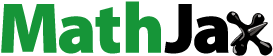
Abstract
Maize lethal necrosis (MLN) is considered an important constraint of maize production in Ethiopia and particularly in southern part of the country. The study was conducted during 2016 and 2017 main cropping seasons to (i) determine the effect of hand weeding and insecticide application against MLN and yield and yield related attributes of maize; and (ii) to determine the economy of additional costs used for the management of MNL. The treatments such as hand weeding, only insecticide applied, hand weeding in combination of insecticide application (HW + InA) and unmanaged were arranged in randomized complete block design with five replications. Results showed that MLN severity was highly significant (p < 0.001) different both during 2016 and 2017 cropping seasons. It was varied among the treatments and between cropping years. In the two years, it was highest on unmanaged plots with 66.33% in 2016 and 56.33% in 2017. This might have been due to severe insect and weed infestation during the growing period over the evaluated plots. Conversely, the lowest MLN severity was recorded on the HW + InA with the value of 26.67% in 2016 and 25.00% in 2017 cropping season. Thus, severity was reduced by 62.31% in 2016, and 52.65% in 2017 in this treatment. As such not much difference in the area under disease progress curve (AUDPC) was observed in the two years. However, in both cropping seasons the lowest (15.96% and 15.01%-days, respectively) mean amount of AUDPC was recorded from plots managed with HW + InA. In 2016 and 2017 cropping seasons, HW + InA proved to be an effective against MLN management and gave the highest yields (4.89 and 5.84 t ha−1, respectively) over the unmanaged (1.06 and 1.39 t ha−1, respectively). Overall, results showed HW + InA was found to be better management option and reduced MLN and increased grain yields. Thus, it is better, as compared to other treatments, to use management option as it gave the relatively higher defense against MLN. However, further studies have to be undertaken in different agro-ecologies elsewhere in Ethiopia for developing solid advice on behalf of stabilizing maize production. Moreover, further research is warranted to confirm the role of insect pests and weeds as vector and alternative hosts, respectively for MCMV, SCMV, MDMV and WSMV causing MLN.
PUBLIC INTEREST STATEMENT
Maize lethal necrosis (MLN) is an emerging disease and major threatening disease of maize production systems in eastern and central Africa. The MLN is a serious challenge in major maize producing areas of Ethiopia. Since the outbreak, it causes considerable yield losses. However, lack of awareness about the role of unwise managements, insect vectors transmission and loaded on alternate hosts by the growers and less attention by the experts to virus diseases become a serious problem to many parts of the country, which demands an urgent reply from stakeholders. To recommend, managing of insect vectors and alternate hosts that influence the MLN intensity is a prerequisite. In this study, therefore, different management approaches in combination or alone have been studied. Thus, the results recommended using insecticide along with hand weeding as it gave relatively higher defense against MLN that target on the insect vectors and alternate hosts.
Competing interests
The authors declare no competing interests.
1. Introduction
Maize (Zea mays L.) is one of the most important and widely grown cereal crops in the world after wheat and rice, and consumed crop in the world (FAOSTAT, Citation2017). The crop is cultivated in different parts of African region under diversified climatic and agro-ecological conditions. It is produced under extensive private farms and subsistence farmers on small land holdings, who have limited access to inputs such as fertilizers and pesticides. The crop is major food crop and served as staple food for human consumption, cash crop for currency, feed for livestock and as raw material for many industrial uses in Africa and in Ethiopia as well, and the most important grain covering wider acres than other grains in sub-Saharan Africa (Central Statistical Agency [CSA], Citation2017; Gibson & Benson, Citation2002; Smale & Jayne, Citation2011).
In Ethiopia, out of the total areas covered by maize, 7.85 million tons have been produced in the 2016/17 main cropping season (CSA, Citation2017). Similarly, 1.09 million tons of maize production have been obtained from 0.32 million hectares of land in southern Ethiopia. Comparatively, total annual productions of maize go beyond all other cereals in area coverage and number of households involved, implying that maize is one of the priority food security crops in Ethiopia (CSA, Citation2017). However, despite its nutritional and economic values, most of the maize growers still obtain very low yields. In this regard the national average productivity is only 3.43 t ha−1, which is far below than the world’s average (5.6 t ha−1) yield (CSA, Citation2015; FAOSTAT, Citation2015). Likewise, the maize productivity in the study areas is even lower (3.36 tha−1) than the national average (3.68 tha−1) yield (CSA, Citation2017). Such low maize yield is attributed to a combination of quite a lot of biotic and abiotic constraints. Amongst the biotic factors, diseases are the most important ones; of which, maize lethal necrosis (MLN) has emerged as the most important constrain in maize production system in the eastern and central Africa (Mahuku et al., Citation2015; Miano, Citation2014).
