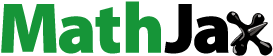
Abstract
This work examined the effect of extraction regime (hot water vs. simulated in vitro gastrointestinal digestion) and postharvest storage on the chemical composition, molecular weight, and bioactive properties of polysaccharides obtained from sweet cherries (Lapins and Staccato varieties) and apples (Gala and Fuji varieties). The yields of the polysaccharides isolated from cherries and apples ranged from 0.2 to 2.4% on a dry weight fruit basis. All of the isolated polysaccharides contained protein, phenolic compounds, and uronic acid. All polysaccharides contained the sugar monomers: rhamnose, arabinose, xylose, mannose, galactose, and glucose. Also, all of the polysaccharides obtained using the different extraction regimes were shown to possess antioxidant activity as determined with the ferric reducing antioxidant power and the 2,2-azinobis (3-ethyl-benzothiazoline-6-sulfonic acid) (ABTS) radical scavenging assays. Only the polysaccharides isolated from the cherry and apples after simulated in vitro gastrointestinal digestion showed appreciable α-glucosidase inhibition activity, with polysaccharides obtained from Staccato cherries showing nearly 90% of the inhibition of α-glucosidase as achieved with the positive control acarbose. This work shows that bioactive polysaccharides are available and/or can be isolated during digestion which supports the concept that fruit polysaccharides play a role in enhancing human health.
Public Interest Statement
This work strived demonstrate whether the health benefits associated with dietary consumption of fruits can be attributed to polysaccharides, and whether physiologically active polysaccharides can be isolated during digestion and thereby provide health- promoting effects. This work evaluated potential structure-dependent differences in bioactivity as influenced by fruit type, variety, storage, and extraction regime. The information generated from this type of study supports the concept that polysaccharides present in fruit may play a role in the health benefits associated with the consumption of fruits.
Competing interests
The authors declare no competing interest.
1. Introduction
Plant polysaccharides have been reported to display antioxidant, immunomodulating, anti-inflammatory, antidiabetic, and even anticancer activities (Fan, Mazza, & Liao, Citation2010; Gan, Hua Zhang, Liang Yang, & Bi Xu, Citation2004; Li, Li, & Zhou, Citation2007; Lui & Lin, Citation2012; Luo, Cai, Yan, Sun, & Corke, Citation2004; Ovodova et al., Citation2009; Popov et al., Citation2011; Xie, Wu, Mehendale, Aung, & Yuan, Citation2004). In fact, the antioxidant activity of water-extractable polysaccharides from cherry, cranberry, and kiwi fruits has been reported (Fan et al., Citation2010) and work by Ross, Siow, et al. (Citation2015) and Ross, Godfrey, and Fukumoto (Citation2015) has examined the antioxidant, immunomodulating, cardioprotective, and α-glucosidase inhibition properties of water-extractable polysaccharides isolated from cherry, raspberry and ginseng berry fruit, yet the effects of gastrointestinal digestion and postharvest storage on the chemical structure and bioactivity of these polysaccharides have not been examined.
As such, chemical-based in vitro activities can only be considered potentially relevant in biological systems as in vivo activities depend also on bioaccessibility, bioavailability, and biotransformation (Mateos-Aparicio, Mateos-Peinado, Jiménez-Escrig, & Rupérez, Citation2010). It is therefore relevant to study the bioactivity of polysaccharides under in vivo conditions or under in vitro conditions of simulated digestion. Simulated digestive processes using a series of buffers and enzymes to simulate the salivary phase, gastric phase, and small intestinal phase have been used to assess changes in biomolecules during digestion (Fogliano et al., Citation2011; Ovodova et al., Citation2009; Popov et al., Citation2011). A pectic polysaccharide isolated from sweet pepper using a simulated gastric medium has been reported to possess anti-inflammatory activity (Popov et al., Citation2011).
Postharvest storage and handling of fruits is an important step in the value chain and therefore any chemical changes that occur in the fresh product during handling and storage must be characterized with respect to bioactivity as this is the state that the fruit will likely be consumed. In commerce, cherries and apples are often stored in low temperatures for extended time periods prior to sale and consumption (Goliáš, Hic, & Kanová, Citation2010; Stebbins, Olsen, & Bluhm, Citation1998). For sweet cherries, phenolics content and antioxidant activity levels have been reported to be influenced by cultivar and storage conditions (Wani, Singh, Gul, Wani, & Langowski, Citation2014). Also it was reported that for certain apple varieties, two or more months of postharvest storage resulted in higher total phenolics content (Adyanthaya, Kwon, Apostolidis, & Shetty, Citation2010). With respect to polysaccharides, it is well known that changes in apple (Billy et al., Citation2008) and cherry (Batisse, Buret, & Coulomb, Citation1996; Batisse, Fils-Lycaon, & Buret, Citation1994) cell wall polysaccharides occur upon storage which are manifested through textural and quality changes. Billy et al. (Citation2008) reported that certain varieties of apples displayed increased total neutral sugar content in the polysaccharides and increased levels of galacturonic acid in the pectin fractions upon storage. Cherries have been shown to display changes in neutral sugars and galacturonic acid content, upon softening (Batisse et al., Citation1994, Citation1996). Changes in cell wall polysaccharides have not been studied with respect to bioactivity. Also, a long held belief is that plant cell wall polysaccharides are resistant to digestion by human enzymes in the stomach and small intestine and are delivered to the colon/large intestine in a chemically unaltered state (Carnachan, Bootten, Mishra, Monro, & Sims, Citation2012). It is important to examine the chemical changes that occur in the cell wall polysaccharides that have been subjected to in vitro digestion and report on how digestion may impact bioactivity (Ovodova et al., Citation2009; Popov et al., Citation2011).
Polysaccharides may play an important role as dietary free radical scavengers for preventing oxidative damage and should be explored novel antioxidants (Dou, Meng, Liu, Li, & Ren, Citation2015). Also control of postprandial hyperglycemia by retarding absorption of glucose by inhibiting the carbohydrate-hydrolyzing enzymes, such α-amylase and α-glucosidase enzymes has been suggested to be important in the treatment of diabetes (Chen, Qu, Fu, Dong, & Zhang, Citation2009). Many efforts have been made to search for effective and safe α-amylase and α-glucosidase inhibitors from natural materials (Chen et al., Citation2009; Palanuvej, Hokputsa, Tunsaringkarn, & Ruangrungsi, Citation2009; Zhang et al., Citation2010).
This work examined the effect of postharvest storage and extraction regime (water vs. simulated in vitro gastrointestinal digestion) on the chemical characteristics and bioactivities (antioxidant activity, α-amylase, and α-glucosidase inhibitory activities) of polysaccharides obtained from two cherry varieties (Lapins and Staccato) and two apple varieties (Gala and Fuji). The polysaccharides studied were (1) isolated using a water-based extraction; (2) isolated using a water-based extraction followed by in vitro gastrointestinal digestion; and (3) isolated after in vitro gastrointestinal digestion of the fruit. As such, the objectives of this work were to: (1) determine the influence of fruit type, variety, and postharvest storage on the bioactivities of polysaccharides, (2) determine whether polysaccharides with bioactivity (antioxidant and α-amylase, and α-glucosidase inhibitory activities) are available and/or can be isolated during digestion, and (3) evaluate potential structure-dependent differences in bioactivity as influenced by fruit type, variety, storage, and extraction regime.
2. Materials and methods
2.1. Samples
Commercially mature sweet cherry (Prunus avium L.) Lapins and Staccato variety and apple (Malus domestica) Gala and Fuji variety samples were obtained from cooperating growers in the Okanagan region of BC. Upon receipt of the fresh sweet cherry (150 kg) and apple (240 kg) samples approximately half of each variety of the cherry samples (75 kg) and approximately two thirds (160 kg) of each apple variety were put into storage. The remaining fresh/unstored samples were processed, in terms of destemming and pitting for the cherries; and coring, peeling, and slicing for the apples.
The storage condition for the cherry samples was cold storage at 0.5°C (32.9 F) for 2 weeks. The apples samples were tested at two storage time points: 8 and 17 weeks. The storage condition for the apple samples stored for 8 weeks was cold storage at 0.5°C and 90% RH. The storage condition for the apple samples stored for 17 weeks was under controlled atmosphere cold storage at 0.5°C, 95% RH, and a gas composition of 1% O2 and 1% CO2. When study samples reached the end time-point for the storage, the stored samples were processed in the same manner as described for the fresh/unstored samples.
The processed samples were freeze dried (Virtis Freeze Dryer, Model 50-SRC-5, Gardiner, NY), placed in polyethylene bags and stored at −20°C (−4 F) until being subjected to water extraction and simulated gastrointestinal digestion experiments. As such, all extractions and simulated in vitro digestions were performed on freeze-dried fruit. All chemicals for analyses, unless explicitly stated otherwise, were obtained from Sigma-Aldrich Co. (St. Louis, MO).
2.2. Extraction and purification of water-soluble fruit polysaccharides
Water-soluble polysaccharides (SPS) were extracted from the fruits samples using methods adapted from the works of He et al. (Citation2013), Ross, Siow, et al. (Citation2015), Wu, Cui, Tang, Wang, and Gu (Citation2007), and Yang, Zhao, Wang, Wang, and Mei (Citation2005). Due to the large amounts of fruit being subjected to extraction and purification, modifications in the solid–liquid separation steps were necessary as centrifugation was not feasible due to laboratory capacity restraints. A filtration method based on work of He et al. (Citation2013) was employed. Figure shows the extraction scheme for obtaining water SPS from sweet cherry and apple samples. The extraction and purification of water-soluble fruit polysaccharides is an important aspect of their characterization and therefore the method is given in detail. Briefly, the freeze-dried samples were ground to a fine powder with a hand blender. The ground fruit samples were first subjected to an 80% ethanol pre-extraction step for 1 h to remove simple sugars, phenolics, and other low molecular weight compounds. As such, a 1:5 solid-to-solvent ratio was used for the 1st ethanol extraction. The solids were separated from the ethanol solution by filtration through a nylon filter bag (25-μm pore size, 76-cm length × 18-cm diameter). The mixture was placed in a nylon filter bag and the ethanol solubles were expressed using a 40-L bladder press (Hydropress, Bosa Grape Winery Supplies, Burnaby, BC). The solid/retentate was re-extracted two more times with 80% ethanol at a 1:2.5 solid-to-solvent ratio. The solids were separated as noted for the 1st ethanol extraction. The solids/retentate was dried overnight in vacuum oven at 60°C (140 F). The ethanol extracted-fruit residue was ground using a hammer mill (Ika, Wilmington, NC) equipped with a 2-mm screen and subjected to water extraction.
