Abstract
Excess cadmium accumulation in shoot decreases the photosynthetic attributes and the activity of carbonic anhydrase (CA; E.C. 4.2.1.1) thereby retarding plant growth metabolism, in a cultivar dependent manner. Two mustard (Brassica juncea) varieties were treated with increasing cadmium doses (0, 25, 50 or 100 mg CdCl2 kg−1 of soil) in the soil in a net house. The photosynthetic features, activity of CA, and the yield attributes were recorded in association with morphological characters. A clear-cut difference in these features was noted among the varieties, where Varuna excelled in its resistance to the cadmium toxicity with better growth and yield, at harvest.
Public Interest Statement
Mustards are important crops as condiment seeds, oil seeds, and leafy vegetables in human diet. Being good accumulator of heavy metals, especially cadmium where make its consumption qualitatively unfit, excess soil cadmium due to excess phosphate fertilizers use, industrially contaminated water and sewage wastes further reduces yield of its consumptionable parts disrupting its physiology and metabolism. Different defense mechanisms induced at specific crop physiological age have been tested in two different genotypes to mark the sensitive response and stress amelioration, limited reports available in physiologically differently performing mustard varieties against stage specific excess heavy metal treatments as soil polluted cadmium. Therefore, locally well-grown mustard varieties in continuation with reported physiological performance of varieties (RH-30 and Varuna), cadmium sensitivity was tested considering important aspect of photosynthetic variation and yield output so that their potential growth in cadmium contaminated areas could be pre-assessed.
Competing interests
The authors declare no competing interest.
1. Introduction
Brassica juncea [L] Czern. & Coss. (family: Brassicaceae) besides an important oil crop is widely used as green vegetable and condiment. The species of B. juncea (mustard) are well-known heavy metal accumulators, particularly cadmium. However, the higher level of cadmium contamination induces toxic responses in mustards plants based on genotypic differences in uptake and distribution (An, Citation2004; Arao, Ae, Sugiyama, & Takahashi, Citation2003; Page & Feller, Citation2015; Zhang, Sun, Li, Liang, & Song, Citation2009). To name amongst are root efficiency of cadmium retention, cadmium-efflux rate, its binding to extracellular matrix, cellular detoxification and complexation, and regulation of cadmium transport to photosynthetically active aerial parts (Irfan, Hayat, Ahmad, & Alyemeni, Citation2013; Marshner, Citation2012; Pérez-Chaca et al., Citation2014; Tanwir et al., Citation2015). The indiscriminate use of phosphate fertilizers, sewage sludge wastes, and wastewater in India has added sufficiently high level of cadmium to agricultural soil (Radha, Kumutha, & Marimuthu, Citation2014; Wuana & Okieimen, Citation2011). Cadmium competes against root absorption of nutrients; induce secondary drought symptoms and cellular toxicity responses (Irfan et al., Citation2013; Tkalec et al., Citation2014). Cadmium-induced disease in mineral uptake seriously affects the activity of carbonic anhydrase (CA), chlorophyll content, and photosynthetic response. The plants, therefore, accumulate lesser dry mass of cultivar which results into reduced plant growth and suboptimal yield output.
Moreover, cadmium is an effective inhibitor of photosynthetic attributes (Krupa, Citation1999; Mohamed, Castagna, Ranieri, & Sanità di Toppi, Citation2012) as it accumulates in the aerial photosynthetic parts to interfere with chloroplast functioning (Babula, Adam, Havel, & Kizek, Citation2012) and Calvin cycle enzymes. Cadmium-induced stomatal resistance (Barceló & Poschenrieder, Citation1990) limits the internal CO2 (López-Climent, Arbona, Pérez-Clemente, & Gómez-Cadenas, Citation2011) and carbon fixation to reduce net photosynthetic rate (Ekmekçi, Tanyolaç, & Ayhan, Citation2008; Mohamed et al., Citation2012). Cadmium and other heavy metals mediated damage of photosynthetic apparatus includes light harvesting complex II and the loss of maximum quantum yield of PSII (Mysliwa-Kurdziel, Stecka, & Strzalka, 2012; Siedlecka, Krupa, Samuelsson, Oquist, & Gardestrom, Citation1997; Tian et al., Citation2014). Reduced photosynthetic efficiency concomitantly led to declined allocation of photosynthates toward sink to compromise fruit yield (Ghani, Citation2010; Patel, Rahi, & Verma, Citation1980). Cadmium also reduced the synthesis and level of photosynthetic pigments (Ekmekçi et al., Citation2008; Mohamed et al., Citation2012; Muradoglu et al., Citation2015; Stobart, Griffiths, Ameen-Bukhari, & Sherwood, Citation1985; Tian et al., Citation2014). The decrease of chlorophyll was reported due to altered structure of chlorophyll molecule where cadmium replaces its central metal, Mg (Küpper, Küpper, & Spiller, Citation1998) and its interference with protochlorophyllide production (Abdel-Basset, Issa, & Adam, Citation1995; Stobart et al., Citation1985). Heavy metals like cadmium and lead also declines the synthesis of 5-aminolavulinic acid and protochlorophyllide reductase complex (Mysliwa-Kurdziel, Prasad, & Strzalka, Citation2004; Pérez-Chaca et al., Citation2014; Stobart et al., Citation1985). Therefore, multiple factors cumulatively decrease chlorophyll content (Gadallah, Citation1995; Usha & Mukherji, Citation1992).
