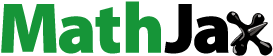
Abstract
Malaria is a parasitic disease that still prevails in the world and has been widely treated with the use of healing herbs. In this work isolation and extraction strategies have been implemented to get five molecules: 5,8-hydroxy-7-methoxyflavone (1), 6,7-dimethoxy-5,8-dihydroxyflavone (2), 6,7-dimethoxy-5-hydroxyflavone (mosloflavone) (3), 5,6-dihydroxy-7-methoxyflavone (negletine) (4), 5-Hydroxy-7-methoxyflavone (5) and to establish an analytical methodology for the chemical standardization of the different promising extracts from species Piper piedecuestanum. The antiplasmodial potential in vitro was validated on continuous cultures of P. falciparum chloroquine-sensitive strain NF-54 and cytotoxicity was tested in U-937 cell lines. Standardization parameters were checked with desirable acceptance criteria such as linearity, accuracy, precision, limits of detection and quantification. As a result, the best biological responses were achieved in extracts of P. piedecuestanum after 6 h of extraction with dichloromethane, presenting an extraction yield of 3.03 ± 0.306%, an antiplasmodial activity of IC50 = 24.7 ± 3.1 μg.mL−1, a cytotoxicity of CC50 = 17.17 ± 1.19 μg.mL−1, a selectivity index (SI) of 0.7 and a content of marker substances of CM5 = 7.8 ± 0.61, CM1 = 1.9 ± 0.02, CM3 = 4.6 ± 0.39, CM4 = 1.6 ± 0.14 mg.L−1. Compound (1) exhibited promising antiplasmodial activity with an IC50 = 7.33 μg.mL−1 (25.7 μM). The development of this new analytical method and the biological standardization for extracts of P. piedecuestanum enable fulfills the biological specifications and to evaluate future pharmacological studies the therapeutic efficacy in murine models with the most promising.
PUBLIC INTEREST STATEMENT
Traditional medicine uses the Piper piedecuestanum plant for the treatment of malaria. This study presents the strategies of preparation of standardized extracts of the plant and the isolation of some molecules to evaluate their activity against malaria parasites (antiplasmodial).
An analytical methodology is established for the chemical standardization of extracts to determine the presence of each of their ingredients or marker substances and their quantity, in such a way as to guarantee the reproducibility of the method.
The study evaluated antiplasmodial activity in a strain of P. falciparum and its cytotoxic activity in cell lines. A promising antiplasmodial and non-toxic activity is published, which can be evaluated in future studies.
Competing Interests
The authors declare no competing interests.
1. Introduction
Malaria is an infectious disease that shows the highest morbidity among diseases of this type and is the third in the world in cases of death by infection, after tuberculosis and HIV/AIDS infection (Olumese, Citation2015). Malaria is caused by protozoan parasites belonging to the family Plasmodiidae, genus Plasmodium, which is transmitted by female mosquitoes of the genus Anopheles (WHO, Citation2017). This disease still prevails in the world and continues to be one of the leading causes of death worldwide. Around 3.2 billion people, about half of the world’s population, are exposed to malaria (WHO, Citation2016). Malaria has been extensively treated with the use of healing herbs, which requires an explanation for their effects upon scientific basis. Well-known antimalarial agents, such as quinine (from Chincona sp.) and artemisinin (from Artemisia annua), were used in traditional medicine (WHO, Citation2015).
The world market for medicinal plants’ extracts is currently estimated to be around $75 mn./per year (Hoareau & DaSilva, Citation1999; Tanae, Lima-Landman, de Lima, Soucca, & Lapa, Citation2007). The great challenge in the development of a standardized extract is the analytical method, which consists of analyzing one or several marker substances in a complex matrix and selecting an optimal and reproducible extraction method (Hostettmann, Gupta, Marston, & Ferreira, Citation2008). Due to the large number of required parameters, it is recommended for the analytical method to be simple, fast, reproducible and friendly to the environment (Braun, et al. Citation2001).
The Piper genus is of great commercial and economic importance for the condiment, pharmaceutical and insecticide industries and has been widely used in traditional food and also in the treatment of various diseases (Albiero, de Souza, Mourao, de Almeida, & Lopes, Citation2005). In traditional medicine, the Piper genus is widely used to treat diseases such as malaria, anemia, cholera, diabetes, asthma, bronchitis, pneumonia, influenza, rheumatism and arthritis (D’Angelo, Xavier, Torres, Lapa, & Souccar, Citation1997). The study of Piper genus is of great importance since it allows finding new structural templates and evaluating their activity as potential antimalarials. Chemically, the most common constituents reported of this genus are prenylated benzoic acids, propenylphenols, lignans, neolignans, terpenes, flavonoids, kavalactones, epoxides and alkaloids, such as isobutylamine, piperidine and pyrrolidine among others (Parmar et al., Citation1997). Chemotaxonomy of Piper has been analyzed for alkaloids (isoquinoline group), volatile chemical profiles and flavonoids, which has allowed the researches to conclude for this genre that delimitation and taxonomical relationships arrive by conventional taxonomy using morphological characters and not by Chemotaxonomy (Ravindran & Nirmal, Citation1994). Specific chemotaxonomic markers have been identified for Piper species, and the biosynthetic pathways of the major compounds have been reported (Ravindran & Babu, Citation1994). This study is the first chemical and biological report about extraction strategy and quantification of marking substances of P. piedecuestanum. In addition, this report also provides for the first time a new analytical methodology for the chemical standardization of the different extracts from P. piedecuestanum. The antiplasmodial activity was evaluated in continuous cultures of P. falciparum chloroquine-sensitive strain NF-54 and cytotoxicity was tested in U-937 cell lines to select which extract meets the best in vitro specifications to be evaluated in the future studies about therapeutic efficacy.