For the water extraction step, the ethanol-extracted fruit ground residue was added to water in a 1:20 solid-to-solvent ratio and stirred with a mixer, (Lightnin, Rochester, NY) at for 2 h at 70°C (158 F). Filtration was used to separate the solid residue from the water solution. The mixture was placed in a nylon filter bag (55-μm pore size, 76-cm length × 18-cm diameter) and the water solubles were expressed using a 40-L bladder press (Hydropress, Bosa Grape Winery Supplies, Burnaby, BC). Both the filtrate and solid residue/retentate from the 1st warm water extraction were collected. The collected solid residue was subjected to another 70°C water extraction for 2 h using a 1:20 sample-to-solvent ratio (i.e. the first extraction step was repeated). Filtration as described for the first hot water extraction was again used to separate the solids from the water extraction solvent. Both the filtrate and solid residue from the 2nd warm water extraction were collected. The filtrates of the 1st and 2nd warm water extractions were pooled. Due to large volumes (~20 L), the pooled aqueous filtrates were subjected to freeze drying to remove the water. The water-soluble freeze-dried solid material was dissolved in 80% ethanol at a 1:75 (solids:ethanol) ratio in 20-L plastic pail and was mixed for at least 3 h with a mixer (Lightnin, Rochester, NY). The mixture was kept overnight at 4°C (39.2 F) to precipitate the polysaccharides. Filtration through nylon filter bags (25 μm pore size, 76 cm length × 18 cm diameter) was used to separate the insoluble polysaccharide (IPS) precipitate from the 80% ethanol solvent. The solid residue/retentate was collected as water-extractable crude polysaccharide (CPS) product and dried in a vacuum oven overnight at 60°C. It was noted that the CPS was extracted using a water solvent that was at a temperature higher (70°C) than the temperature of test solutions used for chemical characterization and bioactivity determination. To remove substances potentially insoluble at lower temperatures and thereby ensure solubility at conditions (temperature and concentrations) used for testing, the CPS product was dissolved in deionized distilled water at a concentration of 10 mg/mL and the solution was covered and stirred for 16–24 h. After stirring, the solution was filtered through a nylon filter bag (55-μm pore size, 76-cm length × 18-cm diameter). Both the filtrate and solid residue/retentate were collected. The retentate was gel-like (i.e. not solid) and was termed the IPS product. The filtrate was freeze dried to obtain the water SPS product.
In accordance with He et al. (Citation2013) and Yang et al. (Citation2005), the SPS product was subjected to a freeze-thaw process to remove any free protein that may have been associated with the water SPS. Eight grams of the SPS product were redissolved in 800 mL of distilled water and was mixed for 16 h to ensure dissolution. The solution was poured into a medium sized resealable plastic bag and was frozen on a tray at −20°C. After freezing for 24 h, the bags were removed and thawed for 24 h. This freeze-thaw process was performed 10 times. Upon the final thawing stage, the aqueous SPS solution was filtered. The solution was filtered through 55 um nylon filter sheet fitted to a Buchner funnel under vacuum. The retentate/residue contains free proteins that were removed from the freeze-thaw process. The filtrate containing the purified water-soluble polysaccharides (PWSP) solution was freeze dried. After freeze drying, the PWSP was vacuum dried overnight at 60°C to ensure complete dryness. This procedure is in agreement with the method described by He et al. (Citation2013) and Wu et al. (Citation2007). The yield of the PWSP was determined by weighing the product amount. The portion of the PWPS product that was intended for chemical characterization and bioactivity testing was subsampled and cryomilled for 1.5 min (Cryomill, SpexCerti Prep, Model 6800-115) to obtained a product with uniform particle size. After cryomilling, the samples were placed in polyethylene bags and stored at −20°C until chemical and bioactivity analyses.
2.3. In vitro digestion studies
The simulated in vitro gastrointestinal digestion experiments were performed by following the method of Luten et al. (Citation1996) and subsequent extraction of the polysaccharides from the digestate was performed using the method of Carnachan et al. (Citation2012) and are described below.
2.3.1. In vitro digestion: gastric and small intestinal digestions
Freeze-dried fruit samples were weighed out and added into 2-L Erlenmeyer flasks. Specifically, 125 g of the Lapins or Staccato sweet cherries at both storage conditions, 125 g of the Gala or Fuji apples at all storage conditions, and 3 g of water-extractable polysaccharides from the Lapins sweet cherries and Gala apples were used for the in vitro digestions. Due to laboratory constraints, only the water-extractable polysaccharides obtained from the Lapins sweet cherries and Gala apples were subjected to simulated in vitro digestion. One liter of ultrapure milliQ water was added and the mixture was stirred to ensure sample was dissolved. Six molar HCl was added to lower pH to 2, and if necessary was readjusted after 15 min prior to adding 37.5 mL of pepsin solution. Pepsin solution was prepared using 16 g of pepsin from porcine gastric mucosa in 100 mL of 0.1-M HCl and stirred until a homogenous mixture was formed. 0.1 M HCl solution was prepared using 16 mL of hydrochloric acid 37% and 1.984 L of ultrapure milliQ water. Ultrapure milliQ water was added to achieve a final total weight of 1250 g of sample mixture before being placed into a 37°C (98.6 F) water bath and shaken for 2 h. Flasks were then removed from the water bath. Sodium bicarbonate (NaHCO3) was used to adjust pH to 7.5, with readjustment after 30 min if necessary. After the pH had stabilized, 312.5 mL of pancreatic mixture was added to each duplicate, and placed into a 37°C shaking water bath for 2 h. Pancreatic mixture was prepared using 2.8 g of pancreatin from porcine pancreas and 17.5 g of bile extract porcine in 700 mL of 0.1 NaHCO3 solution. 0.1 M NaHCO3 solution was prepared using 16.8 g of sodium bicarbonate dissolved in 2 L of ultrapure milliQ water. Due to time constraints, samples were removed from the water bath and placed in 4°C storage overnight. The digestions were performed in duplicate for all samples.
2.3.2. In vitro digestion: separation of soluble and insoluble components in digest mixture
Samples were removed from 4°C storage and centrifuged for 20 min at 9000 RPM (Sorvall RC 5B Plus Centrifuge). The soluble components of the digest mixture were separated by filtration using a Buchner funnel and Whatman 4 filter paper. After filtration, soluble fraction (i.e. the filtrate) was retained for 80% ethanol extraction of polysaccharides. The volume of soluble fraction was determined, and 100% ethanol was added to the soluble fraction to create a final concentration of 80% ethanol. A mixer (Lightnin, Rochester, NY) was used to continuously stir the ethanol mixture. The mixture was cooled and stored overnight at 4°C to complete polysaccharide precipitation.
2.3.3. In vitro digestion: extraction of digested SPS
Samples were removed from cold storage and separated to retain solid polysaccharide fraction using gravity filtration. A 25-μm nylon filter was used to separate the sample, liquid fraction was discarded as waste, and solid fraction was dissolved in minimal amounts of ultrapure milliQ water. Samples were frozen at −20°C until dialysis. Figure shows the scheme of in vitro digestion and isolation of SPS from sweet cherries, apple fruits, and corresponding water-extracted polysaccharides
2.3.4. In vitro digestion: dialysis of digested SPS
Prior to dialysis, samples were removed from −20°C and allowed to thaw for 4 h. Sample was added to dialysis tubing (Spectra/Por MWCO: 1000 Da, (volume/length requirement: 6.4 mL/cm). Water changes were performed during dialysis procedure, with conductivity measurements performed using a conductivity meter and probe (sympHony SP80PC) which assisted in determination of dialysis end point. Post dialysis samples were pooled back together into a plastic bag and frozen at −20°C. These samples were then freeze dried (Virtis Freeze Dryer, Model 50-SRC-5, Gardiner, NY)) cryomilled for 1.5 min (Cryomill, SpexCerti Prep, Model 6800-115) to obtained a product with uniform particle size. After cryomilling, the samples were placed in containers and stored at −20°C until chemical and bioactivity analyses.
2.4. Chemical analyses
2.4.1. Carbohydrate content and uronic acid determination
As described by Ross, Siow, et al. (Citation2015) and Ross, Godfrey, et al. (Citation2015), total carbohydrates in the isolated polysaccharides were assayed with the phenol–sulfuric acid method of DuBois, Gilles, Hamilton, Rebers, and Smith (Citation1956) using glucose as a standard. Uronic acid content of the isolated polysaccharides was quantified by the Scott (Citation1979) method as described by Ross, Siow, et al. (Citation2015) and Ross, Godfrey, et al. (Citation2015). The uronic acid (UA) content was determined by the difference in absorbance at 400 and 450 nm and comparing it to a standard curve of galacturonic acid. Experiments were performed in duplicate and results are expressed on a dry weight basis.