CA is second most abundant soluble protein next to Rubisco, occurring in the chloroplast of C3 plants (Atkins, Patterson, & Graham, Citation1972). It rapidly interconverts CO2 to bicarbonate to maintain CO2 supply of Rubisco. The ratio of CA and Rubisco ascertains the carbon concentration and is regulated at the level of expression for optimal photosynthetic carbon fixation and allocation of photosynthates (Escudero-Almanza et al., Citation2012). Cadmium-induced decline of CA activity could limit Rubisco catalysis through stomatal resistance for internal diffusion of CO2, root competition of zinc availability or directly hindering via oxidative stress at the membranes of chloroplast and thylakoids (Escudero-Almanza et al., Citation2012; López-Climent et al., Citation2011; Poschenrieder, Gunse, & Barcelo, Citation1989). The inhibitor-based study suggested that at thylakoid membrane CA remains closely associated with PSII and possibly regulates the photosynthetic electron transport system (Stemler, Citation1997).
Cadmium-induced inhibition of root Zn uptake seriously affects photosynthesis and other physiological aspects of plant (Escudero-Almanza et al., Citation2012). Redox active metals at low concentrations increase mitochondrial ROS production via Fenton and Haber–Weiss reactions and respiratory electron chain inhibition (Keunen, Remans, Bohler, Vangronsveld, & Cuypers, Citation2011; Pérez-Chaca et al., Citation2014).
The present study was carried out to evaluate the potential of cadmium hyperaccumulation on photosynthetic responses of two mustard varieties along with growth and yield responses. Two local varieties of B. juncea; Varuna and RH-30 have been tested against their tissue level of cadmium, fed at different concentrations of CdCl2 mg kg−1 of soil. The aim was to test the comparative responses of photosynthetic attributes, activity of CA in correlation with growth and yield parameters.
2. Materials and methods
Seeds of B. juncea (cv. Varuna and RH-30) were obtained from National Seed Corporation, New Delhi, India. Surface sterilized seeds (with 0.01% HgCl2 solution) were washed repeatedly with double-distilled water to remove adhering particles of HgCl2. The experiment was arranged in a completely randomized block design in the natural environment of net house of Department of Botany, Aligarh Muslim University, Aligarh, India. The experiment was setup during the winter season of September–February 2009–2010, under ambient environmental conditions with optimum temperature varied from 10 to 30°C.
Seed were sown in earthen pots (25 × 25 cm) filled with sandy loam soil and farmyard manure (6:1 v/v). The soil was polluted with nitrogen from urea, single superphosphate, and muriate of potash added at 40, 138, and 26 mg kg−1 of soil, respectively. The seeds of B. juncea, cv. Varuna or RH-30 were sown at the rate of eight seeds per pot polluted with different concentrations of cadmium (0, 25, 50 or 100 mg CdCl2 kg−1 of soil). After one week, plants were thinned to three plants per pot. Both the varieties were sampled at the vegetative (30 DAS) and flowering stage (60 DAS), and for yield parameters, at harvest.
2.1. Growth analysis
The plants were removed from the pots along with soil and were dipped in a bucket filled with tap water. The plants were gently moved to remove adhering soil particles. The length and fresh mass of shoot and root separately were measured using a meter scale and an electronic balance. The leaf area was measured manually using graph sheet, where the squares covered by the leaf were counted. The plants were then placed in an oven at 80°C for 72 h. The dried shoot and root were then weighed to record dry mass.
2.2. Chlorophyll (SPAD) value and photosynthetic parameters
The SPAD value of chlorophyll was measured using SPAD chlorophyll meter (SPAD-502, Konica, Minolta Sensing, Inc., Japan). Photosynthetic parameters [net photosynthetic rate (P N ), stomatal conductance (g s ), intercellular CO2 concentration (C i ) and transpiration rate (E) and maximum quantum yield of PSII (Fv/Fm)] were measured in a well-expanded upper third leaf while attached to the plant using an infrared gas analyzer portable photosynthetic system (LI-COR 6400, LI-COR, Lincoln, NE, USA), between 11:00 and 12:00 h under clear sunlight. The atmospheric conditions during measurement were photosynthetically active radiation, 1,016 ± 6 μmol m−2s−1, relative humidity 60 ± 3%, atmospheric temperature 22 ± 1°C, and atmospheric CO2 360 μmol mol−1. The duration of the measurement of each sample was 10 min after the establishment of steady-state conditions inside the measurement chamber.
