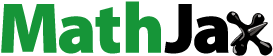
Abstract
Among the pathologic hypotheses of Alzheimer’s disease (AD), cholinergic deficit and oxidative stress have been implicated as two major hallmarks. Therefore, inhibition of cholinesterase and oxidation are the two promising strategies in the development of a drug for AD. Thunbergia grandiflora leaf extract (TGEx) is used in this research to investigate its anticholinesterase and antioxidant potentials. Anticholinesterase activity was measured by modified Ellman method. Antioxidant potentials were evaluated by the assay of reducing power, radical scavenging and inhibition of lipid peroxidation. The Methanolic extract showed strong anticholinesterase effect. Additionally, the extract exhibited pronounced reducing capacity, radical scavenging ability, and lipid peroxidation inhibitory effect. The IC50 values of the extract for DPPH and hydroxyl free radical scavenging and lipid peroxidation were 10.50 ± 0.68, 24.98 ± 1.39 and 21.84 ± 0.91 μg/ml, respectively. Phytochemical screening of the extract revealed the presence of significant amount of total phenolics and flavonoids. The tested sample reflects potential antioxidative and anticholinesterase inhibitory effect which may warrant its effectiveness in the treatment of AD.
Public Interest Statement
Alzheimer's disease is one of the leading neurodegenerative diseases caused mainly due to the oxidative stress. The current therapeutic approach for this disease is based on the use of synthetic drugs which have a number of side effects. They are expensive as well. Therefore, an alternative treatment with indigenous medicinal plant or plant extracts has increased very rapidly. As part of the alternative source to treat Alzheimer's disease, Thunbergia grandifolia extract has been chosen for this study, which has been found to have a very strong effect to reduce oxidative stress and expected to be useful in Alzheimer's disease treatment.
Competing Interests
The authors declare no competing interest.
1. Introduction
Alzheimer’s disease (AD), the major life-threatening neurodegenerative disorder, is responsible for 60% of all dementia in the people having age more than 65 (Ali-Shtayeh & Jamous, Citation2008; Singhal, Bangar, & Naithani, Citation2012). This disorder is characterized by impairment of learning, cognitive deficits, and behavioral disturbance. Around 35 million people are now afflicted by AD and it is believed to reach 65.7 million by 2030. Currently, AD is the fourth leading cause of death in the elderly person (Abou-Donia, Darwish, Toaima, Shawky, & Takla, Citation2014; Koedam et al., Citation2010).
Pathophysiology of AD is complex and several pathogenic pathways are involved in the progression of this disease. The cholinergic deficit, oxidative stress, the formation of neurofibrillary tangles (Wischik, Harrington, & Storey, Citation2014), and deposition of senile plaque hypothesize the main hallmark of AD (Esiri, Citation2001; Selkoe, Citation2001). Based on these hallmarks several lines of pharmacological treatment such as cholinergic inhibitors, antioxidant therapy, neurotransmitter replacement, anti-inflammatory therapy, stimulation of muscarinic receptors, prevention of tau aggregation, prevention of tau hyperphosphorylation, an increase of alpha-secretase activity, inhibition of β and γ-secretase inhibitors have been developed (Small & Mayeux, Citation2005; Tanzi & Bertram, Citation2001). Among all the pathologic conditions of AD, the cholinergic deficit is remarked as one of the main reasons of AD. Several strategies are available to improve cholinergic neurotransmission including stimulation of cholinergic receptors or increasing the ability of ACh release in the synaptic cleft by inhibiting ACh hydrolysis with the acetylcholinesterase (AChE) enzyme (Nilsson, Nordberg, Hardy, Wester, & Winblad, Citation1986). Similarly, butyrylcholinesterase (BChE) enzyme singly or synergistically with AChE hydrolyzes ACh as well as butyrylcholine in the nerve endings (Mesulam et al., Citation2002). In the AD brains, increase level of BChE correlates positively with the development of AD.
Apart from these, an association of reactive oxygen species (ROS) is also evident to cause the pathogenesis of AD. Oxidative stress initiates the death of neuron through several cellular reactions like lipid peroxidation, protein oxidation, ROS formation, intracellular and mitochondrial calcium accumulation (Konrath et al., Citation2012; Sultana et al., Citation2006). The use of antioxidants has been considered a good attempt to slow down the progression of AD and neuronal degeneration (Miao, He, & Zhu, Citation2010; Ramassamy, Citation2006). Therefore, inhibition of cholinesterase and oxidative stress devised as a suitable strategy for providing symptomatic treatment to AD as well as other types of dementia.
Thunbergia grandiflora Roxb., commonly known as Nallata (Acanthaceae) is a large climbing or twining shrub, found all over the world including Bangladesh (Motaleb, Hossain, Alam, Abdullah-Al-Mamun, & Sultana, Citation2013). The plant is widely distributed throughout Bangladesh, especially in forests of Gazipur, Chittagong, Chittagong hill tracts, Cox’s Bazar and Tangail (Medicinal Plants Database of Bangladesh, Citation2015). The species is used for the treatment of blood dysentery, cataract, diabetes, gout, hydrocele, hysteria, malaria, marasmus, post eclampsia, pre-eclampsia, rheumatism, spermatorrhoea (Uddin, Citation2006), stomach complaints (Ahmed et al., Citation2008), ophthalmia, conjunctivitis (Bor & Raizada, Citation1954), elephantiasis, and urinary bladder stone (Ahmed et al., Citation2009). Phytochemical studies of T. grandiflora confirmed several active compounds including iridoid glycosides, isounedoside, and grandifloric acid (Ismail, El-Azizi, Khalifa, & Stermitz, Citation1996). The plant has also been reported to possess important pharmacological effects including antibacterial (Jeeva, Johnson, Aparna, & Irudayaraj, Citation2011), anti-inflammatory and anti-arthritic effect (Alam et al., Citation2015). Due to enormous pharmacological and ethnobotanical benefits of T. grandiflora, this research aimed to investigate whether antioxidative and anticholinesterase potentials to treat Alzheimer’s disease, which has never been tried, are still unrevealed in this plant.
