Abstract
Rain-fed agriculture exists in North Sinai and Marsa Matrouh in Egypt. The objective of this paper was to close the gap in our understanding on how climate would affect agricultural sustainability in rain-fed area. To implement that, integrated modeling approach was used. BISm and Yield–Stress models were used to simulate the effect of climate change on crops productivity in 2030. Furthermore, the effect of manure application to these crops was done to explore its effect on improving productivity under drought conditions. The simulation results revealed that productivity of barley and wheat will severely reduce in 2030, whereas the productivity of fruit trees will reduce with lower magnitude. Simulation of the additive effect of manure application revealed that yield losses in all the studied crops were reduced. Potential barley yield losses were reduced from 48 and 85% in North Sinai and Marsa Matrouh, respectively, to 12 and 71%. Wheat yield losses will be reduced from 62 and 90% in North Sinai and Marsa Matrouh, respectively, to 38 and 82%. Therefore, a production package should be implemented in these areas to increase its resilience to face rain fall variability in the future.
Public Interest Statement
The impact of climate change on rain-fed cultivation in Egypt was not studied before. We used climate change scenarios with high level of accuracy were used to project rain fall in 2030. Integrated modeling approach was used integrated modeling approach to simulate the effect of climate change on crops cultivated in the area. These results are important for policy-makers to be informed about that anticipated effect.
Competing interests
The authors declare no competing interest.
1. Introduction
Crop production in rain-fed agriculture relies on seasonal rain fall, often shows a grim picture of a fragile environment due to drought, soil degradation, low rain (water) use efficiency, poor infrastructure, and inappropriate policies (Pereira, Citation2005). Rain-fed agriculture in the Egyptian North coast constitutes an important part of the existent economic activities. Rain fall rate varies in this area ranging from 130 to 150 mm in the northwestern coast and from 80 mm (west of Al-Arish) to 280 mm (at Rafah) in the northeast. This rate decreases after 20 km south of the Mediterranean in both areas (http://www.emwis-eg.org). Few field crops are cultivated in the rain-fed areas in Egypt, mainly barley and wheat, in addition to few fruit trees. There are several serious threats being faced by rain-fed areas, where most of it are farmed using the old, traditional, and primitive soil and crop management practices. Khalifa et al. (Citation2004) indicated that in North Sinai, barley is cultivated every year, which resulted in low productivity. Similar situation was found in Marsa Matrouh, where wheat productivity under rain-fed conditions is low due to wheat cultivation every year in the same piece of land (Attia & Barsoum, Citation2013). Although, manure is a source of copious content of major and minor nutrition elements available to plant roots absorption, most famers in rain-fed area do not perform this practice (Shams & Kamel, Citation2014).
The alarming results of climate change studies on rain fall globally implied that in fragile environment like North Coast of Egypt, climate change could badly affect it. However, there are no local studies on the effect of climate change on rain-fed agriculture in Egypt. The Fifth Assessment Report (AR5) of the Intergovernmental Panel on Climate Change contained a large number of comprehensive climate models and Earth System Models, whose results form the core of the climate system projections (IPCC, Citation2013). These models produce new Representative Concentration Pathways Scenarios in the future, i.e. RCP2.6, RCP4.5, RCP6.0, and RCP8.5 (Wayne, Citation2013). Using these RCPs could lower the uncertainty in assessment of the effect of climate change on evapotranspiration and crops productivity (Solomon et al., Citation2007). Morsy (Citation2015) indicated that using global models and region models could achieve higher level of certainty in the projection of the effect of climate change.
The objective of this paper was to close the gap in our understanding on how climate would affect cultivated crops in rain-fed areas in Egypt in the future. To implement that, integrated modeling approach was used, where BISm model and Yield–Stress model were used to simulate the effect of climate change in 2030 on productivity of crops grown in rain-fed areas in Egypt. Furthermore, the effect of manure application to these crops was simulated to explore its effect on improving productivity under climate change.
