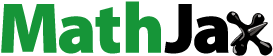
Abstract
This study investigated the properties of fish pellets made with plant-based ingredients intended for rainbow trout (Oncorhynchus mykiss) or other Salmonids. Plant protein from camelina meal (CMM), canola meal (CM), and isolated soybean protein (ISP) were the variable portions constituting 70% (w/w) of the seven formulations. The other 30% of ingredients included binders (7% wheat gluten protein (WGP) and 3% guar gum (GG)), 7.5% vitamins, 5% fish oil and 7.5% fishmeal. The densified pellets were made one at a time using a compaction plunger and die apparatus at 14% moisture, a die temperature of 90 °C and a compaction pressure of 112 MPa. The feed properties studied were morphology of the starting pellet powders, pellet density, durability, disintegration in water (e.g. water stability) and transverse crushing load. Results indicated the mix containing 5% CMM/5% CM/60% ISP had better durability, water stability and transverse tensile strength. The CM contributed to poor pellet performance, while the ISP produced the most favorable results. Camelina meal performed moderately well and resulted in the highest density. This study may help aqua feed producers in the selection, composition and performance of feed containing agricultural products that are more economical than current meat-based fishmeal.
PUBLIC INTEREST STATEMENT
Current fish feed pellets contain mostly fish based meat proteins. With fish stocks reducing worldwide, a new interest is to replace meat proteins with plant based proteins while retaining the same nutritional value. The plant proteins consisted of camelina meal, canola meal, and isolated soybean protein, while binders were wheat gluten protein and guar gum. In addition, smaller amounts of vitamins, fish oil, and fishmeal were added. The goal of this investigation was to understand how these vegetable proteins in various quantities affected pellet mechanics. This study may help aqua feed producers in the selection, composition and performance of feed containing agricultural products that are more economical, nutritious and cheaper than current meat-based fishmeal.
Competing interests
There are no conflicts of interest to disclose.
1. Introduction
Aquaculture is the farming of fish and other water crustaceans. This form of cultivation has gained momentum over the years due to the decrease of wild fish stocks worldwide. Today, aquaculture contributes about half of fish food consumed globally (Food and Agriculture Organization [FAO], Citation2018a). According to the Food and Agriculture Organization (FAO) of the United Nations, as of 2015, the world’s commercial wild fish populations have not improved. About 33.1% of fish stocks have been estimated as fished at an unsustainable level and therefore are considered overfished (FAO, Citation2018b).
Current fish feed mostly contains fish-based meat proteins (fishmeal). In 2016, the amount of the world fish production peaked at 171 million tonnes (mts). Of this amount, 53% or 91 mts was used in aquaculture feed (FAO, Citation2018a). As fish stocks dwindle, vegetable-proteins free of fishmeal have seen growing interests as a replacement for fish meat protein (Adelizi et al., Citation1998). In a recent review article, it has been reported that plant proteins could be substituted for fishmeal without negative effects on growth or feed intake (Daniel, Citation2018). For the aquaculture industry to grow and thrive, it will be essential to decrease the use of raw fishmeal and increase the use of proteins from plant sources.
Fish feed derived from plant-based proteins should maintain the same nutrient profile as fish-based meat protein. One method to measure the efficiency for a fish to turn feed into consumable meat for human consumption is to use the feed conversion ratio (FCR) which is calculated by dividing the total dry feed supplied (grams) by the total weight gain (grams). For instance, if a fish had an FCR of 1.20, it would require about 1.20 kg of feed to produce 1 kg of fish meat. A fish with a low FCR is more efficient at retaining protein and energy from the feed and transforming it into consumable meat. (Zhang et al., Citation2012) replaced fishmeal (Norse-LT94®) with plant based proteins for use in rainbow trout (salmonid) (Oncorhynchus mykiss) diets. The results did not show any adverse effects in feed intake or growth by using plant proteins. The FCR for the fishmeal was 0.72, while the FCR varied between 0.78 to 0.87 for the plant based protein diets. (Kyeong-Jun et al., Citation2010) compared anchovy based fishmeal with plant protein diets in rainbow trout. The plant-based diets did not show drawbacks in growth and feed consumption. The FCR for the fishmeal was 0.85, while the diets based on plant proteins ranged from 0.85 to 0.96 in FCR.
