Abstract
A recently released non-fumigant nematicide, fluensulfone, belonging to the fluoroalkenyl chemical group, fluensulfone, was tested against field populations of root-knot nematodes Meloidogyne sp. in commercial cucumber and tomato greenhouses. It was delivered through the drip irrigation system at doses from 12 to 72 ml 100 m−1. The application of fluensulfone substantially reduced nematode multiplication either in tomato or cucumber cultivation at all doses above 24 ml 100 m−1. Plants cultivated in plots treated with fluensulfone produced significantly more fruits throughout the cropping season for both crops. However, in tomato cultivation, doses higher than 36 ml 100 m−1 have shown a high number of dead plants a fact which was not recorded in cucumber cultivation. Laboratory experiments were conducted to evaluate any possible phytotoxicity of fluensulfone. Three solanaceous (tomato, pepper, and eggplant) and three cucurbitaceous (cucumber, squash and melon) plants were used. The root growth and the seed germination were affected when the recommended dose of fluensulfone was used.
Public interest statement
In this paper, the efficacy of the recently released nematicide, fluensulfone, was evaluated under field conditions. The chemical was delivered through the drip irrigation system at doses from 12 to 72 ml 100 m−1. Fluensulfone has shown a very good control of root-knot nematodes either in tomato or cucumber greenhouse cultivation. However, in tomato cultivation doses higher than 36 ml 100 m−1 have shown a high number of dead plants. Further experimentation has shown that the root growth and the seed germination were affected when fluensulfone was used.
Competing Interests
The authors declare no competing interests.
1. Introduction
Plant parasitic nematodes are a threat for global agriculture, responsible for about 10% losses in annual global crop yields equal to an estimated US $173 billion per year (Dutta, Khan, & Phani, Citation2019; Elling, Citation2013). Root-knot nematodes are responsible for devastating crop losses in many production areas in tropical and subtropical regions all over the world (Sikora & Fernandez, Citation2005) and also in all greenhouse vegetable production areas in Greece (personal observations). Root-knot nematodes can be controlled by chemical nematicides, cultural practices, and resistant hybrids or rootstocks (Giannakou & Anastasiadis, Citation2005; Giannakou & Karpouzas, Citation2003). Synthetic pesticides still constitute an integral part of conventional agricultural systems, compared to pesticides of biological origin or bio-pesticides and formulations based on natural plant extracts which are mostly or solely utilized in organic farming. Although there are some new commercial products containing either microorganisms or natural extracts, their use is not yet that extensive and a conclusion cannot be drawn as far as their efficacy is concerned on a large scale (Hashem et al. Citation2011; Khan et al., Citation2005). The chemical industry keeps testing new molecules in the search for new effective nematicides. A new organophosphorus nematicide under the commercial name of Imicyafos was released in Japan in 2010 (Wada, Toyota, & Takada, Citation2011). This nematicide was effective against Pratylenchus penetrans, while it had no substantial effect on free-living nematodes. Also, a recent example of a new synthetic nematicide is fluensulfone which is the only member of this chemical group released onto the market as a nematicide. It has been tested either as EC or CS formulation by the company Makteshim-Agan (pers. communication) and is registered so far for use in tomato, cucumber and other vegetable crops in Latin America under the commercial name of Nimitz®. Recently it has received an EPA registration (September 2014) for control of plant parasitic nematodes in fruiting vegetables. The mode of action of this molecule was found to be noticeably different from that of organophosphates and carbamates (Opperman & Chang, Citation1990) in a study using Caenorhabditis elegans (Kearn, Ludlow, Dillon, O’Connor, & Holden-Dye, Citation2014). In the same study, they reported that fluensulfone affects nematode reproduction, development, feeding, and motility and ultimately has shown nematicidal action (Kearn et al., Citation2014). In the literature, there are few studies using this nematicide against some genera of plant parasitic nematodes in pot or microplot experiments.
