Abstract
To protect crops from pests, pesticides are used. Pesticides also cause toxicity to crop plants and persist in plant parts in the form of pesticide residues. Brassinosteroids (BRs) are known for their protective role in plants under various abiotic stresses like heavy metal, drought, temperature, pesticide etc. BRs ameliorate pesticide toxicity in intact plants by activating the antioxidative defence system. BRs also enhance the degradation of pesticides that leads to reduction in pesticide residues in plant parts. Present review gives an updated information about the protective roles of BRs in plants and the underlying mechanisms under pesticide stress.
Public Interest Statement
Pesticides are used all over the world to protect crops from pests. However pesticides also cause toxicity to plants and accumulate in plant parts in form of pesticide residues. Brassinosteroids are plant hormones which help in protection of plants from pesticide toxicity and reducing the harmful pesticide residues. Present review describes how exogenous application of brassinosteroids reduces pesticide toxicity and residues in plants.
Competing interest
The authors declare no competing interest.
1. Introduction
Plants are attacked by various pests, resulting in reduction of yield as well as the quality of crops, and to check these pests, various pesticides are utilized (Goh, Yiu, Wong, & Rajan, Citation2011). However, the extensive use of pesticides may cause toxicity to plants which may impair the plant metabolism and may persist in plant parts in the form of pesticide residues (Table , Sharma, Bhardwaj, Kumar, & Thukral, Citation2016; Xia et al., Citation2009; Zhou et al., Citation2015). Plants detoxify pesticides by enzyme mediated three phased detoxification system (Cherian & Oliveira, Citation2005; Coleman, Blake-Kalff, & Davies, Citation1997). First of all the activation of pesticides is catalysed by enzymes P450 monooxygenase, peroxidase and carboxylesterase. After this, conjugation of activated pesticides to glutathione and glucose is catalysed by glutathione-S-transferase and UDP-glycosyltransferase respectively. Finally less toxic pesticide metabolites are stored in the vacuoles/apoplast.
Table 1. Persistence of pesticides in various plant parts
Brassinosteroids (BRs) are plant polyhydroxysteroids which were discovered by Grove et al. (Citation1979). BRs are distributed throughout the plant kingdom and are present in small concentrations in young plant parts including the pollens (Clouse & Sasse, Citation1998; Gupta, Bhardwaj, Nagar, & Kaur, Citation2004; Kanwar, Poonam, & Bhardwaj, Citation2015). Approximately sixty types of BRs have been identified (Haubrick & Assmann, Citation2006), out of which 24-epibrassinolide (EBR, C28H48O6, Figure ) and 28-homobrassinolide (HBR, C29H50O6, Figure ) have been widely used for physiological studies (Vardhini & Anjum, Citation2015). BRs are reported to play an important role for the recovery of plants under abiotic stress conditions like salts, heavy metals and pesticides by activating the antioxidative defence system of the plants (Bajguz, Citation2009; Krishna, Citation2003; Shahzad et al., Citation2018; Sharma, Bhardwaj, & Pati, Citation2015; Sharma, Kumar, Thukral, & Bhardwaj, Citation2016). Additionally, exogenous application of BRs has also been observed to enhance the activities of enzymes involved in three phased pesticide detoxification system mentioned above (Xia et al., Citation2009; Zhou et al., Citation2015). BRs also modulate the expression of genes involved in pigment and secondary metabolite biosynthesis under pesticide stress (Sharma, Thakur, et al., Citation2016). Keeping in mind about the role of BRs in pesticide stress management, the present review explains BR-regulated pesticide detoxification in plants.
2. Climatic/environmental factors and BRs
The main factor which is an integral part of climate change is the CO2 concentration in the environment. It is believed that CO2 interacts with BRs, resulting in the regulation of plant growth. Interaction between CO2 and BRs increase the plant growth, sugar and starch contents, and regulates various the activities of enzymes involved in various photosynthetic and Benson-Calvin cycle by regulating the genes encoding them in cucumber. These genes encodes various enzymes include ribulose-1,5-bisphosphate carboxylase/oxygenase, ribulose-1,5-bisphosphate carboxylase/oxygenase activase, sedoheptulose-1,7-bisphosphatase), ribulose-5-
phosphate kinase, triose-3-phosphate isomerase, glycerate-3-phosphate kinase, fructose-1,6-bisphosphatase (Jiang et al., Citation2012). Environmental factors like light and temperature are involved in the regulation of gene expression in tobacco plants after interacting with BRs. There exist a direct interaction between BR-activated transcript factor (BZR1), and dark and heat transcription factor i.e. phytochrome interacting factor 4 (PIF4). This interaction after perceiving environmental signals is responsible for the regulation of various genes involved in modulation of plant metabolism to regulate these climatic factors (Oh, Zhu, & Wang, Citation2012). It has been noticed that BRs produced under Ni heavy metal stress, further enhanced the resistance of mustard plants. They regulated the antioxidative defense system of plants to counterattack the toxicity of heavy metals (Kanwar et al., Citation2012). Arsenic toxicity has also been reported to regulate the biosynthesis of BRs in mustard plants (Kanwar et al., Citation2015). Under other climatic conditions like drought, BRs interact with other plant growth regulators (PGRs) to regulate biochemical processes of plants (Yuan et al., Citation2010). Moreover, crosstalk of BRs with other PGRs like auxins, abscisic acid, cytokinins, ethylene, gibberellins, salicylic acid etc. is also responsible for BR-mediated regulation of various plant metabolic processes (Choudhary, Yu, Yamaguchi-Shinozaki, Shinozaki, & Tran, Citation2012).
Pesticides are absorbed by plants through roots or leaf surface. There are many environmental factors like temperature, precipitation and physiochemical characteristics of soil, which affect the uptake of pesticides and their metabolism in plants (Finlayson & MacCarthy, Citation1973). These absorbed pesticides may get metabolized by the internal detoxification system of plants or they get accumulated in various plant parts resulting in pesticide bio-magnification (Mwevura, Citation2000). Since BRs are known to protect plants from various environmental factors like temperature, drought, soil-salt toxicity, soil-metal toxicity (El-Mashad & Mohamed, Citation2012; Farooq, Wahid, & Basra, Citation2009; Kanwar et al., Citation2012; Khan, Fariduddin, & Yusuf, Citation2015), it may be attributed that BRs can provide resistance to plants under pesticide stress accompanied by various other environmental factors.
