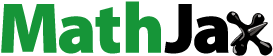
Abstract
The elevated use of inorganic fertilizers due to poor soil quality and the need for an increase in food production to meet the demand of the increasing global population has led to fertility decline in soil. Anaerobic digestion for the production of biogas and digestate is considered a sustainable alternative to non-renewable energy and inorganic fertilisers respectively. In this study, the potential use of anaerobic digestate as an alternative to dung as fertilizer was evaluated. The digestate and dung samples were screened for plant growth promoting bacteria (PGPB), the nutrient and heavy metal contents. The dung contained higher concentrations of heavy metals, phosphorus and potassium (p < 0.05). The identified genera from both samples include Acinetobacter, Bacillus, Pseudomonas, Escherichia, Staphylococcus and Micrococcus. The presence of higher amounts of mineralised nitrogen, reduced heavy metal content and PGPB in the digestate highlighted the potential ability of the digestate to enhance soil fertility.
Public Interest Statement
Conventional agriculture has been practiced to meet the demand of the rapidly growing population. The continuous use of chemical fertilisers has led to a gradual decline in soil fertility and environmental pollution. Anaerobic digestion results in the production of two valuable products which are biogas and a nutrient rich fertiliser also known as a digestate. In the present study, undigested dung and digestate was evaluated for their potential role to be used as a fertiliser. Both the digestate and dung were screened for the presence of microorganisms that can help the plant in nutrient uptake, nitrogen fixation and phosphate solubilisation. Moreover, heavy metals and nutrient content were also evaluated. The use of digestate in agriculture is currently considered inexpensive and can help to reduce cost and environmental implications caused by chemical fertilisers.
Competing interest
The authors declare no competing interest.
1. Introduction
Anaerobic digestion (AD) is an important environmentally friendly technology used to recycle waste. It can be described as a process in which microbial communities, in the absence of oxygen convert organic matter into energy. During AD, renewable energy in the form of a mixture of gases called biogas, is produced through a systematic process that involves four different stages i.e. hydrolysis, acidogenesis, acetogenesis and methanogenesis (Mata-Alvarez, Macé, & Llabrés, Citation2000).
Anaerobic digestion is a complex process that involves different types of microorganisms with various environmental requirements (Leung & Wang, Citation2016). According to Khalid, Arshad, Anjum, Mahmood, and Dawson (Citation2011), microorganisms do not perform the same function under different conditions and in different environments. It is therefore important to monitor the operating conditions of AD. The biogas yield, quality of the digestate and the growth of anaerobic microorganisms in the digester are influenced by many factors. These factors include pH, C/N ratio, microbial composition, nutrient content, temperature, hydraulic retention time, moisture content, design of the digester and feedstock concentration (Khalid et al., Citation2011).
The AD technology is one of the oldest in the world (Shanthi & Natarajan, Citation2016). However, many countries have lagged behind in terms of AD adoption due to lack of information about AD and all of the associated benefits (Agama Energy Pty, Citation2008). The use of digestate as a fertiliser is still not fully exploited. Currently, the interest in AD is increasing due to the high carbon dioxide emissions and other environmental impacts caused by the utilisation of fossil fuels. However, all the products and by-products of AD require more investigation to ensure economic viability of the process (Roopnarain & Adeleke, Citation2017). Besides biogas, which is used as a source of energy, the AD process also results in the production of another waste stream – digestate. This can be a serious limitation/challenge for biogas production technology. A potential solution to such challenge is to utilise the digestate as fertilizer. For this to be beneficial, there is need to ensure the nutrient and microbial composition of the digestate could benefit plants.
The digestate is the material that remains after AD and consists of different microbial communities (Alburquerque et al., Citation2012). In addition, both the organic and inorganic matter contained in the substrates may determine the quality of the digestate. The microbial communities digest most of the organic matter during the AD process and convert some to inorganic compounds. For example, the available nitrogen in the digester, whether from the substrate, atmosphere or from purging, is converted to ammonium and nitrates which remain in the digester until the end of the AD process. The anaerobes do not utilise the inorganic matter during AD. Of importance is the presence of inorganic components such as ammonium in the digestate which is beneficial if the digestate is intended for use as a fertiliser/ soil amendment because ammonium is directly available for plant uptake (Bonten et al., Citation2014).