2. Methods
2.1. Plants materials
Leaves of T. grandiflora were collected from Chittagong, Bangladesh in January and February 2014. The plant was identified and authenticated by Dr Sheikh Bokhtear Uddin, taxonomist, and professor of the Department of Botany, Chittagong University. A voucher specimen is deposited at the Department of Pharmacy, International Islamic University Chittagong, Bangladesh.
2.2. Extract preparation
Freshly collected green leaves were air-dried in a shade. The dried leaves were cut into small pieces and ground into fine powder using a dry grinder. Resulting powder (500 g) was placed into an amber bottle and subjected to extraction with methanol at room temperature for a week with occasional shaking. After 7 days the whole mixture was filtered by Whatman No. 1 filter papers and the filtrate was finally concentrated to collect around 14.59 g (2.91% w/w) crude methanolic extract.
2.3. Enzyme sources
Adult Long-Evans rat (average weight 120–150 g; 80–100 days; both sexes) brain homogenate was used for the source of AChE enzyme. The rats were procured from the animal research branch of the International Centre for Diarrhoeal Disease and Research, Bangladesh (ICDDR, B). Human blood was used as a source of BChE enzyme. The experimental protocol was approved by the Institutional Ethics Committee of International Islamic University Chittagong.
2.4. Chemicals
Almost all chemicals were used in this study are analytical grade. Acetylthiocholine iodide, Butyryl lthiocholine iodide, Donepezil, Galantamine, Folin-Ciocalteu reagent, 2-deoxy-D-ribose, thiobarbituric acid, (+)-catechin, 5,5’ dithio-bis [2-nitrobenzoic acid] (DTNB), and Gallic acid were brought from Sigma-Aldrich (India). Aluminium chloride, Ammonium molybdate, Ascorbic acid, DPPH, Tris-HCl and Triton X-100 were obtained from Wako Pure Chemical Company Ltd. (Osaka, Japan).
2.5. Determination of total phenolic content
The quantification of total phenolic in the TGEx was done by the Folin-Ciocalteu method (Singleton, Orthofer, & Lamuela-Raventós, Citation1999). A 0.5 ml of plant extract at different concentrations was added to 2.5 ml of Folin-Ciocalteu (diluted 10 times with water) reagent and 2.5 ml of sodium carbonate (7.5%) solution. The reaction mixture was incubated for 20 min at 25°C to complete the reaction and the absorbance of the mixture was measured at 760 nm. The Gallic acid standard curve was used to quantify total phenolic contents and the results were expressed as mg of Gallic acid equivalent (GAE)/g of dried extract.
2.6. Determination of total flavonoid content
Total flavonoid of TGEx was determined using Aluminum chloride colorimetric method with slight modification (Zhishen, Mengcheng, & Jianming, Citation1999). Briefly, 1.0 ml of each of the concentrations of TGEx was mixed with 3.0 ml of methanol, 0.2 ml of 10% AlCl3, 0.2 ml of 1 M potassium acetate and 5.6 ml of distilled water was added to the resulting mixture which was then incubated at room temperature for 30 min to complete the reaction. The reaction mixture was recorded for absorbance at 420 nm. Gallic acid was used as standard and the results were expressed as milligrams of gallic acid equivalents per gram of dried fraction.
2.7. Determination of reducing power
The reducing power of TGEx was measured by the method described by Oyaizu (Citation1986) with the formation of Perl’s Prussian blue at 700 nm (Oyaizu, Citation1986). Various concentrations of the sample (1 ml) were mixed with 2.5 ml of phosphate buffer (0.2 M, pH 6.6) and 2.5 ml of potassium ferricyanide (10 mg/ml). The reaction mixture was incubated at 50°C for 20 min adding 2.5 ml of trichloro acetic acid (TCA, 100 mg/L) solution. The mixture was centrifuged at 3,000 rpm for 10 min to collect the upper layer. A small amount (2.5 ml) of solution from upper layer was mixed with 2.5 ml of distilled water and 0.5 ml of ferric chloride (0.1% w/v) solution. After 10 min reaction, the absorbance was measured at 700 nm. Reference standard used in this reaction was Ascorbic acid. The Higher absorbance of the reaction mixture indicates a higher reducing power.
