Abstract
Υ-aminobutyric acid (GABA) is a non-protein amino acid in a wide range of organisms. In plants, GABA is proposed to take multiple functions under non-stressed and stressed conditions. It is a key metabolite for primary and secondary pathways being an important intermediate of nitrogen metabolism and amino acid biosynthesis. In addition, the GABA metabolism through the GABA shunt provides a source for carbon skeletons and energy for down-stream biosynthetic pathways. GABA is also involved in signaling or regulatory mechanisms. It indirectly affects plant growth and development throughout the whole crop cycle and it accumulates rapidly in response to abiotic stresses. It has been shown to contribute to responses to biotic stresses through multiple mechanisms. An overactivation of the GABA shunt can help to restrict the spread of necrotrophic fungi like Botrytis. The activity of GABA against insects may be based either on a direct inhibitory effect or on an induction of down-stream defense reactions or on a combination of both mechanisms. The dual function of GABA as a metabolite and as a component of signaling pathways is a combination enabling plants to cope with different conditions. Exogenously applied GABA triggers similar effects than the intrinsic molecule and may, therefore, offer the potential to improve the overall vigor of plants.
PUBLIC INTEREST STATEMENT
GABA is a non-protein amino-acid that occurs naturally in plants, animals, and microorganisms, and has multiple metabolic and physiological functions in plants which positively impact vigor, growth and development and yield of plants in general and of agricultural crops in particular. The aim of this review is to present a brief overview on the metabolic pathway of GABA in plants and to compile the information on the metabolic and physiological effects of exogenously applied GABA in plants.
1. Introduction
Υ-aminobutyric acid (GABA) a non-protein amino acid is an important component of the free amino acid pool of living organisms. It can be found in all types of organisms including bacteria, fungi, plants and animals (Ramos-Ruiz, Poirot, & Flores-Mosquera, Citation2018). The enzymes involved in its metabolism are highly conserved across all organisms.
Several functions of GABA in plants have been described in detail (Podlesakova, Ugena, Spichal, Dolezal, & De Diego, Citation2019). Further to its metabolic role, GABA acts as an endogenous signaling molecule in plant growth regulation and plant development (Carillo, Citation2018; Fait, Fromm, Walter, Galili, & Fernie, Citation2008; Renault et al., Citation2011). In addition, it seems to be an important component in the regulation of carbon: nitrogen metabolism (Bouche & Fromm, Citation2004). There is strong evidence that GABA is involved in mediating tolerance towards a variety of environmental stresses such as low light, salinity, nitrogen starvation, drought or temperature (Kinnersley & Turano, Citation2000) and that it promotes plant growth and mitigates stress via up-regulating antioxidant defense systems and may therefore also contribute to the improvement of shelf life and crop quality during storage. The GABA metabolism has been shown to be involved in the recycling and reallocation of nitrogen during leaf senescence caused by abiotic stress (Jalil, Ahmad, & Ansari, Citation2017). In addition, it was demonstrated that it can also play a role in the defense of plants towards biotic stress caused by insects and necrotrophic fungi (Bown & Shelp, Citation2016; Seifi et al., Citation2013). The different roles of GABA in plants might be functionally linked and difficult to be separated from each other (Michaeli & Fromm, Citation2015).
The biochemical mode of action of GABA has been elucidated in detail (Michaeli et al., Citation2011; Shelp, Bown, & Zarei, Citation2017). Endogenous GABA is mainly formed from glutamate by the activity of the cytosolic enzyme glutamate decarboxylase (GAD). In the mitochondrial matrix, it is metabolized via the so-called GABA shunt which is associated with various physiological responses including the carbon flux into the tricarboxylic acid cycle (TCAC), the regulation of cytosolic pH, osmoregulation, and energy production and signaling. The connection of the GABA shunt with the TCAC finally links carbon and nitrogen metabolisms in plants.
The objective of this article is to provide an overview on the morphological and physiological effects in whole plants caused by exogenously applied GABA. The metabolic pathway of GABA in plants is presented and the metabolic changes induced by exogenous GABA are discussed.
2. The metabolic pathway of GABA in plants
Within plants GABA is mainly formed from glutamate by an irreversible reaction of cytosolic glutamate decarboxylase (GAD), alternatively through polyamine degradation (Yang, Yin, & Gu, Citation2015) or by a non-enzymatic reaction from proline under oxidative stress (Figure ). In Arabidopsis, five genes encoding GAD are expressed differently in different plant organs and their expression can change according to plant processes and growth conditions (Bouche & Fromm, Citation2004). GAD is a Ca2+ dependent calmodulin binding protein which is stimulated by changes of the cytosolic Ca2+ levels and neutral pH in intact plants (Snedden, Arazi, Fromm, & Shelp, Citation1995).
Figure 1. GABA metabolic pathway in plants. In plants, the glutamine-synthetase/glutamate-synthetase (GS/GOGAT) cycle is the principal nitrogen assimilation pathway into glutamate (Glu) and amino acids. The GABA shunt is composed of three enzymatic steps (blue). Glutamate decarboxylase (GAD) dependent on Ca2+-Calmodulin is located in the cytosol and catalyses the conversion of Glu into γ-aminobutyric acid (GABA). GABA permease (GABA-P) mediates the uptake of GABA into the mitochondrial matrix where it is subsequently converted into succinic semialdehyde (SSA) by GABA transaminase (GABA-T) with either α-ketoglutarate (α-KG) or pyruvate (Pyr) as amino acceptors. Succinic semialdehyde is then reduced by succinic semialdehyde dehydrogenase (SSADH) into succinate (Suc), a component of the tricarboxylic acid cycle (TCAC). Succinate and NADH are electron donors to the mitochondrial electron transport chain (ETC). Alternatively, succinic semialdehyde can be reduced to γ-hydroxybutyric acid by a succinic semialdehyde reductase (SSR) in the cytosol.
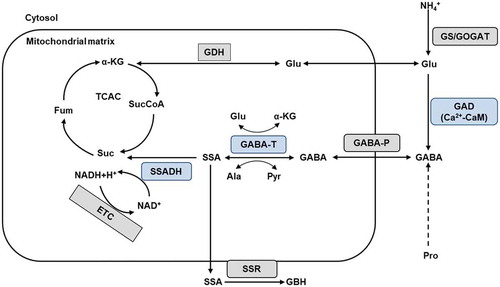
Experiments with pollen tube growth demonstrated a positive feedback control of GAD on Ca2+ channels which are dependent on extracellular GABA signals, i.e. through the application of GABA (Ling, Chen, Jing, Fan, & Wan, Citation2013; Yu, Zou et al., Citation2014). Consequently, changes of intracellular GABA levels induced the expression of signaling and metabolism-associated genes. After wounding of plant cells and subsequent release of the vacuolar content, GAD activation was shown to be Ca2+ independent through acidification of the cytosol (Carrol et al., Citation1994).
