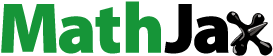
Abstract
Moringa stenopetala is a locally valuable plant; it has nutritional and medicinal values. Its production has progressively grown. The aim of this study was to evaluate the physical properties, and in vitro digestibility of the microencapsulated bioactive product using spray dryer from M. stenopetala leaves extract. The selected spray drying process parameters such as maltodextrin: high methoxyl pectin ratio (10:0, 9.5:0.5, and 9:1), core:coating ratios (1:10, 1:8, and 1:6), and inlet air temperature (120°C, 140°C and 160°C) on the physical properties and digestibility of the microencapsulates. The result showed that process conditions significantly affect the physical properties and the total flavonoid content. The spray drying processing parameters significantly affect the flowability property. The Hausner's ratio, Carr’s index and angle repose were in the range of 1.68–2.11, 40.47–43.73% and 32.32–57.05°, respectively. Moreover, the in vitro digestibility was affected by the coating materials ratio, core: coating ratio and inlet air temperature. In conclusion, the spray drying microencapsulation parameters had significant effects on the physical properties and in vitro digestibility. The physical properties and digestibility of the microencapsulated bioactive product was relatively improved when the mixture of maltodextrin and pectin was used as a coating material than using maltodextrin alone.
PUBLIC INTEREST STATEMENT
Bioactive compounds are secondary metabolites of the plant, which are produced to increase their overall ability to survive and withstand the harsh environment and resists to diseases. Moringa stenopetala plant contains bioactive compounds, which have antihypertensive, anti-inflammatory, antihyperglycemic and prevention of cancer. However, during food processing such as thermal processing, these bioactive compounds can to be destructed. Hence, encapsulation might be an alternative technology to maintain the functionality of the active compounds.
Competing Interests
The authors declare no competing interests.
1. Introduction
Moringa stenopetala is a locally valuable plant, and its production has progressively grown. M. stenopetala is an indigeneous in Ethiopia, Kenya and Somalia; however, it was getting lower attentions when it is compared to M. oleifera in terms of research and promotion (Habtemariam, Citation2015). This plant uses as nutritional sources and it has water clarification effects (Jiru, Sonder, Alemayehu, Mekonen, & Anjulo, Citation2006). Furthermore, the local community uses this plant as a traditional medicine. The medicinal value most probably due to the presence of bioactive constituents such as the phenolic and flavonoid compounds. Thus, it is progressively attracting the attention of researchers to find out the contribution to health and disease prevention due to the biological activity of the plant (Dadi, Emire, Hagos, & Eun, Citation2019). There are a lot of researches have been done on the chemical composition of M. stenopetala leaves (Jiru et al., Citation2006; Melesse, Citation2011). In addition, it has bioactive compounds and antioxidant (Dadi, Emire, Hagos, & Assamo, Citation2018; Dadi et al., Citation2019; Habtemariam, Citation2015), antidiabetic (Habtemariam, Citation2015; Nardos, Makonnen, & Debella, Citation2011), antihypertensive (Geleta, Makonnen, Debella, & Tadele, Citation2016), antimicrobial activities (Walter, Samuel, Peter, & Joseph, Citation2011), and reduce serum glucose and cholesterol level (Ghebreselassie, Mekonnen, Gebru, Ergete, & Huruy, Citation2011). As a result, it has a potential to be used as an alternative source for the development of functional foods and pharmaceutical products.
Spray drying is one of the encapsulation techniques, which is relatively simple, ease of application to large scale and low cost technique used for encapsulation of bioactive compounds (Gharsallaoui, Roudaut, Chambin, Voilley, & Saurel, Citation2007). There are different coating materials such as maltodextrin, pectin, gum arabic, whey protein isolates and modified starches (Zuidam & Shimoni, Citation2010). The coating materials have different encapsulation efficiency and release of active compounds. Moreover, the coating materials also determine the storage stability and digestibility (Sagis, Citation2015). Maltodextrin has high water solubility capacity and low viscosity at high concentration, whereas pectin has good emulsifying power (Sansone et al., Citation2011). These different properties of the coating materials may improve the encapsulation capacity when they are used in mixed form than using a single form.