2. Material and methods
2.1. General experimental procedures
IR spectra were obtained by using KBr pellets on a Jasco FT/IR-6200 spectrometer, spanning the region 4000–600 cm−1. Mass spectra were measured on a Q-TOF mass spectrometer (Waters, Manchester, UK) with electrospray-type combined ionization source and Z-spray design APCI; the capillary voltage of 3.5 KV was used in the positive direction and the cone voltage was set at 20 V. NMR spectra were recorded on Varian Unity spectrometers operating frequencies, 300 and 500 MHz for 1H, 125 and 75 MHz for 13C. The spectra were measured in CDCl3 solution. Chemical shifts (δ) are reported in ppm using the residual solvent signals (δ 7.27 ppm for 1H and 77.0 ppm for 13C) as a reference. Instruments of the analytical method: The standardization of the analytical method and the quantification were carried out in a high-efficiency liquid chromatography equipment coupled to a diode array detector (High-Performance Liquid Chromatography with Diode-Array Detection HPLC-DAD Agilent Technology 1200 series). The chromatography system consisted of a quaternary pump with degasser G1354A, autosampler G1313A, column compartment G1316A, detector UV/Visible with diode array and a ChemStation Software 32bit G2170BA HPLC 2D. The column used for the chromatographic separation was a Luna Phenomenex with a particle size of 5 μ, C18 100Å and dimensions of 250 mm × 4.60 mm, series 564054–31.
2.2. Plant material
Three types of samples were collected from the plant material: a sample for the specimen of herbarium, samples as witnesses of the collected specimens and samples of leaves and stems to obtain the extracts. The herbarium specimen collected in Piedecuesta (Santander, Colombia) was processed, deposited and taxonomically characterized in the herbarium of the Universidad de Antioquia (HUA), and determined as Piper piedecuestanum Trel. & Yunck. (Voucher 164.502).
2.3. Preparation of standardized extracts and isolation of compounds
The plant material of P. piedecuestanum, (0.36 Kg) was washed and dried in shade for a period of 10 days and was reduced to 5 mm particle size. To guarantee an optimal, reproducible and fast extraction process, extractions were carried out taking into account the extracts with promising biological results. The plant extracts were obtained by percolation and encoded as extracts of petroleum ether (PE) (PPHTPE), dichloromethane (D) (PPHTD), ethyl acetate (AE) (PPHTAE), and ethanol (E) (PPHTE). Other extracts were obtained by Soxhlet extraction by triplicate with 20 g of plant material (6 h and 4 h extraction times). Pure solvents and mixtures thereof, such as ethyl acetate (EtOAc), dichloromethane (DCM), dichloromethane-methanol (DCM-MeOH 1:1), dichloromethane-ethyl acetate (DCM-EtOAc 1:1) encoded as (PPDS4, PPAS4, PPDMS4, PPDS6, PPAS6, PPDMS6) were used. Subsequently, all extracts were filtered and concentrated in a rotoevaporator. The dry residue was weighted to obtain the extraction yields according to Equation 1. All the extracts were monitored (1 mg in 50 μL of EtOAc for sowing) by thin layer chromatography (CCD) supported with Silica-gel 60 GF254 Merck® using different elution systems such as petroleum ether (PE), dichloromethane (DCM), Ethyl acetate (EtOAc), dichloromethane: methanol (DCM: MeOH 95: 5) and dichloromethane: methanol (DCM: MeOH 9:1). The plates (CCD) were visualized by a UV lamp (258 nm) and by using universal developer (20% sulfuric acid) or with ethanolic solution of 5% sulfuric acid in ethanol and 1% vanillin to detect the presence of flavonoids and to determine the established metabolites as standards.