MLN, synonym to corn lethal necrosis, is one of the major destructive virus diseases of maize in all parts of the country (Demissie et al., Citation2016). The disease was first observed in Kansas and Nebraska in 1976 (Niblett & Claflin, Citation1978). MLN was the result of synergistic co-infection of the two different maize virus diseases: either of combination of maize chlorotic mottle virus (MCMV) from the genus Maclomovirus in the family Tombuviridae with a virus from the family Potyviridae, especially sugarcane mosaic virus (SCMV), maize dwarf mosaic virus (MDMV) or Wheat streak mosaic virus (WSMV) (Niblett & Claflin, Citation1978; Uyemoto, Bockelman, & Clafin, Citation1980). No infection of maize by any of the Potyviridae group viruses alone to cause MLN. MCMV is transmitted mechanically and spread by several insect vectors including maize thrips (Frankliniella williamsi) (Jiang, Wilkinson, & Berry, Citation1990), corn flea beetle (Systena frontalis), cereal leaf beetles (Oulema melanopus), maize rootworms (Diabrotica undecimpunctata, Diabrotica longicornis and Diabrotica virgifera) and Chaetocnema pulicaria (Cabanas, Watanabe, Higashi, & Bressan, Citation2013; Jensen, Citation1985; Nault et al., Citation1978). SCMV is spread by maize aphids (Singh, Ashutosh, Upadhyaya, & Rao, Citation2005).
MCMV or SCMV typically produce milder symptoms when they infect maize alone; however, in combination they rapidly produce a synergistic reaction that seriously damage or kill the infected maize plant. Symptoms developed by MLN are more severe than symptoms of either MCMV or SCMV or any other Potyviridae group alone, and the complexes of these viruses cause a severe systemic necrosis which lead to the death of maize plant (Niblett & Claflin, Citation1978; Uyemoto et al., Citation1980; Wangai et al., Citation2012a). MLN is mainly spread by a vector transmitting the disease from plant to plant and field to field. The most common vectors for MLN are maize thrips, rootworms, leaf beetles and aphids (Cabanas et al., Citation2013; Nault et al., Citation1978; Zhao, Ho, Wu, He, & Li, Citation2014), and had a number of alternate hosts (Bockelman, Claflin, & Uyemoto, Citation1982; Castillo & Hebert, Citation1974; Gordon, Bradfute, Gingery, Nault, & Uyemoto, Citation1984; Kusia, Citation2014; Niblett & Claflin, Citation1978). Redinbaugh and Zambrano-Mendoza (Citation2014) reported that virus disease to occur its vector and a susceptible host must come together in a suitable environment. For this reasons, to design effective management option the management scheme should target the pathosystem components.
For the last few years, MLN has also been reported in China, Ecuador, Taiwan, Kenya and other African countries found with the result of a synergistic interaction between MCMV and SCMV (Adams et al., Citation2014; Uyemoto, Citation1983; Wangai et al., Citation2012a; Xie et al., Citation2011). The outbreak of MLN in East Africa was first reported in Kenya in 2011 and in 2014 Ethiopia as well and become a serious challenge to maize production and exhibited a big threaten (0–100% yield losses) to food security for the majority of farming communities in this region (Demissie et al., Citation2016; Wangai et al., Citation2012a). In Ethiopia, four types of viruses such as maize streak virus, sugarcane mosaic virus, maize dwarf mosaic virus and maize mottle chlorotic stunt virus had been reported in infected fields of maize (Tewabech, Getachew, Fekede, & Dagne, Citation2002) and the combination of one of the two virus, MCMV and SCMV, favors the occurrence of MLN (Demissie et al., Citation2016). Since the outbreak of MLN, it reappears year after year in the country and causes considerable yield losses. To prevent the effects of MLN, farmers use dominantly cultural practices, eradication of the infected maize stands form the field and throw into either roadside or ditches or footpaths or in rows within the maize field or feed for animals after culling, but they did not recognized the importance of the infected plant, vectors and the weed infestation in the field.
Several methods are available to manage MLN and attempts have been made to manage it through different cultural methods, use of resistance/tolerant genotypes and vector control through insecticidal applications (Redinbaugh & Zambrano-Mendoza, Citation2014; Uyemoto, Citation1983). Although there are some management tactics that can be quickly adapted to the conditions in Ethiopia, some work needs to be done to test and validate them. Likewise, in the study areas field observation showed that there has been high weed infestation in the farm, which favors too many insect pests to harbor the main field and consequently farmers noting did anything in their fields (Terefe & Getachew, Citation2019). Empirical research necessarily conducted to rapidly validate additional potential management option since farmers’ lacks awareness for the transmission and sources of the MLN either vector or alternate hosts. Moreover, management of MLN disease through combination of hand weeding and insecticide application has not been evaluated in the study areas. This research work trying to demonstrate and developing effective management option achieved through the use of integrated approaches by combination of hand weeding and insecticidal management of the vectors. Therefore, the objectives of this research was to (i) evaluate the effect of hand weeding and insecticide application against MLN and yield and yield related attributes of maize; and (ii) determine the economy of additional costs used for the management of MLN around Arba Minch areas in Southern Ethiopia.
