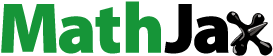
Abstract
Time-dependent aqueous extraction of six tea types was carried out with leaf–water–ratio of 0.5 g/100 ml, temperature of extraction 90°C and time of extraction ranging from 1 to 10 min. UV–vis spectroscopic analysis in the range varying from 220 to 900 nm of the aqueous tea extracts showed a prominent peak at 273 nm in the ultraviolet region which can be associated with n → π* electronic transition of caffeine molecules. Parabolic diffusion, Power law, hyperbolic, Weibull’s and Elovich’s models were fitted to represent the aqueous soluble component extraction behaviour for time-dependent extraction of aqueous extractables. Parabolic diffusion model, Power law and Elovich’s model were a close fit to the experimental data for all the selected tea types with correlation coefficients (R2) ranging 0.8029–0.9953, whereas hyperbolic and Weibull’s models showed poor fitness to represent the extraction behaviour of fanning and AO leaf, LD, fanning and dust, respectively, with R2 < 0.8, for time-dependent aqueous soluble component extraction.
Public Interest Statement
In Middle Eastern countries, the tea beverage is prepared by boiling black tea leaves in a pan for several minutes prior to consumption together with water, milk and sugar. The varied product and preparation differences on the in-cup composition of tea infusion are of importance, because the quality and health properties of the tea beverage are associated with the chemical components extracted from the leaf during brewing. In this study, the time-dependent aqueous extraction behaviour of different tea types has been observed using some solid–liquid extraction models. Fitting of these models validates the extraction behaviour of active aqueous components of tea. This study can ensure the maximum release of desirable components and lesser release of undesirables, complemented with an attractive colour.
Competing interests
The authors declare no competing interests.
1. Introduction
Tea is a non-alcoholic beverage consisting of infusions of the processed and dried leaves of the tea plant, Camellia sinensis (L). It is prepared from the young, tender leaves of tea plant which undergo different unit operations to give various types of teas as black, green and oolong depending on the extent of fermentation. The distribution of bioactive components, especially polyphenols, varies in differently fermented teas, with green tea having the highest concentration of polyphenols such as catechins and black tea having the highest concentration of oxidized polyphenols such as theaflavins and thearubigins. The tea polyphenols and presence of other volatile and non volatile components are responsible for its various health benefits (Ahmad et al., Citation2013, Citation2014; CitationAhmad, Baba, Gani, et al., 2015; CitationAhmad, Baba, Wani, et al., 2015; Shah et al., Citation2015). The tea preparation methods (e.g. leaf to water ratio (LWR), duration of infusion, temperature of extraction/infusion and amount of agitation used) vary worldwide among countries and among individuals within countries as revealed by worldwide consumer observations and questionnaire studies. In Western countries, black tea is drunk which is made by infusing tea leaves with or without a bag in boiling water in a pot or in a cup. It is infused for less than 3 min and the beverage is consumed hot, either with or without added milk and/or sugar. In the Indian sub-continent and some Middle Eastern countries, the tea beverage is prepared by boiling black tea leaves in a pan for several minutes prior to consumption together with water, milk and sugar. The varied product and preparation differences on the in-cup composition of tea infusion are of importance because the quality and health properties of the tea beverage are associated with the chemical components extracted from the leaf during brewing. A number of epidemiological and intervention studies have revealed a relationship between tea consumption and reduced risk of cardiovascular disease and cancer (Astill, Birch, Dacombe, Humphrey, & Martin, Citation2001). Some of the factors affecting the rate of infusion of tea solubles into aqueous solution have been widely studied. Natarajan et al. (Citation1962) studied the influence of different variables such as nature of raw material, the purity and temperature of the water, the infusion time and the water-to-leaf ratio on the brewing behaviour of tea. The rate of extraction of the soluble constituents has been found to increase with decreased particle size, increased brewing temperature and increased water-to-leaf ratio. Spiro and Siddique (Citation1981) and Spiro and Jago (Citation1982) used simple kinetic models to explain the observed rates of extraction of individual tea constituents into aqueous solution. The extracted tea constituents were measured as a function of time, when tea is infused in water at a constant elevated temperature. The concentration of tea soluble was found to increase in the liquor with increase in brew time. The rate of increase in concentration of tea soluble with increased brewing time gradually falls off to equilibrium. The diffusion of the solute through the leaf matrix to the surface was determined to be the rate-determining step in the loose leaf infusion. Price and Spiro (Citation1985) reported the effect of leaf size, origin and manufacture on the rate of infusion of caffeine and theaflavin. With decreased leaf size rate constants increased. Spiro, Jaganyi, and Broom (Citation1992) and Price and Spitzer (Citation1994) compared the rates of caffeine infusion from green and black teas and found that the rate of infusion of caffeine was greater from green tea than from a similarly sized black tea, confirming a possible effect of manufacturing method upon infusion characteristics.