2.3. CA activity
The activity of CA was determined by adopting the procedure described earlier by Dwivedi and Randhawa (Citation1974). Fresh leaf samples (0.2 g) were cut into small pieces and suspended in 10 ml of 0.2 M cysteine hydrochloride solution. The samples were incubated at 4°C for 20 min. The leaf pieces were blotted dry and transferred to test tubes containing 4 ml of phosphate buffer (pH 6.8) followed by the addition of 4 ml of 0.2 M alkaline bicarbonate solution and 0.2 ml of 0.002% bromothymol blue indicator. The test tubes were incubated at 4°C for 20 min. The reaction mixture was titrated against 0.05 N HCl, after the addition of 0.2 ml of methyl red indicator. The results are expressed as mol (CO2) kg−1 (leaf FM) s−1.
2.4. Cadmium accumulation in root and shoot
For cadmium determination, the root and shoot samples were immersed in ice cold 5-mM CaCl2 solution for 10 min to displace extracellular cadmium, rinsed with distilled water. Tissue is oven dried (Meuwly & Rauser, Citation1992). Cadmium concentration in shoot and root was estimated after digesting the samples in nitric acid:perchloric acid (3:1, v/v). Cadmium concentration was determined by atomic absorption spectrophotometer (Perkin-Elmer A, Analyst, 300).
2.5. Yield parameters
Five plants from each treatment (representing five replicates) were randomly sampled and counted for the number of pods per plant at harvest (about 120 DAS). From each treatment, 25 pods were randomly selected and computed to get number of seeds per pod. The pods from each replicate were cleaned, split opened, and weighed subsequently to record the mass of 100 seeds. Seed yield was computed per plant.
3. Statistical analysis
The experiment was conducted according to simple randomized block design. Each treatment was replicated five times and three plants per pot were maintained where each pot was considered as a replicate. Treatment means were compared by the analysis of variance using SPSS 17.0 for Windows (SPSS, Chicago, IL, USA). Least Significant Difference (LSD) between treatment means was calculated at the 5% level of probability.
4. Results
4.1. Growth parameters
The values for all the growth biomarkers (length, fresh and dry mass of shoot and root, and leaf area) of the plants decreased in the soil polluted with cadmium, in comparison to the non-treated (control) plants (Tables and Figure (B)). Out of the various cadmium concentrations (CdCl2; 25, 50 or 100 mg kg−1), 100 mg kg−1 was more toxic and generated maximum reduction of 62.28 and 51.14% in shoot and root length, 70.37 and 56.67% in shoot and root fresh mass, 52.28 and 65.00% shoot and root dry mass and 59.09% in leaf area, in Varuna as compared to the control at 30 DAS for the above-mentioned parameters. Except leaf area, the loss was significant; as compared to the control. The percent loss was less at 60 DAS, denoting the stress acclimation.
Table 1. Effect of soil polluted cadmium (0, 25, 50 or 100 mg CdCl2 kg−1) on root and shoot length (cm) of Varuna and RH-30 variety of B. juncea at 30 and 60 DAS
Table 2. Effect of soil polluted cadmium (0, 25, 50 or 100 mg CdCl2 kg−1) on root fresh mass and root dry mass (g) of Varuna and RH-30 variety of B. juncea at 30 and 60 DAS
Table 3. Effect of soil polluted cadmium (0, 25, 50 or 100 mg CdCl2 kg−1) on shoot fresh mass and shoot dry mass (g) of Varuna and RH-30 variety of B. juncea at 30 and 60 DAS
Table 4. Effect of soil polluted cadmium (0, 25, 50 or 100 mg CdCl2 kg−1) on root and shoot Cd content (μg g−1DW) of Varuna and RH-30 variety of B. juncea at 30 and 60 DAS
4.2. Photosynthetic parameters and leaf chlorophyll level (SPAD value)
The cadmium-stress (CdCl2; 0, 25, 50 or 100 mg kg−1 of soil) significantly lowered most of the photosynthetic attributes (i.e. net photosynthetic rate, stomatal conductance, transpiration rate; maximum quantum yield of PSII and chlorophyll content, SPAD value) except internal CO2 concentration, as compared to the control plants (Figures (A) and (A–D)). At the highest concentration of cadmium (100 mg kg−1 soil), minimum photosynthetic rate was recorded. At the first sampling, (30 DAS) the variety Varuna and RH-30 growth with 25 mg CdCl2 kg−1 soil exhibited a decline of net photosynthetic rate (P N ) by 30.81 and 38.74%, stomatal conductance (g s ); 37.70 and 49.06%, internal CO2 concentration (C i ); 14.73 and 24.80% and transpiration rate (E) 13.59 and 28.28% in comparison to their respective controls. However, the maximum damage in photosynthetic attributes was found in RH-30, against all the levels of cadmium stress. Maximum concentration of cadmium (100 mg CdCl2 kg−1 soil) was most toxic which decreased the SPAD chlorophyll level in Varuna and RH-30 by 51.26 and 75.36% at 30 DAS as compared to their control plants, respectively. However, the percent reduction of SPAD value was lower at 60 DAS. The photosynthesis along with their attributes increased as the growth progressed from 30 to 60 DAS.