2. Material and methods
2.1. Description of experimental area
The field experiment was conducted during 2016 and 2017 main cropping seasons at Arba Minch in southern Ethiopia. Arba Minch is among the major maize producing areas of the southern region of the country (CSA, Citation2017). Latitudinal and longitudinal position of the specified area is 06 06ʹ841″ N and 037 35ʹ122″ E with an altitude of 1216 m above sea level. According to National Meteorological Agency yearly report (Citation2017), the area is characterized by bimodal rainfall pattern: short rainy season (March to April) and main rainy season (mid-August to mid-November). The details of weather data for the areas are presented in Table . In addition, the area is characterized by moderately alkaline with low organic contents (1.05%) and black sandy-loam in the soil type (Ministry of Agriculture and Natural Resources [MoANR], & Ethiopian Agricultural Transformation Agency [EATA], Citation2016).
Table 1. Monthly mean minimum and maximum temperature, rainfall and relative humidity of Arba Minch areas in Southern Ethiopia during 2016 and 2017 cropping seasons
2.2. Planting time, design of experimental and management procedures
The experiment was carried out in the field where the areas are infected in order to ensure the disease occurrence and enhance the natural infections at the begging of the experiment. Planting material, Maize BH140 which is highly susceptible for MLN, was obtained from Bako agricultural research center, Ethiopian Institute of Agricultural Research, in Ethiopia. The layout was designed with 16 m width × 21 m length and a unit plot size of 4.0 m width × 4.0 m length, respectively. Plots were spaced at 1.5 m each other and blocks separated by a safeguard path of 2.5 m to prevent insecticide drift or cross contamination. The plot was consisted of six rows and four harvestable middle rows. Spacing between plants and rows were maintained as 25 cm and 75 cm, respectively. The maize variety was sown on 4 September 2016 and 7 September 2017 cropping season for the two consecutive years.
The experiment was laid out in a randomized complete block design with five replications. A total of four treatments were comprised during experiment: hand weeding, insecticide applied, hand weeding in combination with insecticide application (HW + InA) and unmanaged (no weeding and insecticide application). The insecticide which is used during experiment was lambda-cyhalothrin 5% EC at the rate of 1 L ha−1 for the suppression of maize insect pests at 7 day intervals, which was intended to vector management. The insecticide is a contact type with broad spectrum and has a repellant property and due to this the application was undertaken after insect pest assessment. Spraying of insecticide has been started with the first appearance of the insect pest (29 (in 2016) and 34 days after planting (DAP) (in 2017)) and had continued according to the spray schedule designated by the manufacturer. Hand weeding practice was started at 21 DAP and had continued every 14 days interval for three times. All other cultural practices for maize growing were done uniformly following recommended practices. NPS and N-fertilizer with one to one ratio (100:100 kg ha−1) were used for nutrient management.
2.3. Data collection
2.3.1. Disease assessment
Disease assessment is the procedure which produces all the necessary data that quantify the development of disease. Thus, MLN incidence and severity were recorded in every 10 days interval starting from the first appearance (20 (in 2016) and 26 DAP (in 2017)) of disease symptoms, which was a typical of MLN. A total of seven assessments were conducted per season during the two cropping seasons. Disease incidence was assessed by counting the number of infected plants on the middle four rows and determined as the percentage of maize plants over the total number of maize plants assessed per plot. Disease severity was assessed visually as the percentage of apparently diseased tissue, leaf area affected, (James & Teng, Citation1979) from 10 randomly selected and tagged plants within the plots. It was scored on a rating scale of 1–5; where 1 = no disease symptoms, 2 = fine chlorotic streaks/mottling on lower leaves, 3 = chlorotic mottling and mosaic throughout the whole plant, 4 = excessive chlorotic mottling, mosaic, plant necrosis and/or dead heart, and 5 = dead plant and complete plant necrosis (Beyene et al., Citation2017). Disease severity scores were converted into percentage severity index (PSI) using the formula suggested by Wheeler (Citation1969) as follows:
Area under disease progress curve (AUDPC), the development of disease on a whole plant or part of the plant, was computed separately for disease assessments made on different DAP for each treatment using PSI value. It was used for summarize the epidemics of the MLN in different management options. The values were expressed in %-days (Campbell & Madden, Citation1990; James & Teng, Citation1979):
Where, Xi = PSI of disease at ith assessment; ti = time of the ith assessment in days from the first assessment date; and n = total number of disease assessments.
2.3.2. Insect pest assessment
Insect pests (vectors) and weed species assessment were undertaken by counting for each species categories within the plots. Count of live insect pest, so as to preventing the loss of insect pests either flying or died and fallen to the ground due to the repellant property of the insecticide used, was done pre-spray application of insecticide on the randomly selected plants, which were used for assessment of MLN severity and in the plots summed up each of insect pests recorded. Identification of insect pests was done using Stereomicroscope, Hand lens and Corn field guide (Abendroth et al., Citation2009). The former technique was done under laboratory and the latter two techniques were done under field condition. Totally, counting was made for three times during 2016 and 2017 cropping years.