Various plant-based ingredients have been used in different diet recipes for aquaculture feeds of farmed rainbow trout. Camelina meal (CMM) is an underutilized agricultural product and has been studied as an additive in farmed salmonids. CMM has recently been discovered as a valuable fish feed ingredient due to its high crude protein level and amino acid content (Hixson et al., Citation2016). Canola meal (CM) is a common source of high protein feed for beef, dairy cattle, poultry, and pigs. A study added 0–20% CM to diets of rainbow trout and determined the weight gains were similar to those fed the control diets. The diets with 0% and 20% CM had FCRs of 1.74 and 1.65, respectively. The addition of CM did not adversely reduce the growth and food conversion for the fish (Hardy & Sullivan, Citation1983). Isolated soybean protein (ISP) is a purified form of soybean protein, which reduces the digestive problems linked to the anti-nutritional soybean meal in fish. A study evaluated the effect of growth on rainbow trout fed diets containing fishmeal versus ISP as the main source of protein. The diets containing fishmeal had FCRs of 1.2–1.3, while the ISP diets had higher FCRs of 1.5. The results showed that a complete replacement of fishmeal with ISP had negative effects on the growth performance of the fish (Jurij et al., Citation2012). Perhaps partial replacements of ISP with fishmeal may provide a better alternative, rather than being a major ingredient. Wheat gluten protein (WGP) is obtained by extracting starch from wheat flour. It was reported to be high in protein and performed well as a binder in extruded feed pellets. Adding WGP to rainbow trout feed should be kept low as they have difficulty in digesting this type of protein source (Apper-Bossard, Feneuil, Wagner, & Respondek, Citation2013). In a study with 6% WGP added to various diets for rainbow trout, results showed that the growth performance was not adversely affected (Tusche, Arning, Wuertz, Susenbeth, & Schulz, Citation2012). Guar gum (GG) originates from the Indian cluster bean (Cyamoposis tetrugonobbus). It has also been added as a binder in fish feed for rainbow trout. A study found additions of 2.5% GG to the feed did not affect growth rate of rainbow trout; however, larger quantities reduced growth and feed conversions. The study also found that GG would reduce digestibility of fat in rainbow trout (Storebakken, Citation1985), but helped decrease feces breakdown in turbulent waters and improved buoyancy of waste feces and its particles (Brinker, Koppe, & Rösch, Citation2005). To meet the nutritional requirements of rainbow trout, experimental feed pellets generally contain a vitamin premix (Barrows, Gaylord, Sealey, Porter, & Smith, Citation2008). Vitamins ensured nutritional inadequacies from present plant ingredients were met. The proposed plant-based ingredients for this experimental fish feed were selected to replace conventional fishmeal in terms of amino acids, fatty acids, adequate proteins and energy levels while reducing antigenicity.
The goal of this research was to study the physical quality of fish pellets containing 70% plant-based proteins (CMM, CM and ISP), 10% binders (WGP and GG) and about 20% fish-based protein (vitamin premix, fish oil and fishmeal) for potential rainbow trout feed. By varying the three plant-sourced ingredients and maintaining the fish protein ingredients constant, seven recipes were mixed and pelletized. This study evaluated the microstructural characterization of the ingredients, as well as the density, durability, water disintegration, and transverse crushing load of the densified products in pellet form.
2. Materials and methods
2.1. Feed ingredients
Seven feed pellet diets were produced by varying three vegetable protein ingredients (CMM, CM and ISP), while maintaining the other ingredients (WGP, GG, vitamin premix, fish oil and `fishmeal) constant. Diets of each pellet formulation consisted of CMM obtained from Three Farmers (Saskatoon, Saskatchewan), CM from Cargill (Clavet, Saskatchewan), ISP from Singsino Group Ltd., WGP flour from Duinkerken Foods Inc. and GG from Bulk Barn® (Table ). The vitamin premix and salmon fish oil were purchased from Petland® and the fishmeal from Skretting Canada. All material ingredients were obtained in powder form except for the fish oil which was used in an as-received condition from the suppliers. A control feed fish pellet was obtained from off the shelf (Laguna®).