2.4.2. Neutral sugars monomer content determination
As described by Ross, Siow, et al. (Citation2015) and Ross, Godfrey, et al. (Citation2015), the neutral sugar monomer content of the polysaccharide samples was determined using the methods of Fan et al. (Citation2010) and Blakeney, Harris, Henry, and Stone (Citation1983). The samples were first hydrolyzed with 1-M H2SO4 at 100°C (212 F) for 3 h in a convection laboratory oven into monosaccharides before derivitization into alditol acetates. The alditol acetate derivatives were analyzed via gas–liquid chromatography on an Agilent DB-225 glass-capillary column (30 m × 0.32 mm, i.d., film: 0.25 μm) in an Agilent 6890 N Network GC System (Agilent Technologies, Mississauga, ON), equipped with a FID detector. Different concentrations of neutral sugar standard mixtures (rhamnose, fucose, arabinose, xylose, mannose, galactose, and glucose) were prepared, converted to their derivatives, and analyzed. Sugar concentrations were calculated as the anhydro sugars relative to myo-inositol (used as an internal standard). Experiments were performed in duplicate and results are expressed on a dry weight basis.
2.4.3. Protein content determination
Protein content was estimated from the nitrogen content of the polysaccharide samples using the method of Tamaki and Mazza (Citation2010) as described by Ross, Siow, et al. (Citation2015) and Ross, Godfrey, et al. (Citation2015). Briefly, nitrogen content was determined by combusting the dried samples at 850°C (1562 F) using a Leco FP-528 nitrogen analyzer (Leco Corporation, St. Joseph, MI). Approximately 100 mg of a sample was analyzed. A standard curve for nitrogen was produced using ethylenediaminetetraacetic acid and corn flour (Leco Corporation, St. Joseph, MI). Protein content was estimated by multiplying the nitrogen content (%) by a factor of 6.25. Experiments were performed in duplicate and results are expressed on a dry weight basis.
2.4.4. Total phenolics
As described by Ross, Siow, et al. (Citation2015) and Ross, Godfrey, et al. (Citation2015), the total phenolics content of the polysaccharide samples was determined by the Folin–Ciocalteu colorimetric method based on the procedure described by Singleton and Rossi (Citation1965). Quantification was determined based on a standard curve for gallic acid by measuring absorbance at 765 nm on a spectrophotometer (Cary 50, Agilent Technologies, Mississauga, ON). The total amount of phenolic content was expressed at mg gallic acid equivalent per gram polysaccharide sample (mg GAE/g polysaccharide sample)
2.4.5. Molecular weight determination
The molecular weight of the polysaccharides was determined with high-performance size exclusion chromatography (HPSEC) following the method described by Ross, Siow, et al. (Citation2015) and Ross, Godfrey, et al. (Citation2015). In accordance with the method of Carnachan et al. (Citation2012), molecular weights of polysaccharides were estimated by comparison of the retention/elution times with those of the dextran and glucose standards by use of a standard curve. The standard curve represented the linear relationship of retention time and the logarithm of the respective molecular weights (log MW) of the standards. In order to ensure the strongest linear relationship (i.e. R 2 value), a standard curve containing the larger molecular weights ranging from 6300 to 43 kDa (R 2 = ~0.99) and the smaller molecular weights ranging from 43 to 0.18 kDa (R 2 = ~0.98) were used.
2.5. Bioactivity tests
2.5.1. Antioxidant activity–chemical in vitro assays
The antioxidant activity of the polysaccharides in terms of (1) reducing power toward Fe(III) and the ability to act as a radical scavenging compound was investigated by the Ferric Reducing Antioxidant Power (FRAP) assay and the ABTS•+ radical scavenging assay, respectively. The methods were provided by Ross, Siow, et al. (Citation2015) and Ross, Godfrey, et al. (Citation2015), briefly, for both of these assays, aqueous solutions of the polysaccharide samples were prepared as follows: an aliquot (5 mg) of each polysaccharide sample was placed in a 2-mL polypropylene centrifuge tube; 1 mL of Ultrapure water containing ethanol (10%, v/v) was added, and the tube was vortexed at room temperature to ensure adequate mixing. The samples were then centrifuged at 13,400 rpm for 5 min in Eppendorf Minispin (Eppendorf, Mississauga, ON), and the supernatant was recovered for testing antioxidant activity. The supernatant of each aqueous polysaccharide supernatant was serially diluted for use in the antioxidant assays.
2.5.1.1. ABTS•+ radical scavenging activity
The radical scavenging activity of the polysaccharides was determined using the ABTS (2,2′-azino-bis(3-ethylbenzothiazoline-6-sulphonic acid) decolourization assay as described by Mateos-Aparicio et al. (Citation2010). For analysis, 50 μL of the aqueous polysaccharide supernatant was added to a well on a 96 well plate. To each polysaccharide sample in each well, 250 μL of the diluted ABTS•+ solution was added. After incubating for 5 min at room temperature, the absorbance at 734 nm was measured. A plate reader (SpectraMax M2, Molecular Devices, Sunnyvale, CA) was used to measure spectrophotometric absorbance. Absorbance was measured for each sample in triplicate for each dilution. The results are expressed as Trolox equivalents (TE)/g polysaccharide on a dry weight basis.
2.5.1.2. Ferric reducing antioxidant power
The antioxidant activity, in terms of FRAP, of the polysaccharides was evaluated using the method of Mateos-Aparicio et al. (Citation2010). For analysis, 50 μL of the aqueous polysaccharide supernatant was added to a well on a 96 well plate. To each polysaccharide sample in each well, 250 μL of FRAP was added. After incubating at 5 min at room temperature, the absorbance at 593 nm was measured. A plate reader (SpectraMax M2, Molecular Devices, Sunnyvale, CA) was used to measure spectrophotometric absorbance. Absorbance was measured for each sample in triplicate for each dilution. The results are expressed as Trolox equivalent (TE)/g polysaccharide on a dry weight basis.
2.5.2. Assay for determination of inhibition of α-amylase activity
The ability of the polysaccharides to inhibit α-amylase was determined using an assay based on the hydrolysis of 2-chloro-4-nitrophenol-α-D-maltotrioside (CNPG3) by α-amylase (Kumanan, Manimaran, Saleemulla, Dhanabal, & Nanjan, Citation2010). The principle of this method is the action of α-amylase hydrolyzing CNPG3 to release 2-chloro-4-nitrophenol and form 2-chloro-4-nitrophenol-α-D-maltoside (CNPG2), maltotriose, and glucose. The formation of 2-choloro-4-nitrophenal can be determined by measuring absorbance at 405 nm and this absorbance is an indicator of α-amylase activity. The assay was performed following the method of Kumanan et al. (Citation2010) with some modifications as described by Ross, Godfrey, et al. (Citation2015). Absorbance at 405 nm was measured for each sample in triplicate for each sample. A blank reaction was used without the test sample. Acarbose was used as the positive control inhibitor.
2.5.3. Assay for determination of inhibition of α-glucosidase activity
The ability of the water SPS and purified fractions to inhibit α-glucosidase activity was assayed using p-nitrophenyl-α-D-glucopyranoside (pNPG) as the substrate, which is hydrolyzed by α-glucosidase to release p-nitrophenol. The formation of p-nitrophenol can be determined by measuring absorbance at 405 nm and this absorbance is an indicator of α-glucosidase activity (Zhang et al., Citation2010). The inhibition assay for α-glucosidase activity was performed based on the method of Zhang et al. (Citation2010) and Wu et al. (Citation2012) with modifications as described by Ross, Godfrey, et al. (Citation2015). Absorbance at 405 nm was measured for each sample in triplicate for each sample. A blank reaction was used without the test sample. Acarbose was used as the positive control inhibitor.
As in the work of Ross, Godfrey, et al. (Citation2015), for both α-amylase and α-glucosidase activities, the calculation of results is presented as:
For both α-amylase and α-glucosidase activities, the overall results are expressed as Relative Inhibition (%) which is the inhibition provided by the sample compared to the inhibition provided by 5 mg/mL acarbose. As in the work of Ross, Godfrey, et al. (Citation2015), the calculation of these results is presented as:
2.6. Statistical analysis
Statistical analysis was conducted using SAS Institute Inc. Software, version 9.1. Data were subjected to analysis of variance with replication using the SAS PROC GLM procedure. Least square means and least significant difference at 5% significance level were generated using the SAS PROC GLM procedure.
3. Results and discussion
3.1. Characterization of water-soluble and soluble digested polysaccharides
The bioactivities of polysaccharides can be affected by many factors including chemical components, molecular weight, extraction and isolation methods (Li et al., Citation2010; Mateos-Aparicio et al., Citation2010). Tables and provide chemical characterization data and Table provides bioactivities characterization data for all of the polysaccharide samples. A discussion linking the chemical characteristics of the Lapins and Staccato sweet cherry and Gala and Fuji apple polysaccharides obtained with different extraction regimes with the measured bioactivities will be provided in relation to the bioactivities assayed in this work, in Sections 3.2 and 3.3. Table shows the yield of the PWSP extracted from sweet cherry and apple at the various storage levels, water-extracted polysaccharides subjected to simulated gastrointestinal digestion (SDWSP), and digested SPS isolated after simulated gastrointestinal digestion (SDP) of sweet cherry and apple at the various storage levels. The yields ranged from ~0.2 to 2.4% (dry matter basis). The highest yields (~1.2–2.4%) were observed with the polysaccharides isolated after simulated gastrointestinal digestion of the fruit samples with the highest yield obtained from fresh Gala apples. The PWSP yields ranged from 0.68 to 1.6%. These results are in agreement with the work of Carnachan et al. (Citation2012) which found that simulated in vitro gastrointestinal digestion of kiwi fruit produced higher yields of SPS vs. water extraction. The polysaccharides isolated after subjecting the water-extracted polysaccharides to simulated in vitro gastrointestinal digestion showed the lowest yields ~0.2%. The yield of polysaccharides extracted with cold water from prunes was reported to be 0.5% (Cantu-Jungles et al., Citation2014) and the yield of water-extractable polysaccharides from sweet cherries, raspberries, and ginseng berry pulp has been reported to range from 0.5 to 2.18% (Ross, Godfrey, et al., Citation2015; Ross, Siow, et al., Citation2015). Popov et al. (Citation2011) isolated polysaccharides from sweet bell peppers using a simulated in vitro gastric digestion protocol and obtained a yield of 0.35% (dry matter basis). The values reported in the present work are in agreement of those reported in the literature.