2.3.3. Weed species assessment
Weed species found at each plot were recorded prior to weeding practice starting on weed-free plots during the experiment. However, for weed infested plot the weed species were counted after last weeding of hand weeding treatments. Weed species recording and identification was made from the three central rows by leaving the border rows and one meter from each side of the central rows, the central plot size would be 4 m2. Weed species identification was made using weed identification book illustrated and organized by Stoud and Parker (Citation1989) and Botanical herbarium collected and preserved by Arba Minch crop protection clinic. The collected weed data were summarized using mathematical analysis. Relative Abundance (RA), Dominancy (RD), Frequency (RF), weed density (RWD) and Importance value (IV) of the species were calculated. IV of each species recorded was computed following Das (Citation2008). Moreover, the weed density (WD) was computed as needed for knowing potential impact on the influence of MLN.
2.3.4. Growth and yield parameters assessment
The growth and yield parameter were recorded during the experiment. Parameters like plant height (cm), leaf area (length × width), average number of kernels per row in ear (total number of kernels in each row divided by total number of rows on the ear), average number of rows per ear (total number of rows in each ear divided by total number of sampling ears within the plot), number of healthy and diseased ear due to the MLN, yield (t ha−1) and dry matter (t ha−1) were determined from each plot. Harvest index (HI) was determined from the yield and biomass data (Kawano, Citation1990) and leaf area index (LAI) was also determined as length × width × 0.75 (Birch, Citation1996).
2.4. Data analysis
Prior to data analysis heterogeneity test was performed for each parameters measured for the two seasons. Also, the two seasons were considered as different environments because of the variation in weather conditions during the study period. Consequently, the F-test was applied to test for heterogeneity of the error variance following the procedure described by Gomez and Gomez (Citation1984). Unfortunately, the F-test for heterogeneity of error variance to most of the parameters measured for the two seasons were verified to the heterogeneity of the data and as a result the data were analyzed and presented independently for each year. Analysis of variance was performed on all disease, growth and yield related parameters to test for the presence of significant differences between treatments (Gomez & Gomez, Citation1984) using general linear model of SAS version 9.2 (SAS Institute Inc, Citation2009). Mean separations were made using Fisher’s protected least significant deference (LSD) values at 5% probability level. Linear regression analysis was used to determine the relationships between MLN, number insect pests and WD across the treatments. Linear relationships between grain yield and disease parameter per each treatment were examined using regression analysis. AUDPC was used for predicting of the yield loss in maize in the association study (Campbell & Madden, Citation1990; James & Shih, Citation1973).
2.5. Estimation of cost and benefit and relative yield loss
Based on the pooled data obtained from the two seasons, economic analysis for alone or in combination of management options was performed using partial budget analysis as described by International Maize and Wheat Improvement Center [CIMMYT] (Citation1988). Price of maize grain ($ t−1) was assessed from the local market. Variable input costs like insecticide, knapsack sprayer and labor (spraying and weeding) costs ha−1 were recorded. The unit price of insecticide varied from year to year and analyzed accordingly, $11.36 and $16.95 L−1 in 2016 and 2017 cropping years, respectively. Similarly, the unit prices of maize grain $21.26 t−1 (2016) and $25.86 t−1 (2017). Regarding cost of labor for spraying of insecticide and weeding practices were on average $1.88 and $2.82 per operator per day during 2016 and 2017 cropping seasons, respectively. All costs were converted to per hectare basis for the proposed analysis. During partial budget analysis, costs of agronomic practices were uniform for all treatments and costs of labor and spraying equipment were taken based on the prevailing wage rates in the locality. Before doing partial budget analysis, statistical data analysis was done on the data to compare the average yields between treatments. Since there were differences between treatment means, the obtained economic data were subjected to analysis using the partial budget analysis method and MRR was calculated as:
Where, DNI = difference in net income compared with control, and DIC = difference in input cost compared with control. In addition to this relative percent yield loss, the measurable reduction in yield, from each plot was calculated using the formula suggested by Robert and James (Citation1991):
Where, Ybt is the yield of best treatment (maximum protected plot) and Ylt is the yield of lower treatments.
3. Results
3.1. MLN incidence
Symptoms of MLN were first observed on 20 and 26 DAP on hand weeding and insecticide sprayed plots during 2016 and 2017 cropping seasons, respectively. In both cropping seasons, insecticide sprayed alone and hand weeding did not reveal significant (p > 0.05) difference for MLN incidence at the dates of 20 and 30, and 26, 36, 46 and 56 assessment dates, respectively (Table ). Highly significance difference (p < 0.001) on MLN incidence was detected in insecticide sprayed alone and HW + InA in the two years, at the final date of disease assessment (50–80 in 2016 and 66–86 DAP in 2017). In 2016 cropping seasons, mean disease incidence did not exceed 20% on HW + InA, but reached up to 50% on unmanaged plots at 80 DAP. A mean disease incidence of 45% was recorded on the unmanaged plots at 86 DAT in 2017. HW + InA treatment reduced mean disease incidence by 60% in 2016 and 62.04% in 2017 cropping season at the final date of assessment as compared to unmanaged plots. Conversely, the lowest MLN incidence (20% in 2016 and 17.08% in 2017) was recorded from HW + InA at the final date of assessment. As compared to the two cropping seasons, the highest disease incidence was recorded during 2016 than in 2017 where the former exceeded the later by 10% at final the date of disease assessment. Final MLN incidence was kept generally below ≥50% during the two season experiment (Table ).