Figure 2. Effect of soil amended cadmium (0, 25, 50 or 100 mg CdCl2 kg−1) induced changes on the (A) net photosynthetic rate, (B) stomatal conductance, (C) internal CO2 and (D) transpiration rate
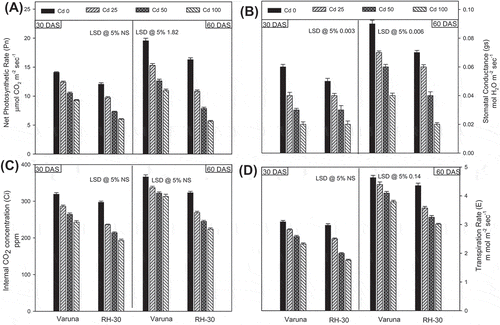
All the soil applied concentrations of cadmium (25, 50 or 100 mg CdCl2 kg−1 soil) reduced the maximum quantum yield of photosystem II (Fv/Fm), more in RH-30, at both the stages (30 and 60 DAS) of growth than Varuna (Figure (C)). However, the reduction of Fv/Fm at highest concentration (100 mg CdCl2 kg−1 soil) in Varuna was 30.19 and 18.95% while in RH-30 it was 46.58 and 42.86%, compared to their respective controls, at the two stages of growth. The cadmium-induced decline in Fv/Fm was sharp at 30 DAS, as compared to 60 DAS.
4.3. Activity of CA enzyme
The activity of CA decreased significantly with respect to cadmium stress (Figure (D)). Of the various concentrations of cadmium (25, 50 or 100 mg CdCl2 kg−1 soil), the highest cadmium level generated maximum loss in both the varieties. At the highest concentration of cadmium, the decrease in CA activity of Varuna and RH-30 was 42.41, 45.44% and 33.10, 56.50% at 30 and 60 DAS to their respective controls.
4.4. Accumulation of cadmium
Both the varieties in general accumulated a higher level of the cadmium in root than shoot which increased further as the growth progressed (Table ). At 30 DAS, cadmium level was 47.11 and 58.02 μg, 97.48 and 118.07 μg, 148.30 and 169.06 μg in the root and 10.28 and 14.83 μg, 25.95 and 37.53 μg, and 59.81 and 83.48 μg in the shoot of Varuna and RH-30, respectively. These values at the same growth stage were significantly higher as compared to the accumulation in root 0.48 and 0.57 μg) and shoots (0.37 and 0.50 μg) of control plants of respective varieties. Cadmium accumulation in the tissues of two varieties increased with the age of plant (30–60 DAS) and was always higher in roots.
4.5. Yield characteristics
Plants grown under cadmium stress (25, 50 or 100 mg CdCl2 kg−1 soil) possessed significantly lower values of number of pods per plant and seed yield per plant, at harvest, compared with the control (Figure ). The maximum loss was noticed in RH-30 at the highest level of CdCl2 (100 mg kg−1 soil), compared to control was 29.58, 33.31, 18.02, and 45.01%, respectively. Furthermore, the loss in Varuna was less pronounced as compared with RH-30.
5. Discussion
Sufficient literature supporting the genotypic variation in tolerance against cadmium toxicity in plants is available (Chaturvedi, Citation2004; Ozturk, Eker, & Ozkutlu, Citation2003; Patel et al., Citation1980). Present investigation shows that length, fresh and dry mass of root and shoot, and leaf area significantly decreased in the two varieties against all the concentrations of cadmium (25, 50 or 100 mg kg−1 CdCl2 soil) polluted into the soil (Tables and Figure (B)). The values for these growth parameters decreased with an increasing accumulation of cadmium in root and shoot tissues (Table ). Roots, however, accumulated more cadmium than shoot, in genotype-independent manner. Patel et al. (Citation1980) and Chaturvedi (Citation2004) also reported similar findings in the genotypes of B. juncea against cadmium stress. The cadmium toxicity in a cultivar might depend upon the capacity of cadmium uptake, its efflux and tolerance and repair mechanisms employed to detoxify the metal (Akhtar, Citation2012; Ozturk et al., Citation2003; Pérez-Chaca et al., Citation2014; Rauser & Meuwly, Citation1995). Moreover, cadmium-induced mineral and water stress could also partially contribute to the decline of fresh and dry mass and other related growth parameters, besides photosynthetic restriction (Escudero-Almanza et al., Citation2012; Marshner, Citation2012).