Table 1. Yield, total carbohydrates, protein, total phenolics contents, and molecular weights of water-extracted polysaccharides, water-extracted polysaccharides subjected to simulated gastrointestinal digestion and polysaccharides obtained after simulated in vitro gastrointestinal digestion of sweet cherries and apples
Table 2. Neutral sugars, uronic acid, and sugar monomer composition of water-extracted polysaccharides, water-extracted polysaccharides subjected to simulated gastrointestinal digestion and polysaccharides obtained after simulated in vitro gastrointestinal digestion of sweet cherries and apples
Table 3. Bioactivities of water-extracted polysaccharides, water-extracted polysaccharides subjected to simulated gastrointestinal digestion and polysaccharides obtained after simulated in vitro gastrointestinal digestion of sweet cherries and apples
The total carbohydrate content of the polysaccharides isolated from all of the fruit samples ranged from ~16 to 37% (Table ). All of the polysaccharides obtained from the cherry samples exhibited lower carbohydrate contents ranging from ~28 to 36% compared to the polysaccharides obtained from the apple samples which possessed carbohydrate contents ranging from ~16 to 28%. For the both cherry varieties and Fuji apple samples, there was no effect of storage on carbohydrate content of the polysaccharides obtained from the various extraction regimes. For the polysaccharides obtained from Gala apples, there was an observed effect of storage on carbohydrate content. The PWSP obtained from Gala apples showed an increase in carbohydrate content upon storage (~20–28%) while the SDP showed a decrease in carbohydrate content upon storage (~27–16%). It has been reported that the yield of water-soluble pectin from apples typically increases as the fruit softens postharvest (Billy et al., Citation2008; Knee, Citation1973). At common extraction condition and storage time, for the polysaccharides obtained from the cherry samples, variety did not significantly affect carbohydrate content while the polysaccharides obtained from the Fuji apple samples generally showed lower total carbohydrate content (~17–22%) than the polysaccharides obtained from the Gala apples (~16–28%). In terms of assessing the influence of extraction condition, the SDWSP obtained from the fresh Lapins cherries had a comparable carbohydrate content (~37%) to that of the SDP (~36%) obtained from the fresh Lapin cherries but higher than the PWSP (28%) obtained from the fresh Lapins cherries. The SDP (~27%) obtained from the fresh Gala apples showed higher carbohydrate content compared to SDWSP (~20%) and PWSP (~20%) samples. These results do show that the polysaccharides are not completely carbohydrate. The polysaccharides were conjugated protein moieties as the protein content of the polysaccharides isolated from all of the fruit samples ranged from ~3 to 37% (Table ). Protein content has been reported in the literature to influence the antioxidant activity of green tea (Chen, Zhang, Qu, & Xie, Citation2008). Generally, the polysaccharides obtained from the cherry samples exhibited higher protein contents (~16–37%) compared to the polysaccharides obtained from the apple samples (~3–19%). In most cases, there was no significant effect of storage on protein content. However, for the PWSP obtained from Staccato cherry samples stored for 2 weeks contained significantly more protein (~22%) than PWSP obtained from the fresh Staccato cherry samples (~16%). For the Lapins cherries, there was an effect of extraction regime on protein content as the SDWSP samples demonstrated the lowest protein content (~22%). The opposite result was observed for the polysaccharides obtained from the Staccato cherries as there was a decrease in protein content in the water-extracted PWSP samples. For both Gala and Fuji apples, the SDP samples showed higher protein contents compared to corresponding PWSP samples. For the apple polysaccharides, the Gala SDWSP sample displayed the highest protein content (~19%). The protein results for the polysaccharides obtained from the cherry samples are higher compared to those presented in the literature. Barbier and Thibaul (Citation1982) reported the protein content of water SPS obtained from cherries (cv. Bigarreaux Napoleon) to be 12.4%. The work of Ross, Siow, et al. (Citation2015) reported that the protein content of the CPS extracted with hot water from cherries ranged from ~6 to 12%. However, the protein content for the whole cherries used in this work ranged from 3 to 6% (dry mass basis, data not shown) while the protein content of the Lapins cherries used in the work of Ross, Siow, et al. (Citation2015) ranged was around 2.75% (dry mass basis, data not shown). It is reasonable that the polysaccharides in the current work may show higher protein levels as the fruit starting material possessed higher protein levels. The nutritional content of horticultural crops may be influenced by factors such as soil composition, climate, crop type, cultivar, cultural and management practices (Hornick, Citation2010) so this may account for differences in the reported values of protein content. Work performed by Kołodziejczyk et al. (Citation2009) noted that apple pomace contained ~2.6–6.4%, which is comparable to protein contents obtained for the polysaccharides isolated with hot water extraction. Yuliarti, Goh, Matia-Merino, Mawson, and Brennan (Citation2015) reported that the protein content of gold kiwifruit pectin polysaccharide ranged from ~9 to 30% depending on both fruit maturity and extraction method.
The polysaccharides isolated from all of the fruit samples were analyzed for total phenolics content and the results indicated (Table ) that the polysaccharides contained phenolics with total phenolics contents ranging from ~6 to 153 mg GAE/g polysaccharide. This work shows that phenolics are associated with the PWSP, water-extracted polysaccharides subjected to simulated in vitro gastrointestinal digestion and polysaccharides isolated after simulated in vitro gastrointestinal digestion of fresh and stored sweet cherries and apples. These phenolics represent non-extractable phenolics. Extractable phenolics are phenolic compounds present in either the extracts of food subjected to solvent extraction or gastrointestinal digestate, and non-extractable phenolics are phenolic compounds that remain unextracted for in the residues of solvent extracted materials or those not released during gastrointestinal digestion and remain associated with the food matrix (Ross, Citation2014; Saura-Calixto, Citation2011). The concept of non-extractable phenolics associated with polysaccharides is important in filling the gap in the field of dietary phenolics in terms of fully defining the bioactive- and health-related properties of phenolics (Ross, Citation2014; Saura-Calixto, Citation2011). Further, the importance of bioactive compounds (such as phenolics) has primarily focused on those that are absorbed through the gastrointestinal tract into the rest of the body (i.e. bioavailability of bioactive compounds) but more attention is being given to the health promoting aspect of exposure of the gastrointestinal tract to high levels of bioactive compounds (i.e. bioaccessibility of bioactive compounds) (Halliwell, Zhao, & Whiteman, Citation2000; Shimizu, Citation2010), which indicates the importance of the present work detailing the chemical structure and bioactivity of polysaccharides obtained after simulated in vitro gastrointestinal digestion. The intestinal epithelial cells of the small intestine form a monolayer covering the surface of the intestinal tract and are important modulators of intestinal function. These cells are constantly exposed to food components, and modulation of the intestinal functions by bioactive food components is essential in promoting health (Shimizu, Citation2010). In fact, Wang (Citation2011) demonstrated that a water SPS isolated from Chinese jujube was bioactive in terms of possessing antioxidant activity as these polysaccharides reduced oxidative damage on intestinal cell caused by ischemia and reperfusion. Assessing the bioactivity of complex polysaccharides such as those containing non-extractable phenolics and protein is important as it is essential for fully understanding the food components responsible for the demonstrated health benefits fruits. Based on the literature is it likely that the polysaccharide (Pawlaczyk et al., Citation2011; Wang, Citation2011), phenolic component (Saura-Calixto, Citation2012), and protein component (Chen et al., Citation2008) contribute to bioactivity. Further, the phenolics content of the polysaccharides reported in this work is in agreement with the phenolics content of the water-extractable CPS reported by Ross, Siow, et al. (Citation2015). Higher phenolics content values were associated with the SDP samples obtained from both cherry and apple samples. Generally, polysaccharides obtained from the cherry samples possessed higher phenolics content (~30–153 mg GAE/g polysaccharide) than the apple polysaccharides (~6–34 mg GAE/g polysaccharide). At common variety and extraction conditions, storage did have an effect on phenolics content. Both the PWSP and SDP samples obtained from the Lapins and Staccato cherries showed an increase in phenolics content upon storage. For both apple varieties, the phenolics content was only higher in the SDP samples upon storage. To our knowledge, no work has been reported on the phenolics content of apple and fruit polysaccharides, as influenced by cultivar and storage conditions. However, literature exists noting the influence of storage on the extractable phenolics content of sweet cherries (Wang & Long, Citation2014) and apples (Kevers, Pincemail, Tabart, Defraigne, & Dommes, Citation2011; Tarozzi, Marchesi, Cantell-Forti, & Hrelia, Citation2004). Our results were in agreement with the study of Gonçalves et al. (Citation2004) which reported levels of phenolics in sweet cherries increased during storage. Generally for the polysaccharides obtained from the cherry samples, there was no significant influence of variety on phenolics content. For the apple varieties tested, generally the PWSP samples showed no influence of variety. Phenolics content was generally higher in the Fuji SDP samples compared to the Gala SDP samples. Literature has shown that the extractable phenolics content of apple (no peel) to be around 192-mg chlorogenic acid/100 g fresh weight (Kevers et al., Citation2011) which is higher than the value phenolics content of the apple polysaccharides presented in this work. There was an effect of extraction condition on phenolics content as SDP samples showed higher phenolics content compared to PWSP samples. The phenolics content of the SDP isolated from the cherries and apples ranged from ~128 to 153 and ~20 to 34 mg GAE/g polysaccharide, respectively. The phenolics content of the PWSP isolated from the cherries and apples ranged from ~35 to 43 and ~6 to 9 mg GAE/g polysaccharide, respectively. The phenolics content of SDWSP isolated from the Lapins cherry and Gala apple samples (~44 and 8 mg GAE/g polysaccharide) was compared to phenolics levels measured for the corresponding PWSP samples (~38 and 9 mg GAE/g polysaccharide).