GABA permease (GABA-P) located in the mitochondrial membrane connects the GABA shunt and the TCA cycle to allow uptake of cytosolic GABA into mitochondria where finally energy and carbon skeletons are provided by the TCA cycle (Michaeli et al., Citation2011). GABA is metabolized via the GABA shunt which bypasses two steps in the TCA cycle from α-ketoglutarate via succinic-CoA to succinate (Bouche, Fait, Bouchez, Møller, & Fromm, Citation2003). In the mitochondrial matrix, GABA is metabolized to succinic semialdehyde (SSA) by GABA transaminase (GABA-T) (Renault et al., Citation2013). Two types of GABA-T use either α-ketoglutarate (GABA-TK) or pyruvate (GABA-TP) as amino acceptors, with the products glutamate or alanine. Subsequently, SSA is converted by SSA dehydrogenase (SSADH) to succinate, a component of the TCA cycle, and NADH. Both compounds are electron donors to the mitochondrial electron transport chain with the final product ATP. The negative feedback regulation of SSADH by NADH and ATP might be a possible explanation of how GABA is linked to other pathways than the TCAC, e.g. signaling pathways. Alternatively, SSA can be reduced to γ-hydroxybutyrate (GHB) by succinic semialdehyde reductase most likely located in the cytosol or in chloroplasts. GHB accumulates as a response to environmental stress (Allan, Simpson, Clark, & Shelp, Citation2008) and ssadh mutants of Arabidopsis accumulating GHB were shown to be less vigorous with reduced chlorophyll content and less flowers than the wild type (Bouche et al., Citation2003). The relationship between GABA and GHB is not yet clear. The bypass of the TCA cycle by the GABA shunt was demonstrated by inhibition of the relevant enzymes of the TCA cycle and the subsequent compensation through an increased flux through the GABA shunt (Araujo, Nunes-Nesri, Trenkamp, Bunik, & Fernie, Citation2008). Contrarily, mutants of Arabidopsis lacking the mitochondrial GABA transporter showed a reduced uptake of GABA into the mitochondria and an increased TCA cycle activity (Michaeli et al., Citation2011). In plants, aluminum-activated malate transporter proteins (ALMT) are assumed to act as GABA receptors inhibited by GABA and activated by anions and commonly available within plant tissue. It is proposed that the GABA mediated ALMT regulation represents a signaling pathway possibly via changing membrane potentials which in turn can trigger physiological changes throughout the plant (Gilliham & Tyerman, Citation2016; Ramesh et al., Citation2015). The distribution and characterization of ALMT in plant cells and across plant tissue have not yet been clarified.
3. Behaviour of GABA within plants
Mechanical wounding of a single leaf in Arabidopsis induced a systemic accumulation of GABA in the intact adjacent leaves (Scholz, Reichelt, Mekonnen, Ludewig, & Mithöfer, Citation2015). Scholz et al. (Citation2017) showed that the systemic accumulation of GABA upon wounding in Arabidopsis is not dependent on the direct translocation of GABA throughout the plant. The application of D2-GABA to wounded leaves of pop2-5 mutant plants which are unable to degrade GABA resulted in an increase of GABA in the wounded leaves and in a de novo synthesis in systemic untreated leaves, but no translocation to unwounded leaves unconnected to the wounded leaf could be demonstrated.
4. Morphological responses of plants to exogenous GABA
After being taken up by the plant cells, exogenously applied GABA acts in the same way as endogenous GABA. It has been shown that exogenous GABA can induce changes of the endogenous GABA level under non-stress and stress conditions (Cekic, Citation2018; Li et al., Citation2017).
The application of GABA can affect different growth stages and morphological attributes of plants. In maize seedlings, a split application of GABA significantly improved root and shoot fresh weight of the seedlings. Depending on the variety, root and shoot dry weight increased as well. Coincidentally different physiological and biochemical mechanisms, a.o. net photosynthesis rate, SPAD, anti-oxidant enzymes and enzymes of the nitrogen metabolism were upregulated suggesting that these parameters positively influenced the morphological growth of the seedlings under the influence of GABA (Li, Lin et al., Citation2016). The performance of white gourds was significantly influenced in consequence of foliar treatment with GABA under field conditions. While a concentration-dependent increase of the leaf length could be demonstrated a similar effect on the leaf width was not observed. In addition, pre-yield factors like the number of female and especially the number of male flowers per plant increased depending on the dosage of the applied product (Ali, Ashrafuzzaman, Ismail, Shahidullah, & Prodhan, Citation2010). Soaking of pak-choi seeds with GABA significantly enhanced the growth and quality of the crop and affected the nitrate metabolism of the plants (Li, Tian, X-L., GONG, & Gao, Citation2016). In grapevines, GABA is present in leaves, bunches and branches in equivalent amounts and only in tendrils the amount of GABA was found to be up to 5 times higher. Treatment of isolated tendrils with GABA induced a transient and reversible positive effect on the coiling of the tendrils (Malabarba, Reichelt, Pasqualil, & Mithöfer, Citation2018).
Citrus seeds, treated with GABA prior to exposure to high concentrations of NaCl, improved the adaptation of the seeds to the saline conditions indicated by a higher germination rate and longer radicles (Ziogas, Tanou, Belghazi, & Diamantidis, Citation2017). Seeds or seedlings of wheat treated with GABA were able to partially compensate adverse effects of NaCl on seed germination and seedling development. Depending on the concentration of GABA and NaCl, germination and shoot dry mass partially improved (Li, Guo, Yang, Meng, and Wei (Citation2016). Root growth of rice seedlings exposed to excessive NH4 was alleviated when stressed plants were cultivated on GABA amended medium (Ma, Zhu, Yang, Gan, & Xla, Citation2016). Growth and survival of rice seedlings exposed to heat stress was restored in stressed plants growing on GABA containing medium (Nayyar, Kaur, Kaur, & Singh, Citation2014). Seedling root elongation of barley exposed to H+ and Al3+ (Song, Xu, Wang, Wang, & Tao, Citation2010) was improved. Exogenous GABA alleviated root growth retardation and restored biochemical functionalities of melon (Wang, Li, Xia, Wu, & Gao, Citation2014) and corn seedlings (Wang et al., Citation2017) under hypoxia. The inhibition of tomato seedling development (Malekzadeh, Khara, & Heydari, Citation2014) due to chilling injury could be compensated by GABA treatments which finally led to the enhancement of the activity of antioxidant enzymes.