3. Pesticide toxicity in plants
Pesticides cause toxicity to plants by means of chlorosis, necrosis and vein discolouration, leading to retarded growth and development by reducing photosynthetic efficiency, nitrogen and carbon metabolism (Kaňa et al., Citation2004). Pesticide application was also reported to inhibit photosynthesis by negatively affecting the plant photosystems (Xia et al., Citation2006). In rice seedlings, imidacloprid and chlorpyrifos were reported to reduce the root length, shoot length, fresh weight and protein content. These pesticides were also reported to degrade the chlorophyll pigment (Sharma, Bhardwaj, & Pati, Citation2012, Citation2013). Sharma et al. (Citation2015) reported that imidacloprid and chlorpyrifos cause oxidative stress to rice seedlings by generating reactive oxygen species (ROS) like superoxide anions and hydrogen peroxide (H2O2). In tomato plants, Zhou et al. (Citation2015) also reported the enhanced levels of H2O2 after the application of chlorothalonil pesticide. Moreover, NADPH oxidases are involved in the production of reactive oxygen species (Kaur, Sharma, Guruprasad, & Pati, Citation2014). Additionally, RBOH1 (respiratory burst oxidase homologue 1) has been reported to regulate the levels of H2O2 in tomato plants under pesticide stress (Zhou et al., Citation2015). In cucumber plants, phytotoxic effects of various pesticides were studied by Xia et al. (Citation2006). They observed that application of pesticides negatively affects the photosynthetic machinery of cucumber plants, resulting in reduced photosynthetic rate (Pn), stomatal conductance (Gs) and intercellular CO2 (Ci). These researchers also reported the inhibitory effects of pesticides on quantum efficiency (Fv/Fm) as well as quantum efficiency (Φ) of PS II. Siddiqui and Ahmed (Citation2006) studied the effect of six different pesticides (topsin-M, demacron, benlate, cypermethrin, dimethride and chlorosulfuron) on soybean plants. They found that at higher concentrations (0.5 and 0.75 g L−1), these pesticides cause declined relative growth rate and crop growth rate. Reduced total phenol and ascorbic acid contents in potatoes were observed after the application of imidacloprid pesticide (Chauhan, Agrawal, & Srivastava, Citation2013). Application of pesticides (mancozeb, flusilazol, dithianon, pirimicarb) on apple tree was reported to inhibit the photosynthesis (Untiedt & Blanke, Citation2004). In mustard plants reduction in photosynthesis was noticed by Sharma, Kumar, Singh, Thukral and Bhardwaj (Citation2016) after imidacloprid insecticide application. In Saccharina japonica, application of diuron pesticide resulted in retarded growth, decreased carotenoid and chlorophyll content, optimal quantum yield and maximum electron transport rate (Kumar, Choo, Yea, Seo, & Han, Citation2010). Studies carried out by Sharma, Kumar, Kohli, Thukral, and Bhardwaj (Citation2015a), Sharma, Kumar, Singh, Thukral, and Bhardwaj (Citation2015b) reported the decreased levels of various phytochemicals after the application of imidacloprid pesticide in Brassica juncea plants. In response to pesticide toxicity, plants have the mechanism to detoxify pesticides, discussed already in introduction. Additionally, recent studies carried out by Huang, Lu, Zhang, Luo, and Yang (Citation2016) demonstrated that laccase encoding genes in rice were involved in the degradation of atrazine and isoproturon. They also reported that laccase activity was increased in pesticide treated plants, in comparison to control plants, which confirmed the possible role of laccases in pesticide detoxification. Lu et al. (Citation2016) reported the role of DNA methylation in the detoxification of atrazine in rice by regulating the genes like CYP70IA8.
4. Physiological and abiotic stress protecting roles of BRs
Important roles played by BRs in physiology of plants include process of cell elongation and differentiation, development of pollen tube, differentiation of vascular bundles, reassembling of nucleic acids resulting in protein formation, activation of antioxidative defense system of plants and regulation of photosynthesis (Sasse, Citation2003; Sharma et al., Citation2015; Xia et al., Citation2006; Yu et al., Citation2004). In dicots, BRs are reported to enhance the elongation of epicotyles, hypocotyls and peduncles, whereas in monocots, they increase the elongation of coleoptiles and mesocotyls (Clouse, Citation1996; Mandava, Citation1988).
BRs like EBR and HBR have been observed to play an important role in promotion of seed germination (Sasse, Smith, & Hudson, Citation1995; Sharma & Bhardwaj, Citation2007). The percentage of germination was observed to increase in Cicer arietinum and Triticum aestivum after seed application of HBR (Ali, Hayat, & Ahmad, Citation2005; Hayat & Ahmad, Citation2003). Increase in yield, carbonic anhydrase activity and net photosynthetic rate was observed when HBR was applied exogenously to B. juncea plants (Hayat, Ahmad, Mobin, Hussain, & Fariduddin, Citation2000). The net photosynthetic rate was reported to be enhanced by the application of BRs in various plant species including B. juncea, C. sativus, G. max, O. sativa and V. radiata (Fariduddin, Ahmad, & Hayat, Citation2003; Farooq et al., Citation2009; Hayat, Ali, Aiman Hasan, & Ahmad, Citation2007; Sharma, Kumar, Singh, Thukral, Bhardwaj, Citation2016; Xia et al., Citation2006; Zhang, Zhai, Tian, Duan, & Li, Citation2008).
BRs also play a role in stimulation of flowering in Arabidopsis thaliana (Domagalska, Sarnowska, Nagy, & Davis, Citation2010). Deluc et al. (Citation2007) found significant role of brassinosteroids in fruit ripening. Pilati et al. (Citation2007) concluded that accumulation of BRs during the process of fruit development can lead to the ripening of fleshy fruits. Many researchers have also reported the enhanced ripening of cucumber, grapes, rice, tomato and yellow passion fruit after the application of BRs (Fu et al., Citation2008; Fujii & Saka, Citation2001; Gomes et al., Citation2006; Symons et al., Citation2006; Vardhini & Rao, Citation2002).
BRs are also reported to affect the expression of other genes that plays important role in plant defense as well as biosynthesis of other plant growth regulators (Bari & Jones, Citation2009). Several studies have documented their important role in protecting plants from adverse environmental stress conditions like drought, heavy metals, pesticides, salinity and viruses (Krishna, Citation2003; Kanwar et al., Citation2012, Citation2013; Özdemir, Bor, Demiral, & Türkan, Citation2004; Sharma, Thakur, et al., Citation2016; Wachsman, López, Ramirez, Galagovsky, & Coto, Citation2000). Recent studies carried out by Derevyanchuk, Litvinovskaya, Khripach, Martinec, and Kravets (Citation2015) have demonstrated the role of EBR in modulation of respiration in A. thaliana under salt stress. BRs help in amelioration of the toxic effects of various abiotic stress conditions in plants by activating the antioxidative defense system (Vardhini & Anjum, Citation2015). Moreover, physiological roles of BRs in plants have also been extensively reviewed by Clouse (Citation2015). Figure shows various abiotic stresses which are regulated by BRs.