Since microencapsulation is helpful to mask the bitter taste and odour, protect heat sensitive compounds from degradation, improves the storage stability and control release of active compounds (Zuidam & Shimoni, Citation2010). Therefore, to maximize the utilization of M. stenopetala, microencapsulation of bioactive compounds from its leaf extract may be one of the better options for industrialization. However, as to our knowledge, no published study was found on the encapsulation of M. stenopetala leaves extract. Therefore, the aim of this study was to evaluate the spray drying process parameters on the physical properties and digestibility of microencapsulated bioactive products from M. stenopetala leaf extract.
2. Materials and methods
2.1. Sample collection
The M. stenopetala leaves sample was collected from Arba Minch, located at 6°01ʹ59” N and 37° 32ʹ59” E, at a distance 505 km from the capital city, Addis Ababa, Ethiopia. The collected sample was washed immediately using distilled water to remove any foreign matter. Subsequently, it was dried for 72 h in a room with an average temperature of 25°C and 62% of relative humidity. The temperature and relative humidity were measured using the iButton temperature/humidity logger (DS1923, iButton, San Jose, CA, USA). The dried sample was ground using an electric blender (SMX-757, Shinil Ind. Co. Ltd., Seoul, Korea) and allowed to pass through a sieve (20 meshes), kept in a sealed polyethylene bag, and stored in the dark until extraction was carried out.
2.2. Extraction of bioactive compounds
The extraction was done according to Dadi et al. (Citation2019). The sample was mixed with 70% ethanol in the ratio of 1:40 (m/V). The extraction was done using the shaking water bath (JSSB-50T, JS Research Inc., Gongju, Korea) at a temperature of 30°C for 24 h. Then, the extract was filtered using the Whatman No. 1 filter paper and dried using a rotary evaporator (Eyela, N-11, Tokyo, Japan) under vacuum at a temperature of 40°C. The dried extract was stored in the refrigerator at 4 C.
2.3. Spray drying encapsulation
Maltodextrin (Cornstarch, Eisse Food Co. Ltd., Korea) with a dextrose equivalent (DE) of 14–20, and high methoxyl pectin (Pectin RS400, Danisco Inc., USA) were used as encapsulating materials. Maltodextrin (MD) solutions were prepared by mixing with distilled water in proportions of 10%, 9.5% and 9% (w/V) using a magnetic stirrer. High methoxyl pectin (HP) was added in the MD solution to obtain a total solids content of 10% (w/V) with the weight ratios of 10:0, 9.5:0.5 and 9:1 (w/w). The mixture was dissolved using a magnetic stirrer. Subsequently, the extract was mixed with the core:coating material at ratios of 1:10, 1:8 and 1:6 (w/w). Then, the mixtures were homogenized using a high-speed homogenizer (T 25 Ultra-Turrax, IKA, Germany) at 35,000 rpm for 3 min. The solutions were finally put on the ultrasonicator for 5 min.
The spray drying was done according to the method described by Sansone et al. (Citation2011) with some modifications. A lab scale spray dryer (Lab Plant, SD-06, Hunmanby, England) was used, which has two fluid nozzles with standard 0.5 mm jet. The spray dryer was adjusted to the following conditions: air speed exhaust at 4.3 m/s, liquid flow rate at 485 mL/h, the deblocker was set at medium and the compressor at two bars. The microencapsulation was done at inlet temperatures of 120°C, 140°C and 160°C. The mixture was continuously stirred as spray drying was performed. Finally, the dried sample was packed and stored in a desiccator for further analysis.
2.4. Water activity
The water activity was measured using thermoconstanter (TH-200, Novasina thermoconstanter, Lachen, Switzerland).
2.5. Flowability property
The flowability of the sample was evaluated as described by Deore, Thombre, and Gide (Citation2013) using Hausner’s ratio and Carr’s index. The angle of repose was determined using a fixed funnel and digital angle ruler (DXN-360, DigiX New, Japan). Then, each value was expressed using the equations below.
where H is the height of the pile, R is the radius at the base of the pile.