Table 2. Influence of insecticide application and hand weeding on MLN incidence at Arba Minch areas in Southern Ethiopia during 2016 and 2017 cropping seasons
3.2. MLN severity
The disease severities on the evaluated treatments during the two cropping seasons are presented in Table . Symptoms noticeable for MLN were observed on leaves and comprised of chlorosis, mosaic and mottling, chlorotic streaks, leaf necrosis, premature drying of leaf sheath and delayed of grain filling on infected maize stands (Figure ). These stands were defoliated after the appearance of the symptom on those plots severely attacked, especially during 2016 compared with 2017 cropping season on the unmanaged plots. The treatments evaluated in this study were very highly significant (p < 0.001) different for MLN severity assessed at 50–80 DAP during 2016 and 66–86 during 2017 cropping seasons. Severity varied from 25% in HW + InA to 66.33% in unmanaged plots in the 2016 cropping season. Similar trends were observed in the 2017 cropping season. As compared to the two cropping seasons, the higher disease severity was recorded on 2016 than 2017. Maize lethal necrotic severity was highest on unmanaged plots with the value of 66.33% in 2016 and 56.33% in 2017. On the contrary, the lowest severity was recorded on the HW + InA with the value of 26.67% in 2016 and 25.00% in 2017 cropping season (Table ). Thus, MLN severity was reduced by 62.31%, 54.27% and 38.18% in 2016, and 52.65%, 50.29% and 23.66% in 2017 on HW + InA, insecticide spray alone and hand weeding over the unmanaged ones, respectively.
Table 3. Influence of insecticide application and hand weeding on MLN severity AUDPC at Arba Minch areas in Southern Ethiopia during 2016 and 2017 cropping seasons
3.3. Area under disease progress curve (AUDPC)
Analysis of variance exhibited highly significant (p < 0.01) differences among the treatments in both cropping years (Table ). As such not much difference in the AUDPC was observed on the insecticide sprayed alone and HW + InA in the two years. In the two years, AUDPC was calculated in the range of 957.60–1392%-days (in 2016) and 900.60–1216.80%-days (in 2017) from the HW + InA to unmanaged plots. The lowest AUDPC values (957.60 and 900.60%-days) were computed from plots managed with HW + InA in 2016 and 2017, respectively. Insecticide spraying in combination with hand weeding lowered AUDPC by 32.21% in 2016 and 25.98% in 2017 as compared to unmanaged plots (Table ).
3.4. Insect pests and their relationship with MLN severity
Both in 2016 and 2017 cropping seasons, insecticide used alone and HW + InA were found to be effective in the reduction of insect pests, which were supposed to be vectors, as compared to control ones (Table ). During experiment, the major identified insect pests were Aphid (Rhopalosiphum maidis), larvae of Maize stalk borer (Busseola fusca) and Fall armyworm (Spodoptera frugiperda) (Table ). The mean numbers of R. maidis and larvae of B. fusca were significantly reduced among the different treatments in the two years. The lowest mean population of R. maidis (33.73 and 29.46 in 2016) and larvae of B. fusca (2.13 and 1.73 in 2017) were recorded in plots managed with HW + InA and insecticide sprayed alone. Whereas, the highest mean population of R. maidis and larvae of B. fusca were recorded in the unmanaged plots in both cropping years (Table ).
Table 4. Mean number of insect pests as influenced by insecticide spray and hand weeding in different pre spray and weeding dates on maize at Arba Minch areas in Southern Ethiopia during 2016 and 2017 cropping seasons
A relationship analysis was made to perceive the association of insect pest infestation with MLN severity per each species in different treatments. As a result, severity was significantly associated (p < 0.05) with higher number of insect pest species in each treatment. The MLN was negatively related with the number of insect pest population per plot (Y = 47.60–0.057a + 0.47b − 0.45c; where a: R. maidis, b: B. fusca and c: S. frugiperda, r = −0.035, −0.032 and −0.34 for each insect pest species, respectively) which was as a result of insecticide application alone and HW + InA (Figure ). Relationship analysis exhibited that unmanaged plots could resulted in higher association than the managed ones. At lower number of insect pest population, the contributions of each insect pest species were higher as compared to individual species. That means the synergistic effect of insect pest population was higher even with low in number than antagonistic effect of insect pest species in higher infestation in development of MLN. As a result, the disease severity was higher at lower insect pest population though at higher number of a single insect pest species the lower MLN severity; in this phenomenon the contribution of a single insect pest species was much lower than a combination of all other insect pest population (Figure ).
