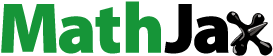
Abstract
Bi2O3/Fe material has been successfully synthesized using the microwave-assisted precipitation method. This study aims to analyze the effect of Fe on the characteristics of Bi2O3 and its ability to photodegrade amoxicillin. XRD characterization showed that all samples (Bi2O3/Fe 0–9%) formed an α-Bi2O3 phase. The addition of Fe concentration did not significantly change the crystal structure of α-Bi2O3. The morphology of Bi2O3/Fe is mostly rod and spherical porous which is indicated as Bi2O3 and Fe particles. EDX characterization informed that all samples contained Bi, O, and Fe elements which were evenly distributed. The addition of Fe up to 3% will increase the crystallite size and specific surface area of Bi2O3 but decrease the energy gap. Meanwhile, the addition of more than 3% Fe causes an increase in the energy gap of the Bi2O3 but will decrease the crystallite size and specific surface area. Bi2O3/Fe 3% is the best sample because it has the largest crystallite size (29.1 nm), the lowest energy gap (2.02 eV), the largest specific surface area (6.864 m2/g), and can degrade amoxicillin to 76.34% with a degradation rate of 0.0079 min−1.
PUBLIC INTEREST STATEMENT
Amoxicillin is one of the most used antibiotics. The use antibiotics of amoxicillin which is quite a lot in daily life in addition to providing health benefits, also make one source of pollution of the water environment through medical waste. The existence of amoxicillin in the water environment can damage the environment and threaten health. Photocatalyst material currently has an important role in the degradation of medical waste. In this study, we found effective and efficient material for the degradation of amoxicillin medical waste, namely Bi2O3/Fe which was synthesized using the precipitation method assisted by microwave radiation. It is hoped that this discovery is useful for overcoming water environmental problems by Amoxicillin medical waste.
1. Introduction
In recent years, environmental pollution from liquid medical waste has become increasingly widespread. It is a challenge for both researchers and the pharmaceutical industry because drugs produce medical waste that can threaten ecosystems and human health (Wang et al., Citation2019). One of the medical waste that is a source of environmental pollution is amoxicillin. Amoxicillin (AMX) is the most widely used type of antibiotic because of its extraordinary antibacterial properties (Jung et al., Citation2012). However, AMX has low biodegradability and remarkable stability with many complex aromatics in its molecule, making it difficult to destroy by conventional methods (Zhang et al., Citation2019). Therefore, we need the proper technique to degrade AMX efficiently.
Several alternatives have been carried out to remove these compounds, including reverse osmosis, adsorption, and advanced oxidation technologies such as fenton reaction, ozonation, and photocatalytic technology (Benitez et al., Citation2011). Photocatalytic shows promising prospects among these methods due to its sustainability and environmental friendliness (Wang et al., Citation2018). Bismuth oxide (Bi2O3) is a photocatalyst material that is of interest to researchers because of its high redox inversion, environmental friendliness, thermal stability, and energy bandgap between 2.58 and 2.85 eV, which shows a response to visible light (Basith et al., Citation2018). The phase (monoclinic) Bi2O3 has semiconductor properties, is stable at low temperatures, and is more energy-efficient in the synthesis process (Gadhi et al., Citation2016). However, photon-induced electron–hole pairs have poor light utilization and fast recombination rates limiting photocatalytic activity (Zhou et al., Citation2019).
In this study, the Bi2O3 semiconductor will be inserted with Fe material with the microwave-assisted precipitation method. This experiment is believed to increase the rate of electron–hole pair separation by acting as a shallow trap where the permissible energy states are close to the conduction band and valence band and reduce the rate of electron–hole pair recombination in Bi2O3 materials (Zhu et al., Citation2011). Finally, the authors hope to be able to produce innovative materials that are effective in degrading AMX.
2. Experiment method
2.1. Synthesis of Bi2O3/Fe
Bi2O3/Fe was synthesized using a microwave-assisted precipitation method. The synthesis process of Bi2O3/Fe is shown in Figure . Bi(NO3)3.5H2O 0.5 g and Fe(NO3)3.9H2O with different mass (1/100, 3/100, 5/100, 7/100, 9/100) (%wt) were dissolved in 50 ml of 5% HNO3 solution (1 mol/l) stirred for 10 minutes using a magnetic stirrer on a hot plate at room temperature. A total of 250 ml of NaOH (1 mol/l) was added to the solution and stirred for 2 hours, then allowed to stand to produce a precipitate. The precipitate was separated and heated on a hotplate for 2 hours at 120°C to produce a still moist Bi2O3/Fe powder. Furthermore, the resulting Bi2O3/Fe powder was then dried by exposing it to 100 Watt microwave power for 3 hours.