The refreshing and soothing properties of tea are due to the hundreds of bioactive compounds such as polyphenols and caffeine, which are water soluble and are readily transferred to the hot brew. Efficient brewing shall contribute to the maximum release of desirable components and lesser release of undesirables, complemented with an attractive colour. In the present study, the time-dependent aqueous extraction behaviour of different tea types has been observed using some solid–liquid extraction models.
2. Materials and methods
Three black tea types (Assam Orthodox Leaf Grade, Assam Orthodox FBOP Grade and Assam Orthodox Dust Grade) used in this study were procured from the Poobong Tea Co. Ltd. Assam, India. Other three samples (Lipton Darjeeling, red label and fannings) were procured from the local market of Sungrur, Punjab, India (Figure ).
Figure 1. Different tea types used in the study: (A) AO LEAF, (B) LD, (C) FBOP, (D) RL, (E) FAN and (F) DUST.
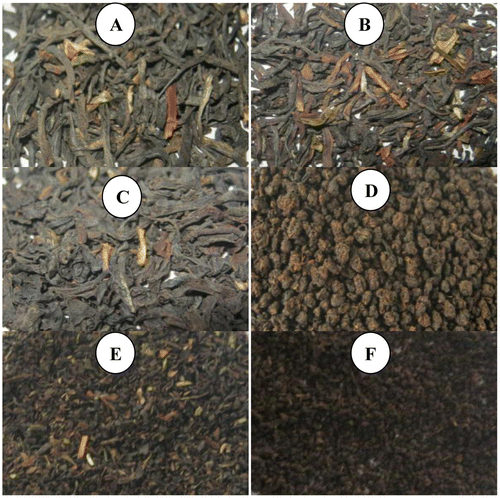
2.1. Parameters for aqueous extraction
Time-dependent aqueous extraction was carried out for the selected tea types with LWR of 0.5 g/100 ml, temperature of extraction as 90°C and extraction time ranging from 1 to 5 min and finally at 10 min interval.
2.2. UV–vis spectral analysis of the aqueous tea extract
UV–vis spectroscopic analysis in the range varying from 220 to 900 nm of the aqueous tea extracts showed a prominent peak at 273 nm in the ultraviolet region without any distinct peak in visible region (Figure ). Based on these findings, the subsequent experimentations were concentrated in ultraviolet spectroscopic analysis. Available empirical kinetic models were used to describe the behaviour of time-dependent extraction of the aqueous extractable tea components in terms of extraction yield at 273 nm.
2.3. Extraction models and model fitting
Available empirical models representing the extraction kinetics of present process in case of tea component extraction are represented (Table ). Fitting of these models and validation of extraction behaviour for active aqueous components of tea have been assessed. The considered models have also been used elsewhere in representing the extraction behaviour (Kitanović, Milenović, & Veljković, Citation2008; Cheung, Siu, & Wu, Citation2013).
Table 1. Empirical kinetic models of solid–liquid extraction
2.4. Adequacy and validation of model fitting
The regression model adequacy was assessed using statistical parameters such as coefficient of multiple determinations (R2) together with statistical significance indexes, F and p values (Peck & Devore, Citation2012).
3. Results and discussion
When electromagnetic radiation of the correct frequency is absorbed by a compound, an electron transition occurs from bonding molecular orbitals (σ or π) or non-bonding orbitals (n) to antibonding molecular orbitals (σ* or π*) (Figure ). UV–vis spectroscopic analysis of the aqueous tea extracts showed a prominent peak at 273 nm in the ultraviolet region. This region can be associated with n → π* electronic transition of caffeine molecules. The absorption band around 275 nm is related to the C=O chromophore absorption of caffeine (Souto et al., Citation2010). This transition kinetics of aqueous extractables of tea is associated with electron excitation from loan-pair orbital localized on the oxygen to the π* orbital of the carbonyl group of caffeine (Swarbrick, Citation2006).
Figure shows the change in the extraction yield (in terms of OD) with time for the six different tea types. In case of dust and fannings, a higher initial yield is observed which may be due to their finer particle size and an increased surface area which may enhance the extraction efficiency of water. AO Leaf and LD showed a comparatively lower initial yield than dust and fannings, which may be due to twisted and folded leaf structures which take some time to hydrate and allow water penetration. FBOP showed the lowest initial extraction yield, which may be due to its lowest bulk density compared to other types, which makes it float on the water surface until its leaves get hydrated and swollen.