In all the extracts the presence of flavonoids was evidenced. The dichloromethane extract (PPD) from P. piedecuestanum Trel. & Yunck. (4.89 g) was fractionated by column chromatography using gradients of petroleum ether: ethyl acetate, EtOAc and MeOH as eluents. Thirty fractions were obtained from which fraction 10 was taken and column chromatography was performed using petroleum ether, petroleum ether: DCM (1: 1), DCM, gradients of DCM: EtOAc and finally MeOH as eluent. Twenty-three fractions were obtained which were pooled between 1 and 7. Preparative plate chromatography was performed using DCM as the eluent which allowed to isolate 20 mg of the compound designated as (4). On the other hand, section 25 of the dichloromethane extract column was submitted to preparative layer chromatography allowing the isolation of two compounds. One of them was a yellowish amorphous solid named (2) (31.8 mg) and the other was a crystalline yellowish amorphous solid referred to as (1) (183.9 mg). The remaining fractions of the petroleum ether extract and dichloromethane were combined to perform column chromatography again using petroleum ether gradients: DCM, DCM: EtOAc, EtOAc: Methanol (MeOH) gradients and finally MeOH, to obtain 14 fractions. Fractions 6–10 were taken and submitted to column chromatography using eluent petroleum ether, petroleum ether: DCM (1: 1), DCM and DCM: MeOH gradients as eluents giving rise to 30 fractions. Fractions 1–9 and 15–17 were taken for preparative layer chromatography. Eluting with petroleum ether: DCM 25: 3 and DCM allowed the isolation of compound (4) (39 mg), compound (3) (59 mg) and compound (5) (58 mg). The chemical structures corresponding to flavonoids isolated and then evaluated for their antiplasmodial and cytotoxic potential was followed according to (Mesa et al., Citation2018).
2.4. Structure elucidation
2.4.1 5, 8-Hydroxy-7-methoxyflavone (1) Yellowish amorphous solid. C16H12O5. TOF MS ES+ [M + H]: 285.0760.1H-NMR-300 MHz δ (CDCl3): 12.50 (1H, s, C5-OH), 7.89 (2H, dd, J= 2.2, 8.0 Hz, Ar-H), 7.56–7.52 (3H, m, Ar-H), 6.69 (1H, s, C6-H), 6.63 (1H, s, C3-H), 4.018 (3H, s, CH3O).13C-NMR-75 MHz δ (CDCl3): 183.06, 165.59, 162.15, 153.33, 146.04, 132.19, 131.88, 130.048, 129.49 (CH x 2), 126.67 (CH x 2), 105.91, 105.85, 90.95, 56.88 (Shin, Kim, Kim, Jeong, & Kim, Citation1999).
2.4.2 6, 7-dimethoxy-5, 8-dihydroxyflavone (2) Yellowish amorphous solid. C17H14O5. TOF MS ES+ [M+ OH]: 315.0869. 1H-NMR-300 MHz δ (CDCl3): 12.63 (1H, s, C5-OH), 8,20 (2H, dd, J= 2.2, 8.0 Hz, Ar-H), 7.53–7.51 (3H, m, Ar-H), 6.82 (1H, bs, C8-OH), 6.56 (1H, s, C3-H), 3.98 (3H, s, CH3O), 3.94 (3H, s, CH3O). 13C-NMR-75 MHz δ (CDCl3): 197.36, 174.85, 159.77, 152.88, 151.92, 136.96, 132.52, 130.72, 129.04 (CH x 2), 128.03 (CH x 2), 96.95, 91.36, 86.65, 61.37, 56.80 (Sawasdee, Sabphon, Sitthiwongwanit, & Kokpol, Citation2009).
2.4.3 6,7-dimethoxy-5-hydroxyflavone (mosloflavone) (3) Yellowish amorphous solid. C17H14O5. TOF MS ES+ [M + H]: 299.0919.1H-NMR-500 MHz δ (CDCl3): 12.68 (1H, s, C5-OH), 7.88 (2H, dd, J= 2.2, 8.0 Hz, Ar-H), 7.54–7.52 (3H, m, Ar-H), 6.66 (1H, s, C3-H), 6.56 (1H, s, C8-H), 3.97 (3H, s, CH3O), 3.93 (3H, s, CH3O). 13C-NMR-125 MHz δ (CDCl3): 182.66, 163.90, 158.87, 153.27, 152.99, 132.66, 131.80, 131.25, 129.05 (CH x 2), 126.20 (CH x 2), 106.24, 105.56, 90.62, 60.80, 56.29 (Righi et al., Citation2010).
2.4.4 5,6-dihydroxy-7-methoxyflavone (negletein) (4) Yellowish amorphous solid. C16H12O5. TOF MS ES+ [M + H]: 285.0767.1H-NMR-300 MHz δ (CDCl3): 11.72 (1H, bs, C5-OH), 8.20 (2H, dd, J= 2.2, 8.0 Hz, Ar-H), 7.53–7.48 (3H, m, Ar-H), 6.51 (1H, s, C3-H), 6.39 (1H, s, C8-H), 3.90 (3H, s, CH3O). 13C-NMR-75 MHz δ (CDCl3): 194.62, 166.54, 166.0, 161.55, 145.36, 131.97, 130.314, 129.49, 129.46(CH x 2), 129.08(CH x 2), 114.04, 104.95, 94.04, 57.60 (Shin et al., Citation1999).