Csinos, Whitehead, Hickman, and LaHue (Citation2010) reported similar or better results than aldicarb using fluensulfone to control Meloidogyne arenaria on flue-cured tobacco. Application of fluensulfone as a foliar spray to peppers prior to Meloidogyne incognita inoculation reduced the galling index by 80% and the number of eggs by 73% to 82% compared to the control. Although the foliar application could reduce root-knot nematodes invasion in peppers, even more than that given by oxamyl and fenamiphos, this should be done prior to juveniles’ infection. However, Morris et al. (Citation2016) observed phytotoxicity in eggplant and tomato after foliar applications at a rate of 12 g a.i. per liter. The use of fluensulfone reduced root-galling in lettuce by reducing juveniles root penetration ability (Oka et al. Citation2012). It was also reported that fluensulfone’s movement is affected by clay and soil organic matter content while nematicidal activity is reduced by peat addition (Oka et al. Citation2012). Different genera of plant parasitic nematodes have shown different susceptibilities to fluensulfone application (Oka, Citation2013). The best efficacy so far has been reported on root-knot nematodes (Morris et al., Citation2015; Oka, Citation2013). Reduced nematode population density, reproduction rate, and root-galling of tomato and cucumber plants caused by Nacobbus aberrans were observed using fluensulfone at doses of 1 to 2 L ha−1 (Cabrera-Hidalgo, Moctezuma, & Mendoza, Citation2015). Noe, Jagdale, Holladay, and Brannen (Citation2015) reported a reduction of Mesocriconema xenoplax populations using fluensulfone in Guardian and Nemaguard peach seedlings. Westerdahl, Long, and Schiller (Citation2013) reported good results in controlling Meloidogyne javanica on carrot, tomato, squash, cucumber, and cantaloupe using fluensulfone. Efficacy given by non-fumigant nematicides is often inferior to that given by 1,3-D (Giannakou, Sidiropoulos, & Prophetou-Athanasiadou, Citation2002). However, Morris et al reported that fluensulfone was similar to 1,3-D in reducing galling index from Meloidogyne spp. on tomato. In many of the published papers, fluensulfone was used as a stand-alone method of controlling plant-parasitic nematodes while in few it was combined with 1,3-D (Morris et al., Citation2015) or a mixture of 1,3-D and chloropicrin (Castillo, Ozores-Hampton, & Navia-Gine, Citation2017). It can control not only root-knot nematodes but also potato cyst nematodes (Globodera pallida) as shown by Norshie, Grove, and Back (Citation2016). Fluensulfone seems to be very promising since it has true nematicidal activity in contrast with other non-fumigant nematicides which act as nematostats, it can be translocated systemically, shows good water solubility and it has lower than other non-fumigants mammalian toxicity (LD50 > 500 mg/Kg). It has 11 to 12 days half-life in the soil which is desirable from an environmental point of view since there are fewer chances to pollute groundwater (Oka, Shuker, & Tkachi, Citation2009). On the other hand, this characteristic might create a problem from a farmer’s point of view since shorter persistence in the soil means a shorter time of nematode control. Norshie, Grove, and Back (Citation2017) reported that a time to 50% dissipation is 24 days, which is a positive attribute as this nematicide may pose a negligible environmental hazard but its persistence at an effective level may be long enough to be effective over the peak hatch period of Globodera pallida.
In the present study, the efficacy of fluensulfone against root-knot nematodes (Meloidogyne sp.) was evaluated in four greenhouse experiments cultivated with either cucumber or tomato. Fluensulfone was delivered through the drip irrigation system. Also, due to preliminary field observations, a series of laboratory experiments were established to evaluate the action of fluensulfone on seeds germination, root growth and plant development of three either solanaceous (tomato, pepper, and eggplant) or cucurbitaceous (cucumber, squash and melon) plants.
Specifically this study aimed to assess: (1) the efficacy of a EC formulation containing fluensulfone on second-stage juveniles (J2s) of Meloidogyne sp, on root-galling and on nematodes per gram of root on tomato and cucumber greenhouse cultivation, (2) the effect of soil-applied fluensulfone on the root and plant growth of three solanaceous and three cucurbitaceous plant species and (3) the effect of a series of different fluensulfone doses on the germination rate of three solanaceous and three cucurbitaceous plant species.