Table shows the coefficient of multiple linear regression fit of the experimental data to different kinetic models for the different tea types and the corresponding model parameters. Parabolic diffusion model showed good fitness to represent the extraction behaviour for all the selected tea types with R2 ranging from 0.9277 to 0.9930. It characterizes a two-stage extraction process, an initial washing stage (to an initial yield A0) followed by a slow stage (with yield increasing linearly with t1/2).The tea suspended in water usually aggregated into soft and loose flocks which was more accessible to the water. Figure shows the plot of extraction yield versus time for the selected tea types with parabolic diffusion model. The Power law also satisfactorily represented the extraction behaviour of the selected tea types with R2 ranging from 0.9208 to 0.9923. This model is the most applicable for extraction of a substance from a non-swelling device (Sinclair & Peppas, Citation1984) with a diffusion exponent n < 1 for extraction of plant materials (Kitanović et al., Citation2008). As observed during the experiments, tea leaves/grains appeared well dispersed in water, suggesting a higher degree of rigidity and similarity to a non-swelling device. Figure shows the plot of extraction yield vs. time for the selected tea types with linearized Power law model. The hyperbolic model showed good fitness to represent the extraction behaviour of the selected tea types except fanning with R2 ranging from 0.8854 to 0.9953. This model represents an extraction kinetic behaviour that is first order, with the yield increasing linearly with time in the initial stage and zero order in the very late stage, with the yield reaching maximum. All the tea types, except fannings, showed the same extraction behaviour, which shows a little deviation from this extraction behaviour (Figure ). This deviated extraction behaviour of fannings makes hyperbolic model unsuitable for it with the R2 of <0.8. Figure shows the plot of extraction yield vs. time for the selected tea types except fanning with linearized hyperbolic model. The Weibull’s model could only represent the extraction behaviour of FBOP and RL with R2 of 0.8029 and 0.8155, respectively, although this model is successfully applied in nuclide release from paraffin waste (Kitanović et al., Citation2008). Figure shows the plot of extraction yield vs. time for FBOP and RL with linearized Weibull’s model. The Elovich’s model also showed good fitness to represent the extraction behaviour of all the selected tea types with large R2 values ranging from 0.9040 to 0.9911. This model is a logarithmic relation fitted to the leaching curves and derived by assuming that leaching rate decreases exponentially with increasing extraction yield. Figure shows the plot of extraction yield vs. time for the selected tea types with Elovich’s model. A kinetic model showing a close fit to the experimental data with a large coefficient of multiple regression (R2) value also had a large F and small p value from regression, indicating the statistical significance of the model fit (Tables and ).
Table 2. Correlation coefficients (R2) and model constants for various kinetic models fitted by linear regression to the aqueous extraction experimental data (extraction yield q vs. time)
Table 3. Statistical significance indexes (F and relative p-values) for the linear regression fit of various kinetic models to the aqueous component extraction of tea experimental data (corresponding to Table )
4. Conclusion
The absorption peak observed at 273 nm of the tea extracts owes to n → π* electronic transition of caffeine molecules. Extraction behaviour as represented through parabolic diffusion model, Power law and Elovich’s models was a close fit to the experimental data for all the selected tea types except for hyperbolic and Weibull’s models which showed poor fit to represent the extraction behaviour of fanning and AO leaf, LD, fanning and dust, respectively.
Nomenclature | ||
AO leaf | = | Assam orthodox leaf grade |
LD | = | Lipton Darjeeling |
FAN | = | fannings |
FBOP | = | flowery broken orange pekoe |
RL | = | red label |
q̅ | = | extraction yield (=absorbance of extractable substances extracted at 273 nm/absorbance of maximum extractables extracted at 273 nm) |
A0 | = | initial yield |
A1 | = | diffusion coefficient |
B | = | rate constant |
n | = | diffusion exponent (kinetic order) |
C1 | = | extraction rate at the very beginning (min−1) |
C2 | = | constant related to maximum extraction yield (capacity constant) |
D | = | scale parameter of the Weibull equation (min m) |
m | = | shape factor of the Weibull equation |
E0 | = | initial yield |
E1 | = | initial extraction rate |
UV | = | ultraviolet |
Additional information
Funding
Notes on contributors
Asir Gani
Adil Gani is an assistant professor in the Department of Food Science and Technology, University of Kashmir, Srinagar. He is currently working on different projects viz: Nutraceutical potential of β-glucan, its utilization for making functional foods and as an encapsulating material for target delivery of probiotics, Wheat flour modification by bacterial, enzymatic and chemical interventions to combat coeliac disorders, Extraction of resistant starch from rice and horse chestnut and its utilization as an encapsulating agent for targeted delivery into the colon and Safety, Quality and Nutraceutical Status of Kradi—A traditional Dairy based fermented food of Himalayan Regions of J& K. Apart from this, he is also associated in teaching, training and other related activities of the University/Department.