2.4.5 5-hydroxy-7-methoxyflavone (5) Yellowish amorphous solid. C17H14O5. TOF MS ES+ [M + H]: 269.0810.1H-NMR-500 MHz δ (CDCl3): 12.72 (1H, s, C5-OH), 7.88 (2H, dd, J= 2.2, 8.0 Hz, Ar-H), 7.53–7.52 (3H, m, Ar-H), 6.67 (1H, s, C3-H), 6.50 (1H, s, C6-H), 6.37 (1H, s, C8-H), 3.88 (3H, s, CH3O). 13C-NMR-125 MHz δ (CDCl3): 182.46, 165.59, 162.15, 157.76, 131.80, 131.28 (CH x 2), 129.05 (CH x 2), 126.64, 105.83, 105.67, 98.17, 92.65, 55.78 (Sawasdee et al., Citation2009).
All extracts and/or compounds were stored at room temperature for the biological assays in vitro of antiplasmodial activity in chloroquine-sensitive Plasmodium falciparum strain NF54 and cytotoxicity in human promonocytes U-937.
2.5. Chemical analyses of the marking substances
Water (A) and acetonitrile (B) of HPLC grade were used as solvents. The injection volume used was 20μL with a flow of 1.0 mL.min−1 for six times. For the quantification, standard curves prepared with five concentrations of marker substance (1000–0.1 mg.L−1), each one in triplicate. The extracts were prepared at a concentration of 3000 mg.L−1. The standards and extracts were dissolved in an acetonitrile-water solution (1:1) and filtered before the injection by Nylon membrane 0.45 μm (hydrophilic) DISMIC-13 NP; 0.39 MPa. ADVANTEC for HPLC analysis. The concentration in mg/mL of marker substance was determined by area/ratio analysis. After stabilizing the system, the following parameters were verified and validated: Resolution: >2.0 (peak of interest and adjacent peak), Queue factor: <2.0 (peak of interest) and % RSD: ≤3.5%, analyses of calibration curve, linearity, range, limit of detection (LOD) and limit of quantification (LOQ) of the analytical method. For the identification of marker substances (1–5) in the different extracts obtained by percolation and Soxhlet a new method is proposed using as a mobile phase water (solvent A) and acetonitrile (solvent B) and mixtures thereof (from 60% of A and 40% of B to 0% of A and 100% of B) at a flow of 1.0 mL/min for 12 min. Under these conditions, the system remains constant after 3 min. The total time of the analysis was 15 min. The reading was carried out at a wavelength of λ = 360 nm. Marker substances were identified by comparing their retention times (tR) under identical analytical conditions.
2.6. Biological activity test
2.6.1. In vitro determination of antiplasmodial activity
In vitro antiplasmodial activity assays were performed on the sensitive (NF-54) chloroquine strain. P. falciparum strains (NF-54) were cultured and maintained according to the method of Trager and Jensen (Citation1976) (30); using a suspension of 5% human A + erythrocytes in RPMI-1640 culture medium (Sigma R6504) dissolved in sterile water with 25 mM HEPES, 5.0% NaHCO3, 10% fresh human A + serum (inactivated at 56° C for 30 min) incubated in 5% O2, 5% CO2 and 90% N2 an atmosphere. Fresh red blood cells were added twice a week. In vitro antiplasmodial activity by the SYBR Green, I® method was performed in the Malaria Group, according to the methodology described by Smilkstein (Smilkstein, Sriwilaijaroen, Kelly, Wilairat, & Riscoe, Citation2004). Assays were performed on Falcon® 96-well flat bottom plates. A suspension of parasitized red blood cells with a hematocrit of 2.5% and a parasitemia of 1% were prepared. Cultivation with treatments and chloroquine (CQ) positive control were incubated at 37°C for 48 h in 5% CO2, 5% O2 and 90% of N2 atmosphere. Subsequently, the contents of each well were transferred to Greiner Pro one dishes, and the parasites were labeled with a solution of SYBR® Green I 2X in lysis buffer. The plates were incubated at room temperature in the dark for 1 h, and the relative fluorescence units (RFU) were read on a spectrofluorometer at 485 nm excitation wavelength and 538 nm emission wavelength. Treatments from each crude extract were prepared to a stock solution of 10 mg.mL−1 in pure DMSO and sonicated to facilitate dissolution. From this solution, 50 μL were taken and adjusted to 1000 μL with complete RPMI-1640 medium, obtaining a final concentration of 0.5 µg.mL−1. The concentration of DMSO in the first dilution was 1% and showed to be non-toxic to the parasite. Seven concentrations of each extract were evaluated in a range between 100 and 1.56 μg.mL−1. Each concentration was evaluated in triplicate on the plate and three independent assays were performed. The CQ control was evaluated in a range between 150 and 4.7 nM and the control of Cinchona officinalis, Peruvian quinoa extract (MeOH: H2O; 70:30) was evaluated in the range of 0.01–10 μg.mL−1. Data from three trials were analyzed to find the inhibitory concentration in μg.mL−1 (IC50). Inhibitory concentrations 50 (IC50 ± SD) were calculated for each compound from a non-linear logistic regression model. A sigmoid concentration–response curve with a slope of Hill (variable slope) was assumed. The data were analyzed and plotted using GraphPad Prism 4 for Macintosh version 4.0b which outputs the adjustment value (r) (GraphPad Software, San Diego, California, USA). To classify the antiplasmodial activity of an extract, the Malaria Group of the University of Antioquia established a consensus for the extracts evaluated: highly active <5 μg.mL−1, promising 6–15 μg.mL−1, moderate activity 16–30 μg.mL−1, low activity 31–50 μg.mL−1 and non-active>50 μg.mL−1. To classify the antiplasmodial activity of a compound, the Malaria Group estimated that a compound is promising if the IC50 is ≤10 μM (Fidock, Rosenthal, Croft, Brun, & Nwaka., Citation2004; Jonville et al., Citation2008; Malebo et al., Citation2009; Muñoz et al., Citation2000; Philippe et al., Citation2005).
