Abstract
This study is conducted at Haramaya University Raaree research site during 2013/2014 main cropping season, eastern Ethiopia, to investigate the impact of brewery sludge on haricot production and soil fertility. The treatments comprised seven levels of brewery sludges (0, 2.0, 4.0, 6.0, 8.0, 10.0 and 12.0 t ha−1) and NP inorganic fertilizer at recommended rate, arranged in randomized complete block design with three replications. In this regard, the maximum increase (58.28% and 73.05% and 43.15% over the control) in survival percentage of plant, plant height, and leave length were recorded by the application of sludge at 6 and 12 t ha−1. Similarly, maximum number of pods per plant (22.90) and seeds per pod (5.40) were recorded when brewery sludge was applied at 10 t ha−1 and 6 t ha−1, respectively. The soil of the experimental site revealed 1.18% of organic carbon and 7.51 mg kg−1 available phosphorus. Moreover, the source brewery sludge (BS) which is used as treatments in this study showed 3.50% of organic carbon and 39.75 mg kg−1 available phosphorus. The total effective nodules per hectare were non-significant and the maximum (261,500) was observed in plot received 4 t ha−1 brewery sludge and the minimum (147,750) was recorded in control and NP fertilized plots. The maximum grain and total N uptakes due to brewery sludge application were obtained at the higher brewery sludge rates (10 and 12 t ha−1) whereas the minimum grain, straw and total N uptake were obtained from control plot.
Keywords:
PUBLIC INTEREST STATEMENT
With a rapidly increasing population and a growing trend of industrial development, problems related to the management of industrial wastes have become of considerable magnitude. One way of reutilizing such byproducts is application on cultivated lands as fertilizer for improvements of soil fertility and crop production which is also a common practice in many countries. This practice stands out as a way to reduce soil fertility depletion and input cost for smallholder farmers. Soil fertility depletion is a serious problem in eastern Ethiopia highlands and use of brewery sludge may contribute to halt the problem of soil fertility and enhance crop production. Therefore, this experiment was carried out with the objectives of investigating the impact of industrial waste sludge on haricot bean crop production and soil fertility.
1. Introduction
Approximately 6.5 billion people currently live on our planet, and by 2050, that number is projected to rise by almost 50% to over 9 billion which need to give high attention on high-quality crops to provide for this growing demand (Merga & Haji, Citation2019a). Although the Harar city cannot be considered among the growing industrial cities in the country, the Harar Beer Factory has been expanding in its production capacity recently. Accordingly, the current daily sludge production of the Brewery has increased significantly along with the expansion and increase in its beer production. It is therefore inevitable to focus on the development of appropriate industrial waste management strategy and management plan that enables to utilize the industrial liquid waste for other purposes in an environmentally friendly manner. The reutilization of such waste product can be linked with minimized losses of resources and production opportunities and with increased productivity and profitability along with environmental benefits.
In the Ethiopia crop agriculture, pillar in balancing the food diet of the majority people, as a whole and the cereals specifically is facing serious and vast challenges which in turn affect the supply of food grains (Merga & Haji, Citation2019b). One way of utilizing such by-products is an application on cultivated lands as fertilizer for improvements of soil fertility and crop production, which is also a common practice in many countries. This practice stands out as a way to reduce soil fertility depletion and input cost for smallholder farmers. This also avoids final destination options such as incineration and disposal in landfills that involve higher production costs on the factory and greater impact on the environment.
Brewery sludge’s are usually rich in organic matter and essential nutrients. As a result, they have great potential for use as fertilizers and soil conditioners, and when they meet the necessary requirements concerning the concentration of heavy metals and pathogens, can replace part or all of mineral fertilizers (Mutz, Hengevoss, Hugi, & Gross, Citation2017). There are scientific evidences of increase in productivity of different crops with the application of brewery sludge (Mills, Pearce, Farrow, Thorpe, & Kirkby, Citation2014; Mutz et al., Citation2017). The benefits of application of bio-solids can exceed those achieved with mineral fertilizers, especially in terms of productivity and economic with fertilizers, mainly nitrogen (N) and in some cases phosphorus (P) and metallic ions like calcium (Ca) and magnesium (Mg). However, studies on the viability of brewery sludge from industries for agricultural use are few and specific for certain industrial byproducts such as tannery and coal (Konrad & Castilhos, Citation2002; Smith, Brown, Ogilvie, Rushton, & Bates, Citation2001). Nevertheless, industrial sludge wastes are diverse, with characteristics that vary according to the raw materials and manufacturing processes/processing systems followed. These justify the need for investigating the technical feasibility, determining the rates of application, evaluating agronomic performances and economic benefits, and/or assessing environmental safety of major industrial wastes. Moreover, the response to the different brewery sludge wastes vary depending on the type of crop species, soil types and other environmental factors such climate particularly soil moisture level during the crop growing period.