The digestate from biogas production is rich in nitrogen, phosphorus and potassium (Islam, Rahman, Rahman, Oh, & Ra, Citation2010). The availability of these nutrients in the digestate are known to improve the soil structure (Nkoa, Citation2014). Inorganic fertilisers are known for causing a gradual decline in soil fertility and environmental pollution through nutrient leaching and heavy metal pollution (Zhu, Sun, Zhang, Zhang, & Qiao, Citation2012). Therefore, there is a need for alternative soil amendment sources. Due to the challenges associated with the use of inorganic fertilizers, the nutrient rich digestate from the AD process has become a viable alternative for soil amendment (Makádi, Tomócsik, & Orosz, Citation2012).
Although many studies have highlighted the presence of plant-available nutrients in the digestate (Bonten et al., Citation2014; Makádi et al., Citation2012; Nkoa, Citation2014), to our knowledge no study has been conducted on the availability of microbial communities within the digestate that possess the ability to enhance plant growth. These microbial communities are referred to as plant growth promoting bacteria (PGPB) (Khalid, Arshad, Shaharoona, & Mahmood, Citation2009). Certain PGPB have the ability to solubilise unavailable phosphate. Studies have shown that large portions of inorganic phosphates applied to the soil as chemical fertilisers are rapidly immobilised immediately after application and become unavailable to the plants, thus highlighting the importance of these PGPB (Bello-Akinosho et al., Citation2016; Rengel & Marschner, Citation2005). Other abilities of PGPB include the biological fixation of atmospheric nitrogen and the production of phytohormones such as indoleacetic acid (IAA) (Higa, Citation1994).
The use of by-products from AD especially the digestate is beneficial to the environment and ensures that the AD process is cost effective. The conversion of biogas to energy is more established in comparison to the use of the digestate as bio-fertiliser. Therefore, the current study focuses on determining the nutrient composition of the feedstock (dung) and digestate. Identification and characterization of the microbial communities for plant growth promoting abilities were also carried out.
2. Material and methods
2.1. Sample collection
Samples were collected from a working, pre-fabricated, fixed dome anaerobic digester situated in Qwa-Qwa, Free State province in South Africa (Latitude 28, 566,867°S, longitude 28, 678,145°E). The digester was fed every second day with 20 kg of fresh cow dung diluted in water at a ratio of 1:1 and the biogas produced was used for cooking purposes. The fresh cow dung and the digestate from the digester inlet and outlet respectively were collected in triplicate in sterile plastic bags. The samples were transported on ice to Agricultural Research Council (ARC)-Institute for Soil, Climate and Water (ISCW) microbiology laboratory. The samples were stored at 4°C until analysis.
2.2. Analytical methods
Samples were sent to the analytical laboratory of the Agricultural Research Council-Institute for Soil, Climate and Water (ARC-ISCW) for heavy metal and nutrient content (N, P and K) analyses. The dried and grounded samples (1 g) were digested in 21 ml HNO3 to determine heavy metal concentrations. The digested samples were analysed using inductively coupled plasma mass spectrophotometry (ICP-MS).
The pH was measured using a pH metre (Adwa, AD 1030). Dry matter content (DM) was determined using the following equation: DM (%) = 100 × WDM/Ws where WDM refers to weight after drying (105°C for 24 h) and Ws refers to wet weight of sample. Volatile solids (VS) were measured by calculating the weight of the ash (Wash) after combusting the oven dried samples at 550°C for 24 h (Cioabla, Popescu, Dumitrel, & Ionel, Citation2012). The following equation was used to calculate the volatile solids, VS (%) = 100 × (WDM − Wash)/WDM.
2.3. Bacterial isolation and characterisation
One gram of dung and digestate was transferred to sterile distilled water and serially diluted up to 10−8. For each dilution, 100 μL was spread on Nutrient Agar and the plates were incubated at 37°C for 24 h. Individual bacterial colonies were selected based on their distinct morphological characteristics and sub culturing was conducted until pure colonies were obtained. Pure isolates were preserved in glycerol (−80°C freezer).