The molecular weight data contained in the HPSEC of the polysaccharides are presented in Table . Molecular weight of polysaccharides has been shown to affect antioxidant activity (Chen et al., Citation2008) and α-glucosidase activity (Chen et al., Citation2009). The molecular weights of the cherry and apple polysaccharides obtained with the various extraction regimes were associated with multimodal distributions from HPSEC results and spanned the entire molecular weight range of the standards, 6300–0.18 kDa. The molecular weight values presented represent the main molecular weight population residing in this class size, and values in parentheses represent the proportion of population associated with the reported molecular weight. For both cherry varieties, the PWSP samples showed a less homogenous size distribution as indicated by lower values of proportion of molecular weight population (~29–36%) compared to proportions ranging from ~75 to 99% observed in the other polysaccharide samples. The PWSP samples obtained from Lapins and Staccato cherries ranged from ~0.24 to 1.89 kDa while the PWSP samples obtained from the Gala and Fuji apples ranged from ~19 to 37 kDa. The SDP samples obtained from Lapins and Staccato cherries ranged from ~4 to 12 kDa from while the SDP samples obtained from Gala and Fuji apples ranged from ~120 to 190 kDa. In terms of effect of storage, only the SDP samples from the Gala apples showed a significant decrease in MW at longer term storage (17 weeks). The work of Gwanpua et al. (Citation2014) reported that significant changes in molecular weight of water-soluble apple polysaccharides were observed only in fruit that were stored under air storage for 6 months. In general, there was no influence of variety on MW, however the MW of the SDP polysaccharide obtained from the 17-week stored Fuji apples (~160 kDa) was significantly higher than the MW of the corresponding polysaccharide obtained from Gala apples (~120 kDa). The SDP samples obtained from the Gala and Fuji apples were significantly higher than corresponding PWSP polysaccharides. Subjecting the cherry and apple polysaccharides obtained from hot water extraction to simulated gastrointestinal in vitro digestion (i.e. SDWSP samples) did not show a significantly lower MW. These results are not in agreement with those of Carnachan et al. (Citation2012) which showed that the molecular weight of soluble kiwi fruit polysaccharides decreases upon simulated in vitro gastrointestinal digestion. It is possible that the lack of molecular weight reduction upon simulated in vitro gastrointestinal digestion was due to the presence of polysaccharide aggregates (Hu, Nie, Min, & Xie, Citation2013).
Table shows results for the neutral sugars content, UA content, and sugar monomer profiles of the polysaccharides obtained from the different fruit samples using different extraction conditions. Certain bioactivities have been related to neutral sugars content, sugar monomer composition, and UA content. Antioxidant activity has been directly correlated to the amount of total glucans content in polysaccharides (Kozarski et al., Citation2011). A direct relationship between the UA contents and the antioxidant activity of complex tea polysaccharides has been reported (Chen, Zhang, & Xie, Citation2004). Monosaccharide composition has been related to antioxidant ability (Tsiapali et al., Citation2001). Galactose present in polysaccharides isolated from mushroom has been shown to influence immunostimulatory activity (Lo, Jiang, Chao, & Chang, Citation2007). The neutral sugars content of the polysaccharides ranged from ~15 to 47% for all of the fruit samples, which as expected, were comparable to the values for total carbohydrates. The cherry SDP samples showed a small decrease in NS content upon storage. The SDP samples obtained from the fresh Gala apples demonstrated the highest NS content (~28%) compared to the other storage levels (~17–18%). This result was not observed for the SDP Fuji samples. There was an observed effect of variety on NS content in the PWSP samples obtained from the fresh Lapins and Staccato cherries. The PWSP samples from the Staccato cherries (~31%) had a higher NS content compared to the PWSP samples from the fresh Lapins cherries (~28%). The opposite trend was observed for the SDP samples obtained from the Staccato and Lapins cherries. The PWSP samples obtained from 4-week stored Gala apples contained a lower NS content (~15%) than the PWSP samples obtained from the 4-week stored Fuji apples (~18%) yet the opposite result was obtained for the PWSP samples obtained from the apples stored for 17 weeks. Also, the SDP samples from the fresh Gala apples exhibited a higher NS content (~28%) than corresponding SDP samples from Fuji apples (~19%). Extraction condition did affect NS content. The SDWSP sample obtained from the fresh Lapins cherries possessed the highest NS content (~47%). SDP samples isolated from the Lapins cherries and Gala and Fuji apples demonstrated higher NS contents (~28–31, ~17–28, and ~20–21%, respectively) than corresponding water-extracted polysaccharides (~27, ~15–25, and ~15–19%, respectively). The UA content of the polysaccharide samples ranged from ~2 to 60% (Table ). Generally, the apple polysaccharides (~30–59%) had higher UA content values than the cherry polysaccharides (~2–8%). The UA content of the SDP samples obtained from Gala apples showed an increase upon storage however there was generally more UA present in the PWSP samples obtained from the Fuji apples compared to the Gala apples. In terms of the effect of extraction regime on UA content of the polysaccharides, for the Lapins cherries the only observed difference in UA content was for the SDWSP sample (~7%) which exhibited a higher UA content compared to corresponding PWSP (~4%) and SDP samples (~2%). The SDP samples obtained from fresh Staccato cherries showed a lower UA (~2%) compared to corresponding PWSP sample (~8%). The UA content of SDP samples obtained from the Gala and Fuji apples (~30 and 21%, respectively) was also lower compared to corresponding PWSP samples (~51 and 19%, respectively). These results are the opposite of those reported by Carnachan et al. (Citation2012) which noted that the UA content of soluble digested polysaccharides from Kiwi fruit was more compared to water SPS.
The sugar monomer content of the polysaccharides obtained from all the fruit was presented (Table ). All polysaccharides contained rhamnose, arabinose, xylose, mannose, galactose, and glucose. The presence of rhamnose, galactose, and arabinose indicated that the polysaccharides were in the form of arabinans, galactans, or arabinogalactans while the presence of xylose, mannose, and glucose could indicate the presence of xyloglucans or could have been a result of the presence of hemicellulose and cellulose (Yuliarti et al., Citation2015). Generally, the main sugars found in the PWSP and SDWSP samples obtained from both cherry and apples varieties were arabinose, galactose, and glucose. The sugars present in the greatest amounts in the SDP samples from cherries were rhamnose, arabinose, galactose, and glucose; the sugars present in the greatest amounts in the SDP samples from apples were arabinose, galactose, and glucose. There was significantly more rhamnose and fucose in the SDP samples obtained from Staccato cherries (~20–21 and ~3%, respectively) vs. Lapins cherries (~15–17 and ~2%, respectively). There was significantly less arabinose and galactose in the SDP samples obtained from Staccato cherries (~14–15 and ~27%, respectively) vs. Lapins cherries (~20 and ~29%, respectively). The decrease in arabinose could be due to increased hydrolysis of arabinan chains (Yuliarti et al., Citation2015). The SDP samples obtained from the Lapins and Staccato cherries exhibited higher levels of fucose and rhamnose while lower levels of mannose and arabinose compared to the corresponding PWSP samples. The SDP samples from Gala apples showed higher levels of fucose, galactose, and less glucose at longer storage times (~2, 40, and 7%, respectively) compared to the PWSP samples (~0.34, 19, and 51%, respectively). The SDP samples from the Fuji samples demonstrated higher levels of fucose, mannose, galactose, and less glucose (~4–5, 4–5, 35–41, and ~8–15%, respectively) than the PWSP samples (0, ~2–3, ~26–37, ~11–30%, respectively). The SDWSP samples obtained from the fresh Lapins cherries samples contained more rhamnose (~8%) than the corresponding PWSP sample (~7%) but less than the corresponding SDP sample (~15%). Comparable levels of fucose (~2%) and arabinose (~20%) as those present in the corresponding SDP (~2 and 21%, respectively) but less than the corresponding PWSP sample (~0.2 and 25%, respectively) were measured in these SDWSP samples. The SDWSP obtained from fresh Lapins cherries also contained a similar amount of xylose (~2.5%) to that found in the corresponding PWSP and SDP samples (~2.7 and 2.2%, respectively). These SDWSP sample contained a level of mannose (~8.7%) which was comparable to that measured in the corresponding PWSP sample (~8.7%). The SDWSP obtained from fresh Lapins cherries also contained considerably more galactose (~42%) than levels determined for the corresponding PWSP and SDP samples (~29.4 and 29.1%, respectively), while these samples contained considerably less glucose (~16%) than amounts measured in the corresponding PWSP and SDP samples (~27 and 23%, respectively). The SDWSP samples from the fresh Gala apples contained more rhamnose (~5.5%), more fucose (~4.8%), and the same amount of arabinose (~26%) and xylose (~3%) compared to the corresponding PWSP (~3.5, 0.3, 23, and 2.5%, respectively) and SDP (~3.8, 1.7, 18, and 2.2%, respectively) samples. The SDWSP samples contained more mannose (~6%) than the SDP (~2.5%) yet less than the PWSP samples (~6.1%). Galactose was found to be present in greater amounts while glucose was present in lower amounts in the SDWSP samples (~34 and 21%, respectively) obtained from Gala apples compared to the corresponding SDP (~21 and 51%, respectively) and PWSP samples (~20 and 45%, respectively). In all, fruit type, variety, storage, and extraction condition did affect the sugar monomer composition of the polysaccharides as these differences will be discussed in terms of their influence on bioactive properties below.