2. Methodology
2.1. Selected governorates and crops
Rain-fed agriculture in Egypt is represented by two governorates, i.e. North Sinai in northeast of Egypt (Latitude = 32.07°, Longitude = 33.45° and Elevation above sea level 17.10 m) and Marsa Matrouh in northwest of Egypt (Latitude = 31.30°, Longitude = 27.20° and Elevation above sea level 7.00 m) as shown in Figure .
Barley field data were obtained from Kamel (Citation2011) in 2008 growing season under rain-fed in North Sinai. Barley was planted on 4January, where rainfall amount was 24.9 mm of and it was harvested on 14April, three weeks after 18.1 mm rainfall. Wheat data were obtained from Hussain (Citation2005) in 2001/02 growing season, where wheat was planted on 2 December after 43.5 mm of rainfall and was harvested on 14 April three weeks after 31.1 mm of rainfall. Olive was grown in 2010/11 at distance of 5 X 5 m in North Sinai (Mohy El-Din, El-Begawy, Shabbara, & Yassen, Citation2013). Furthermore, peach in North Sinai was grown in 2010 under rain-fed conditions at distance of 7 × 7 (El-Kosary, Abdel-Mohsen, El-Merghany, & Badran, Citation2013). The total seasonal rain in 2010 was 140.0 mm, which produced 4.55 and 8.10 ton/ha peach and olive, respectively (Table ).
Table 1. Selected crops, their sowing and harvest dates and amount of seasonal rain fall in the selected governorates
Regarding to Marsa Matrouh, field data for barley were obtained from Kamel (Citation2011) grown in 2006/07 growing season. Barley was planted after major rain fall event on 31 December, where 19.4 mm were fall and was harvested on 25 April. Wheat field data were obtained from Attia and Barsoum (Citation2013) in 2006/07 growing season. With respect to olive grown in Marsa Matrouh, trees were grown at 7 × 7 m apart and the yield was 5.10 ton/ha in 2008 (Attalla, Abdel-Sattar, Mahrous, & Abdel-Azeez, Citation2011), where total rainfall was 91.6 mm. Fig trees were also grown in Marsa Matrouh at 7 × 7 m apart in 2006 growing season with total rainfall equal 75.6 mm and produced 8.78 ton/ha (Al-Desouki, Abd El-Rhman, & Sahar, Citation2009). Table summarizes the data used in the analysis.
2.2. Calculation of reference evapotranspiration
The BISm model (Snyder, Organ, Bali, & Eching, Citation2004) was used to calculate monthly reference evapotranspiration (ET). The model calculates ET using the Penman–Monteith (PM) equation (Monteith, Citation1965) as presented in the United Nations FAO Irrigation and Drainage Paper (FAO, 56) by Allen, Pereira, Raes, and Smith (Citation1998). Weather normals (1985–2014) were used to calculate ET values for each governorate.
Weather data in 2030 were obtained from Morsy (Citation2015). He developed a methodology to determine the most suitable global climate model and scenario for different governorates in Egypt through comparison between measured meteorological data with the projected data from four global climate models represented by its four RCPs scenarios during the period from 2006 to 2012. His results indicated that RCP6.0 scenario from CCSM4 model was more accurate to project meteorological and climate variables. Thus, he used the boundaries of RCP6.0 scenario in WRF-RCM model (regional climate model) to predict weather data in 2030 after comparing its predicted previous weather data with its counterpart of the measured metrological data and he produced weather data in 2013 with 90% degree of accuracy. Furthermore, the predicted weather data by WRF-RCM model was used to project ET values in each governorate in 2030.
2.3. Simulation of crops productivity in rain-fed area
Yield–Stress model (Ouda, Citation2006) was used in this analysis. The model assumes that there is a linear relationship between available water and yield, in which the reduction in available water limits evapotranspiration and consequently reduces yield. The Yield–Stress model was designed to predict the effect of scheduling deficit irrigation on the yield of several crops and on their water consumptive use. Most importantly, its performance was acceptable, when it was compared to CROPWAT (Khalil, Ouda, & Ewias, Citation2008). The model uses daily weather data to calculate evapotranspiration (ET) and crop evapotranspiration (ETc). The model calculates water depletion from root zone and dry matter accumulation. The model was modified to simulate productivity under rain-fed condition. BISm model (Snyder et al., Citation2004) was used to calculate daily ET and crop kc. BISm model contains a list of crops in its database, where it could be used to calculate crop kc as a percentage of its growing season. Sowing and harvest dates are also involved in determining kc for each growth stage. The kc values for the selected crops are presented in Tables and .