Figure 2. Regression analyses of MLN severity and R. maidis, B. fusca and S. frugiperda per plots sprayed with insecticide alone and in combination of insecticide spray and hand weeding at Arba Minch area in Southern Ethiopia during 2016 and 2017 cropping seasons.
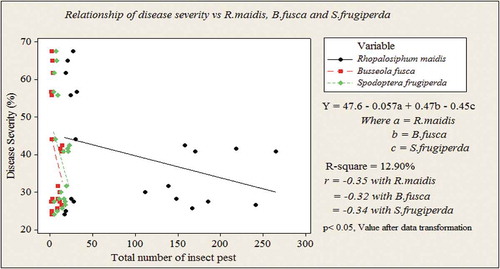
When the insect pest species number getting lower, the disease severity was also lowered, which could resulted from the effect of a single insect pest species though there were higher in number in each treatment. Insecticide spray alone and in combination with hand weeding significantly altered insect pest population within the plot and at the same time relatively better suppression of MLN during the growing periods. Figure shows that insect pest species lowered by insecticide application and hand weeding and only R. maidis was remained as the only important insect pest during the experiments. The distance between the line and all the points indicates whether the analysis has captured a relationship that is strong or weak. Whereas, plots managed with hand weeding alone and unmanaged one showed positively related with the number of insect pest and resulted in the high severity for those particular treatments (Figure ).
3.5. Weed species composition and their relationship with disease severity
The weed species infesting the experimental plots with their corresponding species composition and their mean relative density, frequency, abundance, dominance and IV is presented in Table . More than 20 weed species were identified during the experiment in both cropping seasons. However, for the sake of presentation only 20 dominant weed species are considered and presented in Table . The weed species comprised of broad leaf, grasses and siedges. The weed species consisted of 13 families: Asteraceae with five species, followed by Poaceae, Euphorbiaceae, Commelinaceae and Cyperaceae with four, three, two and two species, respectively. Lamiaceae, Portulacaceae, Tiliaceae, Rubiaceae, Passifloraceae, Loganiaceae and Fabaceae had one species each. Similarly, the weed species noted in the plots were comprised of 16 genera: Euphorbia, Commelina, Digitaria and Cyperus with two species, and the rest of genera had one species for each. In the two cropping seasons, Xanthium strumarium (13.12%, 11.64%, 10.12%, 9.34% and 31.10%) and Galinsoga parviflora (11.44%, 10.02%, 8.83%, 10.26% and 29.11%) were predominant in the plots and had the highest relative WD, frequency, abundance, dominancy and IV, respectively. Among the weed species, Euphorbia heterophylla, Bidens pilosa and Digitaria abyssinica had comparatively higher IVs with 19.44%, 18.83% and 18.47%, respectively, as a forerunner of X. strumarium and G. parviflora in the cropping seasons (Table ).
Table 5. Major weed species composition and their mean relative frequency, abundance, dominance and importance at Arba Minch areas in Southern Ethiopia during 2016 and 2017 cropping seasons
Treatments consisted of HW + InA and weeing practice alone significantly (p < 0.01) affected the weed population and resulted in lower amount of MLN severity in the two years. The MLN severity had positively related with WD per unit area (Y = 0.1236x + 20.55, r = 0.63) (Figure ). The result showed that MLN severity increased with high infestation of weeds (Figure ). The typical distance between the line and all the points indicates whether the relationship between MLN severity and WD has caught a connection, whether strong or weak (Figure ). Taken as a whole, the higher WD in the growing fields, the higher MLN severity to the maize and the stronger the relationship, the line and the points, as shown in the figure.
3.6. Yield related parameters and regression analysis
The effects of insecticide application and hand weeding were highly significant (p < 0.01) different on growth and yield related parameters in both the 2016 and 2017 cropping seasons (Table ). The regression analysis also showed significant influence of insecticide application and hand weeding on growth and yield related parameters (Figure ). LAI, average number of rows per ear, number of healthy ears per plot and number of diseased ears per plot were higher on HW + InA and insecticide spray alone than the unmanaged ones in both cropping years (Table ). However, HW + InA significantly affected the parameters like plant height, average number of kernels per row in ear, dry matter weight and grain yield, followed by insecticide sprayed alone in the two years. But, regarding harvesting index, there was no significant (p > 0.05) difference among the treatments, except for unmanaged ones, in 2016 cropping season. In the 2017 cropping season, harvesting index was significant (p < 0.01) differed on HW + InA and insecticide sprayed alone as compared to hand weeding and unmanaged plots. The parameters measured on HW + InA, insecticide sprayed alone and hand weeding in the year of 2017 were higher than in the year of 2016 cropping season. The unmanaged plots showed lower values in all parameters measured in both cropping seasons (Table ).
Table 6. Effect of MLN on maize growth and yield attributes under integration of insecticide application and hand weeding at Arba Minch areas in Southern Ethiopia during 2016 and 2017 cropping seasons
Figure 4. Estimating relationships between losses in grain yield and AUDPC at Arba Minch areas in Southern Ethiopia during 2016 and 2017 cropping seasons.