2.4. Assessment of soil fertility attributes
2.4.1. Screening for phosphate solubilisation ability
The phosphate solubilisation ability of bacterial isolates were investigated using National Botanical Research Institute’s phosphate (NBRIP) growth medium (Adeleke, Cloete, & Khasa, Citation2012; Nautiyal, Citation1999). The medium contained in a litre; 10 g glucose, 5 g of insoluble Ca3(PO4)2 as a source of phosphate, 5 g MgCl2, 0.25 g MgSO4, 0.2 g KCl, 0.1 g (NH4)2SO4 and 15 g agar. The pH of the medium was adjusted to 7. 0. Ten microliters of a 48 h culture was transferred to wells created on the NBRIP medium. The halo zones and colony diameters were measured after 7 days of incubation at 37°C. Formation of visible halo zones around the microbial colonies on NBRIP media indicates phosphate solubilisation ability of the microorganisms. The ability of bacteria to solubilise insoluble phosphate was described by the solubilisation index (SI) (Equation 1) (Bhawalkar, Citation1996)(1)
(1)
2.4.2. Nitrogen fixation
The isolates were grown on nitrogen free (Burk’s) medium. The medium contained in a litre; 0.2 g MgSO4, 0.80 g K2HPO4, 0.2 g KH2PO4, 0.130 g CaSO4, 0.00145 g FeCl3, 0.000253 g Na2MoO4, 20 g Sucrose and 15 g Agar. Plates were incubated at 30°C for 7 days. The isolates that were able to grow after incubation indicated the potential to fix atmospheric nitrogen.
2.4.3. Indole acetic assay (IAA)
Indole acetic Assay production was determined as described by Bric, Bostock, and Silverstone (Citation1991) with some modifications. Isolates were cultured in LB broth supplemented with L-Tryptophan for 24 h. The cultures were then centrifuged at 10 000 RPM for 10 min at 4°C. One ml of the supernatant was mixed with 2 ml of Salkowski reagent and incubated in the dark for 30 min at room temperature. A colour change of the reagent from yellow to pink indicated indole production.
2.4.4. 16S rDNA gene amplification and sequencing
Pure isolates were subjected to colony PCR as described in Obi, Atagana, and Adeleke (Citation2016). The 16S rRNA was amplified using universal bacterial primers: 907R (5′-CCG TCA ATT CCT TTG AGTTT-3′) and 341F (3′-CCT ACG GGA GGC AGC AG-5′) (Muyzer, De Waal, & Uitterlinden, Citation1993). PCR was performed in a T100 TM thermal cycler (Bio-Rad laboratories) with the annealing temperature of 57°C. PCR products were run on a 1% agarose gel.
The PCR products were sent for Sanger sequencing at the Central Analytical Facilities (CAF) in Stellenbosch University. Sequences were inspected and edited using BioEdit Sequence Alignment Editor (http://www.mbio.ncsu.edu/bioedit/bioedit) and preliminary identification of isolates were conducted using National Centre for Biotechnology Information (NCBI) gene bank using the basic alignment search tool (BLAST) (http://www.ezbiocloud.net/eztaxon). The Mothur software was used to cluster sequences into operational taxonomic units (OTUs) at sequence similarity of 97% (Bello-Akinosho et al., Citation2016). A neighbour-joining phylogenetic tree was constructed using Jukes-Cantor method at 1000 bootstrap replications in MEGA 7.
2.5. Statistical analyses
Two-way ANOVA using XLSTAT version 2015 was used to compare the mean differences between the physico-chemical parameters measured. Student’s t-LSD (Least significant difference) was calculated at a 5% significance level. The correlation to determine the association between variables has been performed using SAS version 9.4 (Statistical Analysis System Institute, Citation1999).
3. Results
3.1. Physico-chemical parameters before and after AD
The physico-chemical characteristics of dung and digestate were compared (Table ). The dry matter, potassium, phosphorus and the volatile solids decreased in the digestate (p < 0.05). However, no significant variation was evidenced in the pH, nitrogen and carbon content of the dung and digestate (p > 0.05). Furthermore, the C/N ratio was higher in the dung as compared to the digestate.