Farmlands in the Harari People Regional State (HPRS) and East Hararghe Zone of Oromia Regional State are known for low levels of productivity associated with their poor soil fertility as a result of continuous cultivation and little or no use of inorganic or organic fertilizers to maintain soil fertility mainly because of shortage of farmyard manure and high prices of chemical fertilizers. In Ethiopia, haricot bean is one of the most economically important cash crops grown by small-scale farmers (Ferris & Kaganzi, Citation2008).
Haricot bean is produced on 329,824 hectares of land and 543,984 tones totally, while the mean yield obtained was 1649.3 kg ha−1 during the 2017 cropping season in Ethiopia (Food and Agriculture Organization [FAO], Citation2019). Some farmers in the Harari People Regional State of Ethiopia have already started using the brewery sludge by-product (sludge) of the Harar Brewery as a fertilizer input for crop production. However, there is little scientific study carried out yet to investigate the impact of brewery waste (sludge) on the haricot bean crop production and soil fertility improvement. Therefore, the objective of this study is to assess the impact of the Harar Beer Brewery sludge on haricot bean production and soil fertility. Study on impacts of beer brewery sludge is of paramount importance for further studies related to the technical feasibility, rates of applications for major crops on different soil types, agronomic and economic importance as well as environmental impacts of the industrial wastes of the Harar Brewery.
2. Materials and methods
2.1. Description of the study site
The Harar Beer Brewery is located in Harar City, the capital of the Harari People National Regional State (HPNRS). The region is situated in the eastern part of the Ethiopia at about 500 km road distance east of the capital city, Addis Ababa. Geographically, the Harari Region is located between 9° 11ʹ 49” and 9° 24ʹ 42” North latitude and between 42° 03ʹ 30” and 42° 16ʹ 24” East longitude. With this location, the Region enjoys a suitable climate which has favored agricultural development-based settlement and civilization of long history. Harar, the capital of the region is located at 09° 18ʹ 43” North and 42° 07ʹ 23” East and 515 km East of Addis Ababa.
The total land area of the Harari region is estimated at 343.2 km2 (34,320 ha) out of which the rural area accounts for 323.7 km2 (94.3%) and the remaining 19.5 km2 (1,950 ha) accounts for the urban area, particularly the area of the Harar city which is the regional capital. The Harari Regional State consists of 6 urban and 3 rural districts. The rural district is Sofi. Farmer’s field in sofi district was used for haricot bean experiment. The study was conducted at Haramaya University Raaree research site 2013/2014 main cropping season, eastern Ethiopia, to investigate the impact of brewery sludge on haricot bean production and soil fertility.
2.2. Experimental procedures and methods
Planting of haricot bean at Haramaya University raaree research site was done in July 2013/2014 main cropping season. The variety of haricot bean used in this study was Awash-1 improved variety. The experiment consisted of eight treatments viz. applications of brewery sludge (BS) that collected from Harar Beer factory at seven levels (0.0, 2.0, 4.0, 6.0, 8.0, 10.0 and 12.0 t ha−1) and recommended rate of NP mineral fertilizer: N (92 kg N ha−1) and P (92 kg P2O5 ha−1) laid out in a randomized complete block design (RCBD) in three replications. It was planted on a plot size of 2.0 m x 3.6 m (7.2 m2) in rows of six per plot at a row spacing of 40 cm while 10 cm was between plants. There was 1 m spacing between plots and blocks. The total experimental area was 8.0 m x 35.8 m = 286.4 m2. The brewery sludge was applied immediately after seedling emergence and incorporated into the plow depth. This was decided as the team could not get the brewery sludge before or at planting from the Harar Brewery while on the other hand, the date of planting of these crops was getting too late. The data was collected from plants found in four internal rows.