5. Amelioration of pesticide toxicity by BRs in plant systems
As a consequence of pesticide toxicity, plant growth and development are negatively affected due to generation of reactive oxygen species. However, in response to this pesticide stress, plants internal defence system (antioxidative defence system) gets activated to cope up with pesticide toxicity. Moreover, BR application further triggers this antioxidative defence system of plant, resulting in enhancing resistance of plants to pesticide toxicity (Figure ). In cucumber plants, exogenous application of 24-EBL resulted in increased photosynthetic rate and stomatal conductance, which were earlier negatively affected by pesticide application (Xia et al., Citation2006). They reported that 0.48 g L−1 chlorpyrifos application decreased photosynthetic rate and stomatal conductance by 81.01 and 71.97% respectively when compared to control plants. However the application of 24-EBL enhanced photosynthetic rate and stomatal conductance by 395 and 277% respectively when compared to chlorpyrifos treated plants. These researchers also observed that application of 24-EBL significantly increased the quantum efficiency of PSII and phytochemical quenching co-efficient. Sharma, Kumar, Singh, Thukral, and Bhardwaj (Citation2016) also observed the recovery of growth and photosynthetic parameters in B. juncea plants raised from 24-EBL treated seeds and grown under imidacloprid toxicity. Antioxidative defence system of plants gets activated under pesticide stress (Sharma et al., Citation2012, Citation2013, Citation2015; Xia et al., Citation2009; Zhou et al., Citation2015). 24-EBL and 28-HBL were reported to enhance the activities of antioxidative enzymes like superoxide dismutase (SOD), catalase (CAT), ascorbate peroxidase (APX), glutathione peroxidase (GPOX), glutathione reductase (GR), dehydroascorbate reductase (DHAR), monodehydroascorbate reductase (MDHAR), and contents of protein, proline, under chlorpyrifos (CPF) and imidacloprid (IMI) pesticide stress in rice seedlings (Sharma et al., Citation2012, Citation2013, Citation2015). They also noticed the stimulatory effect of 24-EBL and 28-HBL on overall growth of rice seedlings under CPF and IMI toxicity. Expression and activities of enzymes involved in enzyme mediated pesticide detoxification system were reported to enhance after the application of BRs (Xia et al., Citation2009; Zhou et al., Citation2015). Seed treatment of 24-EBL (before sowing) has been also reported to significantly enhance the levels of various phytochemicals which were earlier decreased by the application of IMI pesticide in Brassica juncea plants (Sharma et al., Citation2015a, Citation2015b). Contents of non-enzymatic antioxidants like polyphenols, ascorbic acid, tocopherol and glutathione were also observed to enhance in Brassica juncea plants raised from seeds soaked in 100 nM 24-EBL before sowing in IMI (250, 300 and 350 mg IMI Kg−1 soil) supplemented soils (Sharma, Kumar, Thukral, Bhardwaj, Citation2016b). Recent studies carried out by Sharma, Thakur, et al. (Citation2016), Sharma, Kumar, Kanwar, Thukral, and Bhardwaj (Citation2017) has reported that exogenous applied EBR enhanced the contents of organic acids (citric, fumaric, malic, and succinic acid) by modulating the expression of genes involved in their metabolism (CS-citrate synthse, FH-fumarate hydratase, SUCLG1-succinyl Co-A ligase, SDH-succinate dehydrogenase, and MS-malate synthase) in B. juncea seedlings under pesticide toxicity. Moreover, expression of PAL (phenylalanine ammonialyase) was also observed to be regulated by the application of EBR under pesticide stress. BRs are also known to recover the elemental composition of Indian mustard plants under IMI toxicity (Sharma, Kumar, Kanwar, Thukral, & Bhardwaj, Citation2016). Recently, recovery in amino acid and protein content was reported by Sharma, Kumar, Thukral, and Bhardwaj (Citation2017) in the green leaves of Indian mustard plants which were germinated from seeds treated with 24-EBL and grown in soil containing IMI.
Figure 3. Overview and mechanism of plant responses to pesticide stress and BRs.
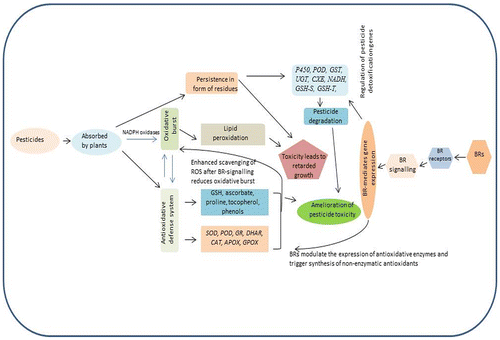
6. Role of BRs in reducing pesticide residues
Exogenous application of BRs can significantly reduce the pesticide residues in intact plants (Sharma, Bhardwaj, Kumar, Thukral, Citation2016; Sharma, Kumar, Bhardwaj, & Thukral, Citation2017; Sharma, Thakur, et al., Citation2017; Zhou et al., Citation2015). This might be due to the BR-regulated expression of various genes encoding key enzymes involved in pesticide detoxification including GST, P450 monooxygenase, POD and carboxylesterase (Sharma, Thakur, Kumar, Kesavan, Thukral, & Bhardwaj Citation2017; Xia et al., Citation2009). A significant decline in IMI residues were seen in seedlings, green leaves and pods of Brassica juncea after the seed pre-sowing treatment with 24-EBL and grown in solutions/soils amended with IMI (Sharma, Bhardwaj, Kumar, Thukral, Citation2016; Sharma, Kumar, Bhardwaj, & Thukral, Citation2017; Sharma, Thakur, Kumar, Kesavan, Thukral, & Bhardwaj Citation2017). Reduction in CHT residues in tomato plants and grapevine were observed by Zhou et al. (Citation2015) and Wang et al. (Citation2017) after the exogenous application of 24-EBL. Xia et al. (Citation2009) studied that, 24-EBL application reduced the pesticide residues (chlorpyrifos, carbendazim, cypermethrin and chlorothalonil) in cucumber plants by more than 30%. They further reported that reduced pesticide residues were accompanied by the enhanced activity of antioxidative enzymes including peroxidase (POD), glutathione-s-transferase (GST) and glutathione reductase (GR). These researchers also observed that exogenous 24-EBL application significantly enhanced the expression of P450 (P450 monooxygenase), GST and MRP (Multidrug resistance associated protein) genes responsible for pesticide detoxification in plants. BRs triggered the pesticide degradation in intact plants by 34 to 71% (chlorpyrifos in cucumber, tea, rice, broccoli and chinese cabbage, phoxim in tea and chinese chives, chlorothalonil in tomato, celery, strawberry and asparagus, omethoate in cucumber, cypermethrin in cucumber, tea, chinese cabbage and broccoli, carbofuran in garlic and chinese chives, and 3-hydroxycarbofuran in chinese chives) (Zhou et al., Citation2015). They also reported that 24-EBL enhanced the expression of genes under chlorothalonil (CHT) pesticide stress in tomato plants. Recently, it has been reported that mitogen activated protein kinase (MAPK) and nitric oxide (NO) play an important role in BR- mediated pesticide detoxification (Yin et al., Citation2016). They also demonstrated that SlMPK1 and SlMPK2 were regulated by EBR resulting in the metabolism of CHT pesticide. EBR was also noticed to regulate the activities of GST, nitrate reductase, S-nitrosoglutathione reductase and contents of S-nitrosothiol and glutathione accompanied with the reduction of CHT residues in tomato plants.