Table 1. Pellet mix formulations for a 30 g batch diets
2.2. Material preparation
The as-received powdered ingredients were dried in an oven at 105 °C for 24 h. The sample size for each of the seven recipes was a total of 40 g. The ingredients were individually weighed and mixed thoroughly using a hand mixer. For proper densification of pellets, single pellets were made using 5.5 g ± 0.5 g of each recipe based on the size of the compaction die volume. Prior to compaction, the recipes were conditioned to 14 % moisture (mass fraction) content by water spray, stored in a sealed plastic jar and placed in a fridge at 4 °C for 72 hours.
2.3. Compaction apparatus
Single pellets were produced at 90 ± 1 °C using a pelletizing apparatus as outlined in (Sudhagar, Tabil, & Sokhansanj, Citation2006). The stationary heated cylindrical die had a height of 135.3 mm ±0.5 mm and diameter of 6.30 ± 0.5 mm (Figure ). The 6.25 mm ±0.5 mm diameter mechanical plunger was operated using an Instron Model 1011 testing machine (Instron Corp., Canton, MA, USA) with a load cell of 5 kN and a Bluehill computer operating program. Compaction of pellets into the die was performed with a load of 3500 N or a calculated stress of 112 MPa based on the applied load divided by the die diameter. The cross-head speed of 50 mm/min was applied for 1 minute to prevent the “spring back” effect of the powdered compact (Figure ). The pellets were dried at room temperature of 23 °C, for 7 days. The control pellets measured approximately 3.6 mm x 3.9 mm (length x diameter).
2.4. Feed composition
The nutritional profiles of rainbow trout were selected to fulfill the requirements of NRC (Jobling, Citation2012). The experimental nutrition profile and composition of diets were formulated to cover the nutritional requirements (Table ). The values were arrived using information provided by the supplier of each ingredient. Diverting from the nutritional profile of trout may affect fish growth and fish flesh quality.
Table 2. Required nutrient profile of trout and experimental nutrient profile achieved for each formulated recipe studied
2.5. Microstructural characterization
The scanning electron microscope (SEM) was used to observe the morphology of the pellet powder ingredients. The SEM model was a JEOL JSM-6010 LV (Tokyo, Japan) and images were taken at an operating voltage of 10kV. Prior to analysis, the samples were mounted on stubs, coated with a thin layer of gold using a sputter coater to improve the conductivity.
2.6. Single pellet density
The compacted single pellet densities were obtained by measuring the weight, while height and diameter were measured with a caliper at three places and averaged. The compact density for each formulation was calculated based on the average of five pellets in kgm−3.
2.7. Single pellet durability
During manufacturing and transportation, pellets may be exposed to gravity forces in chutes, which could cause them to breakdown and be damaged during handling. The impact resistance drop test has been commonly used to evaluate the durability of biomass pellets when there are limited quantities available (Al-Widyan & Al-Jalil, Citation2001; Kambo & Dutta, Citation2014). The test consists of dropping the same pellet two times from a height of 1.85 m onto a hard flat surface. The durability was determined by comparing the final weight (Wf) to the initial weight (Wi) as a percentage of the solid piece remaining as given by Equation 1. Three replicates of each pellet mix were tested and averaged.
2.8. Pellet disintegration in water
The dry pellets could be wasted if they disintegrate before being eaten by the fish. The conditions of temperature, turbulence and flow of water can vary considerably between fish farms. To date, there are no standardized tests for evaluating pellet stability in water that could be used for a laboratory analysis (Obaldo, Divakaran, & Tacon, Citation2001). For this reason, it has been suggested that a visual assessment can be made to evaluate the extent of disintegration (Knights, Citation1983). In this study, to verify the stability of the pellets in water, samples with average pellet diameters of 6.4 ± 0.07 mm and lengths of 4.9 ± 0.32 mm were placed in 250 ml of distilled water at ambient temperature (23 °C). In the lack of actual fish to agitate the water, each water/pellet was stirred one full revolution by a glass stir stick once every minute for the duration of the test to simulate water disturbance during fish feeding. Each pellet formulation was repeated three times. Generally, for rainbow trout feeding, if pellets remain after 15 minutes, the food decomposes into waste, toxifies and removes oxygen from the water. Tests were conducted up to 35 minutes to simulate feeding time and time allocated to collect non-eaten food to prevent waste.