2.6. Dispersibility determination
The dispersibility was determined according to Laokuldilok and Kanha (Citation2015) with some modifications. Briefly, about 1 g of the microencapsulated powder was put in 10 mL of distilled water and mixed vigorously for 25 s. It was allowed to pass through 150 µm sieve. Then, 1 mL of the solution was transferred to the pre-weighed aluminum can and kept in the oven at 105°C for 4 h. Finally, the dispersibility was calculated using the equation:
where S is the amount of microencapsulated powder (g), Ds is the amount of the dried supernatant that passed through the sieve (%), and MC is the moisture content of the microencapsulated powder (%).
2.7. Determination of total phenolic content
The total phenolic content (TPC) was measured according to Dadi et al. (Citation2019). Briefly, 0.5 mL of the extract (1 mg/mL) wasmixed with 2.5 mL of 10% Folin-Ciocalteu reagent and vortexed. After 8 min, 2 mL of 7.5% sodium carbonate wasadded, mixed and kept in the dark at room temperature for 2 h. The same procedure was used for the blank and gallic acid standard at different concentrations to develop a standard curve. The absorbance was measured at 765 nm using a UV-Vis spectrophotometer (Optizen 2120 UV, Mecasys Co., Ltd, Korea). Then, the TPC was expressed as mg of gallic acid equivalent per gram of microencapsulates (mg GAE/g micro).
2.8. Determination of total flavonoid contents
The total flavonoid content (TFC) was determined according to Dadi et al. (Citation2019). Briefly, 0.5 mL of the extract was mixed with 0.15 mL of 5% sodium nitrite and 2.5 mL of distilled water and kept for 6 min. Then, 0.3 mL of 10% aluminum chloride was mixed. This was followed by the addition of 1 mL of 1 M sodium hydroxide. Subsequently, 0.55 mL of distilled water was added. After the mixture was vortexed, it was kept for 15 min. The same procedure was applied to the blank and catechin standard at different concentration to develop a standard curve. Then, the absorbance was measured at 510 nm using a UV-Vis spectrophotometer. Finally, the TFC was expressed as mg catechin equivalent per gram of microencapsulates (mg CE/g micro).
2.9. Evaluation of the in vitro digestibility of the microencapsulate
First, the simulated gastric fluid (SGF) was prepared according to the procedure followed by Saikia, Mahnot, and Mahanta (Citation2015). In brief, 2 g of sodium chloride and 3.2 g of pepsin (from porcine stomach mucosa with the activity of 800 to 2500 Units/mg of protein) was mixed with 7 mL of hydrochloric acid. The solution was made to 1000 mL by adding distilled water. Finally, the pH of the solution was adjusted to 1.2 using 0.2 N sodium hydroxide or 0.2 N hydrochloric acid. Then, 0.2 g of the microencapsulate powder was put in 40 mL centrifuge tube and 2.4 mL of the gastric fluid solution was added. Then, the mixture was sealed and put on the shaking water bath at 80 rpm at 37 ± 0.5°C for 2 h. Thenafter, the solution was allowed to cool and centrifuged at 1915xg at 4°C for 10 min. The supernatant was collected, filtered using 0.45 µm filter and then neutralized by adding 0.2 N sodium hydroxide solution.
The simulated intestinal fluid (SIF) was prepared according to the procedure followed by Saikia et al. (Citation2015). In brief, 6.8 g of monobasic potassium phosphate was mixed in 250 mL of distilled water. Then, 77 mL of 0.2 N sodium hydroxide followed by 500 mL of distilled water. The mixture was mixed again after 10 g of pancreatic enzyme was added. Then, distilled water was added in the solution to make l000 mL. Finally, the resulted solution was adjusted to a pH of 6.8 ± 0.1. Then, the microencapsulate (0.2 g) and 4.8 mL of SIF solution was mixed in 40 mL of a centrifuge tube. The mixture was then sealed and kept in the water bath 37 ± 0.5°C for 2 h without shaking. After this, the solution was allowed to cool and centrifuged at 1915 xg at 4°C for 10 min. Then, the supernatant was collected and filtered using 0.45 µm filter. Then, the solution was acidified by adding 0.2 N hydrochloric acid to stop enzymatic activity. After 15 min, 0.2 N sodium hydroxide was added to neutralize the acidity. Finally, the total phenolic and flavonoid contents were determined according to the methods described above.