2.6.2. Cytotoxicity testing and calculation of the selectivity index (SI)
The method of 3-(4, 5-dimethylthiazol-2-yl)-2, 5-diphenyl tetrazolium bromide (MTT) according to Mosmann, which reveals cellular damage at the mitochondrial level, was used to evaluate the cytotoxic activity of the extracts. U-937 cells are human histiocytic lymphoma promonocytes, which were maintained in continuous cultures in Malaria Group Laboratory (Moore, Gerner, & Franklin, Citation1967; Mosmann, Citation1983). These cells were cultured at 37°C and 5% CO2 in RPMI medium supplemented with 10% inactivated Fetal Bovine Serum (FBS). The media changes were assessed every 48 h or according to pH changes of the medium, with 10-min centrifugation at 1000 rpm and by replacement with fresh medium. In Neubauer’s chamber, U-937 cells were counted and plated in a 96-well flat bottom plate, 200,000 cells.mL−1 in RPMI 1640 medium with 10% FBS. They were incubated at 37°C with 5% CO2 for 72 h in the presence of each of the seven concentrations of each extract and/or compound and evaluated in a range between 100 −1.56 μg.mL−1. Each concentration was evaluated in triplicate in the dish and three independent trials were performed (15). Subsequently, mitochondrial dehydrogenase activity was measured by adding 20 μL by each well of MTT to a concentration of 5 mg.mL−1 and incubated for 3 h at 37°C under 5% CO2. To dissolve the formed crystals, each well of 100 uL were added a 50% solution of isopropanol and 10% SDS and the absorbance was read at 595 nm by an ELISA reader (BioRad). Data from three independent trials were analyzed using the GraphPad Prism 5 program to find the toxic concentration in μg.mL−1 (CC50) using a non-linear logistic regression model. To classify the cytotoxicity of the extract, the Malaria Group of the University of Antioquia established a consensus for the samples evaluated: highly toxic <10 μg.mL−1, cytotoxic 10–40 μg.mL−1, moderately cytotoxic 41–100 μg.mL−1 and no cytotoxic>100 μg.mL−1. In addition, the selectivity index (SI), which indicates selectivity towards the parasite, was calculated as the relationship between cytotoxic CC50 activity and antiplasmodial activity IC50 (Bautista, Acosta, & Toledo, Citation2000; Koch, Tamez, Pezzuto, & Soejarto, Citation2005; Mosmann, Citation1983; Reed, Lynn, & Meade, Citation2002).
2.6.3. Statistical analysis
Measurements were performed in triplicate, and the results are presented as the mean and its standard deviation (SD). The data were analyzed and plotted using GraphPad Prism 4 for Macintosh version 4.0b which outputs the adjustment value (r) (GraphPad Software, San Diego, California, USA).
3. Results
The percentages of extractable material, structurally characterization of flavonoids, the content in mg.mL−1 of the marker compounds, the antiplasmodial in vitro activity in P. falciparum strain sensitive to chloroquine NF-54, the cytotoxicity in human promonocytes U-937 and the determination of the selectivity index from the extracts obtained from P. piedecuestanum are presented in Tables and . Chromatographic profiles of the extracts obtained from the aerial parts of P. piedecuestanum are plotted in Figure . All the extracts obtained by Soxhlet extractions were compared with the most active extracts obtained by percolation and were classified according to the antiplasmodial and cytotoxic potential established by the Malaria Group of the University of Antioquia.
Table 1. Extraction and mg/L of the marker compounds in extracts prepared from the aerial parts P.piedecuestanum.