2.9. Transverse crushing load
During packaging, storage and transportation, cylindrical fish pellets would most often be loaded in the transverse direction (on the sides of the pellets) due to the geometry of the cylinder. The test consists of placing a pellet on its side between two steel plates and applying a load until failure (Stelte et al., Citation2013). Tests were conducted using an Instron 1011 at a strain rate of 50 mm min−1. The resulting transverse crushing load (N) were average values of five samples. Statistical analysis of the results for the control and the formulations were conducted based on a one-way experimental test using MINITAB software and Duncan’s multiple range test at 95% confidence level.
3. Results and discussion
3.1. Microstructural characterization
The characteristics of the as-received ingredients used to manufacture the fish pellets were observed under SEM (Figure ). The CMM (Figure ) exhibited irregular shapes with rough, jagged surfaces. The particles ranged in sizes from about 3 μm to 110 μm. The CM (Figure )), showed rough non-uniform particle surfaces, which appeared to be compacted together in sizes ranging from 5 μm to 200 μm. The ISP (Figure )) had smooth rounded surfaces, almost spherical in shape. The spheres are separate from one another and have a broad size distribution ranging from 5 μm to 80 μm. Some are full spheres, while others appear to be deflated or wrinkled, which may be due to the spray drying process (Ortiz et al., Citation2009). The ISP were not solid spheres as initially thought but hollow spheres. The WGP (Figure ) appeared as asymmetrical granules in sizes ranging from 5 μm to 200 μm. The GG powder (Figure ) showed discrete structures with elongated, rod shapes having smooth surfaces. The average lengths varied from 15 μm to 140 μm. The vitamin premix (Figure ) powder appeared to have an irregular morphology with a variable particle size distribution due to the grinding process. The variety of particles sizes and shapes may have an effect on the densification behaviour of the final pellets.
3.2. Pellet density
Pellet unit density (Table ) is an important property for aquaculture feed as it will dictate if the pellet will float or sink. If the density of the pellet is less than the density of the water, the pellet will float, otherwise it will sink. Therefore, for fresh water pellets with densities greater than 1000 kgm−3, they will tend to sink. Depending on the fish species, the density of the pellets can be adjusted accordingly to produce feed for a targeted species. However, this was not the goal of the current study. The change in density of the single pellets was a function of ingredients rather than the compaction pressure. For biomass samples, the application of load was found to not affect the density after an applied pressure greater than 94.7 MPa (110.6 MPa for this study) as the compact pellet density appoaches its respective particle density (Phani, Tabil, & Schoenau, Citation2009).
Table 3. Pellet density and durability for each formulation mix
The compact unit density of the pellets was determined for the various ingredients used in the fish pellet formulations. The compaction load, hold time and moisture content were fixed, while the variables were the amount of each ingredient. The different diets or recipes had density values within the same range. The density of the control pellets were 1245 ± 7 kgm−3 and the compacted density of the mixes were not considerably different. The highest density was obtained for Mix 1 formulation containing 60/5/5 (CMM/CM/ISP), while the lowest density was for Mix 2 containing 5/60/5 (CMM/CM/ISP). When the ISP content was held constant (Mix 1 and 2 and Mix 4 & 5), adding more CMM showed an increase in the pellet density. Similarily adding more CM decreased the pellet density. Between CMM and CM, CMM was more dominate at increasing density. When CM was held constant (Mix 1 & 3 and Mix 4 & 6), adding more CMM increased the density. Similarly, adding more ISP decreased the density. Between ISP and CMM, CMM was more dominate at increasing density. When CMM was held constant (Mix 2 & 3 and Mix 5 & 6), adding more CM tended to decrease the pellet density, but adding additional ISP increased the density. Between CM and ISP, ISP was more dominate at increasing the density. The results suggest that the addition of CMM was more dominate at increasing the density of the pellets, while CM decreased pellet density. The ISP can provide a rise or drop in density depending on its formulation. It was previously observed for individual biomass wood and straw pellets that a high particle pellet density did not necessarily have an effect on pellet durability (Lehtikangas, Citation2001; Obernberger & Thek, Citation2004; Temmerman, Rabier, Jensen, Hartmann, & Böhm, Citation2006).