2.10. Particle size distribution of the microencapsulates
It was measured according to the method of Patwa, Malcolm, Wilson, and Ambrose (Citation2014), with minor modifications. An automatic standard sieve shaker (SV001, Impact Test Equipment Ltd., Ayrshire, UK) was used. The sieves-set had apertures of 5000, 3150, 2000, 1000, 600, 250, and 150 µm. The weight of each empty sieve was recorded. The microencapsulated bioactive product was placed on the top sieve and shaken for 15 min. Afterward, the product retained on each sieve was weighed and the corresponding fractions percentages were calculated.
2.11. Morphology analysis of the microencapsulates
The morphological analysis of microencapsulate powder was done using scanning electron microscope (SEM) (JSM-7500, Jeol Ltd., Tokyo, Japan) to evaluate the morphological effects of the spray and freeze drying microencapsulation processes and coating materials. The sample was put on a plate with double-sided adhesive tape and vacuum coated with platinum. The morphological analysis was done at an accelerated voltage of 15 kv and 1000x magnification.
2.12. Statistical analysis
To evaluate the effects of maltodextrine: pectin ratio, core: coating ratio and inlet air temperature on the physical properties and digestibility of spay-dried microencapsulated product box-behnken design was used. All the experiments were conducted in triplicate and expressed as mean ± standard deviation. The means comparisons were performed using one way analysis of variance (ANOVA), Tukey HSD test using JMP software (Version 13.0, 2016, SAS institute Inc., Cary, NC, USA). Differences were considered at a significance level of p ≤ 0.05.
3. Results and discussion
3.1. Total flavonoid content
As shown in Table , the total flavonoid contents (TFC) of the microencapsulates significantly affected by the spray drying process conditions. The TFC increased as the core:coating ratio increased from 1:10 to 1:6. This is due to the increment of the concentration of the extract when the core:coating ratio is increased. The same trend was reported by Tolun, Altintas, and Artik (Citation2016) when the core:coating ratio was increased. In addition, when the mixture of maltodextrin and pectin (MDHP) was used, the TFC was higher than the microencapsulates coated with maltodextrin (MD) alone. The mixture of maltodextrin with other coating materials increases the bioactive contents due to the improvement of the encapsulation efficiency (Tolun et al., Citation2016).
Table 1. Total flavonoid contents (TFC) and physical properties of spray dried microencapsulated bioactive product from M. stenopetala leaves extract
The increment of inlet air temperature from 120°C to 160°C, the TFC of the microencapsulates decreased mainly when the core:coating ratio was increased (Table ). This is probably due to lower encapsulation efficiency when the core:coating ratio was increased. Thus, the TFC was decreased to due to the destruction effects of high inlet air temperature. However, the microencapsulates developed at 140°C had high TFC. Similar result was reported by Tolun et al. (Citation2016) on grape polyphenol encapsulates. The phenolic content was destructed when the inlet air temperature was increased (Sansone et al., Citation2011). In addition, TFC was descreased at an inlet air temperature of 160°C. This is due to the destruction of the flavonoid compounds at higher temprature.
3.2. Water activity
The water activity of the microcapsule can determine the cohesiveness as well as the storage stability of the microencapsulate (Mahdavi, Jafari, Assadpoor, & Dehnad, Citation2016). In general, the water activity of the microencapsulated bioactive product was lower (Table ). As a result, the storage stability of the product improved due to the absence of the hydrolysis reaction and microbial growth.
3.3. Dispersibility
Dispersibility is the ability of a powder to separate into individual particles when dispersed in water with gentle mixing (Laokuldilok & Kanha, Citation2015). The spray drying processing conditions affected the dispersibility of the microencapsulated bioactive product of M. stenopetala leaves extract. The dispersibility was significantly (p < 0.05) higher when the core coating ratio and inlet air temperature were raised (Table ). As Ghosal, Indira, and Bhattacharya (Citation2010) reported the dispersibility of the powder increases as its particle size is increased. This probably complies with the results of the present study. In another work, it was found that the increment of the inlet air temperature results in larger particle size (Goula & Adamopoulos, Citation2008). The larger particles might be settled on the bottom leading to greater dispersibility (Ghosal et al., Citation2010; Goula & Adamopoulos, Citation2008).