2. Materials and methods
2.1. Efficacy trials in greenhouse
Experimental plots were established within a greenhouse which had been naturally infested with Meloidogyne sp. for many years. Preliminary soil sampling revealed a population of 80 to 220-s stage juveniles (J2s) per 100 g of soil. Each plot was 50 m2 (2 × 25 m2). Before applying any chemical, all plots were irrigated (40 l m−2). After 3 days, the soil was cultivated many times with an L-type cultivator to ensure moisture and nematode population uniformity. Prior to chemical applications, 20 soil cores (10 cm diameter × 25 cm deep) were collected from each plot using a cylindrical sampling tube for determination of pretreatment nematode population densities (pi) using Cobb’s sieves and the Baermann method. The “tomato experiment” consisted of six treatments and one untreated control while the “cucumber experiment” consisted of seven treatments plus the untreated control. The dosage and timing schedule is not the same in the two plant species since they have shown different sensitivities to the chemical in preliminary tests. All chemicals were delivered via drip irrigation system assuming that the nematicide solution was applied in a 60 cm band and incorporated in a 15 cm depth. All doses are referred to 100 walking meters. Fluensulfone 480 g liter −1 EC was applied in tomato cultivation at five different rates: 12, 24, 36, 48, and 72 ml 100 m−1 seven days prior to transplanting. In cucumber cultivation fluensulfone 480 g liter −1 EC was applied at seven different rates: 12, 24, 36, and 48 ml 100 m−1 as single application 7 days prior to transplanting and 24 and 36 ml, 7 days prior to transplanting plus 18 ml 100 m−1 15 days after transplanting. In cucumber trials the reference chemical was fenamiphos (Nemacur© 40 EC, Alfa Agricultural Supplies, Greece) at the dose of 10 l ha−1 at the day of transplanting, while in tomato trials oxamyl (Vydate© 10 SL, Du Pont, USA) at the dose of 20 l ha−1 at the day of transplanting and after 15 days. Prior to any chemical application, soil was irrigated for 10 min (about 13,000 l ha−1) to moisten soil avoiding surface diffusion of the chemicals at the day of application; then, the chemical solution was applied in 20 min (about 25,000 l ha−1) followed by another 10-min irrigation to flush residues and remove any chemicals from the drip irrigation system. Either nematode-susceptible tomato (cv Belladona) or cucumber (cv Palmera) plants were transplanted in four leaves stage. Plants were irrigated at 3-day intervals with a drip irrigation system and fertilized every week with a compound fertilizer (N:20%, P:20%, K:20%) as advised by the local agronomic authorities.
Soil samples were collected and cores were bulked for each plot at mid-season (45 days after transplanting) and at harvest. Twenty 10 cm soil cores were taken 25 cm deep near the base of plants within the row and second-stage nematode juveniles (J2) were extracted as described previously. At mid-season, 10 plants per plot were randomly marked and uprooted. They were carefully washed free of soil, and galling index was assessed according to a 1–10 scale (Bridge & Page, Citation1980). The above procedure was repeated once more at the end of the cropping season. At mid-season, all developmental stages of nematodes per root system were counted from 10 plants of the central line of each plot (Byrd, Kirk Patrick, & Barker, Citation1983). Fifty grams of roots were randomly collected from 0 to 25 cm depth using a shovel sampler, they were carefully washed free of soil. Roots were boiled for 3 min in a solution of equal volumes of glycerol, lactic acid and distilled water plus 0.05% acid fuchsin. Roots were then washed in water and placed in vials containing equal volumes of glycerol and distilled water plus a few drops of lactic acid. Roots were chopped and two aliquots of 1 g each were taken. All developmental stages of nematodes on the roots were counted using a stereoscopic microscope at 12.5× magnification. Stem diameter was measured at mid-season while the total yield of commercial fruits was estimated from 10 plants from the middle of each plot throughout the cropping season. The greenhouse temperature during the cropping season (May–September) was 20–35◦C. Both experiments (either tomato or cucumber) were conducted twice and due to similar variances data from both experiments were combined and analyzed using one-way ANOVA. Each treatment was replicated five times in both experiments.
2.2. Seed germination and root development in Petri dishes
The germination assay was conducted according to Wang and Zhou (Citation2005). Seeds of vegetables were surface-sterilized with 10% (V/V) sodium hypochlorite solution for 10 min followed by a thorough washing in distilled water. Twenty seeds were placed in each Petri dish (90 mm in diameter) containing a filter paper moistened with fluensulfone solution. The experiment consisted of one untreated control (deionized water only) and nine different doses of fluensulfone as follows: 0.625, 1.25, 2.5, 5, 10, 20, 40, 80, and 160 ppm. Petri dishes were maintained to allow seeds germination for 7 days in the dark at 25 ± 0.5°C and 50% humidity. The percentage of germinated seeds was estimated and also root length was recorded. Each treatment was replicated five times while the experiment was conducted twice and the data were combined since the variances between the experiments were similar.