2.2. Characterization
Structural analysis of Bi2O3/Fe powder was carried out using X-Ray Diffraction (XRD Shimadzu 6100/7000 type). The X-ray wavelength used is 1.5406 Å. Data retrieval is carried out every 0.02° angle range. The crystallite size of Bi2O3/Fe was calculated using the Debbye-Scherrer equation (EquationEquation 1(1)
(1) ; Xie et al., Citation2019).
Ds is the crystallite size (nm), 1.5406 Å is the wavelength of X-rays, k = 0.9 is the Scherrer constant, is the Full Width and Half Maximum or FWHM (in radians), and is the Bragg angle of diffraction or peak position (in degree).
The surface morphology of Bi2O3 powder was obtained from the Scanning Electron Microscopy and Energy Dispersive X-Ray (SEM-EDX) characterization data. The SEM-EDX image is used to determine the element, shape, and particle size. The specific surface area was obtained from the Surface Area Analyzer (SAA) characterization. UV-Vis transmittance data of Bi2O3/Fe powder was used to determine the energy band gap value.
2.3. Amoxicillin degradation
The degradation was carried out by adding 0.02 g of Bi2O3/Fe powder into 50 ml of 10 ppm AMX solution obtained by dissolving 10 mg of AMX powder (Amoxicillin Trihydrate 500 mg generic) into 1 liter of distilled water. The solution was irradiated with UV light for 180 minutes, and the degradation products were taken every 30 minutes. The degradation results were analyzed using a UV-Vis Spectrophotometer UH5300 (Hitachi, Japan). Photocatalyst efficiency (Ef) is calculated using EquationEquation 2(2)
(2) . (Sudrajat et al., Citation2018).
where C0 and Ct are the initial and final concentrations of the solution.
3. Results and disscusions
Bi2O3/Fe has been synthesized using a microwave-assisted precipitation method. This method is very advantageous because it is energy-intensive, homogeneous, efficient, can reach high temperatures, and start reactions quickly. Microwaves simplify and speed up the synthesis process by generating heat in a short time due to the activity of compounds that oscillate and collide with each other. Simultaneously, microwaves help the growth (nucleation) of the material due to the slight gradient or heat difference that occurs (Fatimah et al., Citation2015). The high-frequency motion of molecules from microwaves causes the magnetic dipoles of the molecules to change direction very quickly and cause friction. As a result, friction will generate thermal energy used in the sintering process to form a photocatalyst Bi2O3/Fe. In addition, molecular vibrations due to microwaves cause chemical bonds in the precursor to be rearranged (Rahmayanti et al., Citation2015).
The synthesized powder shows a yellow physical color that shifts brownish with increasing Fe concentration, as shown in Figure . The color change occurs because Fe(NO3)3.9H2O when dissolved, forms a brownish to dark-colored solution due to hydrolysis. As the concentration of Fe increases, the reaction shifts in the opposite direction of the substance. Bi2O3 added with Fe will shift its color from yellow to brown. It follows Le Chatelier’s Principle, which studies changing conditions on an equilibrium system. If colored ions are added to a reaction, the compound formed will be darker (Prakoso et al., Citation2012).
To confirm the success of Bi2O3/Fe synthesis, the first thing to do is test the crystallinity. Based on the XRD Bi2O3/Fe pattern as shown in Figure , the resulting diffraction peaks correspond to JCPDS No. 27–0053 with space group P21/c, monoclinic crystal system, unit cell parameters; a = 5.83 Å b = 8.14 Å c = 7.48 Å β = 67.07°, and density 9.467 g/cm3 (Devi et al., Citation2019). The main diffraction peak is at 2θ = 24.54 ; 25.75
; 26.91
; 27.38
; 27.99
; 32.30
; 33.08
; 35.04
; 37.60
; 46.31
; and 48.54
with miller index (102), (002), (112), (121), (012), (211), (202), (212), (113), (223), and (104). The synthesized Bi2O3 material is in the
-Bi2O3 phase. These results indicate that the Bi2O3 material has been completely formed and has an excellent level of stability at low temperatures (Chen et al., Citation2011). There is a difference in intensity in the XRD pattern, but it does not significantly change the lattice structure. Based on the Debbye-Scherrer equation, the crystallite size of Bi2O3 with the addition of Fe from 0–9%, respectively, was 25.7 nm, 27.1 nm, 29.1 nm, 23.7 nm, 24.0 nm, and 21.7 nm. The addition of Fe resulted in differences in the size of the resulting crystals. The smaller the FWHM, the crystallinity is better .