7. Conclusions and future prospects
On the basis of various reports explaining the role of BRs in pesticide detoxification and amelioration of toxicity, it is concluded that BRs hold strong future prospects in crop protection and can decrease the levels of pesticide residues in food crops. Additionally, total transcriptome sequencing/genome wide expression studies after the application of BRs in plants under pesticide toxicity can add new information to better understanding the protective roles of BRs. Moreover, studying important secondary metabolites and stress signalling pathways can help to understand the exact mechanism behind the responses of plants to pesticide stress. Further, crosstalk studies among different plant growth regulators under pesticide stress can add more information to pesticide stress management in plants.
Funding
The authors received no direct funding for this research.
Acknowledgements
Department of Science and Technology, Government of India, New Delhi is duly acknowledged for providing INSPIRE fellowship to A. Sharma.
Additional information
Notes on contributors
Anket Sharma
Anket Sharma is working as an assistant professor in DAV University, Jalandhar, India. He has done his MSc, MPhil, and PhD in Botany from Guru Nanak Dev University, Amritsar, India. His area of research is plant stress physiology and has expertise in GC-MS and statistical analysis. He has more than 50 articles/chapters in journals/books of national and international repute.
References
- Akoijam, R., & Singh, B. (2014). Persistence and metabolism of imidacloprid in rice. Bulletin of Environmental Contamination and Toxicology, 92(5), 609–615.10.1007/s00128-013-1190-5
- Ali, B., Hayat, S., & Ahmad, A. (2005). Response of germinating seeds of Cicer arietinum to 28-homobrassinolide and/or potassium. General and Applied Plant Physiology, 31(1–2), 55–63.
- Bajguz, A. (2009). Brassinosteroid enhanced the level of abscisic acid in Chlorella vulgaris subjected to short-term heat stress. Journal of Plant Physiology, 166(8), 882–886.10.1016/j.jplph.2008.10.004
- Bari, R., & Jones, J. D. (2009). Role of plant hormones in plant defence responses. Plant Molecular Biology, 69(4), 473–488.10.1007/s11103-008-9435-0
- Chahil, G. S., Mandal, K., Sahoo, S. K., Battu, R. S., & Singh, B. (2014). Risk assessment of β-cyfluthrin and imidacloprid in chickpea pods and leaves. Ecotoxicology and Environmental Safety, 101, 177–183.10.1016/j.ecoenv.2013.12.010
- Chauhan, S. S., Agrawal, S., & Srivastava, A. (2013). Effect of imidacloprid insecticide residue on biochemical parameters in potatoes and its estimation by HPLC. Asian Journal of Pharmaceutical and Clinical Research, 6, 114–117.
- Cherian, S., & Oliveira, M. M. (2005). Transgenic plants in phytoremediation: recent advances and new possibilities. Environmental Science & Technology, 39(24), 9377–9390.10.1021/es051134 l
- Choudhary, S. P., Yu, J. Q., Yamaguchi-Shinozaki, K., Shinozaki, K., & Tran, L. S. P. (2012). Benefits of brassinosteroid crosstalk. Trends in Plant Science, 17(10), 594–605.10.1016/j.tplants.2012.05.012
- Clouse, S. D. (1996). Molecular genetic studies confirm the role of brassinosteroids in plant growth and development. The Plant Journal, 10(1), 1–8.10.1046/j.1365-313X.1996.10010001.x
- Clouse, S. D. (2015). A history of brassinosteroid research from 1970 through 2005: Thirty-five years of phytochemistry, physiology, genes, and mutants. Journal of Plant Growth Regulation, 34(4), 828–844.10.1007/s00344-015-9540-7
- Clouse, S. D., & Sasse, J. M. (1998). Brassinosteroids: Essential regulators of plant growth and development. Annual Review of Plant Physiology and Plant Molecular Biology, 49(1), 427–451.10.1146/annurev.arplant.49.1.427
- Coleman, J., Blake-Kalff, M., & Davies, E. (1997). Detoxification of xenobiotics by plants: Chemical modification and vacuolar compartmentation. Trends in Plant Science, 2(4), 144–151.10.1016/S1360-1385(97)01019-4
- Das, S. K., Mukherjee, I., & Das, S. K. (2012). Dissipation of flubendiamide in/on okra (Abelmoschus esculenta (L.) Moench] fruits. Bulletin of Environmental Contamination and Toxicology, 88(3), 381–384.10.1007/s00128-011-0491-9
- Deluc, L. G., Grimplet, J., Wheatley, M. D., Tillett, R. L., Quilici, D. R., Osborne, C., … Cramer, G. R. (2007). Transcriptomic and metabolite analyses of Cabernet Sauvignon grape berry development. BMC Genomics, 8(1), 429. doi:10.1186/1471-2164-8-429
- Derevyanchuk, M., Litvinovskaya, R., Khripach, V., Martinec, J., & Kravets, V. (2015). Effect of 24-epibrassinolide on Arabidopsis thaliana alternative respiratory pathway under salt stress. Acta Physiologiae Plantarum, 37(10), 199.10.1007/s11738-015-1967-8
- Domagalska, M. A., Sarnowska, E., Nagy, F., & Davis, S. J. (2010). Genetic analyses of interactions among gibberellin, abscisic acid, and brassinosteroids in the control of flowering time in Arabidopsis thaliana. PLoS One, 5(11), e14012.10.1371/journal.pone.0014012
- Donnarumma, L., Pulcini, P., Pochi, D., Rosati, S., Lusco, L., & Conte, E. (2011). Preliminary study on persistence in soil and residues in maize of imidacloprid. Journal of Environmental Science and Health, Part B, 46(6), 469–472.