2.3. Action of fluensulfone on plant development in pot trial
According to the label, the highest recommended concentration of fluensulfone for drip irrigation in 60 cm band is 48 ml of formulated product per 100 m. Assuming that the chemical is evenly distributed into 15 cm depth then the above amount of chemical represents a realistic pesticide concentration of 10 ppm in the soil solution assuming that (1) no adsorption of the pesticide to the soil colloids occurs, (2) the moisture content of the soil is at water-holding capacity, which for a sandy loam soil ranges between 15% and 24% (20% was assumed) and (3) pesticide residues are delivered within the top 15 cm of the surface soil. Subsequently, four lower and four higher concentrations of nematicide were selected for the experiment.
Commercial compost was left to air dry to reduce moisture to zero level. A quantity of 200 ml of fluensulfone solution with the proper concentration was sprayed using a hand-sprayer and thoroughly distributed in 1 Kg soil. Preliminary tests have shown that 200 ml of solution is required for the soil to reach water holding capacity. Thereafter, the soil was equally divided into five 200 cm3 plastic pots. A seedling of tomato, pepper, eggplant, cucumber, squash, or melon was transplanted into each pot. At the time of transplanting the height of each plant was measured. Then, all plants were maintained for 30 days in a greenhouse at 23 ± 3°C and 16 h light. All plants were rearranged every 3 days to avoid any effect of light conditions to plant height. After that period all plants were removed from pots and their height was measured. Each treatment was replicated five times while the experiment was repeated twice.
2.4. Statistical analysis
Analysis of variance (ANOVA) was performed on the data obtained from each experiment using the General Linear Model, while treatments means were separated according to Tukey’s HSD test. Wherever data from repeated experiments were similar and consistent with no variation, they were combined before final analysis. All statistical analyses were conducted using SAS Statistical package (SAS University Edition).
3. Results
3.1. Efficacy trials in greenhouse
The results of both experiments (1 and 2) and both crops (tomato or cucumber) are presented in Tables and . The highest Pf/Pi ratio (P > 0.001) was recorded for the control treatment either in tomato or cucumber experiment. Nematode population as monitored based on soil sampling was kept low in plots treated with fluensulfone treatment at 36 and 18 ml 100 m−1 in the tomato experiments. This Pf/Pi ratio was similar (P < 0.05) to that given in plots treated with fluensulfone at 48, 24 + 18, and 24 ml 100 m−1. In both experiments (either tomato or cucumber) significantly (P < 0.001) higher Pf/Pi ratio was recorded in the plot treated with fluensulfone at 12 ml 100 m−1 comparing to all the other chemical treatments (Tables & ). The root-galling at 21 days after transplanting was substantially higher in the control plots compared to all other fluensulfone treatments. Plots treated with fluensulfone at 12 ml 100 m−1 showed significantly more galls in the roots compared to the other fluensulfone treatments. Root galling was significantly different (P < 0.001) between control and fluensulfone treatments until the end of the cropping season. At that time only the fluensulfone treatment at 12 ml 100 m−1 has shown lower root-galling while all the other fluensulfone treatments have shown similar results amongst them, as far as root-galling is concerned. The highest number of nematodes per gram of root was recorded in the control plots in both crops. However, in tomato crop, there was a significant difference between the dose of 12 ml 100 m−1 and the other treatments which was not recorded in cucumber crop. Although there were differences for the stem diameter among the different treatments, this is not fluensulfone dose-dependent. Fluensulfone-treated plots gave greater yields (P < 0.001) than control, while the yield in the plots treated with 12 ml 100 m−1 only in cucumber crop, was lower to the other fluensulfone treatments.