Thus, the effect of the coating material on the dispersibility is minimized. Laokuldilok and Kanha (Citation2015) reported the same trend on the dispersibility of the anthocyanin powders. In addition, the increment of the dispersibility was found from the microencapsulates coated with maltodextrin alone when it was compared with the mixture of maltodextrin and pectin (Table ). This shows that with increasing pectin concentration there is reduction in the dispersibility the microencapsulates. This might be due to the gelling and stickiness property of the pectin. Moreover, when the core coating ratio was increased, the concentration of the coating material will be reduced, which can have an effect on the agglomeration of the product.
3.4. Flowability
It is difficult to characterize the physical properties of the product using a single method due to the complexity of the granular materials (Lumay et al., Citation2012). Therefore, Hausner’s ratio, Carr’s index and angle of repose were used to evaluate the flowability of the microencapsulated bioactive product. The results showed that the spray drying processing conditions significantly affect the flowability property of the microencapsulates (Table ). The results showed that the Hausner's ratio, Carr’s index and angle repose were in the range of 1.68–2.11, 40.47–43.73% and 32.32–57.05°, respectively (Table ). Generally, the flowability of the products is placed under the poor flowability category when the Hausner’s ratio, Carr’s index, and angle of repose values are more than 1.25, 25%, and 45°; respectively (Deore et al., Citation2013; Mahdavi et al., Citation2016).
The angle of repose was considerably affected by inlet air temperature (Table ). When the inlet air temperature was decreased, a higher angle of repose was found. This is probably because of the higher moisture content and the wrinkled structure of the microencapsulates made at lower temperature. Moreover, when the core:coating ratio increased, the angle of repose was lowered. This might be related to the stickiness property of the coating material. As Saikia et al. (Citation2015) stated the stickiness of the product increases when the concentration of maltodextrin is raised. Therefore, when the angle of repose is higher that indicates a poorer flowability property of the product.
In addition, the cohesiveness of the powder can be evaluated by the Hausner’s ratio. When the Hausner’s ratio was higher, the cohesiveness of the powder is relatively higher, implying a lesser free flowing capacity (Fernandes, Borges, and Botrel (Citation2013). The values of the Hausner’s ratios, Carr’s index and angle of repose showed that the nature of the coating materials had relatively slight impact on the flowability microencapsulates when it was compared with the core:coating ratio and the inlet temperature. In general, the flowability differences originate from the morphological structure, moisture content, frictions and cohesive forces of the microencapsulated product (Lumay et al., Citation2012). In addition, the presence of high surface contact area within particles can lead to the flow resistance of the product (Fernandes et al., Citation2013). The deviations of the Hausner’s ratio and Carr’s index results from the angle of repose were probably due to the applied additional forces during powder tapping. Thus, the occupied volume was reduced.
4. Particle size distribution of microenapsulates
As shown in Table , the particle size distribution of the spray-dried microencapsulated bioactive products were considerably affected by the spray drying microencapsulation conditions. These differences were due to the differences in the coating materials ratio, core: coating ratio and inlet air temperature. As the inlet air temperature increased, the particle size distribution of the microencapsulates was founded on the larger sieve size. On the contrary, the microencapsulates developed at a lower inlet air temperature had relatively low particle size. This finding is in agreement with Tonon, Brabet, and Hubinger (Citation2008) for Euterpe oleraceae powder. This might be due to the contribution of higher inlet air temperature on the enhancement of swelling of the product (Tonon et al., Citation2008); moreover, higher inlet air temperature fosters the formation of the particles, which are not shrunken, and thus of results larger particle size.
Table 2. Particle size distribution of the microencapsulated bioactive product from M. stenopetala leaves extract
The coating materials ratios also have an impact on the particle size distribution (Table ). The particle size distribution of the microencapsulates coated with the maltodexrin indicated that the particles had smaller size when compared to those coated by the mixture of maltodextrin and pectin (Table ). As reasoned out by Tonon et al. (Citation2008), the particle size is related to the molecular size of the coating material, i.e. degree of polymerization of maltodextrin. When the core:coating ratio was low, the particle size of the microencapsulates was relatively large. This indicates that a higher concentration of the coating material contributes to enlarged size of the powder particles. Saikia et al. (Citation2015) reported similar results when the maltodextrin concentration was increased. This is due to the relative increment of the viscosity of the solution at lower core:coating ratio, which results in larger droplets during atomization (Tonon et al., Citation2008). On the contrary, as the maltodextrin concentration increased, the particle size of the spray dried Asian pear juice powder was lower (Lee, Ahmed, Jiang, & Eun, Citation2017). This showed that the particle size distribution of the microencapsulates depends on different spray drying process conditions.