2.8. Determination of DPPH radical scavenging activity
DPPH radical scavenging activity of the TGEx was determined according to the method described by Choi with minor modifications (Choi, Jhun, & Lim, Citation2000). A 2 ml of a methanol solution of plant extract or reference standard at different concentrations was mixed with 3 ml of a methanol solution of DPPH (0.135 mM) into the test tube. The reaction mixture was incubated at room temperature for 30 min in a dark place to complete the reaction. The absorbance of the solution was measured spectrophotometrically at 517 nm. Ascorbic acid was used as a positive control and methanol as a negative control. DPPH free radical scavenging ability (%) was calculated by using the formula:
2.9. Determination of hydroxyl radical scavenging activity
The hydroxyl free radical scavenging ability of the TGEx was determined by the method of Elizabeth and Rao (Citation1990) with a slight modification. Briefly, plant extract or reference compound at different concentrations was mixed with a reaction mixture contained, in a final volume of 1 ml: 2-deoxy-2-ribose (2.8 mM); KH2PO4-KOH buffer (20 mM, pH 7.4); FeCl3 (100 μM); EDTA (100 μM); H2O2 (1.0 mM); ascorbic acid (100 μM) and various concentrations of the test sample or reference compound catechin. The mixture was then incubated for 1 h at 37°C and 0.5 ml of the reaction mixture was heated at 90°C for 15 min after addition of 1 ml of 2.8% TCA and 1 ml of 1% aqueous TBA to develop the color. After cooling, the absorbance was measured at 532 nm against an appropriate blank solution. Hydroxyl radical scavenging ability (%) was calculated by using the formula:
2.10. Determination of lipid peroxidation inhibition activity
The lipid peroxidation inhibition capability of the TGEx was performed by the modified method described by Houghton, Zarka, de las Heras, and Hoult (Citation1995). In this method, the degree of lipid peroxidation was estimated by measuring the thiobarbituric acid reacting substances (TBARS). Rat brain was homogenized with a homogenizer in ice-cold phosphate buffer (50 mM, pH 7.4) with 0.15 M KCl to produce a 1/10 homogenate. The homogenate was centrifuged at 10,000 g for 15 min at 4°C and the resulting supernatant was used as liposome for in vitro lipid peroxidation assay. A 1 ml of 0.15 M KCl and 0.5 ml of brain homogenates and different concentrations of plant extract were mixed and the peroxidation reaction was initiated by adding 100 ml of 0.2 mM ferric chloride. After incubation at 37°C for 30 min, the reaction was stopped by adding 2 ml of ice-cold HCl (0.25 N) containing 15% TCA, 0.38% TBA, and 0.5% BHT. The reaction mixtures were heated at 80°C for 60 min. The samples were cooled, centrifuged, and the absorbance of the supernatants was measured at 532 nm. The percentage inhibition of lipid peroxidation was calculated by the formula:
2.11. Determination of AChE inhibitory activity
Ellman’s colorimetric method was applied to investigate the AChE inhibitory activity of the TGEx and acetyl-thiocholine iodide was used as a substrate (Ellman, Courtney, Andres, & Featherstone, Citation1961). For the enzyme source, the rat brains were homogenized in a homogenizer with ten volumes of a homogenization buffer (10 mMTris-HCl, pH 7.2) containing 1 M NaCl, 50 mM MgCl2 and1% Triton X-100 and centrifuged at 10,000 rpm for 30 min. The resulting supernatant was treated with supersaturated ammonium sulfate solution and supernatants were collected. A solution of these supernatants was used as an enzyme source. The temperature was maintained (4°C) throughout the excretion procedure. The rate of AChE hydrolysis was monitored spectrophotometrically. Each extract or standard (500 μl) was mixed with an enzyme solution (500 μl) and incubated at 37°C for 30 min. An absorbance at 405 nm was recorded immediately after adding Ellman’s reaction mixture (3.5 ml; 0.5 mM acetyl-thiocholine, 1 mM DTNB) in a 50 mM sodium phosphate buffer (pH 8.0) to the above reaction mixture. Readings were repeated for 30 min to verify the reaction. The blank was prepared by substituting buffer saline for the enzyme. Donepezil was used as a positive control. The percentage of AChE inhibition was calculated using the following formula:
2.12. Determination of BChE inhibitory activity
Modified Ellman’s colorimetric method was used to perform BChE inhibitory assay, where butyrylthiocholine iodide was used as a substrate (Ellman et al., Citation1961). For the BChE enzyme source, human blood from anonymous healthy men was provided by the International Islamic University Medical Center, Chittagong, Bangladesh and collected in EDTA-treated (1 mg/ml) glass tubes. The tubes were centrifuged at 2,000 g for 10 min to eliminate the red blood cells. The resulting plasma (supernatant) was then recuperated, diluted (1/200) with 50 mM phosphate buffer (pH 7.4) and was used immediately for studying BChE activity. The BChE hydrolysis rate was monitored spectrophotometrically. Each extract or standard (500 μl) was mixed with an enzyme solution (500 μl) and incubated at 37°C for 30 min. Absorbance at 410 nm was recorded immediately after adding Ellman’s reaction mixture (3.5 ml; 0.5 mM butyryl-thiocholine, 1 mM DTNB) in a 50 mM sodium phosphate buffer (pH 8.0) to the above reaction mixture. To verify the reaction, readings were repeated for 30 min. The blank reaction was measured by substituting saline for the enzyme. Galantamine was used as a reference standard. The percentage of BChE inhibition was calculated using the following formula:
2.13. Statistical analysis
The data was analyzed by one-way analysis of variance (ANOVA) followed by Dunnet’s test to estimate significant differences between the test and control groups with GraphPad Prism Data Editor for Windows, Version 6.0 (GraphPad Software Inc., San Diego, CA). Values were expressed as mean ± Standard error of mean (± SEM). p < 0.05 was considered as statistically significant.