Table 1. The physico-chemical parameters of the digester feedstock before and after anaerobic digestion
3.2. Heavy metal analysis
In South Africa, there is no legislation or guidelines for heavy metal content in digestate. Therefore, the fertiliser regulations from Department of Agriculture, Forestry and Fisheries (Citation2012) was used. The heavy metals in the dung and the digestate were below the threshold recommended by DAFF. The mean concentration of heavy metals before and after the AD process is shown in Figure . There was a significant decrease in the levels of Mn, Cu, Sr, Sn, and Ba after AD (p < 0.05).
3.3. Screening for phosphate solubilisation, IAA production and nitrogen fixation
Twenty-four bacterial isolates were obtained, 15 from the dung and 9 from the digestate. Selection of isolates was based on variations in morphological characteristics of bacterial colonies (Table A1 in Appendix). The isolates were screened for their ability to solubilise phosphate. More isolates from the feedstock (dung) were able to solubilise phosphate in comparison to isolates from the digestate (Figure ). From the nitrogen fixation and IAA production assays, similar trends were observed where more isolates from the dung were able to fix atmospheric nitrogen and produce IAA than the digestate isolates.
3.4. Community analysis for plant growth promotion
Sequences of the isolates were clustered into 7 OTUs (Table ). The phylogenetic tree of the representative OTUs was constructed using the Neighbor Joining method (Figure ). Four genera were obtained from the dung and three genera were obtained from the digestate (Table ). Shared genera from both samples included Acinetobacter and Bacillus.
Table 2. OTUs in relation to the phyla and sequence they contain
Figure 3. Phylogenetic tree showing obtained isolates and relatives.
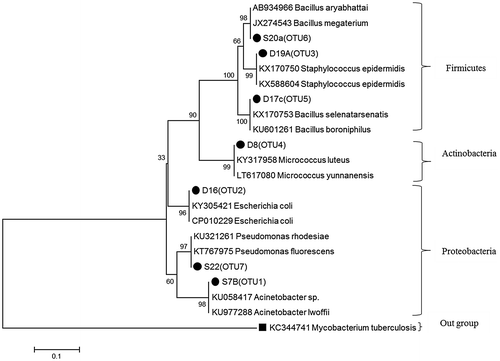
4. Discussion
Utilisation of the digestate material as a source of nutrients for plants is important as it contributes to the sustainability of the anaerobic digestion process. However, the digestate may contain pathogens and heavy metals that might have negative effects on humans and crop yield during application. This study was aimed at evaluating the suitability of the digestate as an organic soil amendment in comparison to the untreated substrate currently being used as feedstock for AD i.e. dung.
A decrease in TS, VS, potassium and phosphorus content was evidenced in the digestate in comparison to the dung samples. Elevated dry matter and volatile solids in the dung in comparison to the digestate was expected because anaerobic bacteria utilize organic matter during the AD process for biogas production (Möller & Müller, Citation2012). The same applies to macro and micro nutrients. However, in the present study, the NH4+content increased after AD. This increase may be attributed to the conversion of organic nitrogen to ammonium during the course of AD. This is advantageous if the digestate is intended for use as a fertilizer because N mineralisation ensures that Nitrogen is available in a plant-accessible form. Similar results have been obtained Gómez-Brandón, Juárez, Zangerle, and Insam (Citation2016) who reported higher ammonium content in digestate than manure. Elevated ammonium levels in the digestate were also attributed to mineralisation of N compounds in the substrate (Gómez-Brandón et al., Citation2016).