As the concentration of cadmium increased in soil, concomitant reduction in leaf area followed the reduction of net photosynthetic rate (P N ), it’s associated parameters viz. internal CO2 concentration (C i ), stomatal conductance (g s ), transpiration rate (E), and photosynthetic quantum yield of PSII (Fv/Fm) (Figures (A and C) and (A–D)). The presence of cadmium inhibits efficiency of photosynthetic apparatus (Mohamed et al., Citation2012; Mysliwa-Kurdziel et al., Citation2012) by inhibiting stomatal opening and interfering with the function of chloroplast (Babula et al., Citation2012). Cadmium competitively excludes mineral nutrients absorption, and impedes water uptake which might lead to the generation of desiccation and nutritional/mineral stress and hence induction of ABA signals (Marshner, Citation2012; Tkalec et al., Citation2014). Cadmium-induced ABA signals decrease stomatal conductance (Poschenrieder et al., Citation1989) limit the availability of CO2 (López-Climent et al., Citation2011) which negatively feedbacks the photosynthetic carbon fixation cycle. Cadmium-mediated desiccation stress and stomatal resistance (Babula et al., Citation2012) also hinders transpiration rate and nutritional availability, therefore, inhibits overall photosynthesis (Ekmekçi et al., Citation2008; Mohamed et al., Citation2012). Cadmium interferes with the performance and photon flux yield of PSII several ways. Cadmium-induced stress and subsequent reduction in photosynthesis are reflected as decreased value of maximum yield of net photosynthetic rate (Fv/Fm) or chlorophyll fluorescence (Li, Yang, Yang, Chen, & Ni, Citation2015), a sensitive measure of plant stress as photosynthetic performance (Kancheva, Borisova, & Iliev, Citation2008). CA is also reported in close association with PSII at thylakoid membrane of chloroplasts (Stemler, Citation1997) altering photosynthetic electron transport chain and maximum quantum yield of PSII.
Cadmium inactivates chlorophyll molecule by replacing its central core of the metal, magnesium (Küpper et al., Citation1998). Moreover, cadmium also activates chlorophyll degrading enzyme chlorophyllase (Abdel-Basset et al., Citation1995) and declines the synthesis of chlorophyll biosynthetic enzymes i.e. 5-aminolavulinic acid and protochlorophyllide reductase complex (Mysliwa-Kurdziel et al., Citation2004; Stobart et al., Citation1985). Therefore, decline in the chlorophyll content in the mustard leaves (Figure (A)) and of others (Gadallah, Citation1995; Usha & Mukherji, Citation1992) has been reported under cadmium stress. The results are also in conformity with where cadmium decreased the chlorophyll level in leaves (Muradoglu et al., Citation2015).
The enzyme CA reversibly hydrates CO2 to bicarbonate at the membrane of chloroplast to enrich the stroma with CO2 to be feed in Calvin cycle for carbon fixation. In cytoplasm, CA possibly brings about cytosolic carboxylation (Escudero-Almanza et al., Citation2012) and regulates cytosolic buffering and pH for the normal activity of housekeeping enzymes similar to that of animal systems. Significant decline of CA activity noticed in cadmium stressed mustard varieties denotes dose-dependent inhibition (Figure (D)). Multiple causes have been suggested for the declined activity of CA, for instance, cadmium–zinc antagonism, reduced substrate (CO2) availability through stomatal restriction, and low activation state of CA (Escudero-Almanza et al., Citation2012; Tkalec et al., Citation2014). Inhibition of activity of CA under soil cadmium stress was reported in other crops too (Hasan, Ali, Hayat, & Ahmad, Citation2007; Hayat, Hasan, & Ahmad, Citation2011).