3.2. Antioxidant activity of water-soluble and soluble digested polysaccharides
The results of the antioxidant activity of the polysaccharides in terms of (1) the reducing power toward Fe(III) and (2) the ability to act as a radical scavenging compound were investigated by the FRAP assay, and the ABTS•+ radical scavenging assay, respectively, was presented in Table . Although the absolute values obtained for the antioxidant activities using these assays are different, in general the trends of antioxidant activity are the same for both assays. The antioxidant activity values were higher in the polysaccharide samples obtained from the cherry fruit compared to the apples as antioxidant activity values for the cherry polysaccharides ranged from ~124 to 720 μmol Trolox eq./g polysaccharide depending on assay, while the antioxidant activity values for the apple polysaccharides ranged from ~12 to 135 μmol Trolox eq./g polysaccharide depending on assay. For the PWSP and SDP samples obtained from the Lapins cherries, there was an observed increase in antioxidant activity upon storage as assessed by both the FRAP and ABTS assays. The antioxidant activity values of the PWSP and SDP samples obtained from the Staccato cherries exhibited an increase upon storage as measured by the FRAP assay. For the PWSP samples obtained from the Gala and Fuji apples, there were was no change in antioxidant activity, as determined by either assay, upon storage. For the SDP samples obtained from the Gala and Fuji apples, there were was an observed increase in antioxidant activity upon storage. As determined by both assays, there was a much higher antioxidant activity in the SDP samples than the PWSP samples for both fruit types. The PWSP samples obtained from the Lapins and Staccato cherries ranged from ~214 to 244 μmol Trolox eq./g polysaccharide for the ABTS method and ~135–170 μmol Trolox eq./g polysaccharide for the FRAP method, while the SDP samples obtained from the Lapins and Staccato cherries ranged from ~660 to 720 μmol Trolox eq./g polysaccharide for the ABTS method and ~314–400 μmol Trolox eq./g polysaccharide for the FRAP method. The SDWSP samples obtained from the fresh Lapins cherries was between ~218 and ~124 μmol Trolox eq./g polysaccharide as determined by the ABTS and FRAP methods, respectively and antioxidant activity levels were not significantly different than levels measured for the corresponding PWSP sample. The antioxidant activity results for the water-extracted cherry polysaccharides are in agreement with those reported by Fan et al. (Citation2010), Ross, Siow, et al. (Citation2015), and Ross, Godfrey, et al. (Citation2015). The PWSP samples obtained from the Gala and Fuji apples ranged from ~22 to 43 μmol Trolox eq./g polysaccharide for the ABTS method and ~12–24 μmol Trolox eq./g polysaccharide for the FRAP method, while the SDP samples obtained from the Gala and Fuji apples ranged from ~67–135 μmol Trolox eq./g polysaccharide for the ABTS method and ~37–67 μmol Trolox eq./g polysaccharide for the FRAP method. The SDWSP samples obtained from the fresh Gala apples was ~91 and ~53 μmol Trolox eq./g polysaccharide as determined by the ABTS and FRAP methods, respectively and were significantly higher than the corresponding PWSP sample. Antioxidant activity of fruits and vegetables has been reported to correlate with total phenolic content (Thetsrimuang, Khammuang, & Sarnthima, Citation2011) and the polysaccharides did contain phenolic compounds (Table ). Generally, the polysaccharides that possessed the highest total phenolics contents did exhibit the highest antioxidant activities. This does suggest that phenolic compounds may be contributing to the antioxidant activity associated with the polysaccharides. However, the SDWSP sample obtained from the fresh Gala apples possessed antioxidant activity values, determined by the ABTS and FRAP methods, which were significantly higher than the antioxidant activity value obtained for the corresponding PWSP sample while there was no significant difference in phenolics content. The Gala SDWSP sample did contain a significantly higher protein content (~19%) along with a significantly higher fucose content (4.8%) and lower glucose content (21%) compared to the corresponding PWSP sample (~3, ~0.26, and ~45%, respectively). A direct relationship between protein content and antioxidant activity has been reported in the literature for green tea polysaccharides (Chen et al., Citation2008). Ross, Siow, et al. (Citation2015) did note a positive relationship between fucose content and antioxidant activity for water-extractable polysaccharides obtained from sweet cherries.
3.3. Inhibition of α-amylase and α-glucosidase activity of water-soluble and soluble digested polysaccharides
Table shows the inhibitory effects of the polysaccharides on the α-amylase and α-glucosidase activity at a test concentration of 5 mg/mL. Acarbose was used as a positive control at a test concentration of 5 mg/mL, and all inhibition measurements are expressed relative to the inhibition of the acarbose which was normalized at 100% at a 5 mg/mL concentration. None of the polysaccharides showed any α-amylase inhibitory activity. This result was not unexpected as the Wang, Yang, and Wei (Citation2010) found that the α-amylase inhibition activity polysaccharides isolated from the leave and flowers of Camellia sinensis was low. Only the SDP samples showed α-glucosidase inhibition. The α-glucosidase inhibitory activity of the Lapins cherries SDP samples increased upon storage while there was no real influence of storage on SDP samples obtained from the Staccato cherries. For the SDP samples isolated from Fuji apple, the α-glucosidase inhibitory activity increased upon storage while there was no real influence of storage on SDP samples obtained from the Gala apples. The SDP samples obtained from the Staccato cherries showed significantly higher α-glucosidase inhibition (~84–88%) than the SDP samples from the Lapins cherries (~53–65%). The SDP samples obtained from the Gala apples showed the lower α-glucosidase inhibition (~20–30%) than the SDP samples obtained from the Fuji apples (~34–55%). These samples did possess higher total phenolics content compared to the other samples, which may have contributed to the higher α-glucosidase inhibitory activity. Raspberry phenolics have been reported to be a potent inhibitor of α-glucosidase (Zhang et al., Citation2010). However, the SDP samples from the Staccatto cherry samples exhibited a phenolics content comparable to the SDP samples from the Lapins cherry samples yet showed significantly higher levels of α-glucosidase inhibition. The same is true for SDP samples isolated from the Gala apples and the Fuji apples, respectively. In terms of chemical differences other than phenolics content, both the SDP samples isolated from the Staccato cherries and Fuji apples demonstrated lower neutral sugars content than the SDP samples isolated from the Lapins cherries and Gala apples. The SDP sample from the Staccato cherries contained higher levels of rhamnose, fucose, but lower levels of arabinose and galactose compared to the SDP sample isolated from the Lapins cherries. The SDP sample from the Fuji apples contained higher levels of xylose and galactose, but lower levels of glucose compared to the SDP sample isolated from the Gala apples. Chen et al. (Citation2009) examined the influence of water SPS extracted from black tea on α-glucosidase activity. These researchers determined a relationship between high levels of α-glucosidase inhibition and lower molecular weight polysaccharides. Molecular weight was not observed to be a factor affecting α-glucosidase activity in this present work. Ross, Godfrey, et al. (Citation2015) used column chromatography to fractionate water SPS isolated from Lapins, Sweetheart, and Skeena variety sweet cherries. The polysaccharide fraction obtained from elution with 1-M NaOH possessed increased levels of fucose and dramatically increased levels of α-glucosidase inhibition. These results do show that polysaccharides with α-glucosidase inhibitory activity are physiologically active after simulated gastrointestinal digestion which is of great relevance in terms of identifying a natural source of α-glucosidase inhibitors for treating/managing type-2 diabetes via control of postprandial hyperglycemia by retarding absorption of glucose.
4. Conclusion
This work evaluated and compared the chemical composition, molecular weight, and bioactive properties (antioxidant activity, α-amylase, and α-glucosidase inhibitory activities) of polysaccharides obtained from sweet cherries (Lapins and Staccato varieties) and apples (Gala and Fuji varieties as influenced by extraction conditions and postharvest storage. The polysaccharides were (1) isolated using a water-based extraction; (2) isolated using a water-based extraction followed by in vitro gastrointestinal digestion; and (3) isolated after in vitro gastrointestinal digestion of the fruit. The polysaccharides isolated from both the sweet cherries and apples contained between ~16 and 37% carbohydrate content and an UA component. For the both cherry varieties and Fuji apple samples, there was no effect of storage on carbohydrate content of the polysaccharides obtained from the various extraction regimes. Generally, the polysaccharides obtained from simulated in vitro gastrointestinal digestion of the sweet cherries and apples showed the highest carbohydrate contents. All of the isolated polysaccharides were conjugated protein moieties and phenolic compounds. All of the polysaccharides obtained using the different extraction regimes were shown to possess antioxidant activity yet, only the polysaccharides isolated from the cherry and apples after simulated in vitro gastrointestinal digestion showed appreciable α-glucosidase inhibition activity, with polysaccharides obtained from Staccato cherries showing nearly 90% of the inhibition of α-glucosidase as achieved with the acarbose control at the same concentration. Although fucose was noted to be a key sugar monomer potentially influencing polysaccharide bioactivity a correlation between chemical characteristics of the polysaccharides and bioactivity could not be established. More study is required to define the relationship between polysaccharides and bioactivity including glycosyl linkage analysis and structural elucidation with NMR. However, this work provides novel information supporting the hypothesis that polysaccharides present in cherries and apples possess bioactivities and retain bioactivity upon storage. Also this work shows that bioactive polysaccharides are available and/or can be isolated during digestion which is an important fact supporting the concept that fruit polysaccharides play a role in enhancing human health.
Acknowledgment
Authors are grateful for the technical assistance of Stanley Chan.
Additional information
Funding
Notes on contributors
Kelly A. Ross
Kelly A. Ross joined as a research scientist in Agriculture and Agri-Food, Canada in October 2008 . She received her PhD from the Department of Food Science at Purdue University. Prior to joining AAFC, at the Department of Food Science and the Department of Agricultural and Biological Engineering at the University of Manitoba, she performed postdoctoral research evaluating chemical components of pulses and determining how storage and processing conditions affected chemical changes and their influence on product quality. Her research is currently focused on isolating and characterizing the polysaccharides present in tree fruits, such as cherries and apples along with berries and ginseng. Ongoing work is concerned with defining the relationships between the chemical composition, and structure of the polysaccharides and bioactivities. Her research is striving to provide fundamental knowledge of production and processing practices that affect the nutritional attributes of foods, including impact of food structure on nutrient release (i.e. the importance of bioaccessability).