- El-Mashad, A. A. A., & Mohamed, H. I. (2012). Brassinolide alleviates salt stress and increases antioxidant activity of cowpea plants (Vigna sinensis). Protoplasma, 249, 625–635.10.1007/s00709-011-0300-7
- Fariduddin, Q., Ahmad, A., & Hayat, S. (2003). Photosynthetic response of Vigna radiata to pre-sowing seed treatment with 28-homobrassinolide. Photosynthetica, 41(2), 307–310.10.1023/B:PHOT.0000011968.78037.b1
- Farooq, M., Wahid, A., & Basra, S. M. A. (2009). Improving water relations and gas exchange with brassinosteroids in rice under drought stress. Journal of Agronomy and Crop Science, 195(4), 262–269.10.1111/jac.2009.195.issue-4
- Finlayson, D. G., & MacCarthy, H. R. (1973). Pesticides residues in plants. In C. A. Edwards (Ed.), Environmental pollution by pesticides (pp. 57–86). London and New York: Plenum Press.10.1007/978-1-4615-8942-6
- Fu, F. Q., Mao, W. H., Shi, K., Zhou, Y. H., Asami, T., & Yu, J. Q. (2008). A role of brassinosteroids in early fruit development in cucumber. Journal of Experimental Botany, 59(9), 2299–2308.10.1093/jxb/ern093
- Fujii, S., & Saka, H. (2001). Distribution of assimilates to each organ in rice plants exposed to a low temperature at the ripening stage, and the effect of brassinolide on the distribution. Plant Production Science, 4(2), 136–144.10.1626/pps.4.136
- Gajbhiye, V. T., Gupta, S., & Gupta, R. K. (2004). Persistence of imidacloprid in/on cabbage and cauliflower. Bulletin of Environmental Contamination and Toxicology, 72(2), 283–288.10.1007/s00128-003-9103-7
- Goh, W. L., Yiu, P. H., Wong, S. C., & Rajan, A. (2011). Safe use of chlorpyrifos for insect pest management in leaf mustard (Brassica juncea L. Coss). Journal of Food Agriculture and Environment, 9, 1064–1066.
- Gomes, M. D. M. A., Campostrini, E., Leal, N. R., Viana, A. P., Ferraz, T. M., do Nascimento Siqueira, L., … Zullo, M. A. T. (2006). Brassinosteroid analogue effects on the yield of yellow passion fruit plants (Passiflora edulis f. flavicarpa). Scientia Horticulturae, 110(3), 235–240.10.1016/j.scienta.2006.06.030
- Grove, M. D., Spencer, G. F., Rohwedder, W. K., Mandava, N., Worley, J. F., Warthen, J. D., … Cook, J. C. (1979). Brassinolide, a plant growth-promoting steroid isolated from Brassica napus pollen. Nature, 281(5728), 216–217.10.1038/281216a0
- Gupta, D., Bhardwaj, R., Nagar, P. K., & Kaur, S. (2004). Isolation and Characterization of brassinosteroids from leaves of Camellia sinensis (L.) O. Kuntze. Plant Growth Regulation, 43(2), 97–100.10.1023/B:GROW.0000040118.68011.6a
- Hassanzadeh, N., Esmaili Sari, A., & Bahramifar, N. (2012). Dissipation of imidacloprid in greenhouse cucumbers at single and double dosages spraying. Journal of Agricultural Science and Technology, 14, 557–564.
- Haubrick, L. L., & Assmann, S. M. (2006). Brassinosteroids and plant function: Some clues, more puzzles. Plant, Cell & Environment, 29(3), 446–457.10.1111/pce.2006.29.issue-3
- Hayat, S., & Ahmad, A. (2003). 28-homobrassinolide induced changes favoured germinability of wheat grains. Bulgarian Journal of Plant Physiology, 29(1–2), 55–62.
- Hayat, S., Ahmad, A., Mobin, M., Hussain, A., & Fariduddin, Q. (2000). Photosynthetic rate, growth, and yield of mustard plants sprayed with 28-homobrassinolide. Photosynthetica, 38(3), 469–471.
- Hayat, S., Ali, B., Aiman Hasan, S. A., & Ahmad, A. (2007). Brassinosteroid enhanced the level of antioxidants under cadmium stress in Brassica juncea. Environmental and Experimental Botany, 60(1), 33–41.10.1016/j.envexpbot.2006.06.002
- Huang, M. T., Lu, Y. C., Zhang, S., Luo, F., & Yang, H. (2016). Rice (Oryza sativa) laccases involved in modification and detoxification of herbicides atrazine and isoproturon residues in plants. Journal of Agricultural and Food Chemistry, 64(33), 6397–6406.10.1021/acs.jafc.6b02187
- Jiang, Y. P., Cheng, F., Zhou, Y. H., Xia, X. J., Shi, K., & Yu, J. Q. (2012). Interactive effects of CO2 enrichment and brassinosteroid on CO2 assimilation and photosynthetic electron transport in Cucumis sativus. Environmental and Experimental Botany, 75, 98–106.10.1016/j.envexpbot.2011.09.002
- Zhang, J. J., Lu, Y. C., Zhang, J. J., Tan, L. R., & Yang, H. (2014). Accumulation and toxicological response of atrazine in rice crops. Ecotoxicology and Environmental Safety, 102, 105–112.10.1016/j.ecoenv.2013.12.034
- Jyot, G., Mandal, K., Battu, R. S., & Singh, B. (2013). Estimation of chlorpyriphos and cypermethrin residues in chilli (Capsicum annuum L.) by gas–liquid chromatography. Environmental Monitoring and Assessment, 185(7), 5703–5714.10.1007/s10661-012-2977-2
- Kadam, D. R., Deore, B. V., & Umate, S. M. (2014). Residues and dissipation of imidacloprid in pomegranate fruits. Asian Journal of Bio Science, 9(2), 246–250.10.15740/HAS/AJBS
- Kaňa, R., Špundová, M., Ilık, P., Lazár, D., Klem, K., Tomek, P., … Prášil, O. (2004). Effect of herbicide clomazone on photosynthetic processes in primary barley (Hordeum vulgare L.) leaves. Pesticide Biochemistry and Physiology, 78(3), 161–170.