3.3. Pellet durability
The pellet durability (Table ) from the impact test was highest for the control pellets at 100 %. The seven formulation mixes had reasonable durability values with the lowest for Mix 5 having a durability of 96.9 %. The higher durability may be attributed to combinations of elevated temperature (90 °C), compaction pressure, moisture content (14 wt. %), binding agents (WGP and GG) and proteins associated with the vegetable feed ingredients as they act as additional binding agents. During elevated temperature processing, the moist ingredients have a tendency for protein denaturation. Denaturation is a phenomenon in which the three-dimensional protein structure breaks down and during cooling, the proteins re-combine and form new bonds with neighboring feed ingredients (Thomas, Van Vliet, & Van der Poel, Citation1998). In addition, during compaction, the ingredients were physically deformed under elevated temperature and formed particle-to-particle bonding. The heating helped with thermal softening and improved adhesive interactions of the amorphous feed materials (cellulose, lignin, hemicellulose and starch) which occurred at or close to the glass transition temperatures (Tg) (Tilay, Azargohar, Drisdelle, Dalai, & Kozinski, Citation2015). In general, the Tg for cellulose, lignin and hemicellulose have been reported to be >100 °C, 50 °C to 100 °C and 40 °C, respectively (Furuta, Aizawa, Yano, & Norimoto, Citation1997).
Moisture also helps with durability of compressed biomass pellets by forming van der Waal forces and hydrogen bonds between proteins (Thomas et al., Citation1998). For example, one study produced canola meal pellets with low and high moisture contents. For the same amount of binder, pellets containing 8% moisture content produced pellets with lower durability then with pellets containing 10–12% moisture (Tilay et al., Citation2015). In this study, Mix 3 had the highest durability which is possibly due to the interaction between the moisture and the ISP. (Buchanan & Moritz, Citation2009) determined pellets made from corn/soybean meal improved in durability when 5 wt. % ISP was added to the mixture possibly due to the moisture contained in the pellets. In addition to moisture content, the amount of binding agents (type not disclosed) was also observed to be important for producing canola meal pellets. Maintaining the moisture constant between 8–12%, the pellet durability reduced when 2 wt. % binder was added, while it improved with 5 wt. % binder (Tilay et al., Citation2015). In this study, WGP was added as a binder which helped with the adhesive interactions for the plant-based ingredients. The Tg of dry wheat gluten protein was reported to be 162–187 °C. However, by increasing moisture content to about 14 %, the Tg values were reported to decrease as low as 50 °C (Pouplin, Redl, & Gontard, Citation1999).
3.4. Pellet disintegration in water
All pellet specimens sank to the bottom of the beaker. The time for the pellets to begin disintegration was recorded for a maximum time of 35 min. Non-quantitative observations showed the control and Mix 3 pellets had good physical integrity since they did not disintegrate and remained intact for the duration of the test, while Mix 2 and Mix 5 pellets disintegrated the fastest in less than 1 and 5 min., respectively. Pellet type from Mix 1, 4, 6, and 7 began to disintegrate after 20, 27, 23 and 16 min., respectively, which fall within the feeding window of 15 min. Observing Mix 2 with 60 wt. % CM and 5 wt. % ISP, while Mix 3 contained 5 wt. % CM and 60% ISP. Correspondingly, Mix 2 contained higher amounts of CM. Similarly, looking at Mix 5, it contained 30% CM and 20 wt. % ISP, while Mix 6 contained 20 wt. % CM and 30 wt. % ISP. These outcomes suggest that the CM increased the disintegration rate of the pellets much more than the ISP. The results corroborate with other studies where the addition of CM in fishmeal displayed significantly lower water stability (Cruz-Suarez, Ricque-Marie, Tapia-Salazar, McCallum, & Hickling, Citation2001). This can be due to the partial presence of oil in the CM (remaining after oil extraction) that can adversely affect the pellet disintegration.