References
- Adyanthaya, I. , Kwon, Y. , Apostolidis, E. , & Shetty, K. (2010). Health benefits of apple phenolics from postharvest stages for potential type 2 diabetes management using in vitro models. Journal of Food Biochemistry , 34 , 31–49. doi:10.1111/j.1745-4514.2009.00257.x
- Barbier, M. , & Thibaul, J. F. (1982). Pectic substances of cherry fruits. Phytochemistry , 21 , 111–115. doi:10.1016/0031-9422(82)80024-1
- Batisse, C. , Buret, M. , & Coulomb, P. J. (1996). Biochemical differences in cell wall of cherry fruit between soft and crisp fruit. Journal of Agricultural Food Chemistry , 44 , 453–457. doi:10.1021/jf950227r
- Batisse, C. , Fils-Lycaon, B. , & Buret, M. (1994). Pectin changes in ripening cherry fruit. Journal of Food Science , 59 , 389–393. doi:10.1111/j.1365-2621.1994.tb06974.x
- Billy, L. , Mehinagic, E. , Royer, G. , Renard, C. M. G. C. , Arvisenet, G. , Prost, C. , & Jourjon, F. (2008). Relationship between texture and pectin composition of two apple cultivars during storage. Postharvest Biology and Technology , 47 , 315–324. doi:10.1016/j.postharvbio.2007.07.011
- Blakeney, A. B. , Harris, P. J. , Henry, R. J. , & Stone, B. A. (1983). A simple and rapid preparation of alditol acetates for monosaccharide analysis. Carbohydrate Research , 113 , 291–299. doi:10.1016/0008-6215(83)88244-5
- Cantu-Jungles, T. M. , Maria-Ferreira, D. , da Silva, L. M. , Baggio, C. , Werner, M. F. , Iacomini, M. , … Cordeiro, L. M. C. (2014). Polysaccharides from prunes: Gastroprotective activity and structural elucidation of bioactive pectins. Food Chemistry , 146 , 492–499. doi:10.1016/j.foodchem.2013.09.093
- Carnachan, S. M. , Bootten, T. J. , Mishra, S. , Monro, J. A. , & Sims, I. M. (2012). Effects of simulated digestion in vitro on cell wall polysaccharides from kiwifruit (Actinidia spp.). Food Chemistry , 133 , 132–139. doi:10.1016/j.foodchem.2011.12.084
- Chen, H. , Qu, Z. , Fu, L. , Dong, P. , & Zhang, X. (2009). Physicochemical properties and antioxidant capacity of 3 polysaccharides from green tea, oolong tea, and black tea. Journal of Food Science , 74 , C469–C474. doi:10.1111/j.1750-3841.2009.01231.x
- Chen, H. , Zhang, M. , Qu, Z. , & Xie, B. (2008). Antioxidant activities of different fractions of polysaccharide conjugates from green tea (Camellia sinensis). Food Chemistry , 106 , 559–563. doi:10.1016/j.foodchem.2007.06.040
- Chen, H. X. , Zhang, M. , & Xie, B. J. (2004). Quantification of uronic acids in tea polysaccharide conjugates and their antioxidant properties. Journal of Agricultural and Food Chemistry , 52 , 3333–3336. doi:10.1021/jf0349679
- Dou, J. , Meng, Y. , Liu, L. , Li, J. , & Ren, D. (2015). Purification, characterization and antioxidant activities of polysaccharides from thinned-young apple. International Journal of Biological Macromolecules , 72 , 31–40. doi:10.1016/j.ijbiomac.2014.07.053
- DuBois, M. , Gilles, K. A. , Hamilton, J. K. , Rebers, P. A. , & Smith, F. (1956). Colorimetric method for determination of sugars and related substances. Analytical Chemistry , 28 , 350–356. doi:10.1021/ac60111a017
- Fan, H. , Mazza, G. , & Liao, X. (2010). Purification, composition and antioxidant activity of polysaccharides from wolfberry, cherry, kiwi, and cranberry fruits. Croatian Journal of Food Science and Technology , 2 , 9–17. Retrieved from http://hrcak.srce.hr/index.php?show=clanak&id_clanak_jezik=89739
- Fogliano, V. , Corollaro, M. L. , Vitaglione, P. , Napolitano, A. , Ferracane, R. , Travaglia, F. , … Gibson, G. (2011). In vitro bioaccessibility and gut biotransformation of polyphenols present in the water-insoluble cocoa fraction. Molecular Nutrition Food Research , 55 , S44–S55. doi:10.1002/mnfr.201000360
- Gan, L. , Hua Zhang, S. , Liang Yang, X. , & Bi Xu, H. (2004). Immunomodulation and antitumor activity by a polysaccharide–protein complex from Lycium barbarum . International Immunopharmacology , 4 , 563–569. Retrieved from http://www.sciencedirect.com/science/article/pii/S1567576904000517 10.1016/j.intimp.2004.01.023
- Goliáš, J. , Hic, P. , & Kanová, J. (2010). Effect of low oxygen storage conditions on volatile emission and anaerobic metabolite concentrations in two plum cultivars. Horticultural Science (Prague) , 37 , 145–154.
- Gonçalves, B. , Landbo, A.-K. , Knudsen, D. , Silva, A. P. , Moutinho-Pereira, J. , Rosa, E. , & Meyer, A. S. (2004). Effect of ripeness and postharvest storage on the phenolic profiles of cherries (Prunus avium L.). Journal of Agricultural and Food Chemistry , 52 , 523–530. doi:10.1021/jf030595s
- Gwanpua, S. G. , Van Buggenhout, S. , Verlinden, B. E. , Christiaens, S. , Shpigelman, A. , Vicent, V. , … Geeraerd, A. (2014). Pectin modifications and the role of pectin-degrading enzymes during postharvest softening of Jonagold apples. Food Chemistry , 158 , 283–291. doi:10.1016/j.foodchem.2014.02.138
- Halliwell, B. , Zhao, K. , & Whiteman, M. (2000). The gastrointestinal tract: A major site of antioxidant action? Free Radical Research , 33 , 819–830. doi:10.1080/10715760000301341
- He, N. , Shi, X. , Zhao, Y. , Tian, L. , Wang, D. , & Yang, X. (2013). Inhibitory effects and molecular mechanisms of selenium-containing tea polysaccharides on human breast cancer MCF-7 cells. Journal of Agricultural and Food Chemistry , 61 , 579–588. doi:10.1021/jf3036929
- Hornick, S. B. (2010). Nutritional quality of crops as affected by management practices . Retrieved from http://www.infrc.or.jp/english/KNF_Data_Base_Web/PDF
- Hu, J.-L. , Nie, S.-P. , Min, F.-F. , & Xie, M.-Y. (2013). Artificial simulated saliva, gastric and intestinal digestion of polysaccharide from the seeds of Plantago asiatica L. Carbohydrate Polymers , 92 , 1143–1150. doi:10.1016/j.carbpol.2012.10.072
- Kevers, C. , Pincemail, J. , Tabart, J. , Defraigne, J.-O. , & Dommes, J. (2011). Influence of cultivar, harvest time, storage conditions, and peeling on the antioxidant capacity and phenolic and ascorbic acid contents of apples and pears. Journal of Agricultural and Food Chemistry , 59 , 6165–6171. doi:10.1021/jf201013k
- Knee, M. (1973). Polysaccharide changes in cell walls of ripening apples. Phytochemistry , 12 , 1543–1549. Retrieved from http://www.plantphysiol.org
- Kołodziejczyk, K. , Kosmala, M. , Milala, J. , Sójka, M. , Uczciwek, M. , Król, B. , … Renard, C. M. G. C. (2009). Characterisation of the chemical composition of scab-resistant apple pomaces. Journal of Horticultural Science & Biotechnology , (ISAFRUIT Special Issue), 89–95. Retrieved from http://www.jhortscib.com/isafruit.htm
- Kozarski, M. , Klaus, A. , Niksic, M. , Jakovljevic, D. , Helsper, J. P. F. G. , & Van Griensven, L. J. L. D. (2011). Antioxidative and immunomodulating activities of polysaccharide extracts of the medicinal mushrooms Agaricus bisporus, Agaricus brasiliensis, Ganoderma lucidum and Phellinus linteus . Food Chemistry , 129 , 1667–1675. doi:10.1016/j.foodchem.2011.06.029
- Kumanan, R. , Manimaran, S. , Saleemulla, K. , Dhanabal, S. P. , & Nanjan, M. J. (2010). Screening of bark of Cinnamomum tamala (Lauraceae) by using α-amylase inhibition assay for anti-diabetic activity. International Journal of Pharmaceutical Biomedical Research , 1 , 69–72. Retrieved from www.pharmscidirect.com
- Li, J. , Wang, Y. , Zhang, D. , Hu, X. , Zhang, Z. , & Xiang, C. (2010). Characterization and bioactivity of water-soluble polysaccharides from the fruit of pumpkin. Journal of Food Agriculture and Environment , 8 , 237–241. Retrieved from www.world-food.net
- Li, X. M. , Li, X. L. , & Zhou, A. G. (2007). Evaluation of antioxidant activity of the polysaccharides extracted from Lycium barbarum fruits in vitro . European Polymer Journal , 43 , 488–497. doi:10.1016/j.eurpolymj.2006.10.025
- Lo, T. C. T. , Jiang, Y. H. , Chao, A. L. J. , & Chang, C. A. (2007). Use of statistical methods to find the polysaccharide structural characteristics and the relationships between monosaccharide composition ratio and macrophage stimulatory activity of regionally different strains of Lentinula edodes . Analytica Chimica Acta , 584 , 50–56. doi:10.1016/j.aca.2006.10.051