References
- Ahmad, M., Baba, W. N., Gani, A., Wani, T. A., Gani, A., & Masoodi, F. A. (2015). Effect of extraction time on antioxidants and bioactive volatile components of green tea (Camellia inensis), using GC/MS. Cogent Food & Agriculture, 1, 1106387. doi:10.1080/23311932.2015.1106387
- Ahmad, M., Baba, W. N., Shah, U., Gani, A., Gani, A., Riyaz, A., & Masoodi, F. A. (2014). Nutraceutical properties of the green tea polyphenols. Journal of Food Processing and Technology, 4, 276. doi:10.4172/2157-7110.1000276
- Ahmad, M., Baba, W. N., Wani, T. A., Gani, A., Gani, A., Shah, U., … Masoodi, F. A. (2015). Effect of green tea powder on thermal, rheological & functional properties of wheat flour and physical, nutraceutical & sensory analysis of cookies. Journal of Food Science and Technology, 52, 5799–5807.
- Ahmad, M., Gani, A., Baba, W. N., Gani, A., Wani, S. M., Shah, A., … Rather, S. A. (2013). Effect of extraction time on physiologically important constituents of green tea (Camellia sinensis) Using GC/MS. Journal of Food Processing and Technology, 4, 276. doi:10.4172/2157-7110.1000276
- Astill, C., Birch, M. R., Dacombe, C., Humphrey, P. G., & Martin, P. T. (2001). Factors affecting the caffeine and polyphenol contents of black and green tea infusions. Journal of Agricultural and Food Chemistry, 49, 5340–5347.10.1021/jf010759+
- Cheung, Y. C., Siu, K. C., & Wu, J. Y. (2013). Kinetic models for ultrasound-assisted extraction of water-soluble components and polysaccharides from medicinal fungi. Food Bioprocess Technology, 6, 2659–2665.
- Kitanović, S., Milenović, D., & Veljković, V. B. (2008). Empirical kinetic models for the resinoid extraction from aerial parts of St. John’s wort (Hypericum perforatum L.). Biochemical Engineering Journal, 41, 1–11.10.1016/j.bej.2008.02.010
- Natarajan, C. P., Ramamani, S., Leelavathi, D. E., Shakunthala, R., Bhatia, D. S., & Subrahmanyan, V. (1962). Studies on the brewing of tea. Food Science (Mysore), 11, 321–332.
- Peck, R., J. L.Devore (2012). Statistics: the exploration and analysis of data (7th ed.). Boston, MA: Brooks/Cole, Cengage Learning
- Price, W. E., & Spiro, M. (1985). Kinetics and equilibria of tea infusion: Rates of extraction of theaflavin, caffeine and theobromine from several whole teas and sieved fractions. Journal of the Science of Food and Agriculture, 36, 1309–1314.10.1002/(ISSN)1097-0010
- Price, W. E., & Spitzer, J. C. (1994). The kinetics of extraction of individual flavanols and caffeine from a Japanese green tea (Sen Cha Uji Tsuyu) as a function of temperature. Food Chemistry, 50, 19–23.10.1016/0308-8146(94)90086-8
- Sinclair, G. W., & Peppas, N. A. (1984). Analysis of non-fickian transport in polymers using simplified exponential expressions. Journal of Membrane Science, 17, 329–331.10.1016/S0376-7388(00)83223-8
- Shah, S., Gani, A., Ahmad, M., Shah, A., Gani, A., & Masoodi, F. A. (2015). In-vitro antioxidant and antiproliferative activity of microwave extracted green tea and black tea (Camellia sinensis): A comparative study. NutraFoods., 14, 207–215. doi:10.1007/s13749-015-0036-7
- Souto, U. T. C. P., Pontes, M. J. C. P., Silva, E. C., Galvão, R. K. H., Araújo, M. C. U., Sanches, F. A. C., … Oliveira, M. S. R. (2010). UV–vis-spectrometric classification of coffees by SPA–LDA. Food Chemistry, 119, 368–371.10.1016/j.foodchem.2009.05.078
- Spiro, M., Jaganyi, D., & Broom, M. C. (1992). Kinetics and equilibria of tea infusion: Part 9. The rates and temperature coefficients of caffeine extraction from green Chun Mee and black Assam Bukial teas. Food Chemistry, 45, 333–335.10.1016/0308-8146(92)90033-X
- Spiro, M., & Jago, D. S. (1982). Kinetics and equilibria of tea infusion. Journal of Chemical Society, Faraday Transaction 1, 78, 295–305.10.1039/f19827800295
- Spiro, M., & Siddique, S. (1981). Kinetics and equilibria of tea infusion (2): Kinetics of extraction of theaflavins, thearubigins and caffeine from Koonsong broken pekoe. Journal of the Science of Food and Agriculture, 32, 1135–1139.10.1002/(ISSN)1097-0010
- Swarbrick, J. (2006). Spectroscopy of pharmaceutical solids (pp. 121–147). New York, NY: Taylor & Francis Group, LLC.