3.5. Transverse tensile strength
The formulations effect on the crushing strength of the generated pellets were evaluated using ANOVA (Table ) and the results were ranked (Figure ) (95% confidence level). According to the results, formulation as a factor had a significant effect on the crushing strength of the pellets. All formulations resulted in pellets with less strength compared with the control, however, formulation number three showed the highest strength among all formulations that was statistically significant (Figure ). The control pellet was stronger than any of the mixes studied with a load of 48.33 ± 2.89 N. The strengths of the mixes were significantly increased by inclusion of ISP. For example, Mix 3 (with highest ISP (60 wt. %) content) had a superior strength compared to all formulated mixes with a failure load of 31.67 ± 4.73 N, while Mix 6, with 30 wt. % ISP was observed to have the second strongest pellets with a failure load of 22.83 ± 3.25 N. Mix 2 had the lowest strength of 6.60 ± 2.23 N where it contained the highest loading of CM (60 wt. %) with 5 wt. % ISP. Addition of ISP may have contributed to the strength of the pellets. This may be explained by the larger contents of protein and protein-to-protein interactions when soy products are incorporated (Brewer, Potter, Sprouls, & Reinhard, Citation1992).
Figure 4. Tensile strength of different pellet formulation types; a, b, c are ranked results of the formulations.
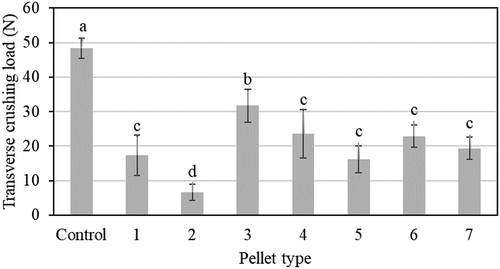
Table 4. ANOVA results of the crushing strength of the pellets from different formulations
4. Conclusion
The effects of vegetable ingredients on the processing conditions were investigated for the quality of compressed pellet fish feed. The combination of moisture content, heating and densification pressure used in this study are encouraging for producing single pellets. The fishmeal ingredients were verified with SEM and revealed various sizes, shapes and geometries, which could be beneficial for densification purposes. The density of the fish pellets in this study ranged from 1110–1280 kg m−3. In future work the density of the pellets could be adjusted to targeted species for buoyancy purposes. The Mix 3 had a better durability, water stability (water disintegration) and transverse crushing load, most likely due to the high loading of ISP and the protein-to-protein interactions. Adding CM was found to decrease the strength and durability of pellets and had a quick water disintegration time. It is recommended to use CM in minimum amounts for fish feed pellets. As an extension of this research, for potential commercial applications, the use of an extruder would industrialize the process, which will involve knowledge of the extrusion processing parameters. A long-term goal would be to feed trout selected mix diets and measure the effect of growth. It is anticipated, the agricultural products from Saskatchewan, Canada could be an alternative to a nutritious and cheaper fishmeal.
Disclaimers
This manuscript is original work and is not under review elsewhere or published elsewhere.
Acknowledgements
The authors would like to acknowledge financial support of the Natural Sciences and Engineering Research Council of Canada (NSERC) under the Discovery Grant (RGPIN 418729) and the University of Saskatchewan Undergraduate Student Research Assistantship.
Additional information
Funding
Notes on contributors
Duncan Cree
Duncan Cree is an assistant professor in the Department of Mechanical Engineering at the University of Saskatchewan, Saskatoon, Canada. His research focuses on manufacturing and characterizing bio-composites produced from sustainable materials. Composite material components/ingredients can be custom-builtfor specific applications as they possess a high level of tailorability. Dr. Cree has worked with epoxy/flax fiber composites for possible applications as roof shingles, sandwich panel skins, container walls and access door construction. His other interests are to address the challenges of sustainability using waste as resources. The addition of waste eggshells as filler materials in polymers could be used as partial or total replacements for natural mined limestone for unique applications. The current project promotes new uses of agricultural plant materials with focus on sustainability.
References
- Adelizi, P. D., Rosati, R. R., Warner, K., Wu, Y. V., Muench, T. R., White, M. R., & Brown, P. B. (1998). Evaluation of fish-meal free diets for rainbow trout, Oncorhynchus mykiss. Aquaculture Nutrition, 4(4), 255–14. doi:10.1046/j.1365-2095.1998.00077.x
- Al-Widyan, M. I., & Al-Jalil, H. F. (2001). Stress-density relationship and energy requirement of compressed only cake. Applied Engineering in Agriculture, 17(6), 749–753.