- Lui, C. J. , & Lin, J. Y. (2012). Anti-inflammatory and anti-apoptotic effects of strawberry and mulberry fruit polysaccharides on lipopolysaccharide-stimulated macrophages through modulating pro-/anti-inflammatory cytokines secretion and Bcl-2/Bak protein ratio. Food Chemistry and Toxicology , 50 , 3032–3039. Retrieved from http://www.sciencedirect.com/science/journal/02786915/50/9
- Luo, Q. , Cai, Y. , Yan, J. , Sun, M. , & Corke, H. (2004). Hypoglycemic and hypolipidemic effects and antioxidant activity of fruit extracts from Lycium barbarum . Life Sciences , 76 , 137–149. Retrieved from http://www.sciencedirect.com/science/journal/00243205/76/2 10.1016/j.lfs.2004.04.056
- Luten, J. , Crews, H. , Flynn, A. , Van Dael, P. , Kastenmayer, P. , & Hurrell, R. (1996). Interlaboratory trial on the determination of the in vitro iron dialysability from food. Journal of the Science of Food and Agriculture , 72 , 415–424. doi:10.1002/(SICI)1097-0010(199612)72:4<415:AID-JSFA675>3.0.CO;2-X
- Mateos-Aparicio, I. , Mateos-Peinado, C. , Jiménez-Escrig, A. , & Rupérez, P. (2010). Multifunctional antioxidant activity of polysaccharide fractions from the soybean byproduct okara. Carbohydrate Polymers , 82 , 245–250. doi:10.1016/j.carbpol.2010.04.020
- Ovodova, R. G. , Golovchenko, V. V. , Popov, S. V. , Popova, G. Y. , Paderin, N. M. , Shashkov, A. S. , & Ovodov, Y. S. (2009). Chemical composition and anti-inflammatory activity of pectic polysaccharide isolated from celery stalks. Food Chemistry , 114 , 610–615. Retrieved from www.elsevier.com/locate/foodchem 10.1016/j.foodchem.2008.09.094
- Palanuvej, C. , Hokputsa, S. , Tunsaringkarn, T. , & Ruangrungsi, N. (2009). In vitro glucose entrapment and alpha-glucosidase inhibition of mucilaginous substances from selected Thai medicinal plants. Scientia Pharmaceutica , 77 , 837–849. doi:10.3797/scipharm.0907-17
- Pawlaczyk, I. , Czerchawski, L. , Kuliczkowski, W. , Karolko, B. , Pilecki, W. , Witkiewicz, W. , & Gancarz, R. (2011). Anticoagulant and anti-platelet activity of polyphenolic-polysaccharide preparation isolated from the medicinal plant Erigeron canadensis L. Thrombosis Research , 127 , 328–340. doi:10.1016/j.thromres.2010.11.031
- Popov, S. V. , Ovodova, R. G. , Golovchenko, V. V. , Popova, G. Y. , Viatyasev, F. V. , Shashkov, A. S. , & Ovodov, Y. S. (2011). Chemical composition and anti-inflammatory activity of a pectic polysaccharide isolated from sweet pepper using a simulated gastric medium. Food Chemistry , 124 , 309–315. Retrieved from www.elsevier.com/locate/foodchem
- Ross, K. A. (2014). Concepts important in understanding the health benefits of phenolics in fruits and vegetables: Extractable and non-extractable phenolics and the influence of cell wall polysaccharides. Research in Health and Nutrition (RHN) , 2 , 29–43. Retrieved from http://www.seipub.org/rhn/paperInfo.aspx?ID=13297
- Ross, K. A. , Godfrey, D. V. , & Fukumoto, L. R. (2015). Purification and characterization of polysaccharides from sweet cherries, raspberries, and ginseng: Chemical composition and bioactivity. Research in Health and Nutrition (RHN) , 3 , 1–13. Retrieved from www.seipub.org/rhn
- Ross, K. A. , Siow, Y. L. , Brown, D. C. W. , Isaak, C. K. , Fukumoto, L. , & Godfrey, D. (2015). Characterization of water extractable crude polysaccharides from cherry, raspberry, and ginseng berry fruits: Chemical composition and bioactivity. International Journal of Food Properties , 18 , 670–689. doi:10.1080/10942912.2013.837066
- Saura-Calixto, F. (2011). Dietary fiber as a carrier of dietary antioxidants: An essential physiological function. Journal of Agricultural and Food Chemistry , 59 , 43–49. doi:10.1021/jf1036596
- Saura-Calixto, F. (2012). Concept and health-related properties of nonextractable polyphenols: The missing dietary polyphenols. Journal of Agricultural and Food Chemistry , 60 , 11195–11200. doi:10.1021/jf303758j
- Scott, R. W. (1979). Colorimetric determination of hexuronic acids in plant materials. Analytical Chemistry , 51 , 936–941. doi:10.1021/ac50043a036
- Shimizu, M. (2010). Interaction between food substances and the intestinal epithelium. Bioscience, Biotechnology, and Biochemistry , 74 , 232–241. doi:10.1271/bbb.90730
- Singleton, V. L. , & Rossi, J. A. (1965). Colorimetry of total phenolics with phosphomolybdic–phosphotungstic acid reagents. American Journal of Enology and Viticulture , 16 , 144–158. Retrieved from http://ajevonline.org/content/16/3/144.abstract
- Stebbins, R. L. , Olsen, J. L. , & Bluhm, W. L. (1998). Picking and storing apples and pears . Retrieved from https://catalog.extension.oregonstate.edu/fs147
- Tamaki, Y. , & Mazza, G. (2010). Measurement of structural carbohydrates, lignins, and micro-components of straw and shives: Effects of extractives, particle size and crop species. Industrial Crops and Products , 31 , 534–541. doi:10.1016/j.indcrop.2010.02.004
- Tarozzi, A. , Marchesi, A. , Cantell-Forti, G. , & Hrelia, P. (2004). Cold-storage affects antioxidant properties of apples in Caco-2 cells. Journal of Nutrition , 134 (5), 1105–1109. Retrieved from http://jn.nutrition.org/content/134/5/1105.full
- Thetsrimuang, C. , Khammuang, S. , & Sarnthima, R. (2011). Antioxidant activity of crude polysaccharides from edible fresh and dry mushroom fruiting bodies of Lentinus sp. strain RJ-2. International Journal of Pharmacology , 7 , 58–65. doi:10.3923/ijp.2011.58.65
- Tsiapali, E. , Whaley, S. , Kalbfleisch, J. , Ensley, H. E. , Browder, I. W. , & Williams, D. L. (2001). Glucans exhibit weak antioxidant activity, but stimulate macrophage free radical activity. Free Radical Biology & Medicine , 30 , 393–402. doi:10.1016/S0891-5849(00)00485-8
- Wani, A. A. , Singh, P. , Gul, K. , Wani, M. H. , & Langowski, H. C. (2014). Sweet cherry (Prunus avium): Critical factors affecting the composition and shelf life. Food Packaging and Shelf Life , 1 , 86–99. doi:10.1016/j.fpsl.2014.01.005
- Wang, B. (2011). Chemical characterization and ameliorating effect of polysaccharide from Chinese jujube on intestine oxidative injury by ischemia and reperfusion. International Journal of Biological Macromolecules , 48 , 386–391. doi:10.1016/j.ijbiomac.2010.12.005
- Wang, Y. , & Long, L. E. (2014). Respiration and quality responses of sweet cherry to different atmospheres during cold storage and shipping. Postharvest Biology and Technology , 92 , 62–69. doi:10.1016/j.postharvbio.2014.01.003
- Wang, Y. , Yang, Z. , & Wei, X. (2010). Sugar compositions, α-glucosidase inhibitory and amylase inhibitory activities of polysaccharides from leaves and flowers of Camellia sinesis obtained by different extraction methods. International Journal of Biological Macromolecules , 47 , 534–538. doi:10.1016/j.ijbiomac.2010.07.007
- Wu, Y. , Cui, S. W. , Tang, J. , Wang, Q. , & Gu, X. (2007). Preparation, partial characterization and bioactivity of water-soluble polysaccharides from boat-fruited sterculia seeds. Carbohydrate Polymers , 70 , 437–443. doi:10.1016/j.carbpol.2007.05.010
- Wu, C. , Shen, J. , He, P. , Chen, Y. , Li, L. , Zhang, L. , … Deng, Y. (2012). The α-glucosidase inhibiting isoflavones isolated from Belamcanda chinensis leaf extract. Natural Products , 6 , 110–120. Retrieved from www.acgpubs.org/RNP
- Xie, J. T. , Wu, J. A. , Mehendale, S. , Aung, H. H. , & Yuan, C. S. (2004). Anti-hyperglycemic effect of the polysaccharides fraction from American ginseng berry extract in ob/ob mice. Phytomedicine , 11 , 182–187. Retrieved from http://www.sciencedirect.com/science/journal/09447113/11/2-3
- Yang, X. , Zhao, Y. , Wang, Q. , Wang, H. , & Mei, Q. (2005). Analysis of the monosaccharide components in angelica polysaccharides by high performance liquid chromatography. Analytical Sciences , 21 , 1177–1180. doi:10.2116/analsci.21.1177
- Yuliarti, O. , Goh, K. K. T. , Matia-Merino, L. , Mawson, J. , & Brennan, C. (2015). Extraction and characterisation of pomace pectin from gold kiwifruit (Actinidia chinensis). Food Chemistry. , 187 , 290–296. doi:10.1016/j.foodchem.2015.03.148
- Zhang, L. , Li, J. , Hogan, S. , Chung, H. , Welbaum, G. E. , & Zhou, K. (2010). Inhibitory effect of raspberries on starch digestive enzyme and their antioxidant properties and phenolic composition. Food Chemistry , 119 , 592–599. doi:10.1016/j.foodchem.2009.06.063