Table 2. Wheat and barley crop coefficients (kc) values in the two studied governorates
Table 3. Olive, peach and fig crop coefficients (kc) values in the two studied governorates
Yield–Stress model used these values of daily ET and crop kc as input to calculate daily ETc. Available water in soil as a characteristic of soil type was input in the model to calculate the depletion of rain water from the root zone and calculate water stress index, whenever water stress exist. Water stress index is calculated as a reduction factor on transpiration then on assimilates translocation. Water stress index takes a value between 0–1. Water stress index equal 0 when there is no moisture in the root zone to fulfill the needs for evapotranspiration. During this time, photosynthesis ceased and no photosynthate translocation or growth occurs (Allen et al., Citation1998).
The model was calibrated for the selected crops using the published data. The calibration process depends on adjustment in crop calibration coefficient (Yc, kg/MJ/day). Yc represents the amount of dry matter in kilogram that is produced by mega joule per day and accumulated in term of biological yield. Thus, Yc is affected by weather conditions in a region and growing season of the cultivated crop. Furthermore, using Yc for calibration of Yield–Stress model can allow very accurate simulation for dry matter accumulation, if great effort was made in the calibration procedure. Under this condition, the simulated yield value can be as the same as the measured values in the field, which is required under rain-fed cultivation to improve the quality of simulation.
Yield–Stress model was used to simulate the effect of projected rain fall amount in 2029/30 on barley, wheat, and the studied fruit trees. Furthermore, the model was also used to simulate the effect of using manure as fertilizer on moisture retention through enhancing soil water storage ability. Thus, we assume that if we start to apply manure now, the available water in the soil can increase from 0.09 m/m to 0.15 m/m by 2030. Then, we simulated the yield of the cultivated crops using the above value of available water in the soil under climate change in 2029/30.
2.4. Comparison between past and future annual rain fall
Deviation of rainfall from normal (long term mean) is the most commonly used indicator for drought monitoring. It was calculated for projected annual rain value in 2030. On the basis of rain fall deviations, four categories are used for monitoring and evaluating the rain fall patterns: ±10% deviation as normal. Furthermore, −10 to −60% deviation in the rain fall is consider as deficit, less than −60% deviation as scanty, and greater than 20% deviation as excess (Kumar, Murthy, SeshaSai, & Roy, Citation2009).
2.5. Water and land productivity
Crop water productivity (kg/m3) and land productivity (kg/m2) measurements are quantitative terms used to define the relationship between crop produced with the amount of applied irrigation water or unit of land involved in crop production. They are useful indicators for quantifying the impact management on final crop yield (FAO, Citation2003).
3. Results
3.1. Calibration of Yield–Stress model for the studied crops
3.1.1. Barley and wheat
Table shows measured and predicted values of grains and biological yield of both crops. The results in that table indicated that Yield–Stress model can be calibrated to predict barley and wheat yield values similar to the measured values in the field, where percentage of difference between measured and predicted values was zero.
Table 4. Measured vs. predicted grain and biological yield of barley and wheat in rain fed area
3.1.2. Olive, peach, and fig trees
Yield–Stress model was calibrated for peach and olive trees in North Sinai and olive and fig trees in Marsa Matrouh using field experiments data in both sites. Table indicated that the model was able to predict values of yield similar to the measured values in the field.
Table 5. Measured vs. predicted olive, peach and fig yield in rain fed area
3.2. Comparison between past and future annual rain fall
The projected annual rain value in 2030 was graphed with annual rain values starting from 1997 to 2014 in North Sinai (Figure ) and in Marsa Matrouh (Figure ). Figure indicated that the projected value of annual rainfall in 2030 will be the lowest of what was recorded from 1997 to 2014, except for the amount in 1999, which was lower. Rainfall deviation in 2030 from the average of 18 years was −27%, which considered deficit.