Table 1. Effect of different doses of fluensulfone on the numbers of second-stage juveniles per 100 g of soil, root galling, nematodes per gram of root, numbers of dead plants, stem diameter, and total yield in cucumber plants cultivated in greenhouse
Table 2. Effect of different doses of fluensulfone on the numbers of second-stage juveniles per 100 g of soil, root galling, nematodes per gram of root, numbers of dead plants, stem diameter, and total yield in tomato plants cultivated in greenhouse
3.2. Seed germination
Statistical analysis has shown a significant difference (P< 0.05) in the germination rate of all solanaceous species while no effects were recorded in all cucurbitaceous species since there was a complete germination of all cucumber, squash and melon seeds after 1-week incubation. There is a gradual decrease of germination of solanaceous seeds as the dose of fluensulfone was increased from 0.625 to 80 ppm. As shown in Figure , the decrease in germination was evident from 0.625 to 20 ppm (P< 0.05) of fluensulfone for eggplant, from 2.5 to 40 ppm (P< 0.05) for pepper and from 10 to 80 ppm (P< 0.05) for tomato seeds, respectively.
3.3. Root growth in Petri dishes
A clear decrease in root growth was recorded in all species, but in some plant species, this growth decrease was much sharper than the others (Figure ). Roots developed in Petri dishes treated with fluensulfone at 10 ppm had always reduced growth compared to the control treatment. There was a significant difference between the control and the fluensulfone treatments in the three solanaceous species (P < 0.001). In squash, the control treatment gave a significantly greater root growth than the 1.5 ppm treatment of fluensulfone. In cucumber lower root development was recorded between control and fluensulfone treatments, while a clear root growth decrease was evident as the dose was increasing. In melon, the control was significantly different to the dose of 1.25 ppm. However a sharp decrease in root growth was recorded when the dose was increased from 5 to 10 ppm.
Figure 2. The effect of fluensulfone on the root length of (a) tomato, (b) pepper, (c) eggplant, (d) squash, (e) cucumber, and (f) melon as affected by different doses of fluensulfone after 7 days incubation at 20°C. Bars with the same letter are not significantly different (P > 0.05). Doses explanations: (1) control, (2) 0.625, (3) 1.25, (4) 2.5, (5) 5, (6) 10, (7) 20, (8) 40, (9) 80, and (10) 160 ppm. Error bars show standard error of the mean values.
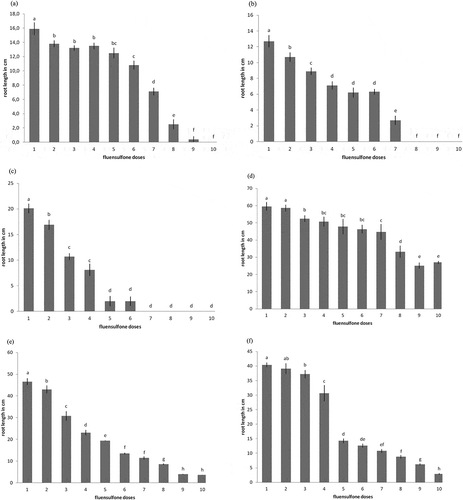
In all plant species (either solanaceous or cucurbitaceous) there is a clear retard of root development even in doses substantially lower than the recommended one. Especially in some plant species (tomato and pepper), a high decrease of root development was recorded when the dose was increased from 10 (recommended dose) to 40 ppm. In eggplant a much sharper decrease of root length was recorded in all doses used and no development was recorded at all when the dose was increased to 40 ppm.
3.4. Action of fluensulfone on plant development in pot trial
In general, differences in plant development were recorded amongst the different plant species (Figure ). In tomato, eggplant, and squash, the recommended dose has shown a significant plant growth reduction (P < 0.05), comparing to the control treatments while no significant differences were revealed after the statistical analysis between control and recommended dose in pepper, cucumber and melon (P > 0.05).
Figure 3. The effect of fluensulfone on plant length of (a) tomato, (b) pepper, (c) eggplant, (d) squash, (e) cucumber, and (f) melon as affected by different doses of fluensulfone 30 days after transplanting in pots maintained at 23 ± 3°C in a greenhouse with 18 h light. Bars with the same letter are not significantly different (P > 0.05). Doses explanations: (1) control, (2) 0.625, (3) 1.25, (4) 2.5, (5) 5, (6) 10, (7) 20, (8) 40, (9) 80, and (10) 160 ppm. Error bars show standard error of the mean values.