4.1. Digestibility of microencapsulates
Microencapsulation-based delivery systems are getting more attention due to their efficient absorption behavior in the digestive system which improves the delivery of the active compounds to the target site (Sansone et al., Citation2011). The TPC in both simulated gastric and intestinal fluids were of closer contents. This indicates that the release of phenolic compounds or the digestibility of the microencapsulate coated with maltodextrin alone were digested almost similar in both SGF and SIF environments (Table ). On the other hand, the relative release of the phenolic compounds of microencapsulate coated with the mixture of maltodextrin and pectin was significantly higher in the intestinal fluid than the gastric fluid (Table ). This result similar to the findings reported by Sansone et al. (Citation2011). Microencapsulates with a mixture of maltodextrin and pectin coating material had higher release rate when it was compared to maltodextrin encapsulated phenolic compound. Besides, the higher release of the phenolic compound was taken place in the gastric fluid (Saikia et al., Citation2015).
Table 3. Evaluation of the digestibility of microencapsulated product interms of total phenolic content in simulated gastric fluid and intestinal fluid
This might be due to the differences on the solubility of the coating materials depending on the gastrointestinal compositions and conditions including enzymatic activities. It has been suggested that the microencapsulates were dependent on the pH of the gut and the type of the coating materials employed (Saikia et al., Citation2015). The solubility of high methoxyl pectin is higher when the pH is around seven, which has similarity with the intestinal fluid. In addition, the micro particle-water interaction might be increased due to the amorphous state of the powder and the hydrophilic nature of pectin (Sansone et al., Citation2011) as well as due to the relative smaller particle size microencapsulate. This is an advantage to release the phenolic compounds at the right target site where their absorption takes place.
As the core: coating ratio was increased, the release of the phenolic compound was higher (Table ). This is related to the concentration of the extract in the microcapsule. In addition, the release of the phenolic compound was lower when the inlet temperature was at 160°C than when it was 120°C. This is probably due to the enhancement of the binding ability of the coating material at a higher inlet temperature, thus, the release of the active compounds will take a longer time.
4.2. Morphology of microencapsulates
The morphological structure of the microencapsulated bioactive product is shown in Figure . In general, the wrinkled and the dent shape of the microencapsulate was noted; However, this was more at a lower inlet air temperature (Figure ). Moreover, the agglomeration of the microencapsulates is observed in Figure . This is probably due to the lower inlet air temperature which results in relatively high moisture content. The variation in the degree of agglomeration among the spray dried microencapsulates might be due to difference in the concentration of maltodextrin. As the concentration of maltodextrin is increased, the agglomeration of the microencapsulate can be more profound due to the higher dextrose equivalent of the maltodextrin. The same result has been reported in spray dried watermelon powders (Quek, Chok, & Swedlund, Citation2007). This agglomeration might be considered as an advantage for the microencapsulated bioactive product since it may reduce the oxidation by minimizing exposure to oxygen (Quek et al., Citation2007). Surface cracking on the microencapsulate was also observed at the inlet air temperature of 160°C. This could lead to the release of the active compoundsfurther to their exposure to the external environment.
5. Conclusion
This study showed that the spray drying process conditions such as the coating material ratios, core: coating ratios and inlet air temperature significantly affect the physical properties of the microencapsulated bioactive product from M. stenopetala leaf extract. The spray drying processing conditions significantly affect the flowability property of the microencapsulates. The Hausner's ratio, Carr’s index and angle repose were in the range of 1.68–2.11, 40.47–43.73% and 32.32–57.05°, respectively. These indicate that the flowability of the products is placed under the poor flowability category when the Hausner’s ratio, Carr’s index, and angle of repose values are more than 1.25, 25%, and 45°; respectively. In addition, the digestibility of the microencapsulated bioactive product was improved when the mixture of maltodextrin and pectin was used as a coating material when it was compared to maltodextrin alone.