Figure 3. Monthly rain fall amounts in 2030 for the studied years of field data and in 2030/30 in North Sinai.
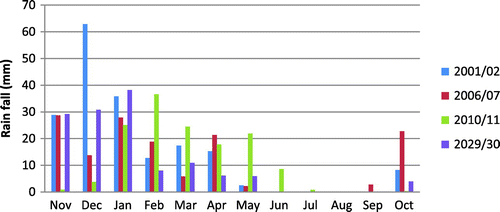
Comparison between monthly rainfall values for the studied field data and projected rainfall data in 2029/30 in North Sinai revealed that the lowest monthly rain fall was found in 2029/30 (Figure ).
Similarly, the projected annual rain fall value in 2030 will be lowest compared to the recorded value from 1997 to 2014 (Figure ). Rainfall deviation in 2030 from the average of 18 years was −95%, which considered scantly. This result implied that drought will highly affect Marsa Matrouh, compared to North Sinai.
Furthermore, monthly rainfall values for the studied years of field data were higher than projected rainfall data in 2029/30 in Marsa Matrouh (Figure ).
Figure 5. Monthly rain fall amount in 2030 for the studied years of field data and in 2029/30 in Marsa Matrouh.
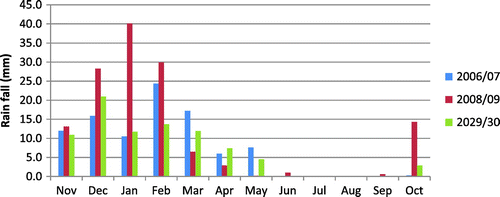
Thus, the daily values of rainfall in the two studied locations are graphed and presented in Figures and to show the frequency and the daily amount of rainfall. Figure indicated that the frequency of rain fall in North Sinai will increase in 2029/30; however the amount will be much lower. The highest amount of rain in the whole season will be 7.2 mm in December 2029. However, lower values in rainfall are projected and last for few days as shown in Figure .
Similar trend was observed in Marsa Matrouh, where the frequency increased and the amount decreased as shown in Figure . The highest value of rainfall was 5.4 mm in March.
3.3. Assessment of the effect of low rain fall on crops productivity
Table showed predicted grain and biological yield of barley and wheat in projected 2029/30 growing season. The results indicated that as a result of lower rain amount in 2030, barley yield will be reduced by 48% and wheat yield will be reduced by 62%.
Table 6. Predicted grain and biological yield of barley and wheat in 2029/30 in North Sinai
More drastic consequences are expected to occur in Marsa Matrouh in 2029/30, where barley and wheat will not produce any grains as a result of low rain fall. The biological yield will be reduced by 85 and 90% for barley and wheat, respectively (Table ). The above results are very disturbing and implied that famers living in Marsa Matrouh will be not able to cultivate barley and wheat in 2029/30.
Table 7. Predicted biological yield of barley and wheat in 2029/30 under climate change in Marsa Matrouh
The simulation procedure was done for fruit trees in 2029/30 in both sites using Yield–Stress model. The simulation results indicated that fruit trees grown in the two sites will be more tolerant to drought and its yield will be reduced by lower percentage, compared to cereal crops. Olive yield will be reduced by 25 and 56% in North Sinai and Marsa Matrouh, respectively (Table ).
Table 8. Predicted yield of fruit trees in 2029/30 under climate change in both sites
3.4. Adaptation of crops structure in rain-fed areas to climate change
Simulation of the effect of manure application at present time on increasing water holding capacity under climate change in 2030 was done. The simulation results in Table indicated that the additive effect of the application of manure under rain-fed in both sites can reduce yield losses of barley and wheat in 2029/30. Potential barley yield losses can be reduced from 48 and 85% in North Sinai and Marsa Matrouh, respectively (Tables and ), to 12 and 71% (Table ). Wheat yield losses can be reduced from 62 and 90% in North Sinai and Marsa Matrouh, respectively (Tables and ) to 38 and 82% (Table ).