The resulting crystallite size is inversely proportional to the FWHM value (Table ), while the intensity of each crystal plane influences the FWHM value, the higher the intensity, the smaller the FWHM value. The smaller the FWHM value, the better crystal quality because it shows that adjacent atoms are easier to adjust the direction and length of their bonds (Suryanarayana, Citation1998).
Table 1. 2 , FWHM, and crystallite size of Bi2O3/Fe
Increasing the concentration of Fe from 5% to 9% causes the emergence of a new phase at 2θ = 41.79 ; 43.77
; 45.62
; 52.62
; 54.26
; 55.88
and 62.05
with miller index (323), (422), (341), (343), (424), (352), and (613) indicated to be the bismuth iron oxide (Bi25FeO40) phase according to JCPDS No. 46–0416 (Basith et al., Citation2018). The phase percentage between
-Bi2O3 and Bi25FeO40 was 82.6%: 17.4%. It indicates that the 5–9% Fe doped still has a main phase of
-Bi2O3, but there is a secondary phase of Bi25FeO40. The secondary phase is formed due to the addition of a larger Fe dopant concentration in Bi2O3, which causes the Bi and Fe atoms to undergo a reaction so that Fe should partially replace the position of the Bi atom, but due to the high concentration of Fe atom, the position of the Bi atom is completely replaced by Fe atom (Yuwita et al., Citation2019). The appropriate Fe dopant can increase the crystallite size. However, more than 3% Fe dopant causes the crystallite size to decrease due to the reaction between Fe and Bi atoms. The difference influences the reaction in the ionic radius of Fe3+ (0.69 Å) smaller than the ionic radius of Bi3+ (1.17 Å). The difference in atomic radius inhibits the Bi nucleation process because the increasing presence of Fe restrains it.
SEM characterization was carried out to determine the surface morphology of the sample. The characterization image can be seen in Figure ). Pure Bi2O3 particles are indicated to have like a rod structure (Mukholit et al., Citation2019), but due to the high agglomeration rate, the rod particles accumulate in one area and form spheres (Figure )). The addition of 1% Fe causes the particle surface to become porous (Figure )). The addition of 3–9% Fe displayed a porous sphere and rod (Figures )). Particles with a porous spherical shape are indicated as Fe particles (McRae et al., Citation2019). The number of particles resembling a porous sphere increases with increasing Fe doping concentration. This is consistent with the results of the SEM-EDX mapping (Table ). The addition of Fe concentration from 1% to 9% increased the %mass and %atoms Fe in the sample. The mapping results also show that the resulting sample contains Bi, O, and Fe elements. However, increasing the concentration of Fe doping resulted in a decrease in the particle size of Bi2O3 because the crystal Bi3+ ions were retained during the sintering process. When Fe3+ ions are inserted into the Bi2O3 crystal lattice, a certain degree of deformation will be introduced into the lattice due to the difference in ionic radius between Fe3+ ions (0.69 Å) and Bi3+ ions (1.17 Å), this is similar to the description of previous studies (Liang et al., Citation2014). Particle size was measured using Image J software. The average particle sizes of Bi2O3 0–9% were 1.5 m, 1.2
m, 4.6
m, 3.6
m, 2.1
m, and 1.6
m. While the average particle sizes of Fe doped 1–9% were 1.5
m, 2.4
m, 2.8
m, 2.2
m, and 1.8
m. Particle size measurement through SEM image has a larger size than XRD pattern crystallite size measurement because the particles group together which causes agglomeration during synthesis (Hernowo & Nurhasanah, Citation2019). The structure of Fe, which resembles a porous sphere, can increase the photocatalytic activity of Bi2O3. One of the requirements for a good photocatalyst material is to have a porous surface to increase the surface area for photon absorption during photocatalysis. The large surface area results in more vacancies, thereby increasing the diffusion between AMX pollutant molecules and the photocatalyst material (Rissa et al., Citation2012; Sistesya & Sutanto, Citation2013).
Table 2. Result of SEM-EDX
The results of the Surface Area Analyzer (SAA) to the Bi2O3/Fe samples showed the specific surface area of the Brunauer-Emmet-Teller (BET). The specific surface area is calculated with N2 adsorption-desorption analysis that obtained successive results for Bi2O3/Fe 0–9% were 3.975 m2/g, 3.354 m2/g, 6.864 m2/g, 2.258 m2/g, 3.634 m2/g, and 3.069 m2/g. Bi2O3/Fe 3% has an optimal specific surface area so that it has the best potential in the AMX degradation process. The specific surface area of a large catalyst powder can increase the amount of AMX absorbed on the surface of the Bi2O3/Fe powder (Nurhasni et al., Citation2018).