Half of the N and the full rate of the P fertilizers on the plots of the fertilized treatments on both crops were applied 5 cm below the seed at time of planting as urea (46% N) and as triple super phosphate or TSP (20% P), respectively. Whereas the second half of the N fertilizer was applied 3–4 weeks after planting at 5–7 cm away from the plant as two side dressing at about 5 cm below the surface. When crops grown in the fields were matured all parameters or agronomic data was collected from the fields for all tested crops according to experimental plan.
2.3. Analytical procedure for soil, plant tissue and brewery sludge chemical properties
Soil samples were collected from all experimental plots and selected soil chemical parameters were analyzed in Haramaya University soil chemistry and central laboratories. The pH of the soils suspension in a 1:2.5 (soil: liquid ratio) was measured potentiometrically using a glass-calomel combination electrode (Zevenbergen, Van Reeuwijk, Bradley, Bloemen, & Comans, Citation1996). The Walkley and Black (Citation1934) wet digestion method was used to determine soil carbon content Total N was analyzed using the Kjeldahl digestion, distillation and titration method as described by Black, Evans, and Dinauer (Citation1965) by oxidizing the organic matter in concentrated sulfuric acid solution (0.1 N H2SO4). Since pH of the soil in the study area ranges from 8.49 to 8.67, available P of soils and brewery sludge was analyzed according to the standard procedure of Olsen (Citation1954). Extractable heavy metals (Fe, Cu, Zn, Mn, Cr, Mo, Co, Pb, Se and Cd) was extracted by DTPA extraction method (Lindsay & Norvell, Citation1978) and all these heavy metals were measured by atomic absorption spectrophotometer.
2.4. Statistical analysis
The data were subjected to analysis of variance (GLM procedure) using SAS software program version 9.1. From the analysis of variance, treatment means that exhibited significant differences was separated using Tukey test at 5% level of significance to determine the homogeneous subsets and to reveal which among the treatments are comparable and the level of significance among treatments.
3. Results and discussions
3.1. Growth and yield components of haricot bean
Analysis of variance revealed that survival percentage of plant, plant height, leave length, pods per plant, seeds per pod, total biomass, grain yield and 1000-grain weight were significantly (P ≤ 0.05) affected by sludge applications whereas pod length, seeds per pod and total effective nodules per plot were not significantly (P ≤ 0.05) affected by sludge applications. The survival percentage of plant was calculated from the number plants reached physiological maturity dividing by full number of plants that had on the plot during germination period (144 plants per plot). Accordingly, 6 t/ha of brewery waste sludge revealed high survival percentage of plant (83) while the control treatment showed least, which is only 52.4 (Table ).
Table 1. Mean growth and yield components of haricot bean
The mean of separation indicated that applications of sludge significantly increased the survival percentage of plant, plant height; leave length, pods per plant, biomass, dry weight and yield and 1000-grain weight over control and NP fertilized plots (Table ). In this regard, the maximum increase (58.28% and 73.05% and 43.15% over control) in survival percentage of plant, plant height, and leave length were recorded by the application of sludge at 6 and 12 t ha−1. Similarly, maximum number of pods per plant (22.90) and seeds per pod (5.40) were recorded when brewery sludge was applied at 10 t ha−1 and 6 t ha−1, respectively. Whereas the lowest number of pods per plant and seeds per pod was obtained from the untreated plot, which was statistically at par with the application of NP fertilized plot (Table ). The total effective nodules per hectare were non-significant and the maximum (26,1500) was observed in plot received 4 t ha−1 brewery sludge and the minimum (14,7750) was recorded in control and NP fertilized plots.
Haricot bean gave highly significant response to sludge application. A comparison of maximum fresh weight with that of control and recommended rate of NP mineral fertilizer, revealed an increase of 165.91% and 123.71%, respectively, at 12 t ha−1 brewery sludge application (Table ).
The data regarding dry weight of plants per hectare also showed significant results at varying levels of brewery sludge application. It is evident from the data that the maximum dry weight (67.75) was recorded at 10 t ha−1 brewery sludge application, which was at par with the application of sludge at 12 t ha−1 whereas the minimum was obtained from control which was statistically at par with recommended rate of NP mineral fertilizer.