Table 9. Predicted grain yield of barley and wheat (ton/ha) in North Sinai and biological yield of barley and wheat with application of manure in 2029/30
Regarding olive, its yield losses will be reduced from 25 and 56% (Table ) 12 to and 41% (Table ) when manure is applied. Similarly, peach and fig yield losses will lower from 27 and 44% (Table ), to 14 and 13% (Table ), respectively, as a result of additive effect of manure application on soil water retention.
Table 10 Predicted yield of fruit trees (ton/ha) with application of manure in 2029/30 in both sites
3.5. Water and land productivity in rain-fed areas
Table indicated that, in general, water, and land productivity was higher in North Sinai under current situation, under climate change and under manure application for all the cultivated crops. This could attribute to the higher amount of rainfall in North Sinai, compared to Marsa Matrouh. Furthermore, water and land productivity for barely was higher than wheat under all studied options, as a result of shorter growing season and lower water requirements for barely in both sites. Climate change effects highly reduced water and land productivity for all crops in both sites due to low rainfall. However, application of manure increased water and land productivity for all crops owing to the role that manure does in increasing water holding capacity of the soil.
Table 11. Current and future water productivity (WP) and land productivity (LP) in rain fed areas
4. Discussion
Rain-fed agriculture is generally risky due to high spatial and temporal variability in rainfall. Innovative approaches need to be adapted to manage such fragile resources under rain-fed conditions in Egypt. To do that, we calibrated Yield–Stress model and used it to simulate the effect of new management on the growing crops in these areas. Our results implied that Yield–Stress model was capable of predicting the same measured values of barley, wheat, olive, peach, and fig yields as a result of fine tuning calibration procedure. This fine tuning facilitated the use of the model for further investigation on the effect of climate change on rain fall. Similar results on the capability of Yield–Stress model in accurate prediction of productivity of wheat were obtained by Khalil, Ouda, and Tantawy (Citation2007) and on productivity of barley (El-Mesiry, Gaballah, & Ouda, Citation2007).
Evidence is emerging that climate change is making the climate variability more intense, with increased frequency of extreme events such as drought (IPCC, Citation2001). Thus, the future of rain-fed agriculture in Egypt is mainly dependent on the amount and frequency of rainfall. As stated previously, there were no studies on the effect of climate change on rain-fed agriculture in Egypt. Furthermore, there is a need to project the incidence and the quantity of rainfall under climate change. Our results indicated that the projected annual amount of rainfall will decrease in the studied sites. In North Sinai, the annual rainfall deviation in 2030 from the average of 18 years was -27%, which considered deficit. Furthermore, the monthly values of rainfall in 2030 were lower than its counterpart values in the years where field data were collected. Similarly, in Marsa Matrouh, the projected annual rainfall value in 2030 will be lowest compared to the recorded value from 1997 to 2014. Rain fall deviation in 2030 from the average of 18 years was - 95%, which considered scantly. This result implied that drought will highly affect Marsa Matrouh, compared to North Sinai. Thus, it is expected under these low amounts of rainfall that productivity of crops grown in Marsa Matrouh will be much lower than its counterpart in North Sinai.
This assumption proved to be true as the simulation results revealed that in North Sinai in 2030, barley grain yield will be reduced by 48% and wheat grain yield will be reduced by 62%. Lower barley yield reduction can be attributed to the fact that barley is adapted to a severe water regime compared with other cereals (Samarah, Citation2005). Furthermore, olive and peach yields were reduced with lower percentage compared to barley and wheat. Sofo, Manfreda, Fiorentino, Dichio, and Xiloyannis (Citation2008) indicated that olive trees developed series of physiological mechanisms to tolerate drought stress and grow under adverse climatic conditions. However, under high water stress, its yield was reduced. Furthermore, the peach tree is known by its ability to withstand low moisture availability in the root zone area (Abrisqueta, Vera, Tapia, Abrisqueta, & Ruiz-Sánchez, Citation2012).