The energy gap value (Figure ) is obtained by plotting and
of the transmittance spectrum using the Tauc Plot method. Calculation of Energy gap (Eg) using EquationEquation 3
(3)
(3) (Raizada et al., Citation2017).
The index (k) is determined from the Bi2O3 semiconductor optical transition type, namely 4 for indirect transition (Liang et al., Citation2014) hv is the photon energy, A is the proportionality constant, and indicates the photon energy the absorption coefficient. The synthesized Bi2O3/Fe has an Eg of about 2.02–2.81 eV, presented in Table . The addition of a suitable Fe dopant can reduce the energy bandgap of the semiconductor. The minimum energy gap is obtained at the addition of 3% Fe, which is 2.02 eV. A small energy gap indicates that the energy band gap is getting narrower between the valence band and the conduction band. So that when excitation occurs due to the influence of photon energy from UV light irradiation, it will facilitate the production of electrons and holes because the energy needed for electrons to move from the valence band to the conduction band is getting smaller. The higher the production of electrons and holes can increase the photocatalytic activity.
The degradation of AMX determined the photocatalytic activity of Bi2O3/Fe. This photocatalytic activity shows a decrease in absorbance, efficiency, and degradation rate constant. Figure ). showed that the absorbance value of AMX decreased with the length of irradiation time. The longer duration of irradiation resulted in more degrading species from the active Bi2O3/Fe photocatalyst so the greater the concentration of degraded AMX. The efficiency of the Bi2O3/Fe photocatalyst in degrading AMX is shown in Figure ). The highest efficiency was obtained at 3% Fe doping, which was 76.00%.
Figure 6. (a) AMX absorbance decrease graph (b) Bi2O3/Fe degradation efficiency (c) Bi2O3/Fe degradation rate.
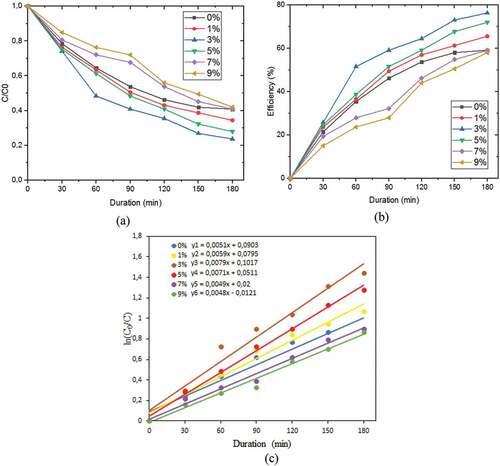
The rate degradation of Bi2O3/Fe concerning AMX according to the Langmuir–Hinshelwood (L–H) kinetic model, determined by the pseudo-first-order kinetic equation (EquationEquation 4(4)
(4) )
is the initial AMX concentration (mg/l), C is the AMX concentration at a certain time (t) (mg/l),
is the pseudo-first-order rate constant (min−1) (Qin et al., Citation2014). The results can be seen in Figure ). and in Table . The calculation results of the degradation rate explain that the 3% Bi2O3/Fe sample has the highest degradation rate constant of 0.0079 min−1, while 9% Bi2O3/Fe has the lowest degradation rate constant of 0.0048 min−1. The rate of degradation describes the speed of the material in degrading waste, the higher the value of the rate of degradation, the faster the material in degrading waste, so that the waste contained in the material is less.
Table 3. Efficiency, degradation rate, and energy gap of Bi2O3/Fe
The photocatalytic activity of Fe-doped Bi2O3 is higher than that of pure Bi2O3 due to the synergistic effect of Fe3+ ions and Bi2O3. The 3% Bi2O3/Fe material has the most significant degradation efficiency. These results indicate that adding Fe up to a concentration limit of 3% in Bi2O3 will increase the photocatalytic activity, while the addition of more than 3% will decrease photocatalytic activity. These results are consistent with previous research by Liang et al. (Citation2014). Photocatalytic activity and degradation efficiency can be related to the crystallite size of Bi2O3/Fe (Nandiyanto et al., Citation2020), where the larger the crystallite size of a material, the greater the photocatalytic ability (Armaković et al., Citation2017; Sharma et al., Citation2014). In addition, the presence of Fe particles resembling a porous sphere on the surface of the Bi2O3 semiconductor as shown in the SEM image also affects the photocatalytic activity. The porous surface can increase the photocatalyst material’s surface area, and the presence of Fe transition metal can suppress the electron–hole recombination rate. This is also under the results of the BET analysis, a large specific surface area can increase photocatalytic activity. The value of the obtained Bi2O3/Fe energy gap also affects the photocatalytic activity. As presented in Table . The minimum Eg occurs at 3% Fe doping, following the results of the photocatalytic activity test, where the efficiency and optimal rate also occur at 3% Fe doping.