Total biomass of haricot bean increased significantly (P < 0.05) over control and Recommended rate of NP mineral fertilizer with the use of sludge. The maximum total biomass weight (6045.00 kg ha−1) was recorded when 12 t ha−1 of brewery sludge applied which was at par with the application of 10 t ha−1 of brewery sludge. On the other hand, the minimum (1,858.95 kg ha−1) was obtained from the control treatment (Table ). The reason for this increase might be the efficient use of all available resources by the plants because of low and continued supply of nutrients as well as more water absorption. As far as 1000-grain weight is concerned, the produce at various levels of sludge revealed that the maximum 1000-seeds weight (372.5 g) was produced when brewery sludge was applied at 6 t ha−1 followed by 4 and 8 t ha−1 brewery sludge application whereas the minimum (317.50 g) 1000-seeds weight was observed in control (Table ).
Results revealed a highly significant (P < 0.001) increase in grain yield of haricot bean among different levels of brewery sludge application (Table ). However, increasing brewery sludge levels from 10 to 12 t ha−1 had no significant effect on haricot bean grain yield (Table ). The haricot bean grown gave a significant grain yield of 5987.0, 5956.9, 4935.8 kg ha−1 at application of 12, 10 and 8 t ha−1, in that order. This resulted in 225%, 223% and 168% more grains over control and 112%, 111% and 75% over NP applied (Table ). This result indicated that application of brewery sludge increased availability of essential nutrients and moisture to the growing plants and in turn improved the growth performance and yield of haricot bean. The maximum (5987.0 kg ha−1) yield was recorded in 12 t ha−1 brewery sludge application followed by 10 t ha−1 and then 8 t ha−1 of brewery sludge application. On the other hand, the lowest (1,842.2 kg ha−1) grain yield of haricot bean was recorded from the control plot. 12 t ha−1 brewery sludge application increased haricot bean grain yield by about 325% over the control. Application of recommended rate of NP mineral fertilizers gave 2815.5 kg ha−1 of grain yield which is lower than the yield recorded for the lowest level of brewery sludge used. The present investigations have demonstrated the improvement of haricot bean growth performance and grain yield by the application of lowest rate of brewery sludge when they were compared with recommended rate of NP mineral fertilizer. The result showed that it is quite possible to increase haricot bean grain yield by the use of 10 t ha−1 of brewery sludge.
Means with the same letter(s) within a column are not significantly different at 5% level of significance. LSD (5%) = Least significant difference at p = 0.05, and CV (%) = Coefficient of variation
3.2. Effect of brewery sludge on heavy metals accumulation in soil
3.2.1. Selected chemical properties of soil and brewery sludge used
Some chemical properties of brewery sludge used for the experiment as treatments and soil of the experimental site are given in Table . The soil of the experimental site revealed 1.18% of organic carbon and 7.51 mg kg−1 available phosphorus. Moreover, the source brewery sludge (BS) which is used as treatments in this study showed 3.50% of organic carbon and 39.75 mg kg−1 available phosphorus.
Table 2. Chemical properties of soil and brewery sludge used for experiment
3.2.2. Effect of brewery sludge (BS) on agricultural soils
As evident from the data presented in Table and , accumulation of heavy metal ions in the soil due to brewery sludge and recommended rate of NP mineral fertilizer were below the tolerable value for most of the metal ions except for Co and Se metal ions. In general, the highest accumulation of Zn (18 mg kg−1), Fe (25.76 mg kg−1) at application of 10 t ha−1, Cd (9.18 mg kg−1) at application of 8 t ha−1, Ni (27.67 mg kg−1) at application of 6 t ha−1, Mn (4.05 mg kg−1) at application of 6 t ha−1, Co (34 mg kg−1) at application of 6 t ha−1 which is higher than indicated tolerable level of 20 mgkg−1, Cu (6.50 mg kg−1) without application of brewery sludge but recommended rate of NP mineral fertilizer, Se (12.87 mg kg−1) at application of 10 t ha−1 and in fact very high for control plots compared to tolerable level of 2 mg kg−1, Mo (1.73 mg kg−1) at application of 10 t ha−1 and Cr (1.50 mg kg−1) at application of recommended rate of NP mineral fertilizer followed by application of 12 t ha−1 of brewery sludge were recorded in the soil treated with different rates of sludge and recommended rate of NP mineral fertilizer (Table and ). Se and Co were already high in the control soil. Sludge application does not significantly increase the heavy metals concentration. The heavy metals concentrations after the sludge application are comparable with the ones after application of an NP fertilizer, and in some cases lower.