The situation will be worst in Marsa Matrouh in 2030, where no grain yield for either cereal crops will be formed, only biological yield will be produced. Furthermore, olive and fig yield will be highly reduced. Although fig tree is characterized by its tolerance to water deficit, its yield was highly reduced as a result of drought. Allam, Adly, and Mourad (Citation2007) and Al-Desouki et al. (Citation2009) indicated that the growth and yield of fig trees was reduced under severe drought stress. Furthermore, our results showed that water and land productivity was highly reduced under climate change for all crops in both sites.
Khalifa et al. (Citation2004) indicated that the soils in the rain-fed areas of Egypt are characterized by being sandy with low soil fertility level and low water holding capacity. Thus, to increase the ability of these soils to hold water in 2030, application of manure in the present time could increase soil available water from 0.09 m/m to 0.15 m/m in 2030. Mosavi et al. (Citation2012) indicated that manure as fertilizer could be a method for soil and water conservation, as well as moisture retention through enhancing soil water storage ability. Wortmann and Shapiro (Citation2008) observed reduction in bulk density, increase in hydraulic conductivity, and increase in available soil water holding capacity by 56% compared to the control and after application of compost or manure for five years. Thus, we simulated the effect of increased soil available water on the productivity of the cultivated crops in the studied areas. The simulation results indicated that the additive effect of the application of manure under rain-fed in both sites can reduce yield losses of the cultivated crops. Shams and Kamel (Citation2014) indicated that manure application to barley and wheat grown solely in Marsa Matrouh increased its yield by 15 and 25%, respectively, whereas, manure application to barely in North Sinai increase yield by 12%, compared to barely cultivation without application (Khalifa et al., Citation2004). Furthermore, application of manure to peach trees grown in North Sinai increased yield per tree by 37% (El-Kosary et al., Citation2013). The acidic effect of manure lowers pH value and facilitates nutrients absorption (Estefanous, Mikhaeel, & Anton, Citation1997). Application of manure improved water and land productivity for all crops planted in both sites.
Thus, to ensure the sustainability of rain-fed agriculture in Egypt, we need to implement improved management practices to enhance productivity of cultivated crops in these areas. We suggested three practices to face drought stress occurrence in rain-fed area in Egypt: application of manure, as well as using crop rotation and inter-planting (cultivation of field crop under trees). Although we were not able to simulate the effect of all of them, the simulation results of application of manure were encouraging to assume that additive effect of the three suggested practices could overcome the risk of drought under current climate and the projected climate change in 2030. Crop diversification by adding legume crops with low water requirements, such as lintel, pea, and faba bean can improve productivity of barley and wheat under crop rotation implementation (Khalifa et al., Citation2004; El-Sadek & Salem, Citation2015). Inter-planting lintel under young olive trees or under fig and peach trees (young or mature) increased the yield of the tree as a result of increase in soil fertility. Furthermore, it reduced wind erosion and conserve moisture content (Kamel, Citation2011). Crop rotations, where legume crops followed cereals crops proved to increase cereals yield, as cereals benefits from nitrogen fixed by legume and decomposition of roots and nodules (Shams & Kamel, Citation2014).
5. Conclusion
This research is the first in Egypt to assess the effect of climate change on rain-fed agriculture. Our results indicated that rainfall in both North Sinai and Marsa Matrouh will be highly reduced in 2029/30. The frequency of rainfall will increase; however the amount will be much lower. As a consequence, the productivity of cereal crops will be highly reduced to the extent that no grain yield will be produced in Marsa Matrouh. Furthermore, the productivity of fruit crops will be affected with lower losses than field crops. The simulated additive effect of manure revealed that it could reduce yield losses for all crops in both sites. Therefore, a production package should be implemented in these areas to increase its resilience to face rainfall variability in the future. Lastly, using modeling to predict rainfall dates and amounts is an important procedure to be done by extension workers in rain-fed areas to increase the resilience of these areas to face rainfall variability.
Acknowledgement
The authors of this paper thank Dr. Mostafa Morsy at Astronomy and Meteorology Department; Faculty of Science; Al Azhar University in Egypt for providing us with weather data in 2029/30.