Chemically, the degradation of AMX by Bi2O3/Fe can be explained as shown in Figure . When Bi2O3 is irradiated by UV light, the electrons in the photocatalyst material Bi2O3 which are in the valence band will be excited to the conduction band. This results in more electrons in the conduction band, while in the valence band there will be many holes formed due to electrons being excited to the conduction band. Electrons that do not recombine will be trapped on the surface of the semiconductor. Photogenerated electrons and holes combine with Fe3+ ions to form Fe4+ and Fe2+ ions (EquationEquation 5(5)
(5) –Equation8
(8)
(8) ).
Fe2+ and Fe4+ ions are relatively unstable ions when compared to Fe3+ ions. Therefore, the trapped charge can be easily released from the Fe2+ ions or Fe4+ ions and then migrate to the surface to start the photocatalytic reaction with the following reaction:
Fe2+ ions can be oxidized to Fe3+ ions by transferring electrons to O2 adsorbed on the surface of Bi2O3. Meanwhile, the adsorbed O2 is reduced to superoxide anion radical () ; EquationEquation 9
(9)
(9) ), which functions as a reducing agent and further reduces the AMX solution. Likewise, Fe4+ ions are reduced to Fe3+ ions by losing electrons, while the surface hydroxyl group (OH−) becomes a hydroxyl radical (
) EquationEquation 10)
(10)
(10) . In addition, the unrecombined holes will be trapped on the surface of the Bi2O3 semiconductor and react with H2O and form hydroxyl radicals (
). The resulting hydroxyl radical is a potent reducing agent to reduce AMX solution (Saravanan et al., Citation2013).
The degradation process occurs through direct charge transfer consisting of photoinduction carriers or reactive oxygen species (ROS; Guo et al., Citation2011). The ROS that contributes to the photocatalytic reaction of Bi2O3/Fe as a robust oxidizing and reducing species to degrade AMX are superoxide anion radicals () and hydroxyl radicals (
) which will form CO2, H2O, and other inorganic components (EquationEquation 11
(11)
(11) –Equation15
(15)
(15) ). These chemical reactions will bind to AMX pollutants around the Bi2O3/Fe material to degrade AMX pollutants. These radicals will be formed continuously as long as the Bi2O3/Fe material is exposed to UV light.
In the presence of Fe transition metal doping, Fe3+ ions are responsible for the electron-hole recombination rate and support the increase in photocatalytic activity. Excess Fe3+ ions is entering the cluster formation. This cluster can resist AMX photodegradation by masking the active site from the Bi2O3 surface. Fe3+ ions will act as photo-generated between holes and electron transfer, the rate of electron-hole recombination during irradiation can be suppressed by increasing the number of trapped electrons to increase the lifetime of electrons and holes. This decrease in the recombination rate increases the photocatalytic activity of Bi2O3/Fe.
The Bi2O3/Fe material produced is in powder form, so that in its application, it can only be used once to degrade AMX medical waste, the possibility of recycling can be carried out further by coating the material on a glass substrate to produce Bi2O3/Fe thin-film material. Materials in the form of thin films can be applied repeatedly in the degradation process (Sutanto et al., Citation2021).
To carry out degradation under solar irradiation, it is necessary to modify the energy bandgap of the Bi2O3/Fe material which corresponds to the photon energy of visible light, which is smaller than 3.1 eV. In this study, researchers have modified the energy band gap of Bi2O3/Fe to 2.81–2.02 eV, so that in principle it is possible for electron transfer and photocatalytic reactions to occur even under sunlight.
4 Conclusion
The synthesis of Bi2O3/Fe has been successfully carried out using the microwave-assisted precipitation method. XRD characterization results showed that the phase formed was α-Bi2O3. The morphology of Bi2O3 is porous rods and spheres indicated as Bi2O3 and Fe particles. Bi2O3/Fe 3% has the largest crystallite size (29.1 nm), the lowest energy gap (2.02 eV), the most optimal specific surface area (6.864 m2/g), the highest degradation rate (0.0079 min−1), and the most optimal degradation efficiency (76.34%). The addition of Fe with a concentration of up to 3% in Bi2O3 can increase the photocatalytic ability, but a concentration of more than 3% will decrease the photocatalytic ability.
Acknowledgements
This research was funded by Kementerian Riset dan Teknologi/BRIN Republik Indonesia for Penelitian Dasar Unggulan Perguruan Tinggi (PDUPT) scheme with contract number: 187-24/UN7.6.1/PP/2021.