3. Results and discussion
3.1. Total phenolics and flavonoid contents of T. grandiflora
This research aims to evaluate the antioxidative and anticholinergic effects of the TGEx. For assessing the antioxidative effects, total phenolic and flavonoid contents of TGEx, its reducing capability, DPPH radical scavenging capacity, hydroxyl radical scavening effect and lipid peroxidation inhibitory effects were screened. Total phenolic and flavonoid contents were calculated using the standard curve for Gallic acid. Phenolic compounds have been reported to show anti-Aβ (amyloid β protein) aggregation effects in AD indicating the pivotal role of phenolics in preventing the Alzheimer’s progression (Asaduzzaman et al., Citation2014; Dhalwal, Shinde, & Namdeo, Citation2008). Flavonoids are known to serve as potential antioxidants for their radical scavenging, ion chelating and lipid peroxidation inhibiting properties (Asaduzzaman et al., Citation2014; Kumarasamy, Byres, Cox, & Jaspars, Citation2007) preventing or slowing the progression of AD by interfering the generation of amyloid-β peptides and its polymerization into neurotoxic oligomeric aggregates thereby reducing aggregation of tau proteins (Dhalwal et al., Citation2008). Higher content of total phenolic (98.53 mg of GAE/g of dried extract) and flavonoid (191.76 mg of GAE/g of dried extract) in TGEx, therefore, may carry an immense importance to evaluate its role for controlling AD.
3.2. Reducing power of T. grandiflora
The reducing power of different TGEx fractions based on the reduction of the Fe3+-ferricyanide complex to the ferrous form is shown in Figure . Indeed, reducing power is linked with antioxidant capacity and compounds having reducing power indicate that they are electron donors and can reduce the oxidized intermediates of lipid peroxidation processes, so that they can act as primary and secondary antioxidants (Chanda & Dave, Citation2009). Yellow color of the reaction solution is changed to green and blue depending on the reducing power of the extract. Presence of reducers converts the Fe3+/ferricyanide complex to the ferrous form forming Pearl’s Prussian blue at 700 nm. This may be due to the decrease in the reducers which would have converted the Fe3+/ferricyanide complex to the ferrous form within a short time. In this assay, methanol extract showed the highest reducing capacity and it was significant (p < 0.05) compared to that of the reference standard, ascorbic acid (Table ).
Figure 1. Reducing power of T. grandiflora extract compared with the standard ascorbic acid as assessed by ferric reducing power assay.
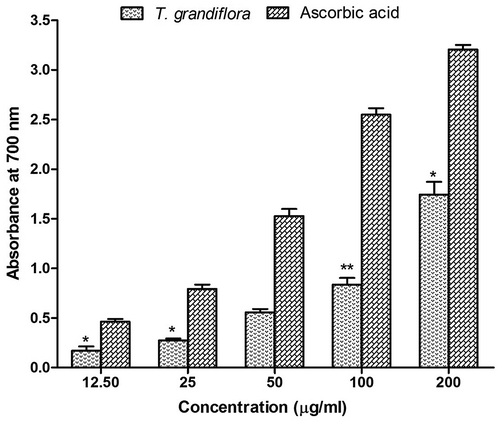
Figure 2. Radical scavenging activity of T. grandiflora extract compared with the standard. (A) Percent of DPPH radical scavenging by different concentrations of the extract and reference standard ascorbic acid as assessed by spectrophotometric method using DPPH free radicals. (B) Percent of hydroxyl radical scavenging by different concentrations of the extract and reference standard (+)-catechin as assessed by the Fenton-reaction initiated deoxyribose degradation method.
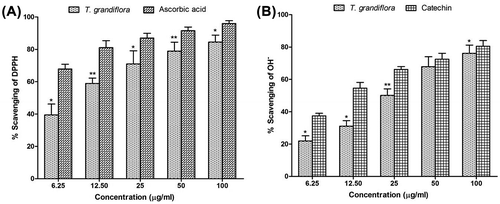
Figure 3. Percentage of lipid peroxidation inhibition at different concentrations of T. grandiflora extract and the reference standard (+)-catechin.
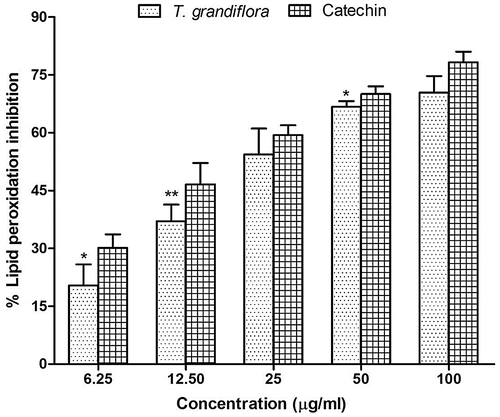
Figure 4. Percentage of inhibition of acetylcholinesterase (A) and butyrylcholinesterase (B) activity at different concentrations of T. grandiflora extract and the reference standard donepezil and galantamine, respectively.