Cadmium reduced the photosynthetic rate and related attributes could negatively draw the biosynthesis of photosynthates which were positively corroborated with the activity of CA, chlorophyll level, and chlorophyll fluorescence (Escudero-Almanza et al., Citation2012; Li et al., Citation2015; Usha & Mukherji, Citation1992). Primary carbon metabolism and restriction of photosynthesis in response to cadmium doses (Mysliwa-Kurdziel et al., Citation2004; Siedlecka et al., Citation1997) severely reduce the quality and quantity of yield (Ghani, Citation2010; Patel et al., Citation1980). The yield parameters (number of pods per plant, number of seeds per pod, weight of 100 seeds and total seed yield per plant) of mustard varieties significantly decreased in response to cadmium toxicity in the two mustard varieties (Figure (A–D)). Therefore, photosynthetic restriction of cadmium toxicity reflected in a genotype-dependent manner which resulted in significant difference of growth and yield attributes (Figure ) which was also shown by Patel et al. (Citation1980) and Ghani (Citation2010). Screening of varieties based on Cd uptake and distribution or Cd sensitivity was tested in different crop plants (An, Citation2004; Arao et al., Citation2003; Page & Feller, Citation2015; Zhang et al., Citation2009). Genetic variations in these varieties seem to regulate different defense traits to impart varieties resistant or sensitivity phenotype. Howden, Coldsbrough, Andersen, and Cobbett (Citation1995) showed cad7 mutants of Arabidopsis thaliana with deficient phytochelatin synthesis were Cd sensitive. These genetic differences further regulate the photosynthetic and biochemical performance in accordance with defense mechanisms deployed which varies from plant to plant (Krupa, Citation1999; Muradoglu et al., Citation2015; Tanwir et al., Citation2015).
6. Conclusion
Cadmium toxicity could be marked as genotype-dependent biochemical and physiological responses. The generalizations of these mechanisms, however, differ in metal accumulator and non-accumulator species. The accumulation of cadmium in resistant variety (here Varuna) is poorly reflected in terms of photosynthetic efficiency and marked activity of CA and lower degradation of chlorophyll molecules. This varietal difference resulted in better seed yield of Varuna as compared to RH-30 under cadmium stress.
Acknowledgment
The author Mohd. Irfan, is thankful to Chairman, Department of Botany, Aligarh Muslim University for providing necessary facilities.
Additional information
Funding
Notes on contributors
Mohd. Irfan
Our research group at Department of Botany, Aligarh Muslim University, Aligarh, India is working on plant stress physiological responses of crop plants and their amelioration using plant growth regulators including recently recognized phytohormones. Mohd. Irfan did his PhD under the supervision of Shamsul Hayat analyzing the role of mustard varieties under cadmium stress and their amelioration using nitric oxide and brassinosteroid analogs as plant growth regulator.
References
- Abdel-Basset, R. , Issa, A. A. , & Adam, M. S. (1995). Chlorophyllase activity: Effect of heavy metals and calcium. Photosynthetica , 31 , 421–425.
- Akhtar, M. F. (2012). Species-specific relationship between transpiration and cadmium translocation in lettuce, barley and radish. Journal of Plant Studies , 1 . doi:10.5539/jps.v1n1p2
- An, Y.-J. (2004). Soil ecotoxicity assessment using cadmium sensitive plants. Environmental Pollution , 127 , 21–26.10.1016/S0269-7491(03)00263-X
- Arao, T. , Ae, N. , Sugiyama, M. , & Takahashi, M. (2003). Genotypic differences in cadmium uptake and distribution in soybean. Plant and Soil , 251 , 247–253.10.1023/A:1023079819086
- Atkins, C. A. , Patterson, B. D. , & Graham, D. (1972). Plant carbonic anhydrases: II. Preparation and some properties of monocotyledon and dicotyledon enzyme types. Plant Physiology , 50 , 218–223.10.1104/pp.50.2.218
- Babula, P. , Adam, V. , Havel, L. & Kizek, R. (2012). Cadmium accumulation by plants of Brassicaceae family and its connection with their primary and secondary metabolism. In N. A. Anjum , I. Ahmad , M. E. Pereira , A. C. Duarte , S. Umar , & N. A. Khan (Eds.), The plant family Brassicaceae ; Environmental Pollution , 21 , 71–97.
- Barceló, J. , & Poschenrieder, C. (1990). Plant water relations as affected by heavy metal stress: A review. Journal of Plant Nutrition , 13 , 1–37.10.1080/01904169009364057
- Chaturvedi, I. (2004). Phytotoxicity of cadmium and its effect on two genotypes of Brassica juncea L. Emirates Journal of Food and Agriculture , 17 , 1–8.10.9755/ejfa
- Dwivedi, R. S. , & Randhawa, N. S. (1974). Evaluation of a rapid test for the hidden hunger of zinc in plants. Plant and Soil , 40 , 445–451.10.1007/BF00011531
- Ekmekçi, Y. , Tanyolaç, D. , & Ayhan, B. (2008). Effects of cadmium on antioxidant enzyme and photosynthetic activities in leaves of two maize cultivars. Journal of Plant Physiology , 165 , 600–611.10.1016/j.jplph.2007.01.017
- Escudero-Almanza, D. J. , Ojeda-Barrios, D. L. , Hernández-Rodríguez, O. A. , Sánchez Chávez, E. , Ruíz-Anchondo, T. , & Sida-Arreola, J. P. (2012). Carbonic anhydrase and zinc in plant physiology. Chilean Journal of Agricultural Research , 72 , 140–146.10.4067/S0718-58392012000100022
- Gadallah, M. A. A. (1995). Effects of cadmium and kinetin on chlorophyll content, saccharides and dry matter accumulation in sunflower plants. Biologia Plantarum , 37 , 233–240.10.1007/BF02913219
- Ghani, A. (2010). Effect of cadmium toxicity on the growth and yield components of mungbean [Vigna radiata (L.) Wilczek]. World Applied Sciences Journal , 8 , 26–29.