- Apper-Bossard, E., Feneuil, A., Wagner, A., & Respondek, F. (2013). Use of vital wheat gluten in aquaculture feeds. Aquatic Biosystems, 9(1), 1–13. doi:10.1186/2046-9063-9-1
- Barrows, F. T., Gaylord, T. G., Sealey, W. M., Porter, L., & Smith, C. E. (2008). The effect of vitamin premix in extruded plant-based and fish meal based diets on growth efficiency and health of rainbow trout, Oncorhynchus mykiss. Aquaculture, 283(1–4), 148–155. doi:10.1016/j.aquaculture.2008.07.014
- Brewer, M., Potter, S. M., Sprouls, G., & Reinhard, M. (1992). Effect of soy protein isolate and soy fiber on color, physical and sensory characteristics of baked products. Journal of Food Quality, 15(4), 245–262. doi:10.1111/jfq.1992.15.issue-4
- Brinker, A., Koppe, W., & Rösch, R. (2005). Optimizing trout farm effluent treatment by stabilizing trout feces: a field trial. North American Journal of Aquaculture, 67(3), 244–258. doi:10.1577/A04-078.1
- Buchanan, N. P., & Moritz, J. S. (2009). Main effects and interactions of varying formulation protein, fiber, and moisture on feed manufacture and pellet quality. The Journal of Applied Poultry Research, 18(2), 274–283. doi:10.3382/japr.2008-00089
- Cruz-Suarez, L. E., Ricque-Marie, D., Tapia-Salazar, M., McCallum, I. M., & Hickling, D. (2001). Assessment of differently processed feed pea (Pisum sativum) meals and canola meal (Brassica sp.) in diets for blue shrimp (Litopenaeus stylirostris). Aquaculture, 196(1–2), 87–104. doi:10.1016/S0044-8486(00)00572-X
- Daniel, N. (2018). A review on replacing fish meal in aqua feeds using plant protein sources. International Journal of Fisheries and Aquatic, 6, 164–179.
- Food and Agriculture Organization (FAO) 2018a. The state of world fisheries and aquaculture 2018. Meeting the sustainable development goals (p.2). Rome, 2018.
- Food and Agriculture Organization (FAO) 2018b. The state of world fisheries and aquaculture 2018. Meeting the sustainable development goals (p.45). Rome, 2018.
- Furuta, Y., Aizawa, H., Yano, H., & Norimoto, M. (1997). Thermal-softening properties of water-swollen wood, IV: The effects of chemical constituents of the cell wall on the thermal-softening properties of wood. Mokuzai Gakkaish (in Japanese), 43, 725–730.
- Hardy, R. W., & Sullivan, C. V. (1983). Canola meal in rainbow trout (Salmo gairdneri) production diets. The Canadian Journal ofFisheries and Aquatic Sciences, 40(3), 281–286. doi:10.1139/f83-042
- Hixson, S. M., Parrish, C. C., Wells, J. S., Winkowski, E. M., Anderson, D. M., & Bullerwell, C. N. (2016). Inclusion of camelina meal as a protein source in diets for farmed salmonids. Aquaculture Nutrition, 22(3), 615–630. doi:10.1111/anu.2016.22.issue-3
- Jobling, M. (2012). National research council (NRC): Nutrient requirements of fish and shrimp. Aquaculture International, 20(3), 601–602. doi:10.1007/s10499-011-9480-6
- Jurij, W., Powell, M., Rodnick, K., Overturf, K., Hill, R. A., & Hardy, R. (2012). Dietary protein source significantly alters growth performance, plasma variables and hepatic gene expression in rainbow trout (Oncorhynchus mykiss) fed amino acid balanced diets. Aquaculture, 356, 223–234.