Table 3. Mean concentration of heavy metals (mg kg−1) in the dry soil before application of treatments and brewery sludge used for the experiment
Table 4. Accumulation of heavy metals in the soil after haricot bean harvest
Brewery sludge’s (BS) are primarily organic solid products, produced by wastewater treatment processes that can be beneficially recycled (Weggler, McLaughlin, & Graham, Citation2004). Application of sewage sludge serves numerous functions including macro and micronutrients supplement to crops, ameliorating the soils physical properties and augmenting the organic matter content in soil; all contributing to an increment in crop production (Hussein, Citation2009). Land application of brewery sludge materials is a common practice in many countries that allow the reuse of brewery sludge. It is estimated that in the United States, more than half of approximately 5.6 million dry tones of sewage sludge used or disposed of annually is land applied, and agricultural utilization of brewery sludge occurs in every region of the country. In the European community, over 30% of the sewage sludge is used as fertilizer in agriculture (Silveira, Alleoni, & Guilherme, Citation2003). In Australia, over 175 000 tons of dry brewery sludge’s are produced each year by the major metropolitan authorities, and currently, most brewery sludge applied to agricultural land are used in arable cropping situations where they can be incorporated into the soil.
However, the application of numerous sludge (e.g., livestock manures, composts, and municipal sewage sludge) to land inadvertently may lead to the accumulation of heavy metals such as As, Cd, Cr, Cu, Pb, Hg, Ni, Se, Mo, Zn, Sb, and so forth, in the soil (Basta, Ryan, & Chaney, Citation2005). The potential of brewery sludge for contaminating soils with heavy metals has caused great concern about their application in agricultural practices (Canet, Pomares, Tarazona, & Estela, Citation1998). Heavy metals most commonly found in brewery sludge are Pb, Ni, Cd, Cr, Cu, and Zn, and the metal concentrations are governed by the nature and the intensity of the industrial activity, as well as the type of process employed during the brewery sludge treatment. Under certain conditions, metals added to soils in applications of brewery sludge can be leached downwards through the soil profile and can have the potential to contaminate groundwater (McLaren, Clucas, & Taylor, Citation2005).
Among organic fertilizers, those derived from sewage sludge often contain several heavy metals, including Cd (Mishima, Kimura, & Inoue, Citation2004), and the Cd concentrations can be substantial (Mori, Hojito, Kondo, & Matsunami, Citation2004). Fertilizer input is also an important source of heavy metal contamination in many countries, including Asian countries. For example, Cd from phosphate fertilizers is a major problem in New Zealand and Australia (Bolan et al., Citation2005). Proper management of sludge utilization must consider many aspects including its heavy metal content, crop type and its nutrient requirement, and biological and physic-chemical properties of soils. These aspects are essential to determine the optimum rate, time and method of sludge application.
Cereal crops grown on Cu deficient soils are occasionally treated with Cu as an addition to the soil, and Mn may similarly be supplied to cereal and root crops. Large quantities of fertilizers are regularly added to soils in intensive farming systems to provide adequate N, P, and K for crop growth. The compounds used to supply these elements contain trace amounts of heavy metals (e.g., Cd and Pb) as impurities, which, after continued fertilizer, application may significantly increase their content in the soil (Jones, Jarvis, Green, & Hayes Citation1981). Metals, such as Cd and Pb have no known physiological activity. Application of certain phosphate fertilizers in advertently adds Cd and other potentially toxic elements to the soil, including F, Hg, and Pb (Wuana & Okieimen, Citation2011). Long term and extensive use of land for agriculture with frequent application of agrochemicals is one of the major causes of trace metal, such as copper, nickel, zinc and cadmium, accumulation in soil. Widespread distribution of Cd and its high mobility makes it a potential contaminant in a wide range of natural environments. Generally, soil Cd concentrations exceeding 0.5 mg kg−1 are considered evidence of soil pollution. Phosphatic fertilizers are one of the most ubiquitous sources of Cd contamination in agricultural soils throughout the world (Zovko & Romic, Citation2011). Beside anthropogenic sources, trace metals can be also found in the parent material from which the soils developed. Whether the said inputs will become toxic and to what degree mobile depends on a number of factors; specific chemical and physical trace metal characteristics, soil type, land use, geomorphological characteristics within the soil type and exposure to emission sources. Processes that control the mobility, transformation and toxicity of metals in soil are of special importance in the soil root developing zone the rhizosphere (Zovko & Romic, Citation2011).