Disclosure statement
No potential conflict of interest was reported by the author(s).
Additional information
Funding
Notes on contributors
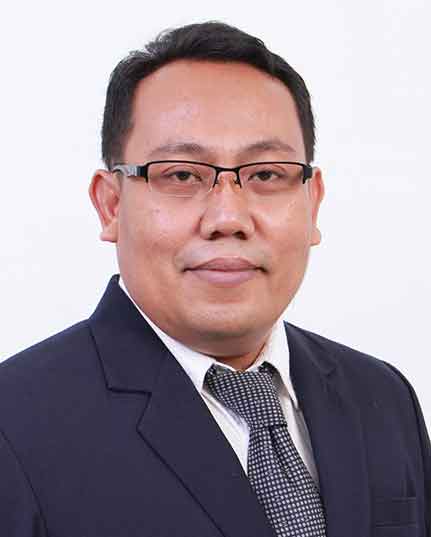
Heri Sutanto
Heri Sutanto is a Full Professor in the Department of Physics, Diponegoro University. Heri Sutanto also leads the Smart Materials Research Center (SMARC), Center of Research and Services-Diponegoro University. He has been doing research in materials science, especially in the development of nanomaterials, semiconductors, thin film, photocatalyst, and radioprotector medical materials, and also focuses on energy-environmental application. He has many publications in scientific journals and recipient of numerous awards both national and international. Bella Aprimanti Utami is a magister student at the Department of Physics, Diponegoro University. This reported research is part of a roadmap for the development of environmentally friendly advanced materials for the degradation of medical waste by the SMARC laboratory.
References
- Armaković, S. J., Grujić-Brojčin, M., Ŝćepanović, M., Armaković, S., Golubović, A., Babić, B., & Abramović, B. F. (2017). Efficiency of La-doped TiO2 calcined at different temperatures in photocatalytic degradation of β-blockers. Arabian Journal of Chemistry, 12(8), 5355–13. https://doi.org/10.1016/j.arabjc.2017.01.001
- Basith, M. A., Ahsan, R., Zarin, I., & Jalil, M. A. (2018). Enhanced photocatalytic dye degradation and hydrogen production ability of Bi25FeO40-rGO nanocomposite and mechanism insight. Scientific Report, 8(1), 1–11. https://www.nature.com/articles/s41598-018-29402-w.
- Benitez, F. J., Acero, J. L., Real, F. J., Roldan, G., & Casas, F. (2011). A comparison study of the removal of selected pharmaceuticals in waters by chemical oxidation treatments. International Journal of Chemical and Molecular Engineering, 5(6), 470–473 https://publications.waset.org/10993/a-comparison-study-of-the-removal-of-selected-pharmaceuticals-in-waters-by-chemical-oxidation-treatments.
- Chen, R., Shen, Z. R., Wang, H., Zhou, H. J., Liu, Y. P., Ding, D. T., & Chen, T. H. (2011). Fabrication of mesh-like bismuth oxide single crystalline nanoflakes and their visible light photocatalytic activity. Journalof Alloys and Compounds, 509(5), 2588–2596. https://doi.org/10.1016/j.jallcom.2010.11.102
- Devi, K. R. S., Mathew, S., Rajan, R., Georgekutty, J., Kasinathan, K., Pinheiro, D., & Sugunan, S. (2019). Biogenic Synthesis of g-C3N4/Bi2O3 heterojunction with enhanced photocatalitics activity and statistical optimization of reaction parameters. Applied Surface Science, 494, 465–476 . https://www.sciencedirect.com/science/article/pii/S0169433219321610?via%3Dihub
- Fatimah, S., Umam, H. I., & Aimon, A. H. (2015). Sintesis Nanokomposit Fe2O3/Zeolite buatan sebagai katalis proses Aquathermolisis. Seminar Kontribusi Fisika 2015, 3, 377 https://ifory.id/proceedings/2015/X9H3ae2VT/skf_2015_siska_fatimah_fffffd279e14b9f51bcf907d14a62294.pdf.
- Gadhi, T. A., Hernandez-Gordillo, A., Bizzaro, M., Jagdale, P., Tagliaferro, A., & Rodil, S. E. (2016). Efficient -Bi2O3 composite for the sequential photodegradation of two-dyes mixture. Ceramic International, 42(11), 13065–13073. https://doi.org/10.1016/j.ceramint.2016.05.087
- Guo, M. Y., Ng, A. M. C., Liu, F., Djurišić, A. B., & Chan, W. K. (2011). Photocatalytic activity of metal oxides-the role of holes and OH radicals. Applied Catalysis B: Environmental, 107(1–2), 150–157. https://doi.org/10.1016/j.apcatb.2011.07.008
- Hernowo, A., & Nurhasanah, I. (2019). Kristalinitas dan Ukuran Nanopartikel ZnO yang dikalsinasi pada Temperatur 100 dan 200. Berkala Fisika, 22(4), 125–131 https://ejournal.undip.ac.id/index.php/berkala_fisika/article/download/27253/16316.