Additional information
Funding
Notes on contributors
S. Ouda
Samiha Ouda is a professor of Agronomy in Water Requirement and Field Irrigation Department; Soils, Water and Environment Research Institute; Agricultural Research Center in Egypt. She had her PhD from Iowa State University. She is specialized in working with simulation models. She is concern with the assessment of the effect of climate change on crops productivity and water requirements. She published over 60 publications on using simulation models to assess the impact of climate change.
References
- Abrisqueta, I., Vera, J., Tapia, L. M., Abrisqueta, J. M., & Ruiz-Sánchez, M. C. (2012). Soil water content criteria for peach trees water stress detection during the postharvest period. Agricultural Water Management, 104, 62–67.10.1016/j.agwat.2011.11.015
- Al-Desouki, M. I., Abd El-Rhman, I. E., & Sahar, A. F. (2009). Effect of some antitranspirants and supplementary irrigation on growth, yield and fruit quality of Sultani fig (Ficus carica) grown in the Egyptian Western Coastal Zone under rain fed conditions. Research Journal of Agriculture and Biological Sciences, 5, 899–908.
- Allam, K. H. A., Adly, M. Y., & Mourad, M. A. (2007). Effect of supplemental irrigation and intercropping treatments on the productivity of fig trees and lentil crop in the Northwest Coast. Misr Journal Agricultural Engineering, 24, 88–102.
- Allen, R. G., Pereira, L. S., Raes, D., Smith, M. (1998) Crop evapotranspiration: Guideline for computing, crop water requirements ( FAO No. 56). Rome: Food and Agriculture Organization.
- Attalla, A. M., Abdel-Sattar, M., Mahrous, A. E., & Abdel-Azeez, A. A. (2011). Olive trees productivity in response to supplemental irrigation under North-Western Coastal conditions in Egypt. American-Eurasian Journal of Agricultural & Environmental Sciences, 11, 609–615.
- Attia, M. A., & Barsoum, M. S. (2013). Effect of supplementary irrigation and bio-fertilizer on wheat yield productivity under rain fed conditions. Alexandria Journal of Agricultural Research, 58, 149–157.
- El-Kosary, S., Abdel-Mohsen, M. A., El-Merghany, S., & Badran, A. M. (2013). Enhancing the productivity of early grand peaches under Northern Sinai conditions via supplemental irrigation and organic fertilization. Journal of Horticultural Science & Ornamental Plants, 5, 77–88.
- El-Mesiry, T., Gaballah, M. S., & Ouda, S. A. (2007). Using yield-stress model in irrigation management for wheat grown under saline conditions. Australian Journal of Basic and Applied Sciences, 1, 600–609.
- El-Sadek, A., & Salem, E. (2015). Impact of ridge–furrow water harvesting system on faba bean (Vicia faba L.) production under rainfed conditions in Matrouh, Egypt. Annals of Agricultural Sciences, 60, 61–66.10.1016/j.aoas.2015.03.003
- Estefanous, A. N., Mikhaeel, F. T., & Anton, G. G. (1997). Effect of mycorrhizal inoculation and organic fertilization on microbial activity and nutrient release in soil. Bulletin of the Faculty of Science of Cairo University, 48, 187–200.
- FAO. (2003). Food insecurity in the world. Author. ISBN 92-5-104986-6
- Hussain, S. (2005). Effect of supplemental irrigations, seeding rates and foliar application of potassium and macro-micro elements on wheat productivity under rain fed conditions. Scientific Journal of Collage of Agriculture, 431–454.
- IPCC. (2013). Climate change 2013: The physical science basis. In T. F. Stocker, D. Qin, G.-K. Plattner, M. Tignor, S. K. Allen, J. Boschung, … P. M. Midgley (Eds.), Contribution of working Group I to the fifth assessment report of the intergovernmental panel on climate change (1535 pp.). Cambridge: Cambridge University Press. doi:10.1017/CBO9781107415324
- IPCC. (2001). Climate change 2001: Impacts, adaptation, and vulnerability ( Report of working Group II of the Intergovernmental Panel on Climate Change (6th Session)). Geneva.
- Kamel. (2011). Cultural practices to combat degradation under rainfed areas in the Northern Coastal plain in Egypt. International Journal of Water Resources and Arid Environments, 1, 304–311.