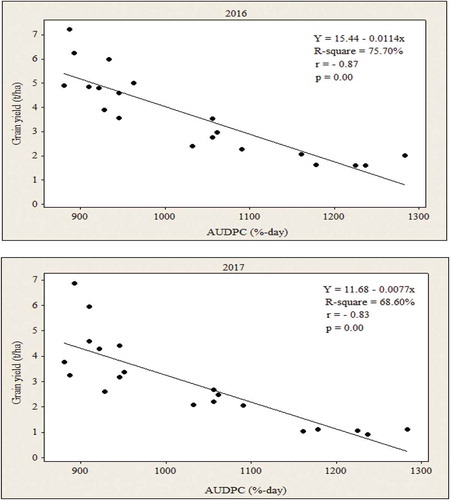
The results of linear regression analysis for each treatment are depicted in Figure . Thus, in both cropping years as it was observed that as AUDPC increases the grain yield decreases, which could indicate the inverse relation between AUDPC and the grain yield. Distance between the line and all the points implied whether the regression analysis has captured a relationship that is strong or weak. This means the closer a line is to the points, the stronger the relationship. On the other hand, based on coefficient of determination (R2), the equation explained about 68.60% in 2016 and 75.70% in 2017 of the variation in grain yield due to AUDPC as a predictor variable. Likewise, the regression equation tried to demonstrate in such a way that for every one unit increased in AUDPC values resulted in grain yield loss of about 0.0077 and 0.0114 t ha−1 in 2016 and 2017 cropping years, respectively (Figure ). Comparison of the two years revealed that the higher grain yield loss due MLN was found in 2016 than in 2017, which exceed by 59.69% on the regression analysis.
3.7. Cost and benefit and relative yield loss analysis
Variation in net benefit (NB), yield advantage (YA) and relative yield loss (RYL) has been observed among the treatments (Table ). Partial budget analysis showed HW + InA gave higher ($ 40.05) NB with MRR of 18.71% though it took the highest (114.44$ ha−1) total input cost than other treatments imposed. Although insecticide sprayed only exhibited the higher controlling effect on insect pests, the NB and MRR were unsatisfactory, and the treatment such as hand weeding and unmanaged ones even resulted in greater benefit than insecticide sprayed. The results implied that use of HW + InA was found better while considering time of insecticide application and hand weeding than other treatments during growing of maize (Table ). On the other hand, variations in grain yield losses were observed among the treatments used to manage MLN in the two cropping seasons (Table ). The highest grain yield losses occurred on the unmanaged plots as compared to the managed ones. In unmanaged plots, a grain yield loss reaching 73.44% was computed compared with HW + InA. Grain yield losses were considerably reduced by HW + InA application, followed by insecticide sprayed alone as compared to the unmanaged plots. Maximum YA of 73.44% was recorded in HW + InA as compared to the unmanaged plots (Table ).
Table 7. Mean economic advantage of insecticide spray and hand weeding under solely and in combination to manage MLN in relation to yield advantage, economic benefit and yield losses at Arba Minch in Southern Ethiopia in during 2016 and 2017 cropping seasons
4. Discussion
MLN is the results of the combined effect of MCMV and SCMV, and one of the most intimidating diseases of maize where the disease has been reported (Adams et al., Citation2014; Demissie et al., Citation2016; Mahuku et al., Citation2015; Mudde et al., Citation2018; Wangai et al., Citation2012a). Under Ethiopian conditions, the combined infection ability of the disease on maize could resulted in a serious risk to subsistence farmers, state and private seed multipliers (Demissie et al., Citation2016). This also holds true in the study areas since the area is located nearby Kenya boarder and a major entrance of the disease through commercialization of planting material and long distance travelling of insect pests (vectors) between the two countries. During the recent outbreak in Kenya the infections rates and yield losses were also high in some cases as high as 100% (Karanja, Derera, Gubba, Mugo, & Wangai., Citation2018; Kusia, Citation2014), and similar circumstances were observed in the maize fields in most of the maize growing areas of southern Ethiopia (Demissie et al., Citation2016).
During experiment, symptoms of MLN were observed within the plots; these consisted of elongated yellow streaks parallel to the leaf veins, mosaic and mottling, chlorotic streaks, dead heart, shorten internodes and male inflorescence, failure to tassel, premature drying of leaf sheath and aging of the plant, leaf necrosis, malformed or no ear, failure of ears to put on grains, brighten chlorosis, delaying of grain filling and stunting. In line with the present study, Nelson, Brewbaker, and Hu (Citation2011), Adams et al. (Citation2014) and Guadie et al. (Citation2018a) who observed that symptoms produced by the disease were typical for MLN. In the meantime, in 2016 cropping season when the MLN appeared for the first time, representative symptomatic leaf samples of maize were tested for MCMV and SCMV and positive results were obtained. This could confirm that MLN is widely spread across the study areas, irrespective of many factors influencing disease epidemiology, vector and alternative host population dynamics in the year (Demissie et al., Citation2016). Similar result was reported by Fentahun, Feyissa, Abraham, and Kwak (Citation2017) and Guadie, Knierim, Winter, Tesfaye, and Abraham (Citation2018b) against MLN in maize plant by detection and characterization of MCMV and SCMV associated with MLN in Ethiopia.