- Jung, Y. J., Kim, W. G., Yoon, Y., Kang, J.-W., Hong, Y. M., & Kim, H. W. (2012). Removal of amoxicillin by UV and UV/H2O2 processes. Science of the Total Environment, 420, 160–167 https://doi.org/10.1016/j.scitotenv.2011.12.011. https://www.sciencedirect.com/science/article/pii/S0048969711014161
- Liang, J., Zhu, G., Liu, P., Luo, X., Tan, C., Jin, L., & Zhou, J. (2014). Synthesis and characterization of Fe-doped -Bi2O3 porous microspheres with enhanced visible light photocatalytic activity. Superlattices and Microstructures, 72, 272–282 https://doi.org/10.1016/j.spmi.2014.05.005. https://www.sciencedirect.com/science/article/pii/S07496036140014
- McRae, M., Julien, P., Salvo, S., Goroshin, S., Frost, D. L., & Bergthorson, J. M. (2019). Stabilized, flat ion flames on a hot counterflow burner. Proceedings of the Combustion Institute 37 (3) , 3185–3191 https://doi.org/10.1016/j.proci.2018.06.134 https://www.sciencedirect.com/science/article/pii/S1540748918303171.
- Mukholit, M., Sutanto, H., Umiati, N. A.K., Hidayanto, E. (2019). Effects of sintering temperature on crystallinity, morphology, and photocatalytic activity of Bi2O3. International Journal of Scientific Research and Management, 07(12), 2321–3418 https://doi.org/10.18535/ijsrm/v7i12.ms01 https://ijsrm.in/index.php/ijsrm/article/view/2465.
- Nandiyanto, A. B. D., Zaen, R., & Oktiani, R. (2020). Correlation between crystallite size and photocatalytic performance of micrometer-sized monoclinic WO3 particles. Arabian Journal of Chemistry, 13(1), 1283–1296. https://doi.org/10.1016/j.arabjc.2017.10.010
- Nurhasni, N., Mar’af, R., & Hendrawati, H. (2018). Pemanfaatan Kulit Kacang Tanah (Arachis hipogaea L.) sebagai Adsorben Zat Warna Metilen Biru. Jurnal Kimia VALENSI, 4(2), 156–167 doi:10.15408/jkv.v4i2.8895 https://journal.uinjkt.ac.id/index.php/valensi/article/view/8895.
- Prakoso, T., Kurniawan, I., & Nugroho, H. (2012). Esterifikasi Asam Lemak Bebas dalam Minyak Sawit Mentah untuk Produksi Metil Ester. Jurnal Teknik Kimia Indonesia, 6(3), 705–709. https://doi.org/10.5614/jtki.2007.6.3.7
- Qin, W., Qi, J., & Wu, X. H. (2014). Photocatalytic property of Cu2+ doped Bi2O3 films under visible light prepared by the sol-gel method. Vacuum, 107, 204–207 https://doi.org/10.1016/j.vacuum.2014.02.003. https://www.sciencedirect.com/science/article/pii/S0042207X14000554
- Rahmayanti, H. D., Sulhadi, S., & Aji M.P, 2015. Synthesis of Sulfur-Doped Carbon Dots by Simple Heating Method. Advanced Materials Research 1123 , (Scientific.Net 233–236 https://www.scientific.net/AMR.1123.233 https://doi.org/10.4028/www.scientific.net/AMR.1123.233).
- Raizada, P., Kumari, J., Shandilya, P., & Singh, P. (2017). Kinetics of photocatalytic mineralization of oxytetracycline and ampicillin using activated carbon supported ZnO/ZnWO4 nanocomposite in simulated waste water. Desalin Water Treatment, 79(79), 204–213. https://doi.org/10.5004/dwt.2017.20831
- Rissa, L. V., Priatmoko, S., & Harjito H. (2012). Sintesis lapis tipis berbasis nanopartikel titania termodifikasi silika secara sol-gel sebagai bahan antifogging. Indonesian Journal of Mathematics and Natural Sciences, 35(1), 57–65 https://journal.unnes.ac.id/nju/index.php/JM/article/view/2097/2210.