These observations indicate that exogenous GABA has beneficial effects on plant development of non-stressed and especially also of stressed plants in different phases even though details of the underlying mechanisms still need clarification.
5. Physiological responses of plants to exogenous GABA
5.1. Carbon and nitrogen metabolism
Nutrient supply of plants is dependent on both photosynthesis and uptake through the roots. Arabidopsis mutants lacking enzymes for respiration showed an increased amino acid accumulation under extended dark. The accumulation was associated with an increase of both, protein degradation and accumulation of GABA and TCA cycle intermediates indicating that the GABA shunt was intensified to maintain respiration (Araujo et al., Citation2010). The metabolic pathway of GABA is divided between cytosol and the mitochondrial matrix and therefore GABA or GABA metabolites have the possibility to enhance photosynthesis via the mitochondrial matrix (Michaeli & Fromm, Citation2015). This was confirmed by Xia, Goa, and Li (Citation2011) who showed a direct effect of the treatment of musk melon seedlings with GABA on the increase of photosynthesis parameters.
Changes of the nutrient environment in plants induce modifications of uptake and metabolism through complex systems. The GABA shunt appears to be a part of the metabolic pathways involved in the balance between carbon and nitrogen through assimilation of carbon from glutamate and the carbon flux through the TCA cycle (Michaeli & Fromm, Citation2015). On the other hand, the assimilation of inorganic nitrogen leads to the production of glutamate, the substrate for the biosynthesis of amino acids, including GABA. The involvement of GABA in the regulation of the nitrogen level in plants is not yet clear. The expression of nitrate transporters on a transcriptional level increased when GABA was added to the growth medium while the effect on nitrate influx was low indicating that additional factors might be involved in the regulation of nitrate uptake (Beuve et al., Citation2004). Barbosa, Singh, Cherry, & Locy, Citation2010) showed that the growth of Arabidopsis thaliana in artificial medium in the presence of exogenous GABA is dependent on the NO3 level in the substrate and enzymes involved in carbon and nitrogen metabolism including nitrate reductase were regulated differently depending on the GABA concentration in the growth medium. These results either indicate that the growth effects seen in response to GABA are mediated via its metabolic role through the TCA cycle or that GABA acts as a signal for primary nitrogen metabolism and nitrate uptake in addition to its metabolic role as a carbon and nitrogen source.
5.2. Responses to biotic stress factors
GABA has been shown to induce resistance of treated crops towards plant pathogenic fungi and it has also been demonstrated that it can play a role in the defense of plants against damaging insects. There is experimental evidence that GABA has a direct measurable inhibitory effect on larvae of Spodoptera littoralis and Choristoneura rosaceana not only when added to their artificial diet but also after an infestation of GABA-enriched Arabidopsis mutants. The development of the larvae was delayed, their growth rate reduced and their survival rate decreased (Bown, Hall, & MacGregor, Citation2002; Bown, MacGregor, & Shelp, Citation2006; Ramputh & Bown, Citation1996; Scholz et al., Citation2015). These results demonstrate that GABA can have a direct and immediate effect on sucking or chewing insects in addition to its involvement in triggering down-stream defence reactions in the infested plants which are related with an accumulation of GABA. Elevated levels of GABA were detected locally in tobacco and soybean leaves as a response to insect damage (Bown et al., Citation2002). It can be assumed that the effect of GABA against the insects is neither based on its direct effect nor on its function as a signaling molecule but that most likely a combination of both mechanisms is involved.
Seifi et al. (Citation2013) demonstrated a biphasic defense response of sitiens tomato mutants towards Botrtytis cinerea comprising an early localized hypersensitive response at the penetration site and a timely overactivation of the GABA shunt. The authors postulate that the supply of energy and carbon skeletons helps both to control the extent of the hypersensitive response at the penetration site and to maintain the viability of the surrounding tissue indicated by a chlorotic ring. When wild type plants were treated with GABA prior to inoculation their susceptibility decreased and they showed similar macroscopic symptoms than the mutant. The authors assume that the same mechanism of overactivation of the GABA shunt in response to the infection with Botrytis cinerea was operating in the susceptible wild type and in the mutant. In case of Botrytis a direct fungitoxic or fungicidal effect of GABA can be excluded because the growth of the fungus was not affected on GABA amended agar.
In studies with tomatoes (Yang et al., Citation2017) and pears (Fu et al., Citation2017; Yu, Zeng, Sheng, Chen, & Yu, Citation2014) treatment with exogenous GABA applied prior to inoculation induced resistance of these crops towards fungal pathogens. The simultaneous increase of antioxidant enzymes such as catalase and peroxidase, in the treated plant parts suggests that GABA induced resistance towards biotic stress is at least in part dependent on the activation of antioxidant enzymes which restrict the plant cell death caused by reactive oxygen species (ROS).
For the time being there are no observations reported which indicate an adaptation or tolerance of organisms towards GABA. The development of resistance against insects or fungi is considered to be unlikely because GABA is a component of natural plant metabolism and, based on the knowledge of today exogenous and endogenous GABA are metabolized in the same way.
5.3. Improvement of shelf life and storage quality
GABA treatment was shown to be an effective approach for postharvest quality maintenance and improvement of the storage performance of bananas (Wang et al., Citation2014), citrus (Sheng, Shen, Luo, Sun, & Cheng, Citation2017), cucumber (Malekzadeh, Kosravi-Nejad, & Hatamnia, Citation2017) or peaches (Aghdam, Farhang, & Fatemeh, Citation2015; Shang, Cao, Yang, Cai, & Zheng, Citation2011; Yang, Cao, Yang, Cai, & Zheng, Citation2011) due to enhancement of antioxidant enzymes. Li et al. (Citation2019) demonstrated that the application of GABA protects pears from peel browning during long-term storage at low temperatures. In addition to lower browning indices also the content of ROS and malondialdehyde decreased while in parallel, gene expression and activities of antioxidant enzymes increased and the mitochondrial structure was maintained. Furthermore, the concentration of endogenous GABA increased compared to untreated pears. When Zucchini fruits were treated with GABA during postharvest cold storage, the quality of the fruits expressed by the chilling injury index and weight loss was improved compared to untreated. On cellular level, a higher GABA content during the whole trial period accompanied by increasing activities of GABA-T and GAD indicated the important role of constituents of the GABA shunt for the compensation of damage due to cold stress during storage (Palma et al., Citation2019). Also, postharvest indices of cut flowers were improved by pre- and postharvest GABA treatments (Aghdam, Naderi, Jannatizadeh, Babalar & Faradonbe, Citation2016; Aghdam, Naderi, Jannatizadeh, Sarcheshmeh, & Babalar, Citation2016). Aghdam, Naderi, Sarchesmeh, and Babalar (Citation2015) observed a dose-dependent positive effect on chilling injury and spathe browning after application of GABA at 1, 5, 10 and 20mM as preharvest spray or postharvest stem-end dipping. Furthermore, the membrane integrity was maintained, the overall antioxidant capacity as well as the activity of PAL enzyme was increased compared to untreated. Therefore, it can be concluded that the application of GABA helped to mitigate the chilling injury of the Anthurium cut flowers.
5.4. Responses to abiotic stress factors
Endogenous GABA levels were enhanced by exogenous GABA and the tolerance of plants towards abiotic stress was improved via positive regulation of the GABA shunt and associated pathways Bao & Li, Citation2015). In many studies in different crops such as lentils, melon, rice, wheat exogenous GABA application effectively alleviated germination or growth inhibition caused by unfavorable environmental conditions such as extreme temperatures, drought, water, salt, light or oxygen stress (Alqarawi et al., Citation2016; Al-Quraan & Al-Omari, Citation2012; El-kereamy et al., Citation2012; Fan, Wu, Tian, Jia, & Gao, Citation2015; Hu, Xu, Xu, Li, & Zhou, Citation2015; Koppitz, Dewender, Oostendorp, & Schmieder, Citation2004; Li, Peng, & Huang, Citation2016; Li, Yu, Peng, & Huang, Citation2016; Lum, Shelp, DeEll, & Bozzo, Citation2016; Mahmud et al., Citation2017; Nagao, Matsui, & Uemura, Citation2008; Takahashi, Matsumura, Kawai-Yamada, & Uchimiya, Citation2008; Vijayakumari & Puthur, Citation2016). The tolerance of wheat cultivars to salt and osmotic stress was shown to correlate with increased GAD expression and GABA accumulation (Al-Quraan, Sartawe, & Qaryout, Citation2013). Li et al. (Citation2016) demonstrated that the improvement of wheat seedling development under salt stress correlated a.o. with partially enhanced photosynthetic capacity and activity of antioxidant enzymes. Also in rice seedlings exposed to heat stress, restoration of growth was accompanied by a stimulation of different antioxidant enzymes (Nayyar et al., Citation2014). A higher expression of GABA receptor genes in drought-tolerant barley seedlings was observed (Guo et al., Citation2009). In plants, the GABA shunt regulated by Ca2+ via GAD was activated under stress conditions (Bai, Yang, Zhang, & Gu, Citation2013). If plants under stress were treated with GABA, the GABA shunt activity increased, the photosynthetic capacity enhanced, the level of endogenous GABA and also the levels of anti-oxidant enzymes increased while in parallel the content of malondialdehyde (MDA), a marker for oxidative stress, and the accumulation of ROS decreased (Gao & Guo, Citation2004; Jia et al., Citation2017; Liu, Zhao, & Yu, Citation2011) and finally the membrane integrity of the treated plants was maintained (Aghdam et al., Citation2015). Possibly enzymes which are bypassed by the GABA shunt are sensitive to oxidative stress. This sensitivity then could lead to a lower efficiency of the TCAC for which the GABA shunt can compensate in stressed plants. A functioning GABA shunt seems to be necessary to restrict ROS increase. Exposure to abiotic stress can cause leaf senescence which is usually accompanied by degradation of proteins and lipids. Degradation products are reallocated to the metabolic process and redistributed to growing plant parts or ripening fruits (Ansari, Hasan, & Jalil, Citation2014). Amino acids formed during the degradation of proteins are in part converted to glutamate and finally to GABA. Nitrogen and carbon skeletons become again available to the metabolic system of the plant through the enhanced activity of the GABA shunt, in particular, GABA-T, and further down-stream pathways as has been demonstrated by Jalil, Ahmad, and Ansari (Citation2016, Citation2017)) with GABA-T mutants of Arabidopsis thaliana.
6. Conclusions
GABA is a key metabolic component in plants where it has two major functions.
On one hand, it acts as a substrate for different key pathways and it is evident that it plays a key role as an interface linking several primary and secondary pathways in plants. Related to this metabolic roles are among others the provision of carbon skeletons and energy, the regulation of cytosolic pH, the involvement in nitrogen metabolism and possibly also the direct effect on insects. On the other hand, many studies demonstrated a relationship between stress perception, GABA accumulation and physiological reactions of the plants suggesting that GABA is also involved in signaling pathways either as a signal itself and/or as a trigger for signals for downstream pathways such as generation of ROS, activity of antioxidant enzymes, interaction with plant hormones or defense reactions against fungi and insects. In plants, exposure to stress leads to the accumulation of cellular Ca2+ and in a next step the Ca2+/CaM activation of GAD stimulates the conversion of Glutamate to GABA. GABA in turn regulates the activity of ALMTs which possibly trigger signals for downstream processes. Details of these complex relationships still need to be elucidated.
It has also been shown that GABA indirectly fine-tunes the growth and development of plants under non-stress conditions. GABA promotes germination, it influences the growth pattern of roots and above-ground plant parts and it modulates the photosynthetic capacity and plays a role in nitrogen metabolism.
The increase of intracellular GABA can induce the expression of signaling and metabolism- associated genes involved in the regulation of plant growth and plant vigor and increased stress tolerance. Together, these positive effects finally may also influence the quality and quantity of yield. Exogenously applied GABA can enhance the level of endogenous GABA thus providing beneficial effects on plant development and plant growth like the intrinsically formed molecule. The positive impact on plants together with the ability to induce stress tolerance in plants suggests that GABA could be considered as a tool to improve the quality of agricultural crops. However, for a better understanding of the potential of GABA more work on a broad range of crops and especially under practical conditions is necessary.
Competing interests
The authors declare no competing interests.
Additional information
Funding
Notes on contributors
Roberto Ramos-Ruiz
Roberto Ramos-Ruiz is technical director at Servalesa, a company aiming to offer products for farming which provide a differential value through their innovation and contribution to ensure healthier crops for healthier consumers. Amongst other responsibilities, Roberto is in charge of research and development. For the past decade, Servalesa has developed several research projects in collaboration with different universities and research organizations. The fundamental objective of these projects is to offer farmers technologies able to mitigate the effects caused by different kinds of plant stress with impact on crops, either biotic or abiotic, with an acceptable efficacy, no residues, a minimal impact on the environment and a toxicological profile with no effect on users and consumers through food treated with these products. Within this research activity, Servalesa has a particular interest in studying the effects on crops and impacts on human health and the environment of naturally occurring substances.
References
- Aghdam, M. S., Farhang, R., & Fatemeh, K. (2015). Maintaining the postharvest nutritional quality of peach fruits by γ-aminobutyric acid. Iranian Journal of Plant Physiology, 5, 1457–12.
- Aghdam, M. S., Naderi, R., Jannatizadeh, A., Babalar, M., & Faradonbe, M. Z. (2016). Impact of exogenous GABA treatments on endogenous GABA metabolism in Anthurium cut flowers in response to postharvest chilling temperature. Plant Physiology and Biochemistry, 106, 11–15. doi:10.1016/j.plaphy.2016.04.045
- Aghdam, M. S., Naderi, R., Jannatizadeh, A., Sarcheshmeh, M. A. A., & Babalar, M. (2016). Enhancement of postharvest chilling tolerance of Anthurium cut flowers by gamma-aminobutyric acid (GABA) treatments. Scientia Horticultura, 198, 52–60. doi:10.1016/j.scienta.2015.11.019
- Aghdam, M. S., Naderi, R., Sarchesmeh, M. A. A., & Babalar, M. (2015). Amelioration of postharvest chilling injury in Anthurium cut flowers by gamma-aminobutyric acid (GABA) treatments. Postharvest Biology and Technology, 110, 70–76. doi:10.1016/j.postharvbio.2015.06.020
- Ali, M. M., Ashrafuzzaman, M., Ismail, M. R., Shahidullah, S. M., & Prodhan, A. K. M. A. (2010). Influence of foliar applied GABA on growth and yield contributing characters of White Gourd (Benincasa hispida). International Journal of Agriculture and Biology, 12, 373–376.
- Allan, W. L., Simpson, J. P., Clark, S. M., & Shelp, B. J. (2008). Gamma-hydroxybutyrate accumulation in Arabodopsis and tobacco plants is a general response to abiotic stress: Puatative regulation by redox balance and gloxylate reductase isoforms. Journal of Experimental Botany, 59, 2555–2564. doi:10.1093/jxb/ern122
- Alqarawi, A. A., Hashem, A., Abd_Allah, E. F., Al-Huqal, A. A., Alshahrani, T. S., Alshalawi, S. R., & Egamberdieva, D. (2016). Protective role of γ-aminobutyric acid on Cassia italica Mill under salt stress. Legume Research, 39, 396–404.
- Al-Quraan, N. A., & Al-Omari, H. A. (2012). GABA accumulation and oxidative damage responses to salt, osmotic and H2O2 treatments in two lentil (Lens culinaris Medik) accessions. Plant Biosystems, 151, 148–157.
- Al-Quraan, N. A., Sartawe, F. A., & Qaryout, M. M. (2013). Characterization of gamma-aminobutyric acid metabolism and oxidative damage in wheat (Triticum aestivum L.) seedlings under salt and osmotic stress. Journal of Plant Physiology, 170, 1003–1009. doi:10.1016/j.jplph.2013.02.010
- Ansari, M. I., Hasan, S., & Jalil, S. U. (2014). Leaf senescence and GABA shunt. Bioinformation, 10, 734–736. doi:10.6026/97320630010734
- Araujo, W. L., Ishizaki, K., Nunes-Nesi, A., Larson, T. R., Tohge, T., & Krahnert, I. (2010). Identification of the 2-hydroxyglutarate and isovaleryl-CoA dehydrogenases as alternative electron donors linking lysine catabolism to the electron transport chain of Arabidopsis mitochondria. The Plant Cell, 22, 1549–1563. doi:10.1105/tpc.110.075630
- Araujo, W. L., Nunes-Nesri, A., Trenkamp, S., Bunik, V. I., & Fernie, A. R. (2008). Inhibition of 2-oxoglutarate dehydrogenase in potato tubers suggests the enzyme is linked to respiration and confirms its importance in nitrogen assimilation. Plant Physiology, 148, 1782–1796. doi:10.1104/pp.108.126219
- Bai, Q., Yang, R., Zhang, L., & Gu, Z. (2013). Salt stress induces accumulation of γ-aminobutyric acid in germinated Foxtail Millet (Setaria italica L.). Cereal Chemistry, 90, 145–149. doi:10.1094/CCHEM-06-12-0071-R
- Bao, H., & Li, Y. (2015). Virus-induced gene silencing reveals control of reactive oxygen species accumulation and salt tolerance in tomato by γ-aminobutyric acid metabolic pathway. Plant Cell and Environment, 38, 600–613. doi:10.1111/pce.12419
- Barbosa, J. M., Singh, N. K., Cherry, J. H., & Locy, R. D. (2010). Nitrate uptake and utilization is modulated by exogenous γ-amino-butyric acid in Arabidopsis thaliana seedlings. Plant Physiology and Biochemistry, 48, 443–450. doi:10.1016/j.plaphy.2010.01.020
- Beuve, N., Rispali, N., Laine, P., Cliquet, J. B., Ourry, A., & Le Deunff, E. (2004). Putative role of γ-aminobutyric acid (GABA) as a long distance signal in up-regulation of nitrate uptake in Brassica napus L. Plant Cell Environment, 27, 1035–1046. doi:10.1111/j.1365-3040.2004.01208.x
- Bouche, N., Fait, A., Bouchez, D., Møller, S. G., & Fromm, H. (2003). Mitochondrial succinic-semialdehyde dehydrogenase of the γ-amino-butyrate shunt is required to restrict levels of reactive oxygen intermediates in plants. Proceedings of the National Academy of Sciences of the United States of America, 100, 6843–6848. doi:10.1073/pnas.1037532100
- Bouche, N., & Fromm, H. (2004). GABA in plants: Just a metabolite? Trends in Plant Science, 9, 110–115. doi:10.1016/j.tplants.2004.01.006
- Bown, A. W., Hall, D. E., & MacGregor, K. B. (2002). Insect footsteps on leaves stimulate the accumulation of γ-amino-butyrate and can be visualized through increased chlorophyll fluorescence and superoxide production. Plant Physiology, 129, 1430–1434. doi:10.1104/pp.006114
- Bown, A. W., MacGregor, K. B., & Shelp, B. J. (2006). Υ-aminobutyrate: Defense against invertebrate pests? Trends in Plant Science, 11, 424–427. doi:10.1016/j.tplants.2006.07.002
- Bown, A. W., & Shelp, B. J. (2016). Plant GABA: Not just a metabolite. Trends in Plant Science, 21, 811–813. doi:10.1016/j.tplants.2016.08.001
- Carillo, P. (2018). GABA Shunt in Durum Wheat. Frontiers in Plant Science, 9, 100. doi:10.3389/fpls.2018.00100
- Carrol, A. D., Fox, G. G., Laurie, S., Philipps, R., Ratcliffe, R. G., & Stewart, G. R. (1994). Ammonium assimilation and the role of γ –Aminobutyric acid in pH homeostasis in carrot suspension cells. Plant Physiology, 106, 513–520. doi:10.1104/pp.106.2.513
- Cekic, F. Ö. (2018). Exogenous GABA stimulates endogenous GABA and phenolic acid contents in tomato plants under salt stress. Celal Bayar University Journal of Science, 14, 61–64.
- El-kereamy, A., Bi, Y.-M., Ranathunge, K., Beatty, P. H., Good, A. G., & Rothstein, S. J. (2012). The rice R2R3-MYB transcription factor OsMYB55 is involved in the tolerance to high temperature and modulates amino acid metabolism. PloS One, 7(12). doi:10.1371/journal.pone.0052030
- Fait, A., Fromm, H., Walter, D., Galili, G., & Fernie, A. R. (2008). Highway or byway: The metabolic role of the GABA shunt in plants. Trends in Plant Science, 13, 14–19. doi:10.1016/j.tplants.2007.10.005
- Fan, L., Wu, X., Tian, Z., Jia, K., & Gao, H. (2015). Comparative proteomic analysis of γ-aminobutyric acid responses in hypoxia-treated and untreated melon roots. Phytochemistry, 116, 28–37. doi:10.1016/j.phytochem.2015.02.023
- Fu, D., Sun, Y., Yu, C., Zheng, X., Yu, T., & Lu, H. (2017). Comparison of the effects of three types of aminobutyric acids on the control of Penicillium expansum infection in pear fruit. Journal of the Science of Food and Agriculture, 97, 1497–1501. doi:10.1002/jsfa.7891
- Gao, H. B., & Guo, S. R. (2004). Effects of exogenous γ-aminobutyric acid on antioxidant enzyme activity and reactive oxygen content in muskmelon seedlings under nutrient solution hypoxia stress. Journal of Plant Physiology and Molecular Biology, 30, 651–659.
- Gilliham, M., & Tyerman, S. D. (2016). Linking metabolism to membrane signaling: The GABA-malate connection. Trends in Plant Science, 21, 295–301. doi:10.1016/j.tplants.2015.11.011
- Guo, P., Baum, M., Grando, S., Ceccarelli, S., Bai, G., Li, R., von Korff, M., Varshney, R.K., Graner, A., & Valkoun, J. (2009). Differentially expressed genes between drought-tolerant and drought-sensitive barley genotypes in response to drought stress during the reproductive stage. Journal of Exp. Botany, 60, 3531–3544.
- Hu, X., Xu, Z., Xu, W., Li, J., & Zhou, Y. (2015). Application of γ-aminobutyric acid demonstrates a protective role of polyamine and GABA metabolism in muskmelon seedlings under Ca(NO3)(2) stress. Plant Physiology and Biochemistry, 92, 1–10. doi:10.1016/j.plaphy.2015.04.006
- Jalil, S. U., Ahmad, I., & Ansari, M. I. (2016). Phenotypic characterization of GABA-Transaminase mutants of Arabidopsis thaliana. Advances in Life Sciences, 5, 10005–10008.
- Jalil, S. U., Ahmad, I., & Ansari, M. I. (2017). Functional loss of GABA transaminase (GABA-T) expressed early leaf senescence under various stress conditions in Arabidopsis thaliana. Current Plant Biology, 9–10, 11–22. doi:10.1016/j.cpb.2017.02.001
- Jia, Y., Zou, D., Wang, J., Sha, H., Liu, H., Inayat, M.A., Sun, J., Zheng, H., Xia, N., & Zhao, H. (2017). Effects of γ-aminobutyric acid, glutamic acid, and calcium chloride on rice (Oryza sativa L.) under cold stress during the early vegetative stage. Journal of Plant Growth Regulation, 36, 240–253. doi:10.1007/s00344-016-9634-x
- Kinnersley, A. M., & Turano, F. J. (2000). Gamma aminobutyric Acid (GABA) and plant responses to stress. Critical Reviews in Plant Science, 19, 479–509. doi:10.1080/07352680091139277
- Koppitz, H., Dewender, M., Oostendorp, W., & Schmieder, K. (2004). Amino acids as indicators of physiological stress in common reed Phragmites australis affected by an extreme flood. Aquatic Botany, 79, 277–294. doi:10.1016/j.aquabot.2004.05.002
- Li, J., Zhou, X., Wei, B., Cheng, S., Zhou, Q., & Ji, S. (2019). GABA application improves the mitochondrial antioxidant sytem and reduces peel browning in “Nanguo” pears after removal from cold storage. Food Chemistry, 297.
- Li, J. R., Tian, Z., X-L., W. U., GONG, -B.-B., & Gao, H.-B. (2016). Regulation of γ-aminobutyric acid on growth and nitrate metabolism of pak-choi treated with high nitrogen application. Acta Horticulturae Sinica, 43, 2182–2192.
- Li, M. F., Guo, S. J., Yang, X. H., Meng, Q. W., & Wei, X. J. (2016). Exogenous γ-aminobutyric acid increases salt tolerance of wheat by improving photosynthesis and enhancing activities of antioxidant enzymes. Biologia Plantarum, 60, 123–131. doi:10.1007/s10535-015-0559-1
- Li, W., Lin, J., Ashraf, U., Li, G., Li, Y., Lu, W., Gao, L., Han, F., & Hu, J. (2016). Exogenous γ-aminobutyric Acid (GABA) application improved early growth, net photosynthesis, and associated physio-biochemical events in maize. Frontiers in Plant Science, 7, 1–13.
- Li, Y., Fan, Y., Jiao, Y., Ma, Y., Zhang, Z., Yue, H., Wang, L., & Jiao, Y. (2017). Effects of exogenous γ-Aminobutyric Acid (GABA) on photosynthesis and antioxidant system in Pepper (Capsicum annuum L.) Seedlings under low light stress. Journal of Plant Growth Regulation, 36, 436–449. doi:10.1007/s00344-016-9652-8
- Li, Z., Peng, Y., & Huang, B. (2016). Physiological effects of γ-aminobutyric acid application on improving heat and drought tolerance in creeping bentgrass. Journal of the American Society for Horticultural Science, 141, 76–84. doi:10.21273/JASHS.141.1.76
- Li, Z., Yu, J., Peng, Y., & Huang, B. (2016). Metabolic pathways regulated by γ-aminobutyric acid (GABA) contributing to heat tolerance in creeping bentgrass (Agrostis stolonifera). Scientific Reports, 6, 1–16.
- Ling, Y., Chen, T., Jing, Y., Fan, L., & Wan, Y. (2013). Υ-aminobutyric acid (GABA) homeostasis regulates pollen germination and polarized growth in Picea wilsonii. Planta, 238, 831–843. doi:10.1007/s00425-013-1938-5
- Liu, C., Zhao, L., & Yu, G. (2011). The dominant glutamic acid metabolic flux to produce γ-aminobutyric acid over proline in Nicotiana tabacum leaves under water stress relates to its significant role in antioxidant activity. Journal of Integrative Plant Biology, 53, 608–618. doi:10.1111/j.1744-7909.2011.01049.x
- Lum, G. B., Shelp, B. J., DeEll, J. R., & Bozzo, G. G. (2016). Oxidative metabolism is associated with physiological disorders in fruits stored under multiple environmental stresses. Plant Science, 245, 143–152. doi:10.1016/j.plantsci.2016.02.005
- Ma, X., Zhu, C., Yang, N., Gan, L., & Xla, K. (2016). Υ-aminobutyric acid addition alleviates ammonium toxicity by limiting ammonium accumulation in rice (Oryza sativa) seedlings. Physiologia Plantarum, 158, 389–401. doi:10.1111/ppl.12473
- Mahmud, J. A., Hasanuzzaman, M., Nahar, K., Rahman, A., Hossain, M. S., & Fujita, M. (2017). Υ -aminobutyric acid (GABA) confers chromium stress tolerance in Brassica juncea L. by modulating the antioxidant defense and glyoxalase systems. Ecotoxicology, 26, 675–690. doi:10.1007/s10646-017-1800-9
- Malabarba, J., Reichelt, M., Pasqualil, G., & Mithöfer, A. (2018). Tendril coiling in Grapevine: Jasmonates and a new role for GABA? Journal of Plant Growth Regulation, 37.
- Malekzadeh, P., Khara, J., & Heydari, R. (2014). Alleviating effects of exogenous γ-aminobutyric acid on tomato seedlings under chilling stress. Physiology and Molecular Biology of Plants, 20, 133–137. doi:10.1007/s12298-013-0203-5
- Malekzadeh, P., Kosravi-Nejad, F., & Hatamnia, A. A. (2017). Impact of postharvest exogenous γ-aminobutyric acid treatment on cucumber fruits in response to chilling tolerance. Physiology and Molecular Biology of Plants, 23, 827–836. doi:10.1007/s12298-017-0475-2
- Michaeli, S., Fait, A., Lagor, K., Nunes-Nesi, A., Grillich, N., Yellin, A.,Bar, D., Khan, M., Fermie, A.R., Turani, F.J. & Fromm, H. (2011). A mitochondrial GABA permease connects the GABA shunt and the TCA cycle, and is essential for normal carbon metabolism. The Plant Journal : for Cell and Molecular Biology, 67, 485–498. doi:10.1111/j.1365-313X.2011.04612.x
- Michaeli, S., & Fromm, H. (2015). Closing the loop on the GABA shunt in plants: Are GABA metabolism and signaling entwined? Frontiers in Plant Science, 6. doi:10.3389/fpls.2015.00419
- Nagao, M., Matsui, K., & Uemura, M. (2008). Klebsormidium flaccidum, a charophycean green alga, exhibits cold acclimation that is closely associated with compatible solute accumulation and ultrastructural changes. Plant Cell and Environment, 31, 872–885. doi:10.1111/j.1365-3040.2008.01804.x
- Nayyar, H., Kaur, R., Kaur, S., & Singh, R. (2014). Υ-aminobutyric acid (GABA) imparts partial protection from heat stress injury to rice seedlings by improving leaf turgor and upregulating osmoprotectants and antioxidants. Journal of Plant Growth Regulation, 33, 408–419. doi:10.1007/s00344-013-9389-6
- Palma, F., Carvajal, F., Jiménez-Muñoz, R., Pulido, A., Jamilena, M., & Garrido, D. (2019). Exogenous y-aminobutyric acid treatment improves cold tolerance of zucchini fruit during postharvest storage. Plant Physiol and Biochemistry, 136, 188–195. doi:10.1016/j.plaphy.2019.01.023
- Podlesakova, K., Ugena, L., Spichal, L., Dolezal, K., & De Diego, N. (2019). Phytohormones and ployamines regulate plant stress respoonses by altering GABA pathways. New Biotechnology, 48, 53–65. doi:10.1016/j.nbt.2018.07.003
- Ramesh, S. A., Tyerman, S. D., Xu, B., Bose, J., Kaur, S., & Conn, V. (2015). GABA signalling modulates plant growth by directly regulating the activity of plant-specific anion transporters. Nature Communications, 6, 7879. doi:10.1038/ncomms8879
- Ramos-Ruiz, R., Poirot, E., & Flores-Mosquera, M. (2018). GABA, a non-protein amino acid ubiquitous in food matrices. Cogent Food & Agriculture, 4, 1534323.
- Ramputh, A. I., & Bown, A. W. (1996). Rapid γ-aminobutyric acid synthesis and the inhibition of the growth and development of oblique-banded leaf roller larvae. Plant Physiology, 111, 1349–1352. doi:10.1104/pp.111.4.1349
- Renault, H., Deleu, C., Palanivelu, R., Updegraff, E. P., Yu, A., Renou, J.-P., … Deleu, C. (2011). GABA accumulation causes cell elongation defects and a decrease in expression of genes encoding secreted and cell-wall-related proteins in Arabidopsis thaliana. Plant Cell Physiology, 52, 894–908. doi:10.1093/pcp/pcr041
- Renault, H., El Amrani, A., Berger, A., Mouille, G., Soubigou-Taconnat, L., & Bouchereau, A. (2013). Υ-aminobutyric acid transaminase deficiency impairs central carbon metabolism and leads to cell wall defects during salt stress in Arabidopsis roots. Plant, Cell and Environment, 36, 1009–1018. doi:10.1111/pce.12033
- Scholz, S. S., Malabarba, J., Reichelt, M., Heyer, M., Ludewig, F., & Mithöfer, A. (2017). Evidence for GABA-induced systemic GABA accumulation in Arabidopsis upon wounding. Frontiers in Plant Science, 8. doi:10.3389/fpls.2017.00388
- Scholz, S. S., Reichelt, M., Mekonnen, D. W., Ludewig, F., & Mithöfer, A. (2015). Insect herbivory-elicited GABA accumulation in plants in wound-induced, direct, systemic and jasmonate-dependent defense response. Frontiers in Plant Science, 6. doi:10.3389/fpls.2015.01128
- Seifi, H. S., Curvers, K., Vleesschauwer, D. D., Delaere, I., Azis, A., & Höfte, M. (2013). Concurrent overactivation of the cytosolic glutamine synthetase and the GABA shunt in the ABA-deficient sitiens mutant of tomato leads to resistance against Botrytis cinerea. New Phytologist, 199, 490–504. doi:10.1111/nph.12283
- Shang, H., Cao, S., Yang, Z., Cai, Y., & Zheng, Y. (2011). Effect of exogenous gamma-aminobutyric acid treatment on proline accumulation and chilling injury in peach fruits after long-term cold storage. Journal of Agricultural and Food Chemistry, 59, 1264–1268. doi:10.1021/jf104424z
- Shelp, B. J., Bown, A. W., & Zarei, A. (2017). Υ-aminobutyrate (GABA): A metabolite and signal with practical significance. Botany, 95, 1015–1032. doi:10.1139/cjb-2017-0135
- Sheng, L., Shen, D., Luo, Y., Sun, X., & Cheng, Y. (2017). Exogenous γ-aminobutyric acid treatment affects citrate and amino acid accumulation to improve fruit quality and storage performance of postharvest citrus fruit. Food Chemistry, 216, 138–145. doi:10.1016/j.foodchem.2016.08.024
- Snedden, W. A., Arazi, T., Fromm, H., & Shelp, B. J. (1995). Calcium/calmodulin activation of soybean glutamate decarboxylase. Plant Physiology, 108, 543–549. doi:10.1104/pp.108.2.543
- Song, H., Xu, X., Wang, H., Wang, H., & Tao, Y. (2010). Exogenous gamma-aminobutyric acid alleviates oxidative damage caused by aluminum and proton stresses on barley seedlings. Journal of the Science of Food and Agriculture, 90, 1410–1416. doi:10.1002/jsfa.3951
- Takahashi, H., Matsumura, H., Kawai-Yamada, M., & Uchimiya, H. (2008). The cell death factor, cell wall elicitor of rice blast fungus (Magnaporthe grisea) causes metabolic alterations including GABA shunt in rice cultured cells. Plant Signaling & Behavior, 3, 945–953. doi:10.4161/psb.6112
- Vijayakumari, K., & Puthur, J. T. (2016). Υ-aminobutyric acid (GABA) priming enhances the osmotic stress tolerance in Piper nigrum Linn. plants subjected to PEG-induced stress. Journal of Plant Growth Regulation, 78, 57–67. doi:10.1007/s10725-015-0074-6
- Wang, C.-Y., Li, J. R., Xia, Q. P., Wu, X. L., & Gao, H. B. (2014). Influence of exogenous γ-aminobutyric acid (GABA) on GABA metabolism and amino acid contents in roots of melon seedling under hypoxia stress. The Journal of Applied Ecology, 25, 2011–2018.
- Wang, Y., Gu, W., Meng, Y., Xie, T., Li, L., & Li, J. (2017). Υ-aminobutyric acid imparts partial protection from salt stress injury to maize seedlings by improving photosynthesis and upregulating osmoprotectants and antioxidants. Scientific Reports, 7, 1–13.
- Wang, Y., Luo, Z., Huang, X., Yang, K., Gao, S., & Du, R. (2014). Effect of exogenous γ-aminobutyric acid (GABA) treatment on chilling injury and antioxidant capacity in banana peel. Scientia Horticulturae, 168, 132–137. doi:10.1016/j.scienta.2014.01.022
- Xia, Q. P., Goa, H.-B., & Li, J.-R. (2011). Effects of γ–Aminobutyric acid on the photosynthesis and chlorophyll fluorescence parameters of muskmelon seedlings under hypoxia stress. Chinese Journal of Applied Ecology, 22, 999–1006.
- Yang, A., Cao, S., Yang, Z., Cai, Y., & Zheng, Y. (2011). Υ-aminobutyric acid treatment reduces chilling injury and activates the defense response of peach fruit. Food Chemistry, 129, 1619–1622. doi:10.1016/j.foodchem.2011.06.018
- Yang, J., Sun, C., Zhang, Y., Fu, D., Zheng, X., & Yu, T. (2017). Induced resistance in tomato fruit by γ-aminobutyric acid for the control of Alternaria rot caused by Alternaria alternata. Food Chemistry, 22, 1014–1020. doi:10.1016/j.foodchem.2016.11.061
- Yang, R., Yin, Y., & Gu, Z. (2015). Polyamine degradation pathway regulating growth and GABA accumulation in germinating Fava bean under hypoxia-NaCl stress. Journal of Agricultural Science and Technology, 17, 311–320.
- Yu, C., Zeng, L., Sheng, K., Chen, F., & Yu, T. (2014). Υ-aminobutyric acid induces resistance against Penicillium expansum by priming of defense responses in pear fruit. Food Chemistry, 159, 29–37. doi:10.1016/j.foodchem.2014.03.011
- Yu, G.-H., Zou, J., Feng, J., Peng, X. B., Wu, J. Y., Palanivelu, R., Sun, M. X. (2014). Exogenous γ-aminobutyric acid (GABA) affects pollen tube growth via modulating putative Ca2+-permeable membrane channels and is coupled to negative regulation on glutamate decarboxylase. Journal of Experimental Botany, 65, 3235–3238. doi:10.1093/jxb/eru171
- Ziogas, V., Tanou, G., Belghazi, M., & Diamantidis, G. (2017). Characterization of β-amino- and γ-aminobutyric acid-induced citrus seeds germination under salinity using nanoLC-MS/MS analysis. Plant Cell Reports, 36, 787–789. doi:10.1007/s00299-016-2063-2