The AD process is carried out by microbial communities that are sensitive to pH fluctuations. According to Chen, Romano, and Zhang (Citation2010), anaerobic bacteria prefer a pH range between 6.5 and 7.5 for optimal biogas production. The pH before and after AD process did not vary (p > 0.05) as shown in Table . As already established in previous studies (Alburquerque, de la Fuente, & Bernal, Citation2012; Astals, Nolla-Ardèvol, & Mata-Alvarez, Citation2012; Lukehurst, Frost, & Al Seadi, Citation2010), the type of substrate used in biogas production influences the nutrient composition and quality of the digestate. The substrate used in this study had the necessary macronutrients required by anaerobic bacteria. In their study, Singh, Lee, Worley, Risse, and Das (Citation2010) reported a lower C/N ratio in the digestate compared to untreated dung, which is similar to the results of the present study. A decrease in the C/N ratio after AD was expected due to the breakdown of organic matter and accumulation of inorganic N sources. However, the C/N ratio of the feedstock (cow dung) in the present study was higher in comparison to that reported by Kataki, Hazarika, and Baruah (Citation2017). Elevated feedstock C/N ratio may be detrimental due to the risk of nitrogen limitation for microbial growth whereas a low C/N ratio may also impede biogas production due to ammonia inhibition (Igoni, Ayotamuno, Eze, Ogaji, & Probert,Citation2008; Rajagopal, Massé, & Singh, Citation2013). The elevated C/N ratio of the substrate in the present study might be attributed to the environmental conditions where the cattle were based, type of cattle feed and if the dung collected was from dairy or beef cattle (Leung & Wang, Citation2016).
The AD process also resulted in a reduction in the heavy metal content, which is beneficial to the environment if the digestate is intended for use as a fertiliser. Physical, chemical and microbial processes during AD can affect the heavy metal concentration (Xie, Ma, Strong, & Clarke, Citation2015). Numerous heavy metals such as manganese, copper, nickel, zinc, chromium and cadmium are toxic to the environment when present in high concentrations (Meena, Kadirvelu, Mishraa, Rajagopal, & Nagar, Citation2008). Heavy metal pollution poses significant environmental threats to humans, fish and invertebrates. The bio-magnification of heavy metals along the food chain further exacerbates the associated risks (Yi, Yang, & Zhang, Citation2011). Furthermore, heavy metal accumulation has the ability to damage plants as it might affect the growth and metabolism of plant cells (Madu, Akpaiyo, & Ikoku, Citation2011).
More isolates were obtained from the feedstock (dung) in comparison to the digestate. This may be attributed to the environmental pressures imposed by the AD process. Some microorganisms in the feedstock (dung) were unable to survive due to unfavourable AD conditions. The identification of the isolates showed that the dung had a greater culturable microbial diversity in comparison to the digestate. However, genera such as Escherichia and Staphylococcus were obtained from the dung. Some of the species that fall under these genera are known to be pathogenic. As reviewed by Carbone, Da Silva, Tavares, and Dias Filho (Citation2002), the faeces of animals contain E.coli as a pathogen that is naturally found in the intestinal tract and shed with faeces. Therefore, the use of untreated manure poses higher risk of containing pathogens as compared to the digestate (Adesemoye & Kloepper, Citation2009).
The isolates were further evaluated for the ability to enhance soil fertility. All isolates from the dung and the digestate had one or more soil enhancing attributes. However, the abilities to solubilise phosphate and IAA production were elevated in most of the isolates in comparison to the ability to fix atmospheric nitrogen. As mentioned before, the quality of the digestate depends on the type of substrate used (Mata-Alvarez et al., Citation2000). In this study, shared genera were obtained from both the dung and the digestate. However, Acinetobacter was found to be the most prominent in both the dung and digestate. A study carried out by Gulati et al. (Citation2010) showed that Acinetobacter is an efficient phosphate solubiliser. The availability of PGPB in the digestate is advantageous because these organisms enhance plant growth in an environmentally friendly way in comparison to chemical fertilisers. A long term study comparing barley yield when using digestate and compost conducted by Odlare et al. (Citation2011) found the highest barley yield using digestate as compared to compost thus AD digestate may be used as a valuable fertiliser in a long term. Therefore, apart from making certain nutrients available for the plant, the presence of PGPB in digestate will also assist in plant nutrient uptake.
In conclusion, both the dung and digestate contained culturable microbial communities with one or more soil fertility enhancing attributes. The AD process resulted in the reduction of heavy metal content and some of the potential pathogenic bacteria from cow dung. In addition, the dung contained pathogenic microorganisms that might damage plants and cause diseases in human. The AD process also results in the mineralisation of organic N enabling the presence of plant-available N in the digestate. Based on our results, digestate seems to have more potential than cow dung as fertiliser for soil amendment. Therefore, digestate from cow dung can potentially be used as a fertiliser. However, more studies need to be conducted on different substrates to ascertain if the resulting digestate can be used as a fertiliser. Furthermore, metagenomics studies can be conducted to determine the presence of non-cultivable plant growth promoting bacteria and pathogens before and after AD.