- Hasan, S. A. , Ali, B. , Hayat, S. , & Ahmad, A. (2007). Cadmium-induced changes in the growth and carbonic anhydrase activity of chickpea. Turkish Journal of Biology , 31 , 137–140.
- Hayat, S. , Hasan, S. A. , & Ahmad, A. (2011). Growth, nitrate reductase activity and antioxidant system in cadmium stressed tomato (Lycopersicon esculentum Mill) cultivars. Biotechnology, Agronomy Society of Environment , 15 , 401–414.
- Howden, R. , Coldsbrough, P. B. , Andersen, C. R. , & Cobbett, C. S. (1995). Cadmium-sensitive, cad1 mutants of Arabidopsis thaliana are phytochelatin deficient. Plant Physiology , 107 , 1059–1066.10.1104/pp.107.4.1059
- Irfan, M. , Hayat, S. , Ahmad, A. , & Alyemeni, M. N. (2013). Soil cadmium enrichment: Allocation and plant physiological manifestations. Saudi Journal of Biological Sciences , 20 , 1–10.10.1016/j.sjbs.2012.11.004
- Kancheva, R. H. , Borisova, D. S. , & Iliev, I. T. (2008). Chlorophyll fluorescence as a plant stress indicator. In Proceedings of the Fifth National Conference , Space Research Institute—Russian Academy of Sciences (Vol. 5, pp. 301–306). Moscow: Azbuka-2000.
- Keunen, E. , Remans, T. , Bohler, S. , Vangronsveld, J. , & Cuypers, A. (2011). Metal-induced oxidative stress and plant mitochondria. International Journal of Molecular Sciences , 12 , 6894–6918.10.3390/ijms12106894
- Krupa, Z. (1999). Cadmium against higher plant photosynthesis—A variety of effects and where do they possibly come from? Zeitschrift für Naturforsch , 54c , 723–729.
- Küpper, H. , Küpper, F. , & Spiller, M. (1998). In situ detection of heavy metal substituted chlorophylls in water plants. Photosynthesis Research , 58 , 123–133.10.1023/A:1006132608181
- Li, S. , Yang, W. , Yang, T. , Chen, Y. , & Ni, W. (2015). Effects of cadmium stress on leaf chlorophyll fluorescence and photosynthesis of Elsholtzia argyi—A cadmium accumulating plant. International Journal of Phytoremediation , 17 , 85–92.10.1080/15226514.2013.828020
- López-Climent, M. F. , Arbona, V. , Pérez-Clemente, R. M. , & Gómez-Cadenas, A. (2011). Effects of cadmium on gas exchange and phytohormone contents in citrus. Biologia Plantarum , 55 , 187–190.10.1007/s10535-011-0028-4
- Marshner, P. (2012). Marschner’s mineral nutrition of higher plants (3rd ed.). London: Academic Press.
- Meuwly, P. , & Rauser, W. E. (1992). Alteration of thiol pools in roots and shoots of maize seedlings exposed to cadmium: Adaptation and developmental cost. Plant Physiology , 99 , 8–15.10.1104/pp.99.1.8
- Mohamed, A. A. , Castagna, A. , Ranieri, A. , & Sanità di Toppi, L. (2012). Cadmium tolerance in Brassica juncea roots and shoots is affected by antioxidant status and phytochelatin biosynthesis. Plant Physiology and Biochemistry , 57 , 15–22.10.1016/j.plaphy.2012.05.002
- Muradoglu, F. , Gundogdu, M. , Ercisli, S. , Encu, T. , Balta, F. , Jaafar, H. Z. E. , & Zia-Ul-Haq, M. (2015). Cadmium toxicity affects chlorophyll a and b content, antioxidant enzyme activities and mineral nutrient accumulation in strawberry. Biological Research , 48 , 11. doi:10.1186/s40659-015-0001-3
- Mysliwa-Kurdziel, B. , Prasad, M. N. V. , & Strzalka, K. (2004). Photosynthesis in heavy metal stressed plants. In M. N. V. Prasad (Ed.), Heavy metal stress in plants, from biomolecules to ecosystems (2nd ed., pp. 47–119). New Delhi: Springer-Verlag. Heidelberg, Narosa
- Mysliwa-Kurdziel, B. , Stecka, A. , & Strzalka, K. (2012). Initial stages of angiosperm greening monitored by low-temperature fluorescence spectra and fluorescence lifetimes. Methods in Molecular Biology , 875 , 231–239.