- Khalifa, H. E., Sherif, M. A., El-Melegy, A., Abo Elenien, R. A., Heliela, H., & Owies, T. (2004). Crop rotations and fertilizer type effects on barley production and water-use efficiency at the rain fed area of North Sinai. In R. Ragab (Ed.), International workshop on management of poor quality water for irrigation; institutional, health and environmental aspects (pp. 241–249). Wallingford: Centre for Ecology and Hydrology (CEH).
- Khalil, F. A., Ouda, S. A., & Ewias, M. (2008). Comparison between CROPWAT and yield-stress models in predicting sesame yield and consumptive use under water stress conditions. Journal of Agricultural Science, 33, 9105–9119.
- Khalil, F. A., Ouda, S., & Tantawy, M. M. (2007). Predicting the effect of optimum irrigation and water stress on yield and water use of barley. Journal of Applied Sciences Research, 3(1), 1–6.
- Kumar, N. M., Murthy, C. S., SeshaSai, M. V. R., & Roy, P. S. (2009). On the use of standardized precipitation index (SPI) for drought intensity assessment. Meteorological Applications. doi:10.1002/met.136
- Mohy El-Din, M. Kh., El-Begawy, H. M., Shabbara, H., & Yassen, Y. A. (2013). The economic revenue of uploading some field crops and legumes on the olive trees in north and middle Sinai. Journal of Applied Sciences Research, 9, 1543–1553.
- Monteith, J. L. (1965). Evaporation and environment. In G. E. Fogg (Ed.), Symposium of the society for experimental biology: The state and movement of water in living organisms (pp. 205–234). New York, NY: Academic Press.
- Morsy, M. (2015). Use of regional climate and crop simulation models to predict wheat and maize productivity and their adaptation under climate change (PhD thesis). Faculty of Science Al-Azhar University, Cairo.
- Mosavi, A., Jafarzadeh, A., Nishabouri, M. R., Ostan, Sh, Feiziasl, V., & Karimi, E. (2012). The effect of different green manure application in dry land condition on some soil physical properties. S. B. International Journal of Agriculture and Crop Sciences, 4, 1233–1239.
- Ouda, S. A. (2006). Predicting the effect of water and salinity stresses on wheat yield and water needs. Journal of Applied Sciences Research, 2, 746–750.
- Pereira, L. S. (2005). Water and agriculture: Facing water scarcity and environmental challenges. Agricultural Engineering International: The CIGR Journal of Scientific Research and Development (Invited Overview Paper), VII, 2–26.
- Samarah, N. H. (2005). Effects of drought stress on growth and yield of barley. Agronomy for Sustainable Development, 25, 145–149.10.1051/agro:2004064
- Shams, A. S., & Kamel, A. S. (2014). Rotations in coastal plains to combat desertification in Egypt. International Journal of Water Resources and Arid Environments, 3, 121–131.
- Snyder, R.L., Organ, M., Bali, K., & Eching, S. (2004). Basic irrigation scheduling BIS. Retrieved from http://www.waterplan.water.ca.gov/landwateruse/wateruse/Ag/CUP/California_Climate_Data_010804.xls
- Sofo, A., Manfreda, S., Fiorentino, M., Dichio, B., & Xiloyannis, C. (2008). The olive tree: A paradigm for drought tolerance in Mediterranean climates. Hydrology and Earth System Sciences, 12, 293–301. Retrieved from www.hydrol-earth-syst-sci.net/12/293/200810.5194/hess-12-293-2008
- Solomon, S., Qin, D., Manning, M., Alley, R. B., Berntsen, T. N., Bindoff, L., … Wratt, D. (2007). Technical summary. In Climate change 2007: The physical science basis (Contribution of Working Group I to the Fourth Assessment Report of the Intergovernmental Panel on Climate Change, 996 pp.). Cambridge: Cambridge University Press.
- Wayne, G. (2013, August). The beginner’s guide to representative concentration pathways (Version 1.0).
- Wortmann, C. S., & Shapiro, C. A. (2008). The effects of manure application on soil aggregation. Nutrient Cycling in Agroecosystems, 80, 173–180.