD from Taiwan National University of Science and Technology (NTUST), Taiwan, in Communication Engineering. He received his MSc and BSc from Addis Ababa University and Bahir Dar University, Ethiopia, respectively. His research interests are in the areas of wireless sensor networks, massive MIMO systems, Millimeter wave communications, cognitive radio and error control coding.
Fikrte Belay Mihrete
Fikrte Belay Mihrete received her MSC and BSC from Bahir Dar University and Kombolcha institute of technology, Ethiopia, respectively. Her research interests are in the areas of wireless communication system and antenna system.
References
- Amadori, P. V., Masouros, C., Vellekoop, A. E., Vrouenraets, B. C., Westhoff, D., van de Beek, D., de Rooij, S. E., & van Munster, B. C. Low RF-complexity millimeter-wave beamspace-MIMO systems by beam selection. (2015). Journal of the American Geriatrics Society, 63(6), 2212–14. IEEE. https://doi.org/10.1111/jgs.13696
- Bj¨ornson, E., Hoydis, J., & Sanguinetti, L. (2017). Massive MIMO networks: Spectral, energy, and hardware efficiency. Foundations and Trends in Signal Processing, 11(3–4), 154–655. https://doi.org/10.1561/2000000093
- Busari, S. A., Huq, K. M. S., Mumtaz, S., Dai, L., & Rodriguez, J. (2017). Millimeter-wave massive MIMO communication for future wireless systems: A survey. IEEE Communications Surveys & Tutorials, 20(2), 836–869. https://doi.org/10.1109/COMST.2017.2787460
- Gao, X., Dai, L., Chen, Z., Wang, Z., & Zhang, Z. (2016). Near-optimal beam selection for beamspace mmWave massive MIMO systems. IEEE Communications Letters, 20(5), 1054–1057. https://doi.org/10.1109/LCOMM.2016.2544937
- Gao, X., Dai, L., Han, S., Chih-Lin, I., & Wang, X. (2017). Reliable beamspace channel estimation for millimeter-wave massive MIMO systems with lens antenna array. IEEE Transactions on Wireless Communications, 16(9), 6010–6021. https://doi.org/10.1109/TWC.2017.2718502
- Gao, X., Dai, L., Zhou, S., Sayeed, A. M., & Hanzo, L. (2019). Wideband beamspace channel estimation for millimeter-wave MIMO systems relying on lens antenna arrays. IEEE Transactions on Signal Processing, 67(18), 4809–4824. https://doi.org/10.1109/TSP.2019.2931202
- Khandaker, M. R. A., & Wong, K.-K. 2018. Signal processing for massive MIMO communications, Academic Press Library in Signal Processing, vol.7, pp.367–401
- Liu, P., Yong, L., Cheng, W., Zhang, W., & Gao, X. (2019). Energy-efficient power allocation for millimeter wave beamspace MIMO-NOMA systems. IEEE Access, 7, 114582–114592. https://doi.org/10.1109/ACCESS.2019.2935495
- Pachaiyappan, G., & Ramanujam, P. Design of low profile coaxial fed high gain stacked patch antenna for Wi-Fi/WLAN/Wi-Max applications. De Gruyter. (2021). 75(12), 27–34. https://doi.org/10.1515/freq-2020-0021
- Pal, R., & Sarawadekar, K. P. 2019. Performance study of mmWave beamspace MU-MIMO-NOMA communication system, 2019 IEEE International Conference on Advanced Networks and Telecommunications Systems (ANTS), pp.1–6.
- Ramanujam, P., Arumugam, C., Venkatesan, R., & Ponnusamy, M. (2020). Design of compact patch antenna with enhanced gain and bandwidth for 5G mm-wave applications. IET Microwaves, Antennas & Propagation, 14(12), 1455–1461. https://doi.org/10.1049/iet-map.2019.0891
- Ramanujam, P., Ponnusamy, M., & Ramanujam, K. A compact wide-bandwidth Antipodal Vivaldi Antenna array with suppressed mutual coupling for 5G mm-wave applications. Elsevier. (2021). 133(12), 153668. https://doi.org/10.1016/j.aeue.2021.153668
- Ramanujam, P., Venkatesan, P. G., Ramesh, & Chandrasekar, A. Electromagnetic interference suppression in stacked patch antenna using complementary split ring resonator. Wiley Library. (2020). 62(1), 193–199. https://doi.org/10.1002/mop.31985
- Tropp, J. A., & Gilbert, A. C. (2007). Signal recovery from random measurements via orthogonal matching pursuit. IEEE Transactions on Information Theory, 53(12), 4655–4666. https://doi.org/10.1109/TIT.2007.909108
- Venugopal, K., Alkhateeb, A., Nuria Gonz´alez, P., & Heath, R. W. (2017). Channel estimation for hybrid architecture-based wideband millimeter wave systems. IEEE Journal on Selected Areas in Communications, 35(9), 1996–2009. https://doi.org/10.1109/JSAC.2017.2720856
- Wang, B., Dai, L., Wang, Z., Ning, G., & Zhou, S. (2017). Spectrum and energy-efficient beamspace MIMO-NOMA for millimeterwave communications using lens antenna array. IEEE Journal on Selected Areas in Communications, 35(10), 2370–2382. https://doi.org/10.1109/JSAC.2017.2725878
- Xie, T., Dai, L., Gao, X., Yao, H., & Wang, X. 2017. On the power leakage problem in beamspace MIMO systems with lens antenna array. 2017 IEEE 86th Vehicular Technology Conference, VTC-Fall (pp. 1–5).