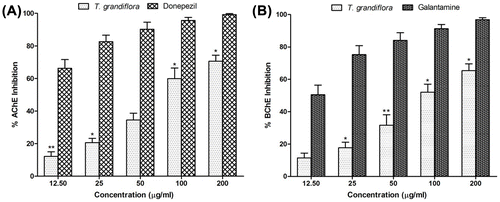
Table 1. IC50 values of the test extract and standards obtained in the radical scavenging and enzyme inhibitory activity assays
3.3. DPPH radical scavenging activity of T. grandiflora
Radical scavenging activity is very important to prevent the deleterious role of free radicals in AD (Moniruzzaman et al., Citation2015). DPPH is a stable free radical and DPPH antioxidant assay is based on the ability of DPPH to decolorize in the presence of antioxidants. The DPPH radical contains an odd electron, which is responsible for a visible deep purple color and maximum absorption at 517 nm. Decolorization of DPPH after accepting an electron donated by an antioxidant compound is quantitatively measured from the change in absorbance and % of scavenging activity. DPPH radical scavenging assay for TGEx is shown in Figure (A). The results of this study showed very strong DPPH scavenging activity with an IC50 value of 10.50 ± 0.68 μg/ml which was found to be comparable with that of the standard antioxidative agent ascorbic acid showing IC50 value 4.41 ± 0.27 μg/ml.
3.4. Hydroxyl radical scavenging activity of T. grandiflora
Hydroxyl radicals are the major reactive oxygen species that severely damage the neurons in AD (Kenche & Barnham, Citation2011). In the hydroxyl radical scavenging activity the ability of TGEx to remove the formed hydroxyl radical in solution was evaluated quantitatively by Fenton-reaction initiated deoxyribose degradation assay and the result has been shown in Figure (B). The test sample significantly scavenged the hydroxyl radicals generated in the reaction in a dose-dependent manner with an IC50 value of 24.98 ± 1.39 μg/ml which was significant compared to that (12.68 ± 0.63 μg/ml) of the reference standard (+)-catechin. When plant extracts and the reference compound, (+)-catechin, added to the reaction mixture they removed hydroxyl radicals from the sugar and prevented degradation. Removal of hydroxyl radicals from the reaction mixture decolorized the pink chromogen which was quantitatively measured from the change in absorbance at 532 nm. The results demonstrate very promising hydroxyl radical scavenging potentials for therapeutic uses because the cut-off value for antioxidative compounds is 1000 μg/ml (Chew, Jessica, & Sasidharan, Citation2012).
3.5. Lipid peroxidation inhibition activity of T. grandiflora
Reactive oxygen species produced by ultraviolet light, ionizing radiation, chemical reactions, and metabolic processes have numerous pathological effects, such as causing lipid peroxidation, protein peroxidation, DNA damage, and cellular degeneration related to a variety of diseases including Alzheimer’s disease (Arlt, Beisiegel, & Kontush, Citation2002; Baptista, Henriques, Silva, Wiltfang, & da Cruz e Silva, Citation2014; Nimmi et al., Citation2012). Lipid peroxidation has been reported to be elevated in the brain of AD. During lipid peroxidation, low molecular weight end products, generally malonaldehyde, are formed by oxidation of poly-unsaturated fatty acids that may react with two molecules of thiobarbituric acid to give a pink red chromogen (Grotto et al., Citation2009). In the lipid peroxidation inhibition activity, the activity of TGEx against non-enzymatic lipid peroxidation in rat brain homogenate was evaluated. Addition of Fe2+-ascorbate to the brain homogenate caused an increase in lipid peroxidation which was quantitatively measured from the change in absorbance at 532 nm and % of inhibition activity of different concentrations were shown in Figure . The TGEx showed that the inhibition of lipid peroxidation was correlated with the increasing concentration of the extract. The IC50 values of the methanol extract and the reference standard (+)-catechin were 21.84 ± 0.91 and 17.69 ± 0.57 μg/ml, respectively.
3.6. AChE inhibitory activity of T. grandiflora
Dysregulation in cholinergic neuron and oxidative imbalance are considered to be the key components in the pathogenesis of AD (Terry & Buccafusco, Citation2003). Therefore, a drug candidate that targets the cholinesterases and multiple components of the oxidative stress would be an effective lead drug candidate for the treatment of AD. Additionally, preventing breaking down of ACh is responsible for the elevation of ACh level in the synaptic cleft by inhibition of AChE is the most significant changes observed in AD (Terry & Buccafusco, Citation2003). The inhibitory activity of TGEx against rat brain AChE was determined by Ellman’s method (Ellman et al., Citation1961) which estimates the level of AChE using acetylthiocholine iodide (substrate) and DTNB. The inhibitory actions of TGEx was measured by the color produced by thiocholine. The inhibitory activity of TGEx was increased with the increasing concentration and the IC50 was 80.81 ± 3.02 μg/ml (Figure (A)). This dose-dependent inhibition of TGEx was significant (p < 0.05) compared to control but the IC50 values were higher than that (IC50 = 5.28 ± 0.23) of the reference drug donepezil.
3.7. BChE inhibitory activity of T. grandiflora
All the cholinesterase inhibitors currently licensed for AD, inhibit AChE and, to a varying extent, BChE (Bonda, Wang, & Perry, Citation2010; Massoud & Gauthier, Citation2010). Moreover, it has been reported earlier that dual inhibition of AChE and BChE might improve the signs of AD and symptoms owing to the key role of BChE in the hydrolysis of acetylcholine (Decker, Kraus, & Heilmann, Citation2008; Neugroschl & Sano, Citation2010). This research has studied the inhibitory actions of TGEx against BChE using the similar methods used for AChE. Similar to AChE inhibitory activity, the TGEx also had very good inhibitory actions against BChE with an IC50 value of 94.30 ± 3.17 μg/ml whereas galantamine showed IC50 value of 12.37 ± 0.56 (Figure (B)). Our results demonstrate a dual activity of T. grandiflora. However, methanol extract showed promising activity against BChE.