3.3. Nitrogen concentration in grain and straw of haricot bean
The grain and straw N contents were affected by the application of brewery sludge levels. Both the grain and straw N contents increased with each successive increase of brewery sludge from 0 to 4 and 6 to 8 t ha−1 whereas it decreased from 10 to 12 t ha−1. Accordingly, the highest grain N (3.369%) and straw N (2.604%) contents were obtained at the rate of 8 t ha−1 brewery sludge and the least were from the NP—fertilizer application for grain N and 6 t ha−1 brewery sludge for straw N contents (Table ). The crop grain N and straw N thus increased by 19.55% and 47.62%, respectively, in response to the application of 8 t ha−1 brewery sludge relative to the recommended rate of NP mineral fertilizer application and 6 t ha−1 brewery sludge in that order. Nevertheless, either of the grain or the straw N contents did not show any clear pattern of variation with the application of brewery sludge rate above 8 t ha−1 (Table ).
Table 5. Nitrogen concentration (%) of haricot bean in grain and straw
Means with the same letter(s) within a column are not significantly different at 5% level of significance. LSD (5%) = Least significant difference at p = 0.05, and CV (%) = Coefficient of variation
3.4. Grain, straw and total N uptake of haricot bean
Analysis of variance exhibited significant differences (P ≤ 0.05) among brewery sludge rate treatments for grain, straw and total N uptake of haricot bean. In this study grain N uptake of haricot bean for treatments ranged from 53.64 kg ha−1 (control) to 88 kg and 187.88 kg ha−1 (10 t ha−1) (Table ). Accordingly, the grain and straw N uptake were increased by 250% and 290%, respectively, and total N uptake increased by 260% in response to 10 t ha−1 relative to the control. The result clearly showed the positive effects of brewery sludge on haricot bean grain and straw yields and the improvement of grain and straw N contents by application of brewery sludge application. The grain N uptake of all brewery sludge rates was much higher than that of the straw uptake due to higher grain N content than the straw (Table ). Hence, the maximum grain and total N uptakes due to brewery sludge application were obtained at t higher brewery sludge rates (10 and 12 t ha−1) whereas the minimum grain, straw and total N uptake were obtained from control plot (Table ).
Table 6. Nitrogen uptake (kg ha−1) of haricot bean grain and straw
Means with the same letter(s) within a column are not significantly different at 5% level of significance. LSD (5%) = Least significant difference at p = 0.05, and CV (%) = Coefficient of variation
4. Conclusion
Haricot bean gave highly significant response to sludge application. A comparison of maximum fresh weight with that of control and recommended rate of NP mineral fertilizer, revealed an increase of 165.91% and 123.71%, respectively, at 12 t ha−1 brewery sludge application. The data regarding dry weight of plants per hectare also showed significant results at varying levels of brewery sludge application. It is evident from the data that the maximum dry weight (67.75) was recorded at 10 t ha−1 brewery sludge application, which was at par with the application of sludge at 12 t ha−1 whereas the minimum was obtained from control which was statistically at par with recommended rate of NP mineral fertilizer. Total biomass of haricot bean increased significantly over control and Recommended rate of NP mineral fertilizer with the use of sludge. The maximum total biomass weight (6,045.00 kg ha−1) was recorded when 12 t ha−1 of brewery sludge applied which was at par with the application of 10 t ha−1 of brewery sludge. On the other hand, the minimum (1,858.95 kg ha−1) was obtained from the control treatment. The reason for this increase might be the efficient use of all available resources by the plants because of low and continued supply of nutrients as well as more water absorption. The maximum grain and total N uptakes due to brewery sludge application were obtained at the higher brewery sludge rates (10 and 12 t ha−1) whereas the minimum grain, straw and total N uptake were obtained from control plot.