Table 2. Antiplasmodial activity of isolated compounds of P. piedecuestanum
Six types of extracts from P. piedecuestanum species, each in triplicate, were obtained. Five marker substances coded as (1–5) were quantified. The percentages of material extractable with the different solvents showed better yields for the standardized extracts obtained by Soxhlet in dichloromethane/methanol (DCM: MeOH 1: 1) at 4 h (PPDMS4, 5.5 ± 0.32 yield) and at 6 h (PPDMS4, 5.3 ± 0.53 yield). However, these extracts were the least active showing an IC50 >50 μg.mL−1. Marking substance (5) that was in greater proportion in all the extracts was the inactive one.
Analyzing all the variables together allows to draw the following conclusions: (a) the most suitable solvents for extraction were dichloromethane and ethyl acetate; (b) the standardized extract with the best biological response was obtained after 6 h of extraction with dichloromethane (PPDS6), with 3.0 ± 0.31 yield, an antiplasmodial activity of IC50 = 24.7 ± 3.1 μg.mL−1, a cytotoxicity of CC50 = 17.17 ± 1.19 μg.mL−1 IS = 0.69 and a content of marker substances of CM5 = 7.8 ± 0.61, CM1 = 1,9 ± 0,02, CM3 = 4.6 ± 0.39, CM4 = 1.6 ± 0.14 μg.mL−1. However, although this extract has the best effect, it was expected that the selectivity indexes were above 2, which implies that this extract has high cytotoxicity. In this sense, the best form of extraction was that made by percolation with active and nontoxic extracts, although the extraction times were longer. Possibly a treatment after the extraction by Soxhlet, and the removal of tannins by means of precipitation with a solution of gelatine and NaCl as a second extraction step, could favor the decrease of the cytotoxicity of the standardized extract.
The extracts of P. piedecuestanum were analyzed to perform their quantification in (mg.mL−1). The separation conditions for the extracts were validated and optimized using a high-efficiency liquid chromatography equipment coupled to a detector with the diode array. Standardization parameters were checked with desirable acceptance criteria such as linearity (r2 = 0.99), accuracy (R = 88.25–102.82%), precision (VC = 0.45% to 4.87%), limits of detection and quantification (0.09 to 13.65 mg.L−1). This chromatographic method allowed us to resolve, identify and quantify four flavones (Figure ). The mobile phase resolved the four flavones with a higher separation efficiency, and a good repeatability at retention times of tR = 7,589 for (5); tR = 7,897 for (1); tR = 10,078 for (4); tR = 11.098 for (3), with a total elution time of 15 min. An example of the chromatographic profiles is displayed in Figure , which shows: (A) the dichloromethane extract, (B) the ethyl acetate extract, both obtained by percolation extraction and (C) the extract obtained by Soxhlet extraction in dichloromethane with an extraction time of 6 h and an average yield of 3.0 ± 0.31.
4. Discussion
The use of standardized extracts from plants that have weaker or less specific pharmacological activities is justified when the active ingredients are not yet known. However, in order to guarantee constant therapeutic quality and efficacy, it is important to standardize these extracts for their content of active ingredients. If these are unknown, those compounds most representative of the chemical composition of the extract are selected. In this sense, we have carried out the chemical standardization of extracts of the P. piedecuestanum species with the five flavonoid-type substances previously isolated and characterized. A variety of standardized extracts are the subject of research, such as the species Mitragyna speciosa Korth (Ketum), which is widely used in Malaysia as a medicinal agent for the treatment of diarrhea, infections, as an analgesic and antipyretic, to determine the acute toxicity in Sprague–Dawley mice of the standardized methanol extract obtained from 100 g of leaves of Mitragyna speciosa Korth by means of Soxhlet extraction in methanol (100% v/v MeOH) for 4 h at 60°C and in which the presence of the alkaloid mitragin was determined to be 1.6% upon analysis by GC-MS (Jachak et al., Citation2011). Likewise, Sanjay M et al., standardized the extracts in EtOAc, MeOH and EtOH 70% from the leaves of Tridax procumbens with potential anti-inflammatory and antioxidant activity, and significant inhibition of cyclooxygenase in rats at an average dose of 200 mg.kg−1, being the EtOAc extract being the most active (Jang, Kim, & Park, Citation2005). The extracts were standardized by HPLC with the help of chemical markers of flavonoid type such as centaurein and bergenine (Harizala, Mansor, Hasnan, Tharakan, & Abdullah, Citation2010). On the other hand, the process of standardization of the aqueous extract (AE) of Cecropia glaziovii was carried out in order to assess its pharmacological characterization as phytomedicine. To standardize the plant, dried leaves were taken and prepared with distilled water at 72°C, then filtered and lyophilized with a yield of 20% (Tanae et al., Citation2007). Then, a partition was made with n-butanol, obtaining the butanolic fraction with a yield of 1%. The activity of the AE in function of the vital organs (cardiovascular, respiratory, gastrointestinal and central nervous system) was determined in vivo. The markers were isolated, purified and identified as catechins, procyanidins, flavonoids, mixed sugars and chlorogenic acid. Their relative concentrations in the EA were 12% of catechins, 19% of procyanidins and 19% of flavonoids (Sutthanutm, Sripanidkulchai, Yenjai, & Jay, Citation2007).