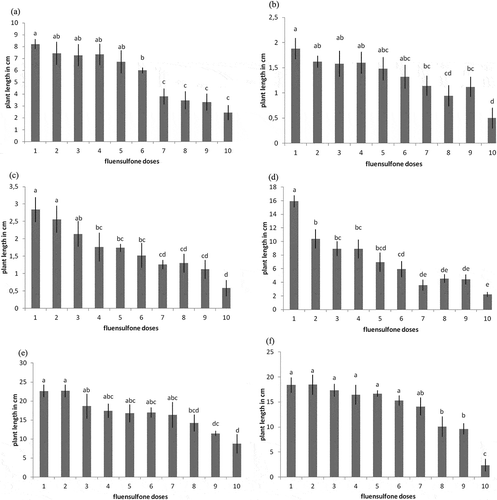
4. Discussion
The results show that fluensulfone is a very effective nematicide, but on the other hand, it has shown a nondesirable phytotoxicity effect, especially in solanaceous plant species. The need for experimentation looking at the phytotoxicity of this substance was initiated by the results and observations at field level. Fluensulfone was initially used as a nematicide in tomato and cucumber greenhouse cultivations. However, many plants were dead within the first week after transplanting accompanying with plant development retard. Further experimentation was conducted clarifying the cause of the observed phytotoxicity. Also, a root retard was obvious for some days after application, plants growth was ceased but later plants were recovered.
The results presented in this study are in agreement with those reported by previous researchers showing the high level of efficacy of fluensulfone against root-knot nematodes in tomato and cucumber (Morris et al., Citation2016), pepper (Oka et al. Citation2012), lima beans (Jones, Kleczewski, Deseager, Meyer, & Johnson, Citation2017) and against PCN (Norshie et al., Citation2016).
In the cucumber trials, a greater root-galling index reduction was achieved using fluensulfone compared to oxamyl. A similar decrease in the numbers of nematodes per gram of root was recorded either with fluensulfone at 6 and 12 l ha−1 or with oxamyl at 4 l ha−1. More dead tomato plants were recorded throughout the cropping season in the plots treated with the recommended or higher doses of fluensulfone. When plots were treated with 36 or 72 100 m−1 then there was a 4 or 7-fold increase in the number of dead tomato plants compared to the control plots. Total yield of cucumber fruits was always higher in the plots treated either with fluensulfone (in any tested dose) or oxamyl. Almost equal increase in the yield was recorded in the plots treated with the recommended dose of oxamyl (32 % higher than the control) or with 8 l ha−1 fluensulfone (31%).
In the tomato trials, the lowest root-galling index was recorded in the plots treated with fluensulfone at 4 l ha−1 which was similar to that given in the plots treated with either 8 l ha−1 (single application) or 4 + 3 l ha−1 (double application). This is desirable from an economic, agronomic and environmental point of view. All chemical treatments significantly reduced the numbers of nematodes per gram of root compared to the control, showing significantly similar results between each other. Surprisingly no differences were revealed in the numbers of dead tomato plants as occurred with the cucumber plants, although cucumber plants in field level seem to be more sensitive after pesticide application. More fruits were harvested by the end of the cropping season from plots treated with either 6 l (single application) or 6 + 3 (double application) liters ha−1 which was almost 36% higher than the control plots. Although in tomato trials there is no clear difference amongst the different chemical treatments, there is a significant difference at the end of the cropping season for the total yield. Probably the degradation products from these chemicals create a better soil environment in the root rhizosphere with more nutrients which reflect a better plant development and yield production.
Oka et al. (Citation2012) reported that pepper is less sensitive than tomato as far as the phytotoxicity of fluensulfone is concerned after soil or foliage application. Fluensulfone has shown an important phytotoxicity when applied in the soil if seedlings are transplanted earlier than a 7-day interval. However, Oka et al. (Citation2009) working with lettuce reported that fluensulfone was less effective when the treated soil was inoculated after 7 days while this did not occur in fenamiphos-treated soil.