4. Conclusion
The obtained results from this bioassay indicated that the methanol extract of T. grandiflora possesses a substantial amount of polyphenolic compounds and displayed potential antioxidants and radical scavenging activities. The extract also has the capability to effectively inhibit AChE and BChE. The plant, therefore, may have promising compounds to be tested as potential drugs for the treatment of diseases resulting from oxidative stress and cholinergic dysfunction like AD. Due to the presence of significant antioxidant activity of this plant, further extensive studies are to be undertaken for isolation and identification of lead compound(s) for an effective and safe use in the treatment of neurodegenerative disorders including AD.
Abbreviations | ||
AD | = | Alzheimer’s disease |
DPPH | = | 1,1-Diphenyl 2-picrylhydrazyl |
AChE | = | Acetylcholinesterase |
BChE | = | Butyrylcholinesterase |
EDTA | = | Ethylenediaminetetraacetic acid |
TCA | = | Trichloroacetic acid |
TBA | = | Thiobarbituric acid |
DTNB | = | 5,5’ dithio-bis (2-nitrobenzoic acid) |
Tris-HCl | = | tris(hydroxymethyl)aminomethane hydrochloride |
Funding
The authors received no direct funding for this research.
Authors’ Contributions
MJU conceived and designed the experiments; MJU carried out the hydroxyl radical scavenging and anticholinesterase activity tests. MNA performed quantitative analysis and DPPH radical scavenging tests. KB conducted the reducing power and lipid peroxidation activity tests. MJU wrote the manuscript and performed statistical analysis. MAR has furnished the designing of the work, interpreted the data, formatted the manuscript, corrected the statistical analysis and shaped the whole manuscript designing. All authors read and approved the final manuscript.
Acknowledgement
The authors are grateful to the Department of Pharmacy, International Islamic University Chittagong, Bangladesh for providing necessary facilities to accomplish this research work. Authors cordially acknowledge the Department of Pharmacy, University of Rajshahi, Bangladesh for providing laboratory facilities with chemicals.
Additional information
Notes on contributors
Md. Atiar Rahman
Dr Md. Atiar Rahman is the principal investigator of the Laboratory of Alternative Medicine and Natural Product research of the Department of Biochemistry and Molecular Biology of the University of Chittagong, Bangladesh. His research group, comprised of PhD, MPhil and MS researchers, has been undertaking the exploration of newer alternative therapeutic sources to treat chronic diseases especially diabetes mellitus, hepatic damage and hyperlipidemia. Medicinally potential plants documented and surrounded over the country are the prime resources as alternative to synthetic drugs which do possess number of side effects. Alzheimer’s disease has recently been included his additional target because of its increasing prevalence over the country. This research focuses the antioxidative and anticholinesterase effects of Thunbergia grandiflora, which are two pivotal biological functions responsible for controlling Alzheimer’s disease. The plant material has been found to be very beneficial in reducing oxidative stress and inhibiting cholinesterase enzymes regarded with cognitive function. An advanced dose-response study can lead to the future formulation of Alzheimer’s disease.
References
- Abou-Donia, A. H., Darwish, F. A., Toaima, S. M., Shawky, E., & Takla, S. S. (2014). A new approach to develop a standardized method for assessment of acetylcholinesterase inhibitory activity of different extracts using HPTLC and image analysis. Journal of Chromatography B, 955, 50–57.10.1016/j.jchromb.2014.02.013
- Ahmed, Z. U., Begum, Z. N. T., Hassan, M. A., Khondker, M., Kabir, S. M. H., Ahmed, M., … Haque, E. U. (2008). Encyclopedia of flora and fauna of Bangladesh, angiosperms: Dicotyledons (Acanthaceae-Asteraceae) (Vol. 6, pp. 74–75). Dhaka: Asiatic Society of Bangladesh.
- Ahmed, Z. U., Hassan, M. A., Begum, Z. N. T., Khondker, M., Kabir, S. M. H., Ahmed, M., … Haque, E. U. (2009). Encyclopedia of flora and fauna of Bangladesh, angiosperms: Dicotyledons (Fabaceae-Lythraceae) (Vol. 8, pp. 40–41). Dhaka: Asiatic Society of Bangladesh.
- Alam, M. N., Biozid, M. S., Islam, M. R., Rahman, M. M., Chowdhury, A. I., & Uddin, M. M. (2015). In-vitro comparative study of anti-inflammatory and anti-arthritic effects of the methanol extract of Cissus pentagona Roxb and Thunbergia grandiflora Roxb leaf. The Pharma Innovation, 4, 39–42.
- Ali-Shtayeh, M. S., & Jamous, R. M. (2008). Traditional Arabic Palestinian Herbal Medicine, TAPHM. Til, Nablus: Biodiversity and Environmental Research Center.