In conclusion, a new analytical methodology which can be used to analyze the marker substance and control the variation of its therapeutic effect in batches of finished products from plant material was established for the analysis of five marker substances in standardized extracts obtained from P. piedecuestanum. The extraction method was optimal, and the analytical method by HPLC was simple, fast, reproducible and friendly to the environment for the quantification of one or several marker substances present in the extracts. The in vitro antiplasmodial potential was evaluated on continuous cultures of P. falciparum chloroquine-sensitive strain NF-54 and cytotoxicity in cell lines U-937. In this study development of this new analytical method and the biological standardization for extracts of P. piedecuestanum enable fulfills the specifications biological to level in vitro and future to evaluated pharmacological studies and the therapeutic efficacy in murine models with the best extract from the P. piedecuestanum.
Conflict of interest
The authors declare no conflict of interest. The authors have no competing interests to declare.
Acknowledgements
The authors are grateful for the financial support provided by the Colombian Ministry of Agriculture (No. 009-2007-V7552-38-07), Jhon Fredy Toro for Isolation of the molecules. Colciencias through the José de Caldas franchises and the University of Antioquia.
Additional information
Funding
Notes on contributors
Ana Maria Mesa Vanegas
Ana Maria Mesa Vanegas Extensive experience in research on the use of biodiversity, emphasis on natural products, biological activity and biotechnology applied to agricultural systems. The research group currently working to in the study of the plants, obtaining extracts, bioactive molecules and the biological evaluation in different cellular models of pathogens and phytopathogens, experience in the structural characterization of molecules and macromolecules isolated from plants by instrumental techniques, HPLC, HPLC-MS, GC-MS, NMR, standardization of in vitro and in vivo biological evaluation techniques, experience in industry and academia leading research processes, formulating, executing and developing research projects and technological innovation, leading a research group.
References
- Albiero, A. L. M., de Souza, L. A., Mourao, K. S. M., de Almeida, O. J. G., & Lopes, W. A. L. (2005). Morphology and anatomy of stem and leaf of Piper gaudichaudianum Kuntze (Piperaceae). Acta Farmacéutica Bonaerense, 24(4), 550–554.
- Bautista, C., Acosta, E., & Toledo, I. (2000). Evaluación del bioensayo del MTT Para determinarla proliferación in vitro de linfocitos de bovino frescos y congelados. Veterinart Hospital of Mexico, 31(2), 101–12.
- Braun, J. M., Ko, H. L., Schierholz, J. M., Weir, D., Blackwell, C. C., & Beuth, J. (2001). Application of standardized mistletoe extracts augment immune response and down regulates metastatic organ colonization in murine models. Cancer Letters, 170(1), 25–31.
- D’Angelo, L. C. A., Xavier, H. S., Torres, L. M. B., Lapa, A. J., & Souccar, C. (1997). Pharmacology of Piper marginatum Jacq. a folk medicinal plant used as an analgesic, antinflammatory and hemostatic. Phytomedicine, 4(1), 33–40.
- Fidock, D. A., Rosenthal, P. J., Croft, S. L., Brun, R., & Nwaka., S. (2004). Antimalarial drug discovery: Efficacy models for compound screening. Nature Reviews Drug Discovery, 3(6), 509–520. doi:10.1038/nrd1416
- Harizala, S. N., Mansor, S. M., Hasnan, J., Tharakan, J. K. J., & Abdullah, J. (2010). Acute toxicity study of the standardized methanolic extract of Mitragyna speciosa Korth in Rodent. Journal of Ethnopharmacology, 131(2), 404–409. doi:10.1016/j.jep.2010.07.013
- Hoareau, L., & DaSilva, E. J. (1999). Medicinal plants: A re-emerging health aid. Electronic Journal of Biotechnology, 2(2), 3–4.
- Hostettmann, K., Gupta, M. P., Marston, A., & Queiroz, E. F. (2008). Manual de estrategias para el aislamiento de productos naturales bioactivos. Programa iberoamericano de Ciencia y Tecnología. CYTED.
- Jachak, S. M., Gautam, R., Selvam, C., Madham, H., Srivastava, A., & Khan, T. (2011). Anti-inflammatory, cyclooxygenase inhibitory and antioxidant activities of standardized extracts of Tridax procumbens L. Fitoterapia, 82(2), 173–177. doi:10.1016/j.fitote.2010.08.016
- Jang, J., Kim, H. P., & Park, H. (2005). Structure and antiinflammtory activity relationships of wogonin derivates. Archives of Pharmacal Research, 28(8), 887–884. doi:10.1007/BF02973870
- Jonville, M. C., Kodja, H., Humeau, L., Fournel, J., De Mol, P., Cao, M. (2008). Screening of medicinal plants from Reunion Island for antimalarial and cytotoxic activity. Journal of Ethnopharmacology, 120(3), 382–386. doi:10.1016/j.jep.2008.09.005
- Koch, A., Tamez, P., Pezzuto, J., & Soejarto, D. (2005). Evaluation of plants used for antimalarial treatment by the Maasai of Kenya. Journal of Ethnopharmacology, 101(1–3), 95–99. doi:10.1016/j.jep.2005.03.011
- Malebo, M. H., Tanja, W., Cal, M., Swaleh, S. A. M., Omolo, M. O., Hassanali, A., (2009). Antiplasmodial, anti-trypanosomal, anti-leishmanial and cytotoxicity activity of selected Tanzanian medicinal plants. Tanzania Journal of Health Research, 11(4), 47–56.