Phytotoxicity on cultivated crops after the use of 1,3-Dichloropropene and Chloropicrin has been reported by Desaeger and Csinos. Also, previous work has shown phytotoxic effect after the use of fluensulfone as a foliar spray in tomato and eggplant (Morris et al., Citation2016). Jones et al. (Citation2017) reported that fluensulfone reduced seedling emergence of lima beans in microplot trials. In our trials, fluensulfone showed phytotoxicity, although it was delivered through the drip irrigation system and not applied as a foliar spray. It has to be explained the fact that the phytotoxicity was evident only in tomato plants and not in cucumber. Based on our experience working on a field level, this is not easily explained since cucumber seems to be more sensitive to the use of pesticides compared to tomato. Probably the higher susceptibility of tomato could be due to some kind of biochemical pathway and it is not only a case of phytotoxicity due to a high dose of a pesticide which is common with many molecules used in cultivated crops. Although the “toxic” effect of fluensulfone as monitored in Petri dishes experiments is reflected in the high number of dead plants in greenhouse experiments using tomato, is not the same in cucumber greenhouse experiments. This cannot be explained and further experimentation is required as mentioned before. Our results have shown that fluensulfone, even in lower than the recommended doses, reduced seedlings emergence. However, this conclusion can be used only ad hoc since different plant species show different emergence and rate of development in the presence of fluensulfone. It could be hypothesized that fluensulfone in the early growth stages of the plants (at the seed level) acts as a root development inhibitor. More trials must be conducted to clarify the level of phytotoxicity in cell level and also in the field.
Disclosure statement
No potential conflict of interest was reported by the authors.
Additional information
Funding
Notes on contributors
Ioannis O. Giannakou
Ioannis O. Giannakou is a member of the Agricultural University of Athens. He has long-standing collaboration in studies regarding the control of plant parasitic nematodes. Dr Giannakou has expertise on the interaction of plant parasitic nematodes with the main crops cultivated in Greece. Dr Giannakou has extensive experience in laboratory and field evaluation of chemical and biological nematicides. During the last decade he has been involved in studies regarding the efficacy of plant extracts and their constituents against root-knot nematodes, cyst nematodes and bulb nematodes. Dr Giannakou and his team could be involved in any international project dealing with the evaluation of new molecules against plant parasitic nematodes and also monitoring the environmental fate of these molecules in the field
References
- Bridge, J., & Page, S. L. J. (1980). Estimation of root-knot nematode infestation levels on roots using a rating chart. Tropical Pest Management, 26, 296–12. doi:10.1080/09670878009414416
- Byrd, D. W., Kirk Patrick, J. T., & Barker, K. R. (1983). An improved technique for clearing and staining plant tissues for detection of nematodes. Journal of Nematology, 15, 142–143.
- Cabrera-Hidalgo, A. J., Moctezuma, E. V., & Mendoza, N. M. (2015). Effect of fluensulfone on the mobility in vitro, and reproduction and root galling of Nacobbus aberrans in microplots. Nematropica, 45, 59–71.
- Castillo, G. X., Ozores-Hampton, M., & Navia-Gine, P. A. (2017). Effects of fluensulfone combined with soil fumigation on root-knot nematodes and fruit yield of drip- irrigated fresh-market tomatoes. Crop Protection, 98, 166–171. doi:10.1016/j.cropro.2017.03.029
- Csinos, A., Whitehead, J., Hickman, L. L., & LaHue, S. S. (2010). Evaluation of fluensylfone for root knot nematode on tobacco. Phytopathology, 100, 28.
- Dutta, T. K., Khan, M. R., & Phani, V. (2019). Plant-parasitic nematode management via biofumigation using brassica and non-brassica plants: Current status and future prospects. Current Plant Biology, 17, 17–32. doi:10.1016/j.cpb.2019.02.001
- Elling, A. A. (2013). Major emerging problems with minor Meloidogyne species. Phytopathology, 103, 1092–1102. doi:10.1094/PHYTO-01-13-0019-RVW