Intensification of crop farming with the use of high yielding crop varieties and high levels of fertilizers of both organic and inorganic sources preferably in an integrated manner along with other inputs and improved management practices is indispensible for attaining meaningful increments in crop yields and improvements in the living conditions of the farming communities. The fact that farmers of the areas have started using of the brewery waste product of the Harar Brewery without having any prior knowledge and/or information about its potential use for soil fertility improvement and increasing crop production and productivity is a proof of the critical soil fertility problem.
Competing interest
The authors declare no competing interests.
Correction
This article has been republished with minor changes. These changes do not impact the academic content of the article
Acknowledgements
The authors sincerely acknowledge the Harar Beer Brewery for financing the study that involves field and laboratory evaluation of the brewery sludge by-product of its Beer Manufacturing Plant. The Haramaya University signed the agreement and assigned us to implement the study. Thus, the University, particularly the Office of Vice President for Enterprise Development and Community Engagement deserves special appreciation for these as well as for administering the project budget and facilitating the overall execution of the investigation. The authors would also like to express their gratitude to the farming community as well as researchers and technical staff members of the University who in one way or another assisted and/or collaborated with them.
Additional information
Funding
Notes on contributors
Abdulatif Ahmed
Abdulatif Ahmed is a senior Instructor in the School of Plant Science under Agronomy Programme at Haramaya University of Ethiopia. His academic rank is assistant professor (PhD in Crop Science).
Muktar Mohammed
Muktar Mohammed currently works at Oda Bultum University, Ethiopia. He is doing research in natural resources management, environmental protection, soil science, agroforestry, rehabilitation of degraded land, vegetation ecology, climate smart agriculture, and Remote Sensing.
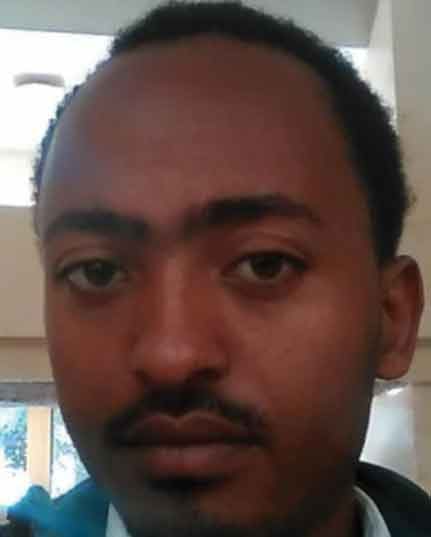
Bulti Merga
Bulti Merga is a lecturer and researcher in School of Plant Sciences, Haramaya University, Ethiopia. His profession is Horticulture and Agronomy, and Coordinator for Highland Pulse Crops Research Improvement Programmee at same academic institution. His key research area is on potato, carrot, pulses, and other vegetable crops research improvement.
References
- Basta, N. T., Ryan, J. A., & Chaney, R. L. (2005). Trace element chemistry in residual-treated soil. Journal of Environmental Quality, 34(1), 49–12. doi:10.2134/jeq2005.0049dup
- Black, C. A., Evans, D. D., & Dinauer, R. C. (1965). Methods of soil analysis (Vol. 9, pp. 653–708). Madison, WI: American Society of Agronomy.
- Bolan, N. S., Adriano, D. C., Naidu, R., La Mora, D., Luz, M., & Santiagio, M. (2005). Phosphorus-trace element interactions in soil-plant systems (No. phosphorusagric, pp. 317-352). American Society of Agronomy, Crop Science Society of America, and Soil Science Society of America.
- Canet, R., Pomares, F., Tarazona, F., & Estela, M. (1998). Sequential fractionation and plant availability of heavy metals as affected by sewage sludge applications to soil. Communications in Soil Science and Plant Analysis, 29(5–6), 697–716. doi:10.1080/00103629809369978
- Ferris, S., & Kaganzi, E. (2008). Evaluating marketing opportunities for haricot beans in Ethiopia (IPMS Working Paper 7). Nairobi (Kenya): ILRI.
- Food and Agriculture Organization (FAO). (2019). FAOSTAT statistical database of the United Nation food and agriculture organization (FAO) statistical division. Rome.
- Hussein, A. H. A. (2009). Impact of sewage sludge as organic manure on some soil properties, growth, yield and nutrient contents of cucumber crop. Journal of Applied Sciences, 9(8), 1401–1411. doi:10.3923/jas.2009.1401.1411
- Jones, L. H. P., Jarvis, S. C., Green, D. J., & Hayes, M. H. B. (1981). The fate of heavy metals in the chemistry of soil processes. New York.