Greater damage to the maize crop is more likely when early plant infection was occurred (Adams et al., Citation2014; Mahuku et al., Citation2015) and this phenomenon was factual for the experimental plots during the growing periods. However, different agronomic practices including disease management tactics can influence the epidemic development of the diseases during the growing period. The MLN is vector transmitted and has many alternate hosts; the disease management should be focused on these factors under field condition. Results showed that combination of more than one disease management tactic exhibited a great influence on MLN during 2016 and 2017 cropping seasons. In the current study, it was observed that incidence, severity and AUDPC were higher on plots managed with hand weeding and unmanaged plots in both cropping seasons. This might have been due to severe insect and weed infestation during the growing periods. The results of the present study also indicated that incidence, severity and AUDPC were significantly reduced by HW + InA and insecticide spray alone at weekly interval starting from the first appearance of insect pest and timely hand weeding of the plots. Nelson et al. (Citation2011), Wangai et al. (Citation2012a) and Redinbaugh and Zambrano-Mendoza (Citation2014) reported that the best approach for the management of virus diseases is to employ integrated pest management practices encompassing cultural control and vector control using foliar sprays on insecticide.
In the two years, the reduction in disease amount were might have been due to the higher sprayed of insecticide and better hand weeding practices beyond the experimental plots and less chances to occur the insect pest, except for S. frugiperda during the growing period, and infestation of weeds; there was outbreak of S. frugiperda in 2017 cropping season and farmers availed much amount of insecticide for the management of S. frugiperda, this might be reduced number insect species in the surrounding. The results showed that sprays of lamdex 5% EC at seven days interval in combination with three times hand weeding practices, HW + InA, could effectively reduce the magnitude of MLN severity. Therefore, it is advisable to use this insecticide accordingly and weeding practices as suggested. This finding was supported by Uyemoto (Citation1983) and ASARECA (Citation2013) who reported that integrated management of MLN through application of insecticide and proper weeding practice significantly reduced disease pressure as compared to lonely applied management option and the unprotected ones. Similarly, Braidwood et al. (Citation2017), Karanja et al. (Citation2018) and Namikoye, Kariuki, Kinyua, Githendu, and Kasina (Citation2018) also indicated that insecticide application in combination with weed management option should be considered in the management of MLN.
In the current study, about three insect species were identified: R. maidis, larvae of B. fusca and S. frugiperda. In unmanaged plots, the presence of high number of insect pests and their interactive effects resulted in highest MLN severity than the managed plots in the two years; this indicated that the insect pests might have a responsible for aggravating the disease development. However, use of management options alters insect pest population with various levels of number during experiment and significantly reduced their synergistic effects. Proper weeding practice along with wise application of insecticide (HW + InA) significantly reduced the effects of insect pests as compared to other management options, insecticide sprayed alone and hand weeding. In agreement with this study Tang, Wu, Ali, and Ren (Citation2013) and Fatma, Tileye, and Ndakidemi (Citation2016) found that insecticide can be used to control vectors that transmit and spread those viruses responsible for MLN. Although the insecticide used in this study was a broad spectrum, having the ability to kill wide ranges insect pest species and a repellant property, which did not affect the population of S. frugiperda since this might have been the inherent ability of the S. frugiperda or ineffectiveness of the insecticide to killed and/or repelled this insect pest during 2017 cropping year.
On the other hand, the relationship results showed that the associations between number of insect pests and management tactics were negative. As the graph showed, synergistic effects of different insect pest population revealed the higher MLN severity than the effects produced by a single insect pest species though there were higher in numbers, R. maidis. This could be the results of HW + InA, which is significantly altered insect pest population within the plot and resulted in better suppression of MLN development. Thus, the better controlling of the vectors through integration of different management options, the better reducing of the effect of vector transmitted disease like MLN since it has a strong relationship between management options in relation to vector controls. Many research reports showed that the two co-infective viruses, MCMV and SCMV, are transmitted mechanically and spread by several insect vectors: R. maidis and larvae of B. fusca (Brault, Uzest, Monsion, Jacquot, & Blanc, Citation2010; Cabanas et al., Citation2013; Kiruwa, Tileye, & Patrick, Citation2016; Nault et al., Citation1978). MLN causing viruses transmitting vectors are also endemic in East Africa (Cabanas et al., Citation2013; De Groote et al., Citation2008).
Maize is a primary host for MLN although several other hosts have been identified (Kusia, Citation2014; Mariki, Citation2017; Scheets, Citation2004). During experiment, several weed species were noted, they dominantly belong to Asteraceae followed by Poaceae family. The host range for the two co-infecting viruses, SCMV and MCMV, are restricted to members of the Poaceae family as of maize and sugarcane being the natural hosts of them, respectively (Kusia et al., Citation2015; Scheets, Citation2004; Shukla, Ward, & Brunt, