Abstract
This study examined the effects of pH, temperature, NaCl concentration and heat resistances of Raoultella spp. isolated from a seafood processing plant in Thailand. The ability to resist to cleaning and sanitizing agents were also investigated. The effect of growth medium (Tryptic Soy Broth) adjusted to various pHs (3, 4, 5, 6, 7, 8, 9, 10) revealed Raoultella ornithinolytica and R. terrigena were able to grow well at pH 6–9, slow growth at 4 and 10 but not at strong acidic pH 3. Growth at different temperature was done (4, 15, 20, 37, 40°C) and found optimum growth was ranged at 15–37°C. Sodium chloride concentrations test was at 1%, 1.5%, 2.5%, 3.5%, 4.5%, 5.5% and 6.5% revealed the optimal growth at 1–4.5% NaCl. D-values of both species gave similar result as 0.66–0.79 min at 57°C, with Z-value 4.2–4.5°C. When cell suspension and biofilm were exposed to cleaning agent (sodium hydroxide, lauryl dimethylamine oxide, and sodium hypochlorite), and three sanitizers, namely, sodium hypochlorite (NaClO), hydrogen peroxide (H2O2), and ozonated water. NaClO was able to eliminate single biofilm of Raoultella spp. at 50 ppm in 5 min while ozone had less efficacy to kill biofilm cells. H2O2 at 1% and 2% succeeded to eliminate biofilm. This finding properties of Raoultella spp. will be useful for designing strategies to prevent and control contamination these species regarding seafood plant sanitation.
PUBLIC INTEREST STATEMENT
This study focus on Raoultella spp. (R. ornithinolytica and R. terrigena) because of the capacity to be a major spoilage bacteria in seafood products. These species has also been mentioned as the potential of histamine producing bacteria. Currently, some studies have been reported on the growth characteristics of R. terrrigena, but only a few mentioned the growth factors pertaining to R. ornithinolytica. The food industry realized that adhesion and colonization of bacteria may cause problems, and an important source of bacterial contamination. Therefore, this study investigated the growth parameters of the two species of Raoultella isolated from seafood processing environments; the cleaning of biofilm produced under stress conditions simulated to seafood processing area was also examined
This article reveals the growth ability, thermal resistance of R. ornithinolytica and R. terrrigena. Expectation that these information may assist to the control strategies to prevent and control the growth of these bacteria, and to enhance the safety of seafood products.
Competing Interests
The authors declared that they have no competing interests.
1. Introduction
Seafood products are one of the important export commodities in Thailand, exporting of seafood has a major share on country export revenue count as 2129 million USD in the year 2017 (Ministry of Commerce, Citation2018). Quality and safety of seafood products are the major concern of the Thai food industry, the detention of exporting seafood products has been reported occasional due to bacterial contamination. In the year 2015, according to RASFF database, European Commission reported that fishery products from Thailand faced the border rejection due to microbiological quality; bacterial contamination (Listeria monocytogenes, Staphylococcus epidermis, and high aerobic plate count) was found in canned tuna and frozen surimi (European commission, Citation2017).
An appropriate cleaning and sanitization in the seafood industry became the issue of concern. Formation of biofilms, which acts as a contamination reservoir, may form if the cleaning method and chemical use are not appropriate. Biofilm is regarded as a source of potential spoilage and pathogenic microorganisms, and has potentially re-contaminated into a finished product if the process is lack of hygienic. However, some microorganisms in seafood environment have been reported that they have ability to form biofilm (Shikongo-Nambabi, Shoolongela, & Schneider, Citation2012). The formation and development of biofilms depend on the specific bacteria strain, material surface properties and environmental parameters such as pH, temperature and nutrient levels (Tezel & Şanhbaba, Citation2017).
In 2014, Hoa investigated the microbial population in the biofilm that had adhered to different locations on equipment surfaces at a seafood processing plant in Thailand and found that two-third of the major biofilm producers consisted of the genus Raoultella (R. ornithinolytica and R. terrigena) (30%) and Pseudomonas spp. (30%), the remaining was Serratia (17%). Many research works have reported the types of microorganisms associated with seafood plant environments (Feldhusen, Citation2000; Møretro et al. Citation2016). Among these microorganisms, some species have been identified as biological hazards which should be controlled in seafood processing (Feldhusen, Citation2000).
The genus Raoultella is within the Family Enterobacteriaceae, this genus consists of Gram-negative, non-motile, aerobic, oxidase-negative, and facultative anaerobic bacilli (O’Connell, Kelly, & NiRiain, Citation2010). They are found in aquatic environment, fish, and insects (Sandal & Ozen, Citation2014). Raoultella spp. have recently been separated from the former genus Klebsiella based on 16S rRNA sequences and rpoB genes (Drancourt, Bollet, Carta, & Rousselier, Citation2001; Park, Jarquin, Hanning, Almeida, & Ricke, Citation2011), resulting in the creation of the new genus Raoultella. Members of the Raoultella genus include four species, namely, Raoultella electrica, R. terrigena, R. planticola, and R. ornithinolytica (Chun, Yun, Huh, & Lee, Citation2015). The members of this genus have been well documented as human pathogen and cause the diseases on many occasions, but with a low prevalent rate of human bloodstream infections (Chun et al., Citation2015). In food, the report in 2011 mentioned that R. terrigena was isolated from the intestine of puffer fish and this bacteria was able to produce tetrodotoxin (Yu, Yu, Ho, & Lee, Citation2011). R. ornithinolytica was found from the gut of fish, and other insect species such as ticks and termites (Morais, Daporta, Bao, Campello, & Andŕes, Citation2009). Raoultella spp. became the genus of interest in food products since bacteria was reported as a potent histamine producer, particular in R. ornithinolytica (Dalgaard & Emborg, Citation2009; Tsai et al., Citation2007). Hoa (Citation2014) found both species, R. ornithinolytica and R. terrigena, had ability to form biofilm in single cultivation under stressed condition simulated to seafood process condition and could compete with Pseudomonas aeruginosa and Listeria monocytogenes when cultured as mixed biofilm.
The problem of biofilm in the food industry not only impacts on the shelf life of the products but may cause foodborne diseases, if the biofilm is formed by the pathogenic strain. Many species have been used as microbial indicator for biofilm formation such as P. aeruginosa and S. epidermidis (Dunne, Citation2002). Recently the members of Enterobacteriaceae in processing plants, namely, Pantoea, Enterobacter, Raoultella, Escherichia, and Leclecia were proposed to use as a hygienic indicator for plant sanitation by Lim, Lee, Kim, and Koo (Citation2017). These prevalent species could be used as the microbial target for cleaning and sanitizing in the seafood industry. Thus, routine inspection of Raoultella could indicate the accumulation of enteric bacteria. It also could be possible to prevent the cause of allergic symptom due to histamine in seafood consumption.
Based on research data supported that Raoultella spp. contamination in seafood products could affect to consumers safety. Therefore, the investigation of growth parameters of R. ornithinolytica and R. terrigena isolated from a seafood processing plant could help to indicate the occurrence of these species in an seafood process environment. The investigation of the growth and survival of R. ornithinolytica and R. terrigena against various factors such as temperature, pH, and sodium chloride was conducted. Heat resistance of both species was also investigated. The challenges to against the four chemicals common used in seafood industry were examined. The expected result could be used as the information to assist the strategies on cleaning and sanitizing to prevent and control the bacterial contamination particular biofilm formation in seafood processing plant.
2. Materials and methods
2.1. Microorganisms and inoculum preparation
R. ornithinolytica and R. terrigena were obtained from Hoa, Mahakarnchanakul, Sajjaanantakul, and Kitpreechavanich (Citation2015); these isolates were identified and isolated from a biofilm on equipment surfaces in a seafood processing plant in Thailand. Bacterial strains were maintained as frozen stocks at −18°C in cryotubes (Scharlau Microbiology, Spain). One loop was added into 10 mL Tryptic Soy Broth (TSB; Merck, Germany) and incubated at 37°C for 24-h. Then, the culture was streaked on Tryptic Soy Agar (TSA; Merck, Germany) to obtain a single colony. A single colony was then suspended in peptone water and a 10 μL was added into 10 mL of TSB and incubated at 37°C for 24-h. Subcultured consecutively for 2 days was done and the third day, culture that had been incubated at 37°C for 18 h prior was used as inoculum.
2.2. Biofilm preparation
Stainless steel coupons (304, finish#2B) were transferred to a modified CDC bioreactor. A biofilm reactor contains 24 coupons (2 cm × 5 cm × 0.8 cm) and sterilized at 121°C for 15 min in an autoclave, then 2,000 mL of 0.3 g/L TSB were added in the bioreactor. After that, inoculum about 2 mL was added in the bioreactor (Hoa, Citation2014). The initial population of bacteria in the bioreactor of each sample was about 5.0 ± 0.5 log CFU/mL. The bioreactors were incubated at 15°C for 10 days, a low temperature was set to imitate the biofilm formation in seafood facilities. Every day fresh medium will be replaced and let stand for 8 h, then drained out and let the bioreactor empty for 16 h, next day the medium will be refilled and consequently the similar manner was done for every day until day 10. All coupon samples were taken after 10 days for chemical testing.
2.3. Cell morphology and growth rate observation
Inocula of R. ornithinolytica and R. terrigena were streaked on TSA and incubated at 37°C for 24 h. Cell morphology and gram staining were done according to the Food and Drug Administration instructions (Food and Drug Administration [FDA], Citation1995). Growth curve observation at 37°C was conducted from an initial population of R. ornithinolytica and R. terrigena at 4.0 ± 0.5 log CFU/mL. At sampling time intervals of 1–2 h, samples were taken and counted using spiral plating (Spiral Biotech model Autoplate 4000, USA) on TSA in duplicate until the bacterial growth had reached the stationary phase by 18 h. The cell populations were plotted as the logarithm of viable cells per ml against the incubation time, and the generation time was calculated by G (generation time) = (time, in min or h)/n (number of generations) and specific growth rate by the three-phase linear model (Koch, Citation1995).
2.4. Bacterial growth observation at various pH, temperature and NaCl concentration
TSB was adjusted to tested pH values of 3, 4, 5, 6, 7, 8, 9, and 10, using 1 N NaOH (Merck, Germany) and 1 M HCl (Merck, Germany) to the final tested pH, and monitored using a pH meter (EUTECH instruments, Malaysia) before sterilized. An amount of 5 mL of culture (initial population 4 log CFU/mL) was added to 45 mL to TSB, then transferred 3 mL of cell suspension into each test tube, and incubated at 37°C. Samples were taken at 0, 6, 18, and 24 h, then serially diluted and using spiral plating spread on TSA. A culture was incubated at 37°C for 24 h before counting.
Similar cultivation and enumeration were done regarding bacteria growth behavior to pH. Fifty viable cells from 250 mL flasks were separately added in a test tube, each tubes containing 3 mL were incubated separately at 4°C, 15°C, 20°C, 37°C, and 40°C. Cells were also challenged at 1.0%, 1.5%, 2.5%, 3.5%, 4.5%, 5.5%, and 6.5% NaCl (RCI Labscan, Thailand) (w/v). NaCl was added in TSB with an initial 0.5% NaCl as present in the formula. The counts were determined on TSA at sampling times of 0, 6, 18, and 24 h.
2.5. Thermal resistance enumeration
An amount of 1 mL of inoculum (initial population 5 log CFU/ml) was added to 9 mL pre-heated TSB in tubes at 53°C, 55°C, and 57°C, then placed in a water bath (Memmert, Germany). Survivors were enumerated by taking samples at 53°C, 55°C, and 57°C after 0, 5, 10, 15, and 20 min. After the samples were removed from the bath, the tubes were immediately immersed in an ice-water bath (4°C) for 30 s, serially diluted and using spiral plating on TSA. Survival curves by plotting the logarithm of survivals and their corresponding heating times.
2.6. Chemical test for planktonic cells and biofilm
The chemical stocks were prepared following the manufacturer’s instructions in sterile 0.85% saline solution. Ozonated water was produced using an ozone generator (ED-0GR6, EcoDesign, Inc., Ltd., Japan) following the recommended concentration and time according to the USFDA (Rice & Graham., Citation2001). Bacterial cell suspensions in 0.85% saline solution were utilized as the control. For each chemical tested, an analysis was conducted in triplicate, with duplicate samples taken for each trial. One mL of suspended cells (population 8 log CFU/mL) was added to 100 mL of 0.85% saline solution in a 250 mL Erlenmeyer flask to reach a final concentration of 6 log CFU/mL. Chemical testing was done by adding 1 mL of suspended cells to the prepared test solution according to Table . Cleaning agent composes of sodium hydroxide 5%, Lauryl dimethylamine oxide 10% and sodium hypochlorite 4.6%. Test solutions were immediately mixed at 628 rad/min using a shaker (SK-300, Jeio Tech, Korea). After the exposure time, 1 mL of the aliquot from test and control cell suspension was immediately serially diluted in 0.85% saline solution and plated on TSA, incubated at 37°C for 24 h and enumerated for survivors. The chlorine and ozone concentrations were measured using N, N-diethyl-p-phenelenediamine (DPD) tablets No.1 and No.4 (Dulcotest DT1 Photometer, PCH 40156, Germany).
Table 1. Concentration and exposure time of chemical agents testing for planktonic cells and biofilm
Biofilm elimination of R. ornithinolytica was tested according to Table . Biofilm on stainless steel coupon (SS) was rinsed three times with 30 mL of sterile water to remove loosely attached cells. The initial number of attached bacteria on coupon was quantified by the swab method. Before subjecting to chemicals, all coupons were rinsed with sterile water then exposed to sodium hypochlorite, ozone, hydrogen peroxide. After treated with chemicals, the coupons were held in a 30 ml 1% sodium thiosulfate for 1 min to neutralize any remaining chemicals and thereafter rinsed with 30 mL sterile water. The residual cells on SS on one side of coupon were determined. The swab heads were broken off into a test tube containing 10 mL of sterile saline solution (0.85%), shake for 60 s, serial dilution and spread duplicate onto TSA. The culture was incubated at 37°C for 24 h and count was expressed as log CFU/cm2.
3. Results and discussion
3.1. Cell morphology and growth observation
The characteristics of R. ornithinolytica and R. terrigena cultivated 18 h on TSA at 37°C were observed and found the smooth circular colony with convex and entire margin. The colony diameter of both species was similar by 2.20 ± 0.01 and 2.00 ± 0.01 mm. According to microscopic examination under Gram-negative strain, R. ornithinolytica and R. terrigena cell shape was short rod, with cell length 1.73 ± 0.19 μm and 1.60 ± 0.08 μm, respectively. Raoultella spp. has been reported to closely related to Enterobacteriaceae group (O’Connell et al., Citation2010; Saha, Bechanko, Bestervelt, & Donofrio, Citation2011), and found to be associated with aquatic environment and remarked as a major histamine producers similar to genus Morganella (Dalgaard & Emborg, Citation2009). In seafood products such as dried milkfish R. ornithinolytica has been isolated and reported to produce more than 800 mg histamine/kg in laboratory media supplement 1% L-histidine (Tsai et al. Citation2007) while R. planticola produced more than 1,000 mg histamine/kg in fresh tuna (Dalgaard & Emborg, Citation2009).
The growth observation of R. ornithinolytica and R. terrigena was done at 37°C. Initial populations of R. ornithinolytica and R. terrigena cultured in TSB at 0 h were 2.9–3.1 log CFU/mL, cells reached a maximum population about 9 log CFU/mL after 18 h. Log phase was started after 2 h of incubation and entered to the mid-log phase within 6 h. The growth rate of R. ornithinolytica and R. terrigena were similar as 0.55 and 0.56 ∆log CFU/hr at 37°C (data not shown). The test was done at 37°C since at this temperature is used for evaluating the virulence properties of various human pathogenic bacteria for instance in Listeria (Johansson et al., Citation2002). There was an evidence of human infections caused by bacteria of genus Raoultella, and case of enteric fever-like syndrome and bacteremia caused by R. ornithinolytica was reported by Morais et al. (Citation2009); Sandal and Ozen (Citation2014).
3.2. Bacterial growth observation at various pH, temperature and NaCl concentration
3.2.1. Effect of pH
The growth observation of the R. ornithinolytica and R. terrigena against pH was done at 37°C in TSB. The results showed the optimum pH was at pH 6–9 when cultivated in TSB at 18 h; R. ornithinolytica and R. terrigena cells rapidly multiplied even at alkaline pH range of 8–9, while they grew slowly at pH 4 and 10. No growth was observed at strong acidic pH 3 which was clearly as at pH acidic will not support the growth of R. ornithinolytica and R. terrigena compare to alkaline pH (Figure ,b)). In this study, the result was similarly found by Yu et al. (Citation2011), in which R. terrigena grew well at pH 7–9 but not at pH 5–6 in sterilized Ocean Research Institute medium culture (ORM) according to Yu et al. (Citation2011). The different compositions in ORM medium compared to TSB may effect on the ability to resist at lower pH as pH 5–6 besides the incubation was done at 30°C for 3–5 days. Considering food composition such as fresh tuna meat or canned tuna meat which has a pH range of 5.9–6.2; if the tuna is contaminated with Raoultella spp., this pH range obviously supported the growth of Raoultella spp. and may possibly cause a potential amount of histamine to cause the illness from fish meat consumption. Our study indicated that at pH 5–6 under the optimal nutrient and temperature at 37°C, R. ornithinolytica and R. terrigena has ability to grow well.
Figure 1. Effect of pH, temperature and salt concentrations on R. ornithinolytica for 0 (□), 6(□), 18 (□) and 24 (▪) h; pH effect; A, temperature effect; C and salt effect; E and R. terrigena. pH effect; B, temperature effect; D and salt effect; F. Each value represents the mean of duplication (n = 4).
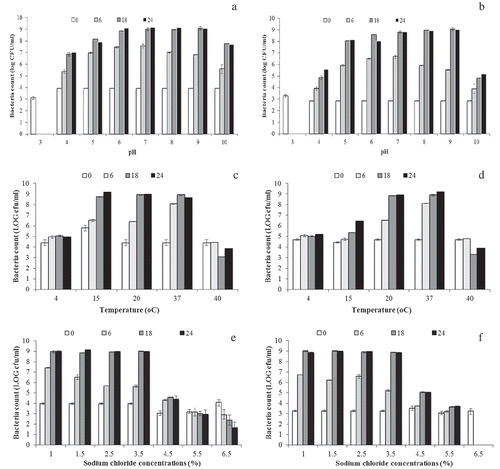
3.2.2. Effect of temperature
The effect of temperature showed considerbly impact on the growth of R. ornithinolytica and R. terrigena. Both species grew slowly at 4°C but grew rapidly at 20 and 37°C. Limit of growth was shown at 40°C. At refrigeration temperature, the growth of R. terrigena slightly increased at 15°C after 18 h compared to the growth of R. ornithinolytica which obviously grew well at 15°C. The different increasing population at low temperature indicated that R. ornithinolytica was quite psychrotrope while R. terrigena was trend to be mesophile. However at 37°C, the total population of both Raoultella spp was similar. At 4°C, the population remained constant from 6 to 24 h, cells can survive. While at 40°C the bacterial growth decreased after 6 h of incubation showed the heat sensitive in both species (Figure ). Therefore, using the warm water in cleaning could be useful during cleaning practices to get rid of these bacteria. Our study found that R. ornithinolytica and R. terrigena had limited growth rates at 4°C (0.02 ± 0.00 ∆log CFU/h), thus at refrigerated temperature (4°C) could inhibit the growth of Raoultella spp., to extend the shelf life of seafood and may prevent histamine production in rich histidine medium such as tuna meat.
Temperature is one of the important factors affecting the growth rate of bacteria in food (Lambert, Smith, & Dodds, Citation1991). Incubation temperatures in this study represented those that are employed in the production line of seafood and meat processed plant in Thailand; general control temperature in the processing area was at 20–25°C. The results demonstrated that both species were able to grow well at 20°C but grew rapidly at 37°C. At 37°C cultured in TSB (pH 6.7–7.4) was the best for growth temperature for Raoultella spp. with the growth rate of 0.55–0.56 ∆log CFU/h and this information was related to the body temperature and the cause of human illnesses. In comparison to the growth rate of Pseudomonas which is the common spoilage found in fish and seafood, P. marginalis in medium contained of pectin (4 g/L),yeast extract (2 g/L)-NH4Cl (1 g/L)-MgSO4(0.5 g/L) and other supplements (Membré & Burlot, Citation1994), the growth rate of P. marginalis at 30°C was 0.23 ∆log CFU/h while at low temperature as 4°C was 0.033 ∆log CFU/h, and at 18°C was 0.1 ∆log CFU/h. Another research work of Psuedomonas spp. in raw poultry (Dominguez & Schaffner, Citation2007), reported that at 15°C the growth rate (0.15 ∆log CFU/h) was slower than the growth at 20°C. (0.24 ∆log CFU/h). Hereby, the growth rate of R. ornithinolytica and R. terrigena in our study showed higher growth rate at 20°C (0.33 and 0.31 ∆log CFU/h, respectively) compare to Pseudomonas spp. growth. This may explain that Raoultella spp. are trend to be mesophile bacteria while Pseudomonas spp. are psychrotrophile bacteria, although both genus have the ability to grow differently at 15°C. The growth rate of R. ornithinolytica and R. terrigena in our study at 15°C were 0.16 and 0.08 ∆log CFU/h, respectively. The most rapid growth hereby, 0.61 ∆log CFU/h was observed in R. ornithinolytica at 37°C, while R. terrigena appeared to have a slightly different as 0.57 ∆log CFU/h.
Temperature condition can control the growth of Raoultella spp., therefore it will be useful to apply the prevention and control histamine production in risky seafood products particular histidine-rich seafood. Dalgaard and Emborg (Citation2009) reported at temperatures below 7–10°C, mesophilic and strongly histamine poisoning bacteria (HPB), such as Morganella and Raoultella, could not form histamine at toxic concentrations in fish products. Thus rapid chilling (below 7°C) and freezing are possible strategies to control the risk of histamine-producing bacteria and lower the risk in fish consumption caused by histamine. Later, Lin, Kung, Lin, Tsai, and Tsai (Citation2016) studied histamine production by R. ornithinolytica in canned tuna meat at different temperatures of 4, 15, 25, and 37°C. The histamine content quickly increased to more than 500 mg of histamine/kg stored at 37°C within 12 h, even if in the low inoculated samples (2 log CFU/g). At temperatures of 25–37°C within 12 h the rapid growth of this bacteria resulting in an increase in the histamine content to more than 2,000 mg of histamine/kg, whereas histamine formation was inhibited at 4°C. At a refrigerated temperature as 4°C some previous research found R. ornithinolytica isolated from fish was able to grow slowly at 4°C in TSB, but notably could produce histamine, though the amount was not specified (Kanki, Yoda, Tsukamoto, & Shibata, Citation2002). Therefore, the best control is to prevent the contamination into raw material before processing and implement a cleaning method to prevent the buildup of biofilm. In addition, keeping food at a low temperature below 4°C assist to inhibit the histamine formation by Raoultella spp.
3.2.3. Effect of sodium chloride
Sodium chloride has an effect on bacterial growth. R. ornithinolytica and R. terrigena could grow well in NaCl concentrations in the range 1–4.5% (Figure ). Their growth increased similarly when the NaCl concentrations increased 1% to 3.5% but were limited at NaCl over 3.5%. Both species had slow growth at 4.5–5.5% NaCl, and cells died off at 6.5% NaCl. R. terrigena exhibited less NaCl resistance compare to R. ornithinolytica, particular at 6.5%. The effects of the NaCl content on bacterial growth and survival in the range 4.5% downward are important as this corresponds to the salinity in many commercial ready-to-eat meals (Gudbjörnsdóttir et al., Citation2004). Many seafood products contain sodium chloride and may increase to more than 5.5%, at which level the survival of R. ornithinolytica and R. terrigena was clearly observed. In contrast, Dalgaard and Emborg (Citation2009) reported the concentrations of NaCl above 1–2% could reduce the growth of Gram-negative and strongly histamine-producing bacteria. The survey of histamine producing bacteria in dried mahi-mahi products in Taiwan by Lin et al. (Citation2014) exhibited the isolates of R. ornithinolytica from products containing NaCl range 0.6–20.1% (with an average NaCl content of 2.9%). When the particular isolate, R. ornithinolytica strain Lc22-2, was cultivated at the optimal NaCl concentrate on in TSB supplemented with 1.0% L-histidine, bacteria could grow and produce histamine (50 mg of histamine/100 g) even at a NaCl concentration of 5% after incubation at 35°C for 36 h. Thus, it is very possible that for processed fish containing 1–5% NaCl, if there is a chance of R. ornithinolytica contamination in fish during manufacturing, under the optimal conditions of temperature and time, cells could multiply and produce histamine. This condition may support the increase amounts up to hazardous levels. Notably the NaCl content in foods particularly seafood product had less effect on preventing and control histamine production from Raoultella spp. compare to other factors such as temperature and pH due to the limitation of consumer perception.
3.3. Heat resistance of R. ornithinolytica and R. terrigena
Comparing to the other genus within Enterobacteriaceae, R. ornithinolytica and R. terrigena exhibited the heat resistance higher than the other genus according to Denis, Cadot, Leguerinel, Thuault, and Sohier (Citation2005) (Table ). However, at 57°C cells died off rapidly compare to Enterobacter cloacae Aérial A3 and Serratia liquefaciens Anie 135. Between two species, D-values at 53°C, 55°C and 57°C of R. ornithinolytica were slightly higher than R. terrigena resulting in different Z-values. Z-value of R. ornithinolytica and of R. terrigena were 4.51 ± 0.58°C and 4.16 ± 0.54°C, respectively. The moderate thermal resistance properties of R. ornithinolytica and R. terrigena hereby indicated that bacteria could easily be destroyed by heat levels used in common cooking. Thus, food plant sanitation practices using the hot water at 80°C during cleaning and sanitizing could help to eliminate Raoultella spp. and to prevent biofilm formation particularly on food contact surfaces. According to Denis et al. (Citation2005), when the heat resistance of Klebsiella terrigena Anie 94 was determined at temperatures of 53°C and 54°C, Z-values revealed 4.54 ± 2.92°C, respectively, while the Z-value of Raoultella spp. ranged 4.16–4.51°C. The Z-value of Raoultella strains was closely to related species Klebsiella terrigena Anie 94, although the level of heat challenge, heating method, and heat determination methods were different. The difference may result from the capillary tube method applied by Denis et al. (Citation2005), while we used the tube method.
Table 2. D-values and Z-value for Enterobacteriaceae species.
3.4. Chemical treatment of R. ornithinolytica
In Good Manufacturing Practices, cleaning, and sanitizing procedure becomes the important part of the sanitation standard operating procedures and it need to strictly implement to assure a food safety programs. Improperly cleaned and sanitized surfaces allow harmful microorganisms to be transferred from one food to other foods (Fraser, Citation1998). Therefore, the process of disinfection or sanitization in a sanitation program needs to be designed appropriately to achieve both safety and quality of food. Choosing effective chemicals type is one of the key success, but there are many factors involved in their efficiency. The factors which control the efficiency of disinfectants include microbial type and growth condition, interfering substances, acidity-pH, temperature, contact time, and concentration (Bessems, Citation1998; Chmielewski & Frank, Citation2003).
The results of efficacy on microbial decontamination using different kinds of commercial chemicals are shown in Table . Cleaning A is a recommended chemical to use as a cleaning agent in our observed seafood processing plants. This chemical formula contains sodium hypochlorite at 4.6% (w/w) which acts as a sanitizer, combined with surface achieve agent lauryl dimethylamine oxide 10%, and alkaline cleaning agent as sodium hydroxide 5% to remove fat and protein. Hereby, when apply cleaning A at low concentrations (1–3 mg/L) could not kill R. ornithinolytica cells, higher concentration of 5 mg/L of cleaning A was needed to kill bacteria with an optimum contact time of 10 min. At this condition, the effectiveness was approximately 6 log reduction. Result was similar to applying sodium hypochlorite, at concentration of 0.5 mg/L for 5 min bacteria are completely killed, but at small amount 0.3 mg/L need longer contact time for 10 min. Ozone at 0.1–0.5 mg/L was very effective in inactivating R. ornithinolytica cells within 5 min of exposure. Ozone showed the highest efficacy in terms of killing R. ornithinolytica planktonic cells among the four tested chemicals. Ozone is claimed to have a faster inactivation mechanism than that of other sanitizers (Greene, Güzel-Seydim, & Seydim, Citation2012). The mechanism of ozone includes the disruption or lysis of the bacterial cell wall through the oxidation of the sulfhydryl groups and amino acids of enzymes; the degradation of the peptides and protein to smaller peptides; and lastly the oxidization of polyunsaturated fatty acids to acid peroxides. In 2001, the US Food and Drug Administration (FDA) approved ozone as an antimicrobial agent for direct food contact and allowed ozone to be used in food processing due to less concern regarding residual compounds and reaction byproducts (Greene et al., Citation2012). According to the requirements of the US Environmental Protecting Agency, ozonated water is allowed to use in water treatment at a maximum dose of ozone of 0.4 mg/L−and the contact time should last for 4 min. (Rice & Graham., Citation2001). In this study, Raoultella spp. planktonic cells was completely kill with ozone at low concentration (0.1 mg/L) for 5 min.
Table 3. Survival of R. ornithinolytica (log CFU/cm3) planktonic cells and biofilm cells after applying various chemical agents.
Sodium hypochlorite (NaClO) is used widely as a sanitizer in food industries. As a strong oxidizing agent, hypochlorite is known to be very active in killing most bacteria, fungi, and viruses and contributes to the destruction of enzymes, membranes and deoxyribonucleic acid (DNA) (Shikongo-Nambabi et al., Citation2012). However, in term of planktonic cells ozone exhibited a much higher efficacy in killing bacteria than sodium hypochlorite due to the higher degree of oxidizing power (mV) of ozone (Shikongo-Nambabi, Kachigunda, & Venter, Citation2010).
In case of biofilm cells inactivation, hydrogen peroxide (H2O2) was chosen to replace a cleaning A since the high efficiency of H2O2 on inactivation of biofilm was exhibited on the mixed strains biofilm of R. ornithinolytica, Pseudomonas aeruginasa and Listeria monocytogenes (Hoa et al., Citation2015). Hereby the initial population of R. ornithinolytica in biofilm on SS coupons ranged from 4.1–4.3 log CFU/cm2. These biofilms coupons were formed under stressed condition under low temperature (15°C), starvation (peptone water 0.3g/L) and dissication condition (16 hrs) to simulate the surface contact area in process seafood plant. Stressed cells were reported to be better resistance to chemicals and exhibited the cross-protection to other stresses (Giouris, Samoilis, Chorianopoulos, Ercolini, & Nychas, Citation2013) therefore stressed cells presented possible survival after cleaning. High reductions of Raoultella spp. biofilm on SS coupon was shown in Table , but the cleaning condition require harsh condition when applying chlorine (50 and 100 mg/L NaOCl), and H2O2 (1% and 2% v/v) for 5 and 10 min. In this study, NaOCl at lower concentration (25 mg/L) was tested during the preliminary test but less removal of Raoultella biofilm; however, the biofilm resistance is often linked to the biofilm matrix which is mainly composed of exopolysaccharides, proteins, nucleic acids and lipids (Flemming & Wingender, Citation2010). The complex components prevent chemical agent to penetrate deeper layers in biofilm (Chmielewski & Frank, Citation2003). At 50 and 100 mg/L required to diminish the Raoultella biofilm although the condition gave the unpleasant smell. Limited quantities of NaOCl for sanitation of clean equipment was noted at up to 100 mg/L, improper use of NaOCl can be ineffective or cause severe damage to equipment and injury to staff. (FAO and WHO, Citation2008).
In this study, ozone was found less effective on bacteria reduction when exposed to Raoultella spp. biofilm compared to planktonic cells at 1 mg/mL ozone. It can be explained that ozone in pure water rather quickly degrades to oxygen and even more rapidly in impure solution as biofilm conditions. Ozone was mentioned as less effective on biofilm (Gonçalves, Citation2009). According to Gezel-Seydim et al. (Citation2004) reported that 1-min ozonation had little effect against Pseudomonas fluorescens (ATCC 35030) and Bacillus licheniformis (ATCC 14580) when exposed to ozone (0.6 mg/L). The higher concentration of ozone was needed to employ in biofilm decontamination due to the complexity component of biofilm and the stress response of stressed cell.
Similarly, Tang, Flint, Bennett, and Brooks (Citation2010) studied the efficacy of ozonated water (0.5 mg/L) on ultrafiltration (UF) membranes from the dairy industry and found ozonated water was the weakest among these sanitizers tested. Lowest log reduction was recorded around 0.27 log CFU/cm2 when exposed to ozonated water 0.5 mg/L for 10 min. Thus, bacteria attached to surfaces could be reduced but not completely eradicated by using one single method or one single detergent or disinfectant (Jessen et al. Citation2003). In order to minimize biofilm-bound bacteria on processing equipment, the critical sites should be identified and paid full attention to during sanitation. The best biofilm elimination to focus on scrubbing the risk location but the limitation of accessibility of equipment therefor applying chemical becomes a choice of cleaning. The right choice and usage of cleaners and disinfectants, as well as an adequate sanitation program, must be thoroughly considered and designed.
4. Conclusion
In conclusion, Raoultella spp. could grow a wide range of temperatures (15–37°C), NaCl content (1–5.5%) and pH (4–9). pH had a little effect on the microbial growth parameters, when compared with the temperature and NaCl concentrations. R. ornithinolytica and R. terrigena could be destroyed under cooking temperature. In addition, common chemical agents using in food industry such as NaOCl reduced bacteria population effectively both on planktonic cell and biofilm. The complexity component of biofilm cause ozone was less effective, but H2O2 can be used as an alternative agent to remove biofilms in food processing equipment. The stress condition in the processing area resulting from stressed cells and the development of biofilms need to take into consideration when applying chemical agents on elimination Raoutella spp. Gaining knowledge on the extent of growth of R. ornithinolytica and R. terrigena against the growth parameters and chemical agents will be a useful information to prevent and control both in food process and cleaning protocol to prevent the possible cross contamination by Raoutella spp. to enhance the safety of seafood products.
Acknowledgements
This work was supported by the Office of the Higher Education Commission and the graduate scholarship from the National Research Council of Thailand (NRCT). The authors thank Nguyen Thi Quynh Anh for valuable assistance and Department of Food Science and Technology, Kasetsart University for providing instruments.
Additional information
Funding
Notes on contributors
Adisara Tantasuttikul
Adisara Tantasuttikul is a Ph.D. student in Food Science and Technology at Department of Food Science and Technology, Kasetsart University, Bangkok, Thailand. Her interest is quality and safety of seafood and hygienic practices in seafood process.
Warapa Mahakarnchanakul
Warapa Mahakarnchanakul is an assistant professor in the Department of Food Science and Technology at Kasetsart University, Bangkok, Thailand. Her Ph.D. is in Food Science from The University of Georgia, USA. Her currently research is the cleaning and sanitizing of fresh produce and seafood. Microbial stress response in food is also her research of interest.
References
- Bessems, E. (1998). The effect of practical conditions on the efficacy of disinfectants. International Biodeterioration and Biodegradation, 41, 177–14. doi:10.1016/S0964-8305(98)00022-5
- Chmielewski, R. A. N., & Frank, J. F. (2003). Biofilm formation and control infood processing facilities. Comprehensive Reviews in Food Science and Food Safety, 2, 22–32. doi:10.1111/crfs.2003.2.issue-1
- Chun, S., Yun, J. W., Huh, H. J., & Lee, N. Y. (2015). Clinical characteristics of Raoultella ornithinolytica bacteremia. Infection, 43, 59–64. doi:10.1007/s15010-014-0696-z
- Dalgaard, P., & Emborg, J. (2009). Histamine fish poisoning-new information to control a common seafood safety issue. In C. W. Blackburn & P. McClure (Eds.), Foodborne Pathogens: Hazards, risk analysis and control (pp. 1140–1160). Cambridge, UK: Woodhead Publishing Limited.
- Denis, C., Cadot, P., Leguerinel, I., Thuault, D., & Sohier, D. (2005). Heat resistance of coliform species isolated from cooked ham, snail flesh and ‘bouchées à la reine’. Letters in Applied Microbiology, 42, 160–164. doi:10.1111/j.1472-765X.2005.01838.x
- Dominguez, S. A., & Schaffner, D. W. (2007). Development and validation of a mathematical model to describe the growth of Pseudomonas spp. in raw poultry stored under aerobic conditions. International Journal of Food Microbiology, 120(3), 287–295. doi:10.1016/j.ijfoodmicro.2007.09.005
- Drancourt, M., Bollet, C., Carta, A., & Rousselier, P. (2001). Phylogenetic analyses of Klebsiella species delineate Klebsiella and Raoultella gen. nov., with description of Raoultella ornithinolytica comb. nov., Raoultella terrigena comb. nov. and Raoultella planticola comb. nov. International Journal of Systematic and Evolutionary Microbiology, 51, 925–932. doi:10.1099/00207713-51-3-925
- Dunne, W. M. (2002). Bacterial adhesion: Seen any good biofilms lately? Clinical Microbiology Reviews, 15(2), 155–166.
- European commission. (2017). Rapid alert system for food and feed. Retrieved from https://webgate.ec.europa.eu/rasffwindow/portal/?event=searchResultList.
- Feldhusen, F. (2000). The role of seafood in bacterial foodborne diseases Microbes and Infection, 2(13), 1651–1660. doi:10.1016/S1286-4579(00)01321-6
- Flemming, H. C., & Winngender, J. (2010). The biofilm matrix. Nature Reviews Microbiology, 8, 623–633. doi:10.1038/nrmicro2415
- Food and Drug Administration [FDA]. (1995). Bacteriological analytical manual. In J. R. Bryce & P. L. Poelma (Eds.), Microscopic examination of foods and care and use of the microscope (8th ed., pp. 2.01). Gaithersburg, MD.
- Fraser, A. M. (1998). Cleaning and sanitizing. Retrieved from www.foodsafetysite.com/resources/word/microbiology/FMC12Clening.doc.
- Gaithersburg, M. D. Food and Agriculture Organization of the United Nations & World Health Organization. (2008). Benefit and risks of the use of chlorine-containing disinfectants in food production and food processing. Report of a Joint FAO/WHO Expert Meeting (pp. 16–22).Ann Arbor, MI.
- Giouris, E., Samoilis, G., Chorianopoulos, N., Ercolini, D., & Nychas, G. J. (2013). Differential protein expression patterns between planktonic and biofilm cells of Salmonella enterica serovar Enteritidis PT4 on stainless steel surface. International Journal of Food Microbiology, 162, 105–113. doi:10.1016/j.ijfoodmicro.2012.12.023
- Gonçalves, A. A. (2009). Ozone: An emerging technology for the seafood industry. Brazillian Archives of Biology and Technology, 52, 1–26.
- Greene, A. K., Güzel-Seydim, Z. B., & Seydim, A. C. (2012). Chemical and physical properties of ozone. In C. O’Donnell, B. K. Tiwari, P. J. Cullen, & R. G. Rice (Eds.), Ozone in Food Processing (pp. 19–32). New Jersey, NY: Blackwell Publishing Ltd.
- Gudbjörnsdóttir, B., Suihko, M. L., Gustavsson, P., Thorkelsson, G., Salo, S., Sjöberg, A. M., Bredholt, S. (2004). The incidence of Listeria monocytogenes in meat, poultry and seafood plants in the Nordic countries. Food Microbiology, 21, 217–225. doi:10.1016/S0740-0020(03)00012-1
- Guzel-Seydim, Z. B., Greene, A. K., & Seydim, A. C. (2004). Use of ozone in the food industry. Lebensm.-Wiss.U.-Technol, 37, 453–460. doi:10.1016/j.lwt.2003.10.014
- Hoa, B. T. Q. (2014). Assessment of Microbial Adhesion to Processing Surface in Shrimp Processing Plant, Biofilm Formation and Control. Bangkok, Thailand: Kasetsart University.
- Hoa, B. T. Q., Mahakarnchanakul, W., Sajjaanantakul, T., & Kitpreechavanich, V. (2015). Adhesive microflora on stainless steel coupons in seafood processing plant. Journal of Food and Nutrition Sciences, 3(1–2), 28–32. doi:10.11648/j.jfns.s.2015030102.15
- Jessen, B., & Lammert, L. (2003). Biofilm and disinfection in meat processing plants. International Biodeterioration and Biodegradation, 51, 265–269. doi:10.1016/S0964-8305(03)00046-5
- Johansson, J., Mandin, P., Renzoni, A., Chiaruttini, C., Springer, M., & Cossart, P. (2002). An RNA thermosensor controls expression of virulence genes in Listeria monoctogenes. Cells, 110(5), 551–561. doi:10.1016/S0092-8674(02)00905-4
- Kanki, M., Yoda, T., Tsukamoto, T., & Shibata, T. (2002). Klebsiella pneumoniae produces no histamine: Raoultella planticola and Raoultella ornithinolytica strains are histamine producers. Applied and Environmental Microbiology, 68(7), 3462–3466. doi:10.1128/AEM.68.7.3462-3466.2002
- Koch, A. L. (1995). Bacterial growth. In Bacterial growth and form (pp. 37–87). NewYork, NY: Chapman & Hall, NY.