Funding
This work was supported by the National Research Foundation [grant number 96735] and the South Africa Norway, Research Co-operation [grant number RCN 234203].
Additional information
Notes on contributors
Mashudu Mukhuba
Mashudu Mukhuba is a Masters student in Environmental Science at the University of South Africa (UNISA). She is also a research student at ARC-Soil, Climate and Water (ARC-SCW). Her research interests are biotechnology and renewable energy (Biogas).
Ashira Roopnarain
Ashira Roopnarain is a postdoctoral research fellow at the ARC-SCW. She obtained her PhD from University of Witwatersrand. Her research interests include biotechnology and bioenergy (biogas and biofertilizer production from organic waste).
Rasheed Adeleke
Rasheed Adeleke is a Professor, Senior Researcher and Research Group leader. He obtained his PhD degree from the University of Pretoria. His current research interests include microbial ecology, bioremediation, soil biotechnology and plant-microbe interactions.
Mokhele Moeletsi
Mokhele Moeletsi holds PhD in Agrometeorology. He is a senior researcher at ARC-SCW. His research interests include agriculture, climate change and vulnerability.
Rosina Makofane
Rosina Makofane is a Masters student at UNISA. She is also a research student at ARC-SCW. Her research interests are biotechnology and renewable energy (Biogas).
References
- Adeleke, R., Cloete, T. E., & Khasa, D. P. (2012). Culturable microorganisms associated with Sishen iron ore and their potential roles in biobeneficiation. World Journal of Microbiology and Biotechnology, 28(3), 1057–1070.10.1007/s11274-011-0904-2
- Adesemoye, A. O., & Kloepper, J. W. (2009). Plant–microbes interactions in enhanced fertilizer-use efficiency. Applied Microbiology and Biotechnology, 85(1), 1–12.10.1007/s00253-009-2196-0
- Agama Energy Pty. (2008). Biofuels in the City of Cape Town: Energy from waste. Prepared for UNDP/CCT/SI.
- Alburquerque, J. A., de la Fuente, C., & Bernal, M. P. (2012). Chemical properties of anaerobic digestates affecting C and N dynamics in amended soils. Agriculture, Ecosystems & Environment, 160, 15–22.10.1016/j.agee.2011.03.007
- Alburquerque, J. A., de la Fuente, C., Ferrer-Costa, A., Carrasco, L., Cegarra, J., Abad, M., & Bernal, M. P. (2012). Assessment of the fertiliser potential of digestates from farm and agroindustrial residues. Biomass and Bioenergy, 40, 181–189.10.1016/j.biombioe.2012.02.018
- Astals, S., Nolla-Ardèvol, V., & Mata-Alvarez, J. (2012). Anaerobic co-digestion of pig manure and crude glycerol at mesophilic conditions: Biogas and digestate. Bioresource Technology, 110, 63–70.10.1016/j.biortech.2012.01.080
- Bello-Akinosho, M., Makofane, R., Adeleke, R., Thantsha, M., Pillay, M., & Chirima, G. J. (2016). Potential of polycyclic aromatic hydrocarbon-degrading bacterial isolates to contribute to soil fertility. BioMed Research International, 2016.
- Bhawalkar, U. S. (1996). Vermiculture ecotechnology. Pune: Bhawalkar Earthworm Research Institute.
- Bonten, L. T. C., Zwart, K. B., Rietra, R. P. J. J., Postma, R., De Haas, M. J. G., & Nysingh, S. L. (2014). Bio-slurry as fertilizer: Is bio-slurry from household digesters a better fertilizer than manure?: A literature review (No. 2519). Wageningen: Alterra Wageningen UR.
- Bric, J. M., Bostock, R. M., & Silverstone, S. E. (1991). Rapid in situ assay for indoleacetic acid production by bacteria immobilized on a nitrocellulose membrane. Applied and Environmental Microbiology, 57(2), 535–538.
- Carbone, S. R., Da Silva, F. M., Tavares, C. R. G., & Dias Filho, B. P. (2002). Bacterial population of a two-phase anaerobic digestion process treating effluent of cassava starch factory. Environmental Technology, 23(5), 591–597.
- Chen, X., Romano, R. T., & Zhang, R. (2010). Anaerobic digestion of food wastes for biogas production. International Journal of Agricultural and Biological Engineering, 3(4), 61–72.
- Cioabla, A. E., Popescu, F., Dumitrel, G. A., & Ionel, I. (2012). Comparative study on factors affecting anaerobic digestion of agricultural vegetal residues. Biotechnology for Biofuels, 5(1), 39.10.1186/1754-6834-5-39
- Department of Agriculture, Forestry and Fisheries. (2012). Fertilisers, farm feeds, agricultural remedies and stock remedies ACT No.36 of 1947. Pretoria: Author.
- Gómez-Brandón, M., Juárez, M. F. D., Zangerle, M., & Insam, H. (2016). Effects of digestate on soil chemical and microbiological properties: A comparative study with compost and vermicompost. Journal of Hazardous Materials, 302, 267–274.10.1016/j.jhazmat.2015.09.067
- Gulati, A., Sharma, N., Vyas, P., Sood, S., Rahi, P., Pathania, V., & Prasad, R. (2010). Organic acid production and plant growth promotion as a function of phosphate solubilization by Acinetobacter rhizosphaerae strain BIHB 723 isolated from the cold deserts of the trans-Himalayas. Archives of Microbiology, 192(11), 975–983.10.1007/s00203-010-0615-3
- Higa, T. (1994). Effective microorganisms: A new dimension for nature farming. In Proceedings of the 2nd International Conference on Kyusei Nature Farming (pp. 20–22). Washington, DC: USDA.
- Igoni, A. H., Ayotamuno, M. J., Eze, C. L., Ogaji, S. O. T., & Probert, S. D. (2008). Designs of anaerobic digesters for producing biogas from municipal solid-waste. Applied Energy, 85(6), 430–438.10.1016/j.apenergy.2007.07.013
- Islam, M. R., Rahman, S. M. E., Rahman, M. M., Oh, D. H., & Ra, C. S. (2010). The effects of biogas slurry on the production and quality of maize fodder. Turkish Journal of Agriculture and Forestry, 34(1), 91–99.
- Singh, K., Lee, K., Worley, J., Risse, L. M., & Das, K. C. (2010). Anaerobic digestion of poultry litter: A review. Applied Engineering in Agriculture, 26(4), 677–688.10.13031/2013.32061
- Kataki, S., Hazarika, S., & Baruah, D. C. (2017). Assessment of by-products of bioenergy systems (anaerobic digestion and gasification) as potential crop nutrient. Waste Management, 59, 102–117.
- Khalid, A., Arshad, M., Anjum, M., Mahmood, T., & Dawson, L. (2011). The anaerobic digestion of solid organic waste. Waste Management, 31(8), 1737–1744.10.1016/j.wasman.2011.03.021
- Khalid, A., Arshad, M., Shaharoona, B., & Mahmood, T. (2009). Plant growth promoting rhizobacteria and sustainable agriculture. In Microbial strategies for crop improvement (pp. 133–160). Berlin: Springer.10.1007/978-3-642-01979-1
- Leung, D. Y., & Wang, J. (2016). An overview on biogas generation from anaerobic digestion of food waste. International Journal of Green Energy, 13(2), 119–131.10.1080/15435075.2014.909355
- Lukehurst, C. T., Frost, P., & Al Seadi, T. (2010). Utilisation of digestate from biogas plants as biofertiliser. IEA Bioenergy, 1–36.
- Madu, P. C., Akpaiyo, G. D., & Ikoku, P. (2011). Biosorption of Cr 3+, Pb 2+, and Cd 2+ ions from aqueous solution using modified and unmodified millet chaff. Journal of Chemical and Pharmaceutical Research, 3, 467–477.
- Makádi, M., Tomócsik, A., & Orosz, V. (2012). Digestate: A new nutrient source-review. In Biogas. InTech.
- Mata-Alvarez, J., Macé, S., & Llabrés, P. (2000). Anaerobic digestion of organic solid wastes. An overview of research achievements and perspectives. Bioresource Technology, 74(1), 3–16.10.1016/S0960-8524(00)00023-7
- Meena, A. K., Kadirvelu, K., Mishraa, G. K., Rajagopal, C., & Nagar, P. N. (2008). Adsorption of Pb(II) and Cd(II) metal ions from aqueous solutions by mustard husk. Journal of Hazardous Materials, 150(3), 619–625.10.1016/j.jhazmat.2007.05.011
- Möller, K., & Müller, T. (2012). Effects of anaerobic digestion on digestate nutrient availability and crop growth: A review. Engineering in Life Sciences, 12(3), 242–257.10.1002/elsc.201100085
- Muyzer, G., De Waal, E. C., & Uitterlinden, A. G. (1993). Profiling of complex microbial populations by denaturing gradient gel electrophoresis analysis of polymerase chain reaction-amplified genes coding for 16S rRNA. Applied and Environmental Microbiology, 59(3), 695–700.
- Nautiyal, C. S. (1999). An efficient microbiological growth medium for screening phosphate solubilizing microorganisms. FEMS Microbiology Letters, 170(1), 265–270.10.1111/fml.1999.170.issue-1
- Nkoa, R. (2014). Agricultural benefits and environmental risks of soil fertilization with anaerobic digestates: A review. Agronomy for Sustainable Development, 34(2), 473–492.10.1007/s13593-013-0196-z
- Odlare, M., Arthurson, V., Pell, M., Svensson, K., Nehrenheim, E., & Abubaker, J. (2011). Land application of organic waste–effects on the soil ecosystem. Applied Energy, 88(6), 2210–2218.
- Obi, L. U., Atagana, H. I., & Adeleke, R. A. (2016). Isolation and characterisation of crude oil sludge degrading bacteria. SpringerPlus, 5(1), 5.10.1186/s40064-016-3617-z
- Rajagopal, R., Massé, D. I., & Singh, G. (2013). A critical review on inhibition of anaerobic digestion process by excess ammonia. Bioresource Technology, 143, 632–641.10.1016/j.biortech.2013.06.030
- Rengel, Z., & Marschner, P. (2005). Nutrient availability and management in the rhizosphere: Exploiting genotypic differences. New Phytologist, 168(2), 305–312.10.1111/j.1469-8137.2005.01558.x
- Roopnarain, A., & Adeleke, R. (2017). Current status, hurdles and future prospects of biogas digestion technology in Africa. Renewable and Sustainable Energy Reviews, 67, 1162–1179.10.1016/j.rser.2016.09.087
- Shanthi, P., & Natarajan, M. (2016). Anaerobic digestion of municipal solid biodegradable wastes for methane production: A review. International Journal for Research in Applied Science and Engineering Technology, 4(12), 208–215.
- Statistical Analysis System Institute. (1999). SAS/STAT user’s guide, version 8 (Vol. 2). SAS Institute.
- Xie, S., Ma, Y., Strong, P. J., & Clarke, W. P. (2015). Fluctuation of dissolved heavy metal concentrations in the leachate from anaerobic digestion of municipal solid waste in commercial scale landfill bioreactors: The effect of pH and associated mechanisms. Journal of Hazardous Materials, 299, 577–583.10.1016/j.jhazmat.2015.07.065
- Yi, Y., Yang, Z., & Zhang, S. (2011). Ecological risk assessment of heavy metals in sediment and human health risk assessment of heavy metals in fishes in the middle and lower reaches of the Yangtze River basin. Environmental Pollution, 159(10), 2575–2585.10.1016/j.envpol.2011.06.011
- Zhu, H. J., Sun, L. F., Zhang, Y. F., Zhang, X. L., & Qiao, J. J. (2012). Conversion of spent mushroom substrate to biofertilizer using a stress-tolerant phosphate-solubilizing Pichia farinose FL7. Bioresource Technology, 111, 410–416.10.1016/j.biortech.2012.02.042
Appendix
Table A1. Morphological characterisation of bacterial isolates