- Konrad, E. E., & Castilhos, D. D. (2002). Soil chemical changes and corn growth as affected by the addition of tannery sludges. Revista Brasileira De Ciência Do Solo, 26(1), 257–265. doi:10.1590/S0100-06832002000100027
- Lindsay, W. L., & Norvell, W. A. (1978). Development of a DTPA soil test for zinc, iron, manganese, and copper 1. Soil Science Society of America Journal, 42(3), 421–428. doi:10.2136/sssaj1978.03615995004200030009x
- McLaren, R. G., Clucas, L. M., & Taylor, M. D. (2005). Leaching of macronutrients and metals from undisturbed soils treated with metal-spiked sewage sludge. 3. Distribution of residual metals. Soil Research, 43(2), 159–170. doi:10.1071/SR04109
- Merga, B., & Haji, J. (2019a). Economic importance of chickpea: Production, value, and world trade. Cogent Food & Agriculture, 5(1), 1615718. doi:10.1080/23311932.2019.1615718
- Merga, B., & Haji, J. (2019b). Factors impeding effective crop production in Ethiopia. Journal of Agricultural Science, 11(10), 1–14. doi:10.5539/jas.v11n10p1
- Mills, N., Pearce, P., Farrow, J., Thorpe, R. B., & Kirkby, N. F. (2014). Environmental & economic life cycle assessment of current & future sewage sludge to energy technologies. Waste Management, 34(1), 185–195. doi:10.1016/j.wasman
- Mishima, S. I., Kimura, R., & Inoue, T. (2004). Estimation of cadmium load on Japanese farmland associated with the application of chemical fertilizers and livestock excreta. Soil Science and Plant Nutrition, 50(2), 263–267. doi:10.1080/00380768.2004.10408476
- Mori, A., Hojito, M., Kondo, H., & Matsunami, H. (2004). Estimation of trace heavy metals balances in grassland and forage cropping areas of Japan: Loads from farmyard manure and uptake by grass and forage crops. Japanese Journal of Soil Science and Plant Nutrition (Japan).
- Mutz, D., Hengevoss, D., Hugi, C., & Gross, T. (2017). Waste-to-Energy options in municipal solid waste management: A guide for decision makers in developing and emerging countries. Deutsche Gesellschaft für Internationale Zusammenarbeit (GIZ) GmbH.
- Olsen, S. R. (1954). Estimation of available phosphorus in soils by extraction with sodium bicarbonate. United States Department of Agriculture, 939, 1–19.
- Silveira, M. L. A., Alleoni, L. R. F., & Guilherme, L. R. G. (2003). Biosolids and heavy metals in soils. Scientia Agricola, 60(4), 793–806. doi:10.1590/S0103-90162003000400029
- Smith, A., Brown, K., Ogilvie, S., Rushton, K., & Bates, J. (2001). Waste management options and climate change: Final report to the European Commission. In Waste management options and climate change: Final report to the European Commission. European Commission.
- Walkley, A., & Black, I. A. (1934). An examination of the Degtjareff method for determining soil organic matter, and a proposed modification of the chromic acid titration method. Soil Science, 37(1), 29–38. doi:10.1097/00010694-193401000-00003
- Weggler, K., McLaughlin, M. J., & Graham, R. D. (2004). Effect of chloride in soil solution on the plant availability of biosolid-borne cadmium. Journal of Environmental Quality, 33(2), 496–504. doi:10.2134/jeq2004.4960
- Wuana, R. A., & Okieimen, F. E. (2011). Heavy metals in contaminated soils: A review of sources, chemistry, risks and best available strategies for remediation. Isrn Ecology, 2011, 1–20. doi:10.5402/2011/402647
- Zevenbergen, C., Van Reeuwijk, L. P., Bradley, J. P., Bloemen, P., & Comans, R. N. J. (1996). Mechanism and conditions of clay formation during natural weathering of MSWI bottom ash. Clays and Clay Minerals, 44(4), 546–552. doi:10.1346/CCMN.1996.0440414
- Zovko, M., & Romic, M. (2011). Soil contamination by trace metals: Geochemical behaviour as an element of risk assessment. In Earth and environmental sciences. IntechOpen.