- Arlt, S., Beisiegel, U., & Kontush, A. (2002). Lipid peroxidation in neurodegeneration: New insights into Alzheimerʼs disease. Current Opinion in Lipidology, 13, 289–294.10.1097/00041433-200206000-00009
- Asaduzzaman, M., Uddin, M. J., Kader, M. A., Alam, A. H. M. K., Rahman, A. A., Rashid, M., … Sadik, G. (2014). In vitro acetylcholinesterase inhibitory activity and the antioxidant properties of Aegle marmelos leaf extract, implications for the treatment of Alzheimer’s disease. Psychogeriatrics, 14, 1–10.10.1111/psyg.2014.14.issue-1
- Baptista, F. I., Henriques, A. G., Silva, A. M. S., Wiltfang, J., & da Cruz e Silva, O. A. (2014). Flavonoids as therapeutic compounds targeting key proteins involved in Alzheimer’s disease. ACS Chemical Neuroscience, 5, 83–92.10.1021/cn400213r
- Bonda, D. J., Wang, X., & Perry, G. (2010). Oxidative stress in Alzheimer disease: A possibility for prevention. Neuropharmacology, 59, 290–294.10.1016/j.neuropharm.2010.04.005
- Bor, N. L., & Raizada, M. B. (1954). Some beautiful indian climbers and shrubs (110–122). Bombay Natural History Society. Bombay: Oxford University Press.
- Chanda, S., & Dave, R. (2009). In vitro models for antioxidant activity evaluation and some medicinal plants possessing antioxidant properties: An overview. African Journal of Microbiology Research, 3, 981–996.
- Chew, A. L., Jessica, J. J. A., & Sasidharan, S. (2012). Antioxidant and antibacterial activity of different parts of Leucas aspera. Asian Pacific Journal of Tropical Biomedicine, 2, 176–180.10.1016/S2221-1691(12)60037-9
- Choi, H. Y., Jhun, E. J., & Lim, B. O. (2000). Application of flow injection-chemilumineacence to the study of radical scavenging activity in plants. Phytotherapy Research, 14, 250–253.10.1002/(ISSN)1099-1573
- Decker, M., Kraus, B., & Heilmann, J. (2008). Design, synthesis and pharmacological evaluation of hybrid molecules out of quinazolinimines and lipoic acid lead to highly potent and selective butyrylcholinesterase inhibitors with antioxidant properties. Bioorganic and Medicinal Chemistry, 16, 4252–4261.10.1016/j.bmc.2008.02.083
- Dhalwal, K., Shinde, V. M., & Namdeo, A. G. (2008). Antioxidant profile and HPTLC-densitometric analysis of umbelliferone and psoralen in Aegle marmelos. Pharmaceutical Biology, 46, 266–272.10.1080/13880200701741088
- Elizabeth, K., & Rao, M. N. A. (1990). Oxygen radical scavenging activity of curcumin. International Journal of Pharmaceutics, 58, 237–240.
- Ellman, G. L., Courtney, K. D., Andres, V., & Featherstone, R. M. (1961). A new and rapid colorimetric determination of acetylcholinesterase activity. Biochemical Pharmacology, 7, 88–95.10.1016/0006-2952(61)90145-9
- Esiri, M. M. (2001). The neuropathology of Alzheimer’s disease. In D. Dawbarn & S. J. Allen (Eds.), Neurobiology of Alzheimer’s disease (pp. 33–53). Oxford: Oxford University Press.
- Grotto, D., Maria, L. S., Valentini, J., Paniz, C., Schmitt, G., Garcia, S. C., … Farina, M. (2009). Importance of the lipid peroxidation biomarkers and methodological aspects FOR malondialdehyde quantification. Química Nova, 32, 169–174.10.1590/S0100-40422009000100032
- Houghton, P. J., Zarka, R., de las Heras, B., & Hoult, J. R. S. (1995). Fixed oil of nigella sativa and derived thymoquinone inhibit eicosanoid generation in leukocytes and membrane lipid peroxidation. Planta Medica, 61, 33–36.10.1055/s-2006-957994
- Ismail, L. D., El-Azizi, M. M., Khalifa, T. I., & Stermitz, F. R. (1996). Iridoid glycosides from Thunbergia grandiflora. Phytochemistry, 42, 1223–1225.10.1016/0031-9422(96)00071-4
- Jeeva, S., Johnson, M., Aparna, J. S., & Irudayaraj, V. (2011). Preliminary phytochemical and antibacterial studies on flowers of selected medicinal plants. International Journal Medicinal and Aromatic Plants, 1, 107–114.
- Kenche, V. B., & Barnham, K. J. (2011). Alzheimer’s disease & metals: Therapeutic opportunities. British Journal of Pharmacology, 163, 211–219.10.1111/bph.2011.163.issue-2
- Koedam, E. L. G. E., Lauffer, V., van der Vlies, A. E., van der Flier, W. M., Scheltens, P., & Pijnenburg, Y. A. L. (2010). Early-versus late-onset Alzheimer’s disease: More than age alone. Journal of Alzheimer’s Disease, 19, 1401–1408.