- Lambert, A. D., Smith, J. P., & Dodds, K. L. (1991). Shelf life extension and microbiological safety of fresh meat- a review. Food Microbiology, 8(4), 267–297. doi:10.1016/S0740-0020(05)80002-4
- Lim, E. S., Lee, J. E., Kim, J. S., & Koo, O. K. (2017). Isolation of indigenous bacteria from a cafeteria kitchen and their biofilm formation and disinfectant susceptibility. LWT Food Science and Technology, 77, 376–382. doi:10.1016/j.lwt.2016.11.060
- Lin, C. S., Kung, H. F., Lin, C. M., Tsai, H. C., & Tsai, Y. H. (2016). Histamine production by Raoultella ornithinolytica in mahi-mahi meat at various storage temperatures. Journal of Food and Drug Analysis, 24, 305–310. doi:10.1016/j.jfda.2014.06.010
- Lin, C.S, Tsai, H.C, Lin, C.M, Huang, C.Y, Kung, H.F, & Tsai, Y.H. (2014). Histamine content and histamine-forming bacteria in mahi-mahi (coryphaena hippurus) fillets and dried products. Food Control, 42, 165-171. doi:
- Membre, J.M, & Burlot, P.M. (1994). Effects of temperature, ph, and nacl on growth and pectinolytic activity of pseudomonas marginalis. Applied and Environmental Microbiology, 60, 2017-2022.
- Ministry of Commerce. (2018). Thai’s export quantity and value of canned tuna to the world. Retrieved from http://www.ops3.moc.go.th/infor/menucomth/stru1_export_market/report.asp.
- Morais, V. P., Daporta, M. T., Bao, A. F., Campello, M. G., & Andŕes, G. Q. (2009). Letters to the Editor: Enteric fever-like syndrome caused by Raoultella ornithinolytica (Klebsiella ornithinolytica). Journal of Clinical Microbiology, 47(3), 868–869. doi:10.1128/JCM.01709-08
- Møretrø, T., Moen, B., Heir, E., Hansen, A. A., & Langsrud, S. (2016). Contamination of salmon fillets and processing plants with spoilage bacteria. International Journal of Food Microbiology, 237, 98–108. doi:10.1016/j.ijfoodmicro.2016.08.016
- O’Connell, K., Kelly, J., & NiRiain, U. (2010). A rare case of soft-tissue infection caused by Raoultella planticola. Case Reports in Medicine, 1–2. doi:10.1155/2010/134086
- Park, S. H., Jarquin, R., Hanning, I., Almeida, G., & Ricke, S. C. (2011). Detection of Salmonella spp. survival and virulence in poultry feed by targeting the hilA gene. Journal of Applied Microbiology, 111(2), 426–432. doi:10.1111/jam.2011.111.issue-2
- Rice, R. G., & Graham., D. M. (2001). U.S. FDA regulatory approval of ozone as an antimicrobial agent –What is allowed and what needs to be understood. Ozone News, 29(5), 22–31.
- Saha, R., Bechanko, R., Bestervelt, L. L., & Donofrio, R. S. (2011). Evaluation of molecular techniques for identification and enumeration of Raoultella terrigena ATCC 33257 in water purifier efficacy testing. Journal of Industrial Microbiology and Biotechnology, 38, 1337–1344. doi:10.1007/s10295-010-0917-0
- Sandal, G., & Ozen, M. (2014). Fatal Raoultella ornithinolytica sepsis and purpura fluminans in a preterm newborn. Indian Journal of Paediatric Dermatology, 15(1), 24–26. doi:10.4103/2319-7250.131833
- Shikongo-Nambabi, M. N. N. N., Kachigunda, B., & Venter, S. N. (2010). Evaluatuion of oxidizing disinfectants to control Vibrio biofilms in treated seawater used for fish processing. Water Research Commission, 36(3), 215–220.
- Shikongo-Nambabi, M. N. N. N., Shoolongela, A., & Schneider, M. B. (2012). Control of bacterial contamination during marine fish processing. Journal of Biology and Life Science, 3(1), 1–17.
- Tang, X., Flint, S. H., Bennett, R. J., & Brooks, J. D. (2010). The efficacy of different cleaners and sanitizers in cleaning biofilm on UF membranes used in the dairy industry. Journal of Membrane Science, 352, 71–75. doi:10.1016/j.memsci.2010.01.063
- Tezel, B. U., & Şanhbaba, P. (2017). Science within food: Up-to-date advances on research and educationl. In A. Méndez-Vilas (Ed.), A major concern in food industry, contamination reservoir: Bacterial Biofilm (pp. 1–10). Badajoz, Spain: Formatex Research Center.
- Tsai, Y. H., Kung, H. F., Chen, H. C., Chang, S. C., Hsu, H. H., & Wei, C. I. (2007). Determination of histamine and histamine-forming bacteria in dried milkfish (Chanos chanos) implicated in a food-borne poisoning. Food Chemistry, 105(3), 1289–1296. doi:10.1016/j.foodchem.2007.03.018
- U. S. FDA. (U. S. Food and Drug Administration). (2001). Chapter 7. Scombrotoxin (histamine) formation. In Fish and Fishery products hazards and controls guide (3rd ed., pp. 73–93). Food and Drug Administration, Center for Food Safety and Applied Nutrition, Office of Seafoods, Washington DC.
- Yu, V. C. H., Yu, P. H. F., Ho, K. C., & Lee, F. W. F. (2011). Isolation and identification of new tetrodotoxin-producing bacteria species, Raoultella terrigena, from Hong Kong marine puffer fish (Takifugu niphobles). Marine Drugs, 9(11), 2384–2396. doi:10.3390/md9112384