- Ozturk, L. , Eker, S. , & Ozkutlu, F. (2003). Effect of cadmium on growth and concentrations of cadmium, ascorbic acid and sulphydryl groups in durum wheat cultivars. Turkish Journal of Agricultural Forestry , 27 , 161–168.
- Page, V. , & Feller, U. (2015). Heavy metals in crop plants: Transport and redistribution processes on the whole plant level. Agronomy , 5 , 447–463.10.3390/agronomy5030447
- Patel, V. K. , Rahi, B. B. , & Verma, C. S. (1980). Genotypic differences in effects of cadmium on yield and nutrient composition in Brassica plants. Agronomy Journal , 72 , 45–47.
- Pérez-Chaca, M. V. , Rodríguez-Serrano, M. , Molina, A. S. , Pedranzani, H. E. , Zirulnik, F. , Sandalio, L. M. , & Romero-Puertas, M. C. (2014). Cadmium induces two waves of reactive oxygen species in Glycine max (L.) roots. Plant, Cell & Environment , 37 , 1672–1687.10.1111/pce.2014.37.issue-7
- Poschenrieder, C. , Gunse, B. , & Barcelo, J. (1989). Influence of cadmium on water relations, stomatal resistance, and abscisic acid content in expanding bean leaves. Plant Physiology , 90 , 1365–1371.10.1104/pp.90.4.1365
- Radha, R. V. , Kumutha, K. , & Marimuthu, P. (2014). Assessment of cadmium contamination of soils in sewage disposal areasof Coimbatore district, Tamil Nadu, India. Current World Environment , 9 , 379–386.10.12944/CWE
- Rauser, W. E. , & Meuwly, P. (1995). Retention of cadmium in roots of maize seedlings. Role of complexation by phytochelatins and related thiol peptides. Plant Physiology , 109 , 195–202.10.1104/pp.109.1.195
- Siedlecka, A. , Krupa, Z. , Samuelsson, G. , Oquist, G. , & Gardestrom, P. (1997). Primary carbon metabolism in Phaseolus vulgaris plants under Cd (II)/Fe interaction. Plant Physiology Biochemistry , 35 , 951–957.
- Stemler, A. J. (1997). The case for chloroplast thylakoid carbonic anhydrase. Physiologia Plantarum , 99 , 348–353.10.1111/ppl.1997.99.issue-2
- Stobart, A. K. , Griffiths, W. T. , Ameen-Bukhari, I. , & Sherwood, R. P. (1985). The effect of Cd2+ on the biosynthesis of chlorophyll in leaves of barley. Physiologia Plantarum , 63 , 293–298.10.1111/ppl.1985.63.issue-3
- Tanwir, K. , Akram, M. S. , Masood, S. , Chaudhary, H. J. , Lindberg, S. , & Javed, M. T. (2015). Cadmium-induced rhizospheric pH dynamics modulated nutrient acquisition and physiological attributes of maize (Zea mays L.). Environmental Science and Pollution Research , 22 , 9193–9203.10.1007/s11356-015-4076-8
- Tian, T. , Ali, B. , Qin, Y. , Malik, Z. , Gill, R. A. , Ali, S. , & Zhou, W. (2014). Alleviation of lead toxicity by 5-aminolevulinic acid is related to elevated growth, photosynthesis, and suppressed ultrastructural damages in oilseed rape. BioMed Research International . doi:10.1155/2014/530642
- Tkalec, M. , Štefanić, P. P. , Cvjetko, P. , Šikić, S. , Pavlica, M. , & Balen, B. (2014). The effects of cadmium–zinc interactions on biochemical responses in tobacco seedlings and adult plants. PLoS ONE , 9 , e87582.10.1371/journal.pone.0087582
- Usha, K. , & Mukherji, S. (1992). Effect of cadmium toxicity on chlorophyll content, hill activity and chlorophyllase activity in Vigna radiata L. leaves. Indian Journal of Plant Physiology , 35 , 225–230.
- Wuana, R. A. , & Okieimen, F. E. (2011). Heavy metals in contaminated soils: A review of sources, chemistry, risks and best available strategies for remediation. ISRN Ecology . doi:10.5402/2011/402647
- Zhang, J. , Sun, W. , Li, Z. , Liang, Y. , & Song, A. (2009). Cadmium fate and tolerance in rice cultivars. Agronomy for Sustainable Development , 29 , 483–490.