- Mesa, V. A. M., Suaza, J. F. T., Cardona, A. M. V., Trujillo, S. B., Jaramillo, C. P., Oltra, S. D., & Carda, M. (2018). Antiplasmodial and cytotoxic activity of piper piedecuestanum Trel. and Yunck. Research Journal of Medicinal Plants, 12(57), 64.
- Moore, G. E., Gerner, R. E., & Franklin, H. A. (1967). Culture of normal human leukocytes. JAMA, 199(8), 519–524.
- Mosmann, T. (1983). Rapid colorimetric assay for cellular growth and survival application to proliferation and cytotoxicity assays. Journal of Immunological Methods, 65(1–2), 55–63.
- Muñoz, V., Sauvain, M., Bourdy, G., Callapa, J., Rojas, I., Vargas, L. (2000). The search for natural bioactive compounds through a multidisciplinary approach in Bolivia. Part II. Antimalarial activity of some plants used by Mosetene Indian. Journal of Ethnopharmacology, 69(2), 139–155.
- Olumese, P. (2015). Guidelines for the treatment of malaria (3rd ed., pp. 313). World Health Organization.
- Parmar, V. S., Jain, S. C., Bisht, K. S., Jain, R., Taneja, P., Jha, A. (1997). Phytochemistry of the genus Piper. Phytochemistry, 46(4), 597–673. doi:10.1016/S0031-9422(97)00328-2
- Philippe, G., Angenot, L., De Mol, P., Goffin, E., Hayette, M. P., Tits, M. (2005). In vitro screening of some Strychnos species for antiplasmodial activity. Journal of Ethnopharmacology, 97(3), 535–539. doi:10.1016/j.jep.2004.12.011
- Ravindran, P. N., & Nirmal, K. (1994). Chemotaxonomy of South Indian Piper. Journal of Spices and Aromatic Crops, 3(1), 6–13.
- Reed, G. F., Lynn, F., & Meade, B. D. (2002). Use of coefficient of variation in assessing variability of quantitative assays. Clinical and Vaccine Immunology, 9(6), 1235–1239.
- Righi, G., Antonioletti, R., Silvestri, I. P., D‘Antona, N., Lambusta, D., & Bovicelli, P. (2010). Convergent synthesis of mosloflavone, negletein and baicalein from cysin. Tetrahedron, 66, 1294–1298. doi:10.1016/j.tet.2009.12.021
- Sawasdee, P., Sabphon, C., Sitthiwongwanit, D., & Kokpol, U. (2009). Anticholinesterase activity of 7-methoxyflavones isolated from Kaempferia parviflora. Phytotherapy Research, 23(12), 1792–1794. doi:10.1002/ptr.2858
- Shin, J., Kim, K., Kim, M., Jeong, J., & Kim, B. (1999). Synthesis and hypoglycemic effect of chrysin derivates. Bioorganic & Medicinal Chemistry Letters, 9(6), 869–874.
- Smilkstein, M., Sriwilaijaroen, N., Kelly, J. X., Wilairat, P., & Riscoe, M. (2004). Simple and inexpensive fluorescence-based technique for high-throughput antimalarial drug screening. Antimicrobial Agents and Chemotherapy, 48(5), 1803–1806.
- Sutthanutm, K., Sripanidkulchai, B., Yenjai, C., & Jay, M. (2007). Simultaneous identification and quantitation of 11 flavonoid constituents in Kaempferia parviflora by gas chromatography. Journal of Chromatography A, 1143(1–2), 227–233. doi:10.1016/j.chroma.2007.01.033
- Tanae, M. M., Lima-Landman, M. T. R., de Lima, T. C. M., Soucca, C., & Lapa, A. J. (2007). Chemical standardization of the aqueous extract of Cecropia glaziovii Sneth endowed with antihypertensive, bronchodilator, antiacid secretion and antidepressant-like activities. Phytomedicine, 14(5), 309–313. doi:10.1016/j.phymed.2007.03.002
- Trager, W., & Jensen, J. B. (1976). Human malaria parasites in continuous culture. Science, 193(4254), 673–675.
- World Health Organization. (2016). World Malaria report 2015. Geneva: Author.
- WHO. (2016). Global technical strategy against Malaria 2016–2030. Geneva: Author.
- WHO. (2017). World malaria report 2017. Geneva: Author.