- Giannakou, I. O., & Anastasiadis, I. (2005). Evaluation of chemical strategies as alternatives to methyl bromide for the control of root-knot nematodes in greenhouse cultivated crops. Crop Protection, 24, 499–506. doi:10.1016/j.cropro.2004.09.007
- Giannakou, I. O., & Karpouzas, D. G. (2003). Evaluation of chemical and integrated strategies as alternatives to methyl bromide for the control of root-knot nematodes in Greece. Pest Management Science, 59, 883–892. doi:10.1002/ps.692
- Giannakou, I. O., Sidiropoulos, A., & Prophetou-Athanasiadou, D. A. (2002). Chemical alternatives of methyl-bromide for the control of root-knot nematodes in greenhouses. Pest Management Science, 58, 290–296. doi:10.1002/ps.453
- Hashem, M., & Abo-Elyousr, K. A. (2011). Management of the root-knot nematodes Meloidogyne incognita on tomato with combinations of different biocontrol organisms. Crop Protection, 30, 285–292. doi:10.1016/j.cropro.2010.12.009
- Jones, J. G., Kleczewski, N. M., Deseager, J., Meyer, S. L. F., & Johnson, G. C. (2017). Evaluation of nematicides for southern root-knot nematode management in lima beans. Crop Protection, 96, 151–157. doi:10.1016/j.cropro.2017.02.015
- Kearn, J., Ludlow, E., Dillon, J., O’Connor, V., & Holden-Dye, L. (2014). Fluensulfone nematicide with a mode of action distinct from anticholisterases and macrocyclic lactones. Pesticide Biochemistry and Physiology, 109, 44–57. doi:10.1016/j.pestbp.2014.01.004
- Khan, Z., Park, S. D., Shin, S. Y., Bae, S. G., Yeon, I. K., & Seo, Y. J. (2005). Management of Meloidogyne incognita on tomato by root-dip treatment in culture filtrate of the blue-green alga, Microcoleus vaginatus. Bioresource Technology, 96, 1338–1341. doi:10.1016/j.biortech.2004.11.012
- Morris, K. A., Langston, D. B., Davis, R. F., Noe, J. P., Dickson, D. W., & Timper, P. (2016). Efficacy of various application methods of fluensulfone for managing root-knot nematodes in vegetables. Journal of Nematology, 48(2), 65–71.
- Morris, K. A., Langston, D. B., Dickson, D. W., Davis, R. F., Timper, P., & Noe, J. P. (2015). Efficacy of fluensulfone in a tomato-cucumber double cropping system. Journal of Nematology, 47(4), 310–315.
- Noe, J., Jagdale, G. B., Holladay, W. T., & Brannen, P. M. (2015). Fluensulfone for management of Mesocriconema xenoplax on peach. Journal of Nematology, 47(3), 259.
- Norshie, P. M., Grove, I. G., & Back, M. A. (2016). Field evaluation of the nematicide fluensulfone for control of the potato cyst nematode Globodera pallid. Pest Management Science, 72, 2001–2007. doi:10.1002/ps.4329
- Norshie, P. M., Grove, I. G., & Back, M. A. (2017). Persistence of the nematicide fluensulfone in potato (Solanum tuberosum spp. tuberosum) beds under field conditions. Nematology, 19, 739–747. doi:10.1163/15685411-00003085
- Oka, Y. (2013). Nematicidal activity of fluensulfone against some migratory nematodes under laboratory conditions. Pest Management Science, 70, 1850–1858. doi:10.1002/ps.3730
- Oka, Y., Shuker, S., & Tkachi, N. (2009). Nematicidal efficacy of MCW-2, a new nematicide of the fluoroalkenyl group, against the root-knot nematode Meloidogyne javanica. Pest Management Science, 65, 1082–1089. doi:10.1002/ps.1796
- Oka, Y., Shuker, S., & Tkachi, N. (2012). Systemic nematicidal activity of fluensulfone against the root-knot nematode Meloidogyne incognita on pepper. Pest Management Science, 68, 268–275. doi:10.1002/ps.2256
- Opperman, C. H., & Chang, S. (1990). Plant-parasitic nematode acetylcholinesterase inhibition by carbamate and organophosphate nematicides. Journal of Nematology, 22, 481–488.
- Sikora, R. A., & Fernandez, E. (2005). Nematode parasites of vegetables. In M. Luc, R. A. Sikora, & J. Bridge (Eds.), Plant parasitic nematodes in subtropical and tropical agriculture (pp. 319–392). Wallingford, CT: CABI Publishing.
- Wada, S., Toyota, K., & Takada, A. (2011). Effects of the nematicide imicyafos on soil nematode community structure and damage to radish caused by Pratylenchus penetrans. Journal of Nematology, 43(1), 1–6.
- Wang, M., & Zhou, Q. (2005). Single and joint toxicity of chlorimuron-ethyl, cadmium, and copper acting on wheat Triticum aestivum. Ecotoxicology and Environmental Safety, 60, 169–175. doi:10.1016/j.ecoenv.2003.12.012
- Westerdahl, B., Long, D., & Schiller, C. T. (2013). MCW-2 for management of root-knot nematode on annual crops. Journal of Nematology, 45(4), 279–330.