- Konrath, E. L., Neves, B. M., Lunardi, P. S., Passos, C. D. S., Simões-Pires, A., Ortega, M. G., … Henriques, A. T. (2012). Investigation of the in vitro and ex vivo acetylcholinesterase and antioxidant activities of traditionally used Lycopodium species from South America on alkaloid extracts. Journal of Ethnopharmacology, 139, 58–67.10.1016/j.jep.2011.10.042
- Kumarasamy, Y., Byres, M., Cox, P. J., & Jaspars, M. (2007). Screening seeds of some Scottish plants for free radical scavenging activity. Phytotherapy Research, 21, 615–621.10.1002/(ISSN)1099-1573
- Massoud, F., & Gauthier, S. (2010). Update on the pharmacological treatment of Alzheimers disease. Current Neuropharmacology, 8, 69–80.10.2174/157015910790909520
- Medicinal Plants Database of Bangladesh. (2015). Thunbergia grandiflora Roxb. Retrieved March, 22, 2015, from http://www.mpbd.info/plants/thunbergiagrandiflora.php
- Mesulam, M., Guillozet, A., Shaw, P., Levey, A., Duysen, E., & Lockridge, O. (2002). Acetylcholinesterase knockouts establish central cholinergic pathways and can use butyrylcholinesterase to hydrolyze acetylcholine. Neuroscience, 110, 627–639.10.1016/S0306-4522(01)00613-3
- Miao, Y., He, N., & Zhu, J. J. (2010). History and new developments of assays for cholinesterase activity and inhibition. Chemical Reviews, 110, 5216–5234.10.1021/cr900214c
- Moniruzzaman, M., Asaduzzaman, M., Hossain, M. S., Sarker, J., Rahman, S. M. A., Rashid, M., & Rahman, M. M. (2015). In vitro antioxidant and cholinesterase inhibitory activities of methanolic fruit extract of Phyllanthus acidus. BMC Complementary and Alternative Medicine, 15, 69.10.1186/s12906-015-0930-y
- Motaleb, M. A., Hossain, M. K., Alam, M. K., Abdullah-Al-Mamun, M. M., & Sultana, M. (2013). Commonly used medicinal herbs and shrubs by traditional herbal practitioners. Glimpses from Thanchiupazila of Bandarban (pp. 1–294). Dhaka: The International Union for Conservation of Nature.
- Neugroschl, J., & Sano, M. (2010). Current treatment and recent clinical research in Alzheimer’s disease. Mount Sinai Journal of Medicine: A Journal of Translational and Personalized Medicine, 77, 3–16.10.1002/msj.v77:1
- Nilsson, L., Nordberg, A., Hardy, J. A., Wester, P., & Winblad, B. (1986). Physostigmine restores 3H-acetylcholine efflux from Alzheimer brain slices to normal level. Journal of Neural Transmission, 67, 275–285.10.1007/BF01243353
- Nimmi, I., Jahan, I. A., Hossain, M. H., Uddin, M. B., Rana, M. S., & Haq, M. M. (2012). A comparative study on antioxidant properties of two phyllanthus species growing in Bangladesh. Dhaka University Journal of Pharmaceutical Science, 11, 191–197.
- Oyaizu, M. (1986). Studies on products of browning reaction. Antioxidative activities of products of browning reaction prepared from glucosamine. The Japanese Journal of Nutrition and Dietetics, 44, 307–315.10.5264/eiyogakuzashi.44.307
- Ramassamy, C. (2006). Emerging role of polyphenolic compounds in the treatment of neurodegenerative diseases: A review of their intracellular targets. European Journal of Pharmacology, 545, 51–64.10.1016/j.ejphar.2006.06.025
- Selkoe, D. J. (2001). Alzheimer’s disease: Genes, proteins, and therapy. Physiological Reviews, 81, 741–766.
- Singhal, A. K., Bangar, O. P., & Naithani, V. (2012). Medicinal plants with a potential to treat Alzheimer and associated symptoms. International journal of Nutrition, Pharmacology, Neurological Diseases, 2, 84–91.10.4103/2231-0738.95927
- Singleton, V. L., Orthofer, R., & Lamuela-Raventós, R. M. (1999). Analysis of total phenols and other oxidation substrates and antioxidants by means of Folin-Ciocalteu reagent. Methods in Enzymology, 299, 152–178.10.1016/S0076-6879(99)99017-1
- Small, S. A., & Mayeux, R. (2005). Alzheimer’s disease and related dementias. In Rowland, L. P. (Ed.), Merrits Neurology (Vol. 107, pp. 771–776). Philadelphia, PA: Lippincott Williums & Wilkins.
- Sultana, R., Poon, H. F., Cai, J., Pierce, W. M., Merchant, M., Klein, J. B., … Butterfield, D. A. (2006). Identification of nitrated proteins in Alzheimer’s disease brain using a redox proteomics approach. Neurobiology of Disease, 22, 76–87.10.1016/j.nbd.2005.10.004
- Tanzi, R. E., & Bertram, L. (2001). New frontiers in Alzheimer’s disease genetics. Neuron, 32, 181–184.10.1016/S0896-6273(01)00476-7
- Terry Jr., A. V., & Buccafusco, J. J. (2003). The cholinergic hypothesis of age and Alzheimer’s disease-related cognitive deficits: Recent challenges and their implications for novel drug development. Journal of Pharmacology and Experimental Therapeutics, 306, 821–827.10.1124/jpet.102.041616
- Uddin, S. N. (2006). Traditional uses of ethnomedicinal plants of the Chittagong hill tracts (p. 992). Dhaka: Bangladesh National Herbarium.
- Wischik, C. M., Harrington, C. R., & Storey, J. M. D. (2014). Tau-aggregation inhibitor therapy for Alzheimer’s disease. Biochemical Pharmacology, 88, 529–539.
- Zhishen, J., Mengcheng, T., & Jianming, W. (1999). The determination of flavonoid contents in mulberry and their scavenging effects on superoxide radicals. Food Chemistry, 64, 555–559.10.1016/S0308-8146(98)00102-2