- Kambo, H. S., & Dutta, A. (2014). Strength, storage, and combustion characteristics of densified lignocellulosic biomass produced via torrefaction and hydrothermal carbonization. Applied Energy, 135, 182–191. doi:10.1016/j.apenergy.2014.08.094
- Knights, B. (1983). Food particle-size preferences and feeding behaviour in warmwater aquaculture of European eel, Anguilla anguilla (L.). Aquaculture, 30(1–4), 173–190. doi:10.1016/0044-8486(83)90160-6
- Kyeong-Jun, L., Powell, M. S., Barrows, F. T., Smiley, S., Bechtel, P., & Hardy, R. W. (2010). Evaluation of supplemental fish bone meal made from Alaska seafood processing byproducts and dicalcium phosphate in plant protein based diets for rainbow trout (Oncorhynchus mykiss). Aquaculture, 302(3–4), 248–255. doi:10.1016/j.aquaculture.2010.02.034
- Lehtikangas, P. (2001). Quality properties of pelletised sawdust. Logging Residues and Bark. Biomass and Bioenergy, 20(5), 351–360. doi:10.1016/S0961-9534(00)00092-1
- Obaldo, L. G., Divakaran, S., & Tacon, A. G. (2001). Method for determining the physical stability of shrimp feeds in water. Aquaculture Research, 33(5), 369–377. doi:10.1046/j.1365-2109.2002.00681.x
- Obernberger, I., & Thek, G. (2004). Physical characterisation and chemical composition of densified biomass fuels with regard to their combustion behaviour. Biomass and Bioenergy, 27(6), 653–669. doi:10.1016/j.biombioe.2003.07.006
- Ortiz, S. E. M., Adriana, M., Ednelí, S. M. Q., Trindade, M. A., Santana, A. S., & Favaro-Trindade, C. S. (2009). Production and properties of casein hydrolysate microencapsulated by spray drying with soybean protein isolate. LWT - Food Science and Technology, 42(5), 919–923. doi:10.1016/j.lwt.2008.12.004
- Phani, A., Tabil, L., & Schoenau, G. (2009). Compaction characteristics of barley, canola, oat and wheat straw. Biosystems Engineering, 104(3), 335–344. doi:10.1016/j.biosystemseng.2009.06.022
- Pouplin, M., Redl, A., & Gontard, N. (1999). Glass transition of wheat gluten plasticized with water, glycerol, or sorbitol. The Journal of Agricultural and Food Chemistry, 47(2), 538–543. doi:10.1021/jf980697w
- Stelte, W., Niels, P. K., Nielsen, H. O. H., Dahl, J., Shang, L., & Sanadi, A. R. (2013). Reprint of: Pelletizing properties of torrefied wheat straw. Biomass and Bioenergy, 53, 105–112. doi:10.1016/j.biombioe.2013.03.012
- Storebakken, T. (1985). Binders in fish feeds: I. Effect of alginate and guar gum on growth, digestibility, feed intake and passage through the gastrointestinal tract of rainbow trout. Aquaculture, 47(1), 11–26. doi:10.1016/0044-8486(85)90004-3
- Sudhagar, M., Tabil, L. G., & Sokhansanj, S. (2006). Effects of compressive force, particle size and moisture content on mechanical properties of biomass pellets from grasses. Biomass and Bioenergy, 30(7), 648–654. doi:10.1016/j.biombioe.2005.01.004
- Temmerman, M., Rabier, F., Jensen, P. D., Hartmann, H., & Böhm, T. (2006). Comparative study of durability test methods for pellets and briquettes. Biomass and Bioenergy, 30(11), 964–972. doi:10.1016/j.biombioe.2006.06.008
- Thomas, M., Van Vliet, T., & Van der Poel, A. F. B. (1998). Physical quality of pelleted animal feed 3. Contribution of feedstuff components. Animal Feed Science and Technology, 70(1–2), 59–78. doi:10.1016/S0377-8401(97)00072-2
- Tilay, A., Azargohar, R., Drisdelle, M., Dalai, A., & Kozinski, J. (2015). Canola meal moisture-resistant fuel pellets: Study on the effects of process variables and additives on the pellet quality and compression characteristics. Industrial Crops and Products, 63, 337–348. doi:10.1016/j.indcrop.2014.10.008
- Tusche, K., Arning, S., Wuertz, S., Susenbeth, A., & Schulz, C. (2012). Wheat gluten and potato protein concentrate-Promising protein sources for organic farming of rainbow trout (Oncorhynchus mykiss). Aquaculture, 344, 120–125. doi:10.1016/j.aquaculture.2012.03.009
- Zhang, Y., Øverland, M., Shearer, K. D., Sørensen, M., Mydland, L. T., & Storebakken, T. (2012). Optimizing plant protein combinations in fish meal-free diets for rainbow trout (Oncorhynchus mykiss) by a mixture model. Aquaculture, 360, 25–36. doi:10.1016/j.aquaculture.2012.07.003