- Saravanan, R., Karthikeyan, N., Gupta, V. K., Thirumal, E., Thangadurai, P., Narayanan, V., & Stephen, A. (2013). ZnO/Ag nanocomposite: An efficient catalyst for degradation studies of textile effluents under visible light. Material Science and Engineering C, 33(4), 2235–2244 https://doi.org/10.1016/j.msec.2013.01.046 https://www.sciencedirect.com/science/article/pii/S0928493113000593.
- Sharma, J., Vashishtha, M., & Shah, D. O. (2014). Crystallite size dependence on structural parameters ang photocatalytic activity of microemulsion mediated synthesized zno nanoparticles annealed at different temperatures. Global Journal of Science Frontier Research: B Chemistry 14 5 18–31 https://journalofscience.org/index.php/GJSFR/article/view/1326 .
- Sistesya, D., & Sutanto, H. (2013). Sifat optis lapisan ZnO:Ag yang dideposisi di atas substrat kaca menggunakan metode chemical solution deposition (CSD) dan aplikasinya pada degradasi zat warna methylene blue. Youngster Physics Journal, 2(3), 71–80 https://ejournal3.undip.ac.id/index.php/bfd/article/view/3154.
- Sudrajat, H., Hartuti, S., & Park, J. (2018). A newly constructed photoactive system, Fe(III)-C/N-Bi2O3, for efficient visible light photocatalysis. Journal of Alloys and Compounds, 748, 390–397 https://doi.org/10.1016/j.jallcom.2018.03.149. https://www.sciencedirect.com/science/article/pii/S092583881831017X
- Suryanarayana, C. M. G. N. (1998). X-Ray diffraction A practical approach. Plenum.
- Sutanto, H., Alkian, I., Mukholit, M., Nugraha, A.A., Hidayanto, E., Marhaendrajaya, I., Priyono, P. (2021 Analysis of Fe-doped ZnO thin films for degradation of rhodamine b, methylene blue, and Escherichia coli under visible light). Materials Research Express, 8 (11) . 1–10 https://iopscience.iop.org/article/10.1088/2053-1591/ac33fe/meta https://doi.org/10.1088/2053-1591/ac33fe .
- Wang, Q., Wang, W., Zhong, L., Liu, D., Cao, X., & Cul, F. (2018). Oxygen vacancy-rich 2D/2D BiOCl-g-C3N4 ultrathin heterostructure nanosheets for enhanced visible-light-driven photocatalytic activity in environmental remediation. Applied Catalysis B Environmental, 220, 290–302 https://doi.org/10.1016/j.apcatb.2017.08.049. https://www.sciencedirect.com/science/article/pii/S0926337317307853
- Wang, X., Yin, R., Zeng, L., & Zhu, M. A. (2019). Review of graphene-based nanomaterials for removal of antibiotics from aqueous environments. Environmental Pollution, 253, 100–110 https://doi.org/10.1016/j.envpol.2019.06.067. https://www.sciencedirect.com/science/article/pii/S0269749119314216
- Xie, Y., Zhang, C., Wang, D., Lu, J., Wang, Y., Wang, J., Zhang, L., & Zhang, R. (2019). Catalytic performance of a Bi2O3–Fe2O3 system in soot combustion. New Journal of Chemistry, 43(38), 15368–15374. https://doi.org/10.1039/C9NJ03419F
- Yuwita, P. E., Ardianti, A. D., Mas’udah, K. W., & Darminto D., 2019. Structural, optical, and magnetic properties of Mn-doped ZnO nanoparticles synthesized by coprecipitation method. IOP Conferences Series: Materials Science Engineering 515 (IOP Publishing)1–6 https://iopscience.iop.org/article/10.1088/1757-899X/515/1/012065/pdf doi:10.1088/1757-899X/515/1/012065 .
- Zhang, F., Li, Y.-H., Li, J.-Y., Tang, Z.-R., & Xu, Y.-J. (2019). 3D graphene-based gel photocatalysts for environmental pollutants degradation. Environmental Pollution, 253, 365–376 https://doi.org/10.1016/j.envpol.2019.06.089. https://www.sciencedirect.com/science/article/pii/S026974911931591X
- Zhou, W., Sun, S., Jiang, Y., Zhang, M., Lawan, I., Fernando, G. F., Wang, L., & Yuan, Z. (2019). Template in situ synthesis of flower-like BiOBr/microcystalline cellulose composite with highly visible-light photocatalytic activity. Cellulose, 26(18), 9529–9541. https://doi.org/10.1007/s10570-019-02722-4
- Zhu, G. Q., Que, W. X., & Zhang, J. (2011). Synthesis and photocatalytic performance of Ag-loaded -Bi2O3 microspheres under visible light irradiation. Alloys Compound, 509(39), 9479–9486. https://doi.org/10.1016/j.jallcom.2011.07.046