Conflict of interest
The authors declare no conflict of interest
Acknowledgements
The authors would like to thank the financial support of Addis Ababa University and National Institute for International Education, and Chonnam National University, South Korea. We also would like to thank Professor Kim-Young Soo, for his willingness to use the spray dryer and Mrs.Hae Mi Kim for her technical support, Chonbuk National University, South Korea.
Additional information
Funding
Notes on contributors
Debebe Worku Dadi
Dr. Eng. Debebe Worku Dadi is an Assistant Professor of Food Engineering at Ambo University, Ethiopia. His research is functional foods and nutraceutical product development.
Shimelis Admassu Emire
Dr. Eng. Shimelis Admassu Emire is an associate professor of Food Engineering at Addis Ababa University, Ethiopia and does research in Food Processing and Agro-food industry.
Asfaw Debella Hagos
Dr. Asfaw Debella Hagos is a Lead Researcher at Ethiopian Public Health Institute, Ethiopia. His research is Phytochemical and traditional medicine.
Jong-Bang Eun
Prof. Jong-Bang Eun is a professor in Food Science and Technology at Chonnam National University, South Korea. His research is on functional food development.
References
- Dadi, D. W., Emire, S. A., Hagos, A. D., & Assamo, F. T. (2018). Influences of different drying methods and extraction solvents on total phenolic and flavonoids, and antioxidant capacity of moringa stenopetala leaves. Journal of Pharmacognosy and Phytochemistry, 7(1), 962–12.
- Dadi, D. W., Emire, S. A., Hagos, A. D., & Eun, J. B. (2019). Evaluation of ultrasonic-assisted extraction of moringa stenopetala leaves on bioactive compounds and antioxidant effect. Food Technology and Biotechnology, 57(1), 77–86. doi:10.17113/ftb.57.01.19.5877
- Deore, K. L., Thombre, N. A., & Gide, P. S. (2013). Formulation and development of tinidazole microspheres for colon targeted drug delivery system. Journal of Pharmacy Research, 6(1), 158–165. doi:10.1016/j.jopr.2012.11.034
- Fernandes, R. V. D. B., Borges, S. V., & Botrel, D. A. (2013). Influence of spray drying operating conditions on microencapsulated rosemary essential oil properties. Food Science and Technology, 33, 171–178. doi:10.1590/S0101-20612013000500025
- Geleta, B., Makonnen, E., Debella, A., & Tadele, A. (2016). In vivo antihypertensive and antihyperlipidemic effects of the crude extracts and fractions of Moringa stenopetala (Baker f.) Cufod. Leaves in rats. Frontiers in Pharmacology, 7. doi:10.3389/fphar.2016.00097
- Gharsallaoui, A., Roudaut, G., Chambin, O., Voilley, A., & Saurel, R. (2007). Applications of spray-drying in microencapsulation of food ingredients: An overview. Food Research International, 40(9), 1107–1121. doi:10.1016/j.foodres.2007.07.004
- Ghebreselassie, D., Mekonnen, Y., Gebru, G., Ergete, W., & Huruy, K. (2011). The effects of Moringa stenopetala on blood parameters and histopathology of liver and kidney in mice. Ethiopian Journal of Health Development, 25(1), 51–57. doi:10.4314/ejhd.v25i1.69850
- Ghosal, S., Indira, T., & Bhattacharya, S. (2010). Agglomeration of a model food powder: Effect of maltodextrin and gum Arabic dispersions on flow behavior and compacted mass. Journal of Food Engineering, 96(2), 222–228. doi:10.1016/j.jfoodeng.2009.07.016
- Goula, A. M., & Adamopoulos, K. G. (2008). Effect of maltodextrin addition during spray drying of tomato pulp in dehumidified air: II. Powder properties. Drying Technology, 26(6), 726–737. doi:10.1080/07373930802046377
- Habtemariam, S. (2015). Investigation into the antioxidant and antidiabetic potential of Moringa stenopetala: Identification of the active principles. Natural Product Communications, 10(3), 475–478. doi:10.1177/1934578X1501000324
- Jiru, D., Sonder, K., Alemayehu, L., Mekonen, Y., & Anjulo, A. (2006). Leaf yield and nutritive value of Moringa stenopetala and Moringa oleifera accessions: Its potential role in food security in constrained dry farming agroforestry system. Proceedings of the Moringa and Other Highly Nutritious Plant Resources: Strategies, Standards and Markets for a Better Impact on Nutrition in Africa, Accra, Ghana, 16–18.