- Kanwar, M. K., Bhardwaj, R., Arora, P., Chowdhary, S. P., Sharma, P., & Kumar, S. (2012). Plant steroid hormones produced under Ni stress are involved in the regulation of metal uptake and oxidative stress in Brassica juncea L. Chemosphere, 86(1), 41–49.10.1016/j.chemosphere.2011.08.048
- Kanwar, M. K., Bhardwaj, R., Chowdhary, S. P., Arora, P., Sharma, P., & Kumar, S. (2013). Isolation and characterization of 24-Epibrassinolide from Brassica juncea L. and its effects on growth, Ni ion uptake, antioxidant defense of Brassica plants and in vitro cytotoxicity. Acta Physiologiae Plantarum, 35(4), 1351–1362.10.1007/s11738-012-1175-8
- Kanwar, M. K., Poonam, & Bhardwaj, R. (2015). Arsenic induced modulation of antioxidative defense system and brassinosteroids in Brassica juncea L. Ecotoxicology and Environmental Safety, 115, 119–125.10.1016/j.ecoenv.2015.02.016
- Kaur, G., Sharma, A., Guruprasad, K., & Pati, P. K. (2014). Versatile roles of plant NADPH oxidases and emerging concepts. Biotechnology Advances, 32(3), 551–563.10.1016/j.biotechadv.2014.02.002
- Khan, T. A., Fariduddin, Q., & Yusuf, M. (2015). Lycopersicon esculentum under low temperature stress: An approach toward enhanced antioxidants and yield. Environmental Science and Pollution Research, 22, 14178–14188.10.1007/s11356-015-4658-5
- Kooner, R., Sahoo, S. K., Singh, B., & Battu, R. S. (2010). Dissipation kinetics of flubendiamide and thiacloprid on tomato (Lycopersicon esculentum Mill) and soil. Quality Assurance and Safety of Crops & Foods, 2(1), 36–40.10.1111/(ISSN)1757-837X
- Krishna, P. (2003). Brassinosteroid-mediated stress responses. Journal of Plant Growth Regulation, 22(4), 289–297.10.1007/s00344-003-0058-z
- Kumar, K. S., Choo, K. S., Yea, S. S., Seo, Y., & Han, T. (2010). Effects of the phenylurea herbicide diuron on the physiology of Saccharina japonica aresch. Toxicology and Environmental Health Sciences, 2(3), 188–199.10.1007/BF03216505
- Kumar, R., & Dikshit, A. K. (2001). Assessment of imidacloprid in Brassica environment. Journal of Environmental Science and Health, Part B, 36(5), 619–629.10.1081/PFC-100106190
- Lu, Y. C., Feng, S. J., Zhang, J. J., Luo, F., Zhang, S., & Yang, H. (2016). Genome-wide identification of DNA methylation provides insights into the association of gene expression in rice exposed to pesticide atrazine. Scientific Reports, 6, 328. doi:10.1038/srep18985
- Mandal, K., Chahil, G. S., Sahoo, S. K., Battu, R. S., & Singh, B. (2010). Dissipation kinetics of β-Cyfluthrin and imidacloprid in brinjal and soil under subtropical conditions of Punjab, India. Bulletin of Environmental Contamination and Toxicology, 84(2), 225–229.10.1007/s00128-009-9903-5
- Mandal, K., & Singh, B. (2014). Persistence and metabolism of Fipronil in sugarcane leaves and juice. Bulletin of Environmental Contamination and Toxicology, 92(2), 220–224.10.1007/s00128-013-1176-3
- Mandava, N. B. (1988). Plant growth-promoting brassinosteroids. Annual Review of Plant Physiology and Plant Molecular Biology, 39(1), 23–52.10.1146/annurev.pp.39.060188.000323
- Mohapatra, S., Ahuja, A. K., Deepa, M., Sharma, D., Jagadish, G. K., & Rashmi, N. (2010). Persistence and dissipation of flubendiamide and des-iodo flubendiamide in cabbage (Brassica oleracea Linne) and soil. Bulletin of Environmental Contamination and Toxicology, 85(3), 352–356.10.1007/s00128-010-0063-4
- Mohapatra, S., Ahuja, A. K., Sharma, D., Deepa, M., Prakash, G. S., & Kumar, S. (2011). Residue study of imidacloprid in grapes (Vitis vinifera L.) and soil. Quality Assurance and Safety of Crops & Foods, 3(1), 24–27.10.1111/qas.2011.3.issue-1
- Mukherjee, I., & Gopal, M. (2000). Environmental behaviour and translocation of imidacloprid in eggplant, cabbage and mustard. Pest Management Science, 56(10), 932–936.10.1002/(ISSN)1526-4998
- Mukherjee, I., Gopal, M., & Mathur, D. S. (2007). Behavior of β-Cyfluthrin after foliar application on chickpea (Cicer aretinium L. ) and Pigeon Pea (Cajanus cajan L.). Bulletin of Environmental Contamination and Toxicology, 78(1), 85–89.10.1007/s00128-007-9018-9
- Mwevura, H. (2000). Study on the levels of organochlorine pesticide residues from selected aquatic bodies of Tanzania (MSc thesis). University of Dar es Salaam, Tanzania.
- Nasr, H. M., Abbassy, M. A., Marzouk, M. A., & Mansy, A. S. (2014). Determination of imidacloprid and tetraconazol residues in cucumber fruits. Journal of Pollution Effects & Control, 2. doi:10.4172/jpe.1000107
- Oh, E., Zhu, J. Y., & Wang, Z. Y. (2012). Interaction between BZR1 and PIF4 integrates brassinosteroid and environmental responses. Nature Cell Biology, 14(8), 802.10.1038/ncb2545
- Özdemir, F., Bor, M., Demiral, T., & Türkan, İ. (2004). Effects of 24-epibrassinolide on seed germination, seedling growth, lipid peroxidation, proline content and antioxidative system of rice (Oryza sativa L.) under salinity stress. Plant Growth Regulation, 42(3), 203–211.10.1023/B:GROW.0000026509.25995.13
- Paramasivam, M., & Banerjee, H. (2012). Persistence and dissipation of the insecticide flubendiamide and its metabolite desiodo flubendiamide residues in tomato fruit and soil. Bulletin of Environmental Contamination and Toxicology, 88(3), 344–348.10.1007/s00128-011-0461-2
- Paramasivam, M., Selvi, C., & Chandrasekaran, S. (2014). Persistence and dissipation of flubendiamide and its risk assessment on gherkin (Cucumis anguria L.). Environmental Monitoring and Assessment, 186(8), 4881–4887.10.1007/s10661-014-3745-2
- Pilati, S., Perazzolli, M., Malossini, A., Cestaro, A., Demattè, L., Fontana, P., … Moser, C. (2007). Genome-wide transcriptional analysis of grapevine berry ripening reveals a set of genes similarly modulated during three seasons and the occurrence of an oxidative burst at vèraison. BMC Genomics, 8(1), 428. doi:10.1186/1471-2164-8-428
- Pramanik, S. K., Bhattacharyya, J., Dutta, S., Dey, P. K., & Bhattacharyya, A. (2006). Persistence of acetamiprid in/on mustard (Brassica juncea L.). Bulletin of Environmental Contamination & Toxicology, 76(2), 356–360. doi:10.1007/s00128-006-0929-7
- Romeh, A. A., Mekky, T. M., Ramadan, R. A., & Hendawi, M. Y. (2009). Dissipation of profenofos, imidacloprid and penconazole in tomato fruits and products. Bulletin of Environmental Contamination and Toxicology, 83(6), 812.10.1007/s00128-009-9852-z
- Sanyal, D., Chakma, D., & Alam, S. (2008). Persistence of a neonicotinoid insecticide, acetamiprid on chili (Capsicum annum L.). Bulletin of Environmental Contamination and Toxicology, 81(4), 365–368.10.1007/s00128-008-9479-5
- Sasse, J. M. (2003). Physiological actions of brassinosteroids: An update. Journal of Plant Growth Regulation, 22(4), 276–288.10.1007/s00344-003-0062-3
- Sasse, J. M., Smith, R., & Hudson, I. (1995). Effect of 24-epibrassinolide on germination of seeds of Eucalyptus camaldulensis in saline conditions. In Proceedings-plant growth regulator society of america-annual meeting (Vol. 22, pp. 136–141). Plant Growth Regulator Society of America.
- Shahzad, B., Tanveer, M., Che, Z., Rehman, A., Cheema, S. A., Sharma, A., … Zhaorong, D. (2018). Role of 24-epibrassinolide (EBL) in mediating heavy metal and pesticide induced oxidative stress in plants: A review. Ecotoxicology and Environmental Safety, 147, 935–944.10.1016/j.ecoenv.2017.09.066
- Sharma, A., Bhardwaj, R., Kumar, V., & Thukral, A. K. (2016). GC-MS studies reveal stimulated pesticide detoxification by brassinolide application in Brassica juncea. Environmental Science and Pollution Research, 23(14), 14518–14525.10.1007/s11356-016-6650-0
- Sharma, A., Kumar, V., Bhardwaj, R., & Thukral, A. K. (2017). Seed pre-soaking with 24-epibrassinolide reduces the imidacloprid pesticide residues in green pods of Brassica juncea L. Toxicological & Environmental Chemistry, 99(1), 95–103.10.1080/02772248.2016.1146955
- Sharma, A., Kumar, V., Kanwar, M. K., Thukral, A. K., & Bhardwaj, R. (2016). Multivariate analysis reveals the role of 24-epibrassinolide in elemental uptake by Brassica juncea L. under imidacloprid toxicity. Pollution Research, 35, 749–756.
- Sharma, A., Kumar, V., Kanwar, M. K., Thukral, A. K., & Bhardwaj, R. (2017). Ameliorating imidacloprid induced oxidative stress by 24-epibrassinolide in Brassica juncea L. Russian Journal of Plant Physiology, 64(4), 509–517.10.1134/S1021443717040124
- Sharma, A., Kumar, V., Kohli, S. K., Thukral, A. K., & Bhardwaj, R. (2015a). Phytochemicals in Brassica juncea L. seedlings under imidacloprid-epibrassinolide treatment using GC-MS. Journal of Chemical and Pharmaceutical Research, 7(10), 708–711.
- Sharma, A., Kumar, V., Singh, R., Thukral, A. K., & Bhardwaj, R. (2015b). 24-Epibrassinolide induces the synthesis of phytochemicals effected by imidacloprid pesticide stress in Brassica juncea L. Journal of Pharmacognosy and Phytochemistry, 4, 60–64.
- Sharma, A., Kumar, V., Singh, R., Thukral, A. K., & Bhardwaj, R. (2016). Effect of seed pre-soaking with 24-epibrassinolide on growth and photosynthetic parameters of Brassica juncea L. in imidacloprid soil. Ecotoxicology and Environmental Safety, 133, 195–201.10.1016/j.ecoenv.2016.07.008
- Sharma, A., Kumar, V., Thukral, A. K., & Bhardwaj, R. (2016). Epibrassinolide-imidacloprid interaction enhances non-enzymatic antioxidants in Brassica juncea L. Indian Journal of Plant Physiology, 21(1), 70–75.10.1007/s40502-016-0203-x
- Sharma, A., Kumar, V., Thukral, A. K., & Bhardwaj, R. (2017). 24-epibrassinolide restores the synthesis of proteins and amino acids in Brassica juncea L. plants under imidacloprid stress. Journal of Horticultural Research, 25. doi:10.1515/johr-2017
- Sharma, I., Bhardwaj, R., & Pati, P. K. (2012). Mitigation of adverse effects of chlorpyrifos by 24-epibrassinolide and analysis of stress markers in a rice variety Pusa Basmati-1. Ecotoxicology and Environmental Safety, 85, 72–81.10.1016/j.ecoenv.2012.07.003
- Sharma, I., Bhardwaj, R., & Pati, P. K. (2013). Stress modulation response of 24-epibrassinolide against imidacloprid in an elite indica rice variety Pusa Basmati-1. Pesticide Biochemistry and Physiology, 105(2), 144–153.10.1016/j.pestbp.2013.01.004
- Sharma, I., Bhardwaj, R., & Pati, P. K. (2015). Exogenous application of 28-homobrassinolide modulates the dynamics of salt and pesticides induced stress responses in an elite rice variety Pusa Basmati-1. Journal of Plant Growth Regulation, 34(3), 509–518.10.1007/s00344-015-9486-9
- Sharma, P., & Bhardwaj, R. (2007). Effects of 24-epibrassinolide on growth and metal uptake in Brassica juncea L. under copper metal stress. Acta Physiologiae Plantarum, 29(3), 259–263.10.1007/s11738-007-0032-7
- Sharma, S., & Singh, B. (2014). Persistence of imidacloprid and its major metabolites in sugarcane leaves and juice following its soil application. International Journal of Environmental Analytical Chemistry, 94(4), 319–331.10.1080/03067319.2013.853759
- Sharma, A., Thakur, S., Kumar, V., Kanwar, M. K., Kesavan, A. K., Thukral, A. K., … Ahmad, P. (2016). Pre-sowing seed treatment with 24-epibrassinolide ameliorates pesticide stress in Brassica juncea L. through the modulation of stress markers. Frontiers. Plant Science, 7, 1569. doi:10.3389/fpls.2016.01569
- Sharma, A., Thakur, S., Kumar, V., Kesavan, A. K., Thukral, A. K., & Bhardwaj, R. (2017). 24-epibrassinolide stimulates imidacloprid detoxification by modulating the gene expression of Brassica juncea L. BMC Plant Biology, 17(1), 5336.10.1186/s12870-017-1003-9
- Siddiqui, Z. S., & Ahmed, S. (2006). Combined effects of pesticide on growth and nutritive composition of soybean plants. Pakistan Journal of Botany, 38(3), 721–733.
- Singh, G., Sahoo, S. K., Takkar, R., Battu, R. S., Singh, B., & Chahil, G. S. (2011). Residual behaviour and risk assessment of flubendiamide on Chickpea (Cicer arietinum L.). Chemosphere, 84(10), 1416–1421.10.1016/j.chemosphere.2011.04.065
- Singh, P. P., Singh, B., & Battu, R. S. (1990). Residues of cypermethrin, fenvalerate and deltamethrin on cauliflower. Phytoparasitica, 18(2), 153–158.10.1007/BF02981232
- Sinha, S., & Gopal, M. (2002). Evaluating the safety of β-cyfluthrin insecticide for usage in eggplant (Solanum melongena L.) crop. Bulletin of Environmental Contamination and Toxicology, 68(3), 400–405.
- Symons, G. M., Davies, C., Shavrukov, Y., Dry, I. B., Reid, J. B., & Thomas, M. R. (2006). Grapes on steroids. Brassinosteroids are involved in grape berry ripening. Plant Physiology, 140(1), 150–158.
- Untiedt, R., & Blanke, M. M. (2004). Effects of fungicide and insecticide mixtures on apple tree canopy photosynthesis, dark respiration and carbon economy. Crop Protection, 23(10), 1001–1006.10.1016/j.cropro.2004.02.012
- Vardhini, B. V., & Anjum, N. A. (2015). Brassinosteroids make plant life easier under abiotic stresses mainly by modulating major components of antioxidant defense system. Frontiers in Environmental Science, 2, 67. doi:10.3389/fenvs.2014.00067
- Vardhini, B. V., & Rao, S. S. R. (2002). Acceleration of ripening of tomato pericarp discs by brassinosteroids. Phytochemistry, 61(7), 843–847.10.1016/S0031-9422(02)00223-6
- Wachsman, M. B., López, E. M., Ramirez, J. A., Galagovsky, L. R., & Coto, C. E. (2000). Antiviral effect of brassinosteroids against herpes virus and arenaviruses. Antiviral Chemistry and Chemotherapy, 11(1), 71–77.10.1177/095632020001100107
- Wang, Z., Jiang, Y., Peng, X., Xu, S., Zhang, H., Gao, J., & Xi, Z. (2017). Exogenous 24-epibrassinolide regulates antioxidant and pesticide detoxification systems in grapevine after chlorothalonil treatment. Plant Growth Regulation, 81(3), 455–466.10.1007/s10725-016-0223-6
- Westwood, F., Bean, K. M., Dewar, A. M., Bromilow, R. H., & Chamberlain, K. (1998). Movement and persistence of [14C] imidacloprid in sugar-beet plants following application to pelleted sugar-beet seed. Pesticide Science, 52(2), 97–103.10.1002/(SICI)1096-9063(199802)52:2<>1.0.CO;2-F
- Xavier, G., Chandran, M., George, T., Beevi, S. N., Mathew, T. B., Paul, A., … Rajith, R. (2014). Persistence and effect of processing on reduction of fipronil and its metabolites in chilli pepper (Capsicum annum L.) fruits. Environmental Monitoring and Assessment, 186(9), 5429–5437.10.1007/s10661-014-3792-8
- Xia, X. J., Huang, Y. Y., Wang, L., Huang, L. F., Yu, Y. L., Zhou, Y. H., & Yu, J. Q. (2006). Pesticides-induced depression of photosynthesis was alleviated by 24-epibrassinolide pretreatment in Cucumis sativus L. Pesticide Biochemistry and Physiology, 86(1), 42–48.10.1016/j.pestbp.2006.01.005
- Xia, X. J., Zhang, Y., Wu, J. X., Wang, J. T., Zhou, Y. H., Shi, K., … Yu, J. Q. (2009). Brassinosteroids promote metabolism of pesticides in cucumber. Journal of Agricultural and Food Chemistry, 57(18), 8406–8413.10.1021/jf901915a
- Yin, Y. L., Zhou, Y., Zhou, Y. H., Shi, K., Zhou, J., Yu, Y., … Xia, X. J. (2016). Interplay between mitogen-activated protein kinase and nitric oxide in brassinosteroid-induced pesticide metabolism in Solanum lycopersicum. Journal of Hazardous Materials, 316, 221–231.10.1016/j.jhazmat.2016.04.070
- Yu, J. Q., Huang, L. F., Hu, W. H., Zhou, Y. H., Mao, W. H., Ye, S. F., & Nogués, S. (2004). A role for brassinosteroids in the regulation of photosynthesis in Cucumis sativus. Journal of Experimental Botany, 55(399), 1135–1143.10.1093/jxb/erh124
- Yuan, G. F., Jia, C. G., Li, Z., Sun, B., Zhang, L. P., Liu, N., & Wang, Q. M. (2010). Effect of brassinosteroids on drought resistance and abscisic acid concentration in tomato under water stress. Scientia Horticulturae, 126(2), 103–108.10.1016/j.scienta.2010.06.014
- Zhang, M., Zhai, Z., Tian, X., Duan, L., & Li, Z. (2008). Brassinolide alleviated the adverse effect of water deficits on photosynthesis and the antioxidant of soybean (Glycine max L.). Plant Growth Regulation, 56(3), 257–264.10.1007/s10725-008-9305-4
- Zhou, Y., Xia, X., Yu, G., Wang, J., Wu, J., Wang, M., … Gan, J. (2015). Brassinosteroids play a critical role in the regulation of pesticide metabolism in crop plants. Scientific Reports, 5, 31. doi:10.1038/srep09018