- Laokuldilok, T., & Kanha, N. (2015). Effects of processing conditions on powder properties of black glutinous rice (Oryza sativa L.) bran anthocyanins produced by spray drying and freeze drying. LWT-Food Science and Technology, 64(1), 405–411. doi:10.1016/j.lwt.2015.05.015
- Lee, C.-G., Ahmed, M., Jiang, G.-H., & Eun, J.-B. (2017). Color, bioactive compounds and morphological characteristics of encapsulated Asian pear juice powder during spray drying. Journal of Food Science and Technology, 54(9), 2717–2727. doi:10.1007/s13197-017-2708-3
- Lumay, G., Boschini, F., Traina, K., Bontempi, S., Remy, J.-C., Cloots, R., & Vandewalle, N. (2012). Measuring the flowing properties of powders and grains. Powder Technology, 224, 19–27. doi:10.1016/j.powtec.2012.02.015
- Mahdavi, S. A., Jafari, S. M., Assadpoor, E., & Dehnad, D. (2016). Microencapsulation optimization of natural anthocyanins with maltodextrin, gum Arabic and gelatin. International Journal of Biological Macromolecules, 85, 379–385. doi:10.1016/j.ijbiomac.2016.01.011
- Melesse, A. (2011). Comparative assessment on chemical compositions and feeding values of leaves of Moringa stenopetala and Moringa oleifera using in vitro gas production method. J. Appl. Sci. Technol, 2(2), 31–41.
- Nardos, A., Makonnen, E., & Debella, A. (2011). Effects of crude extracts and fractions of Moringa stenopetala (Baker f) Cufodontis leaves in normoglycemic and alloxan-induced diabetic mice. African Journal of Pharmacy and Pharmacology, 5(20), 2220–2225.
- Patwa, A., Malcolm, B., Wilson, J., & Ambrose, K. R. (2014). Particle size analysis of two distinct classes of wheat flour by sieving. Transactions of the ASABE, 57(1), 151–159.
- Quek, S. Y., Chok, N. K., & Swedlund, P. (2007). The physicochemical properties of spray-dried watermelon powders. Chemical Engineering and Processing: Process Intensification, 46(5), 386–392. doi:10.1016/j.cep.2006.06.020
- Sagis, L. M. (2015). Microencapsulation and microspheres for food applications. New York, USA: Academic Press.
- Saikia, S., Mahnot, N. K., & Mahanta, C. L. (2015). Optimisation of phenolic extraction from Averrhoa carambola pomace by response surface methodology and its microencapsulation by spray and freeze drying. Food Chemistry, 171, 144–152. doi:10.1016/j.foodchem.2014.08.064
- Sansone, F., Mencherini, T., Picerno, P., d’Amore, M., Aquino, R. P., & Lauro, M. R. (2011). Maltodextrin/pectin microparticles by spray drying as carrier for nutraceutical extracts. Journal of Food Engineering, 105(3), 468–476. doi:10.1016/j.jfoodeng.2011.03.004
- Tolun, A., Altintas, Z., & Artik, N. (2016). Microencapsulation of grape polyphenols using maltodextrin and gum arabic as two alternative coating materials: Development and characterization. Journal of Biotechnology, 239, 23–33. doi:10.1016/j.jbiotec.2016.10.001
- Tonon, R. V., Brabet, C., & Hubinger, M. D. (2008). Influence of process conditions on the physicochemical properties of açai (Euterpe oleraceae Mart.) powder produced by spray drying. Journal of Food Engineering, 88(3), 411–418. doi:10.1016/j.jfoodeng.2008.02.029
- Walter, A., Samuel, W., Peter, A., & Joseph, O. (2011). Antibacterial activity of Moringa oleifera and Moringa stenopetala methanol and n-hexane seed extracts on bacteria implicated in water borne diseases. African Journal of Microbiology Research, 5(2), 153–157.
- Zuidam, N. J., & Shimoni, E. (2010). Overview of microencapsulates for use in food products or processes and methods to make them. In Encapsulation technologies for active food ingredients and food processing (pp. 3–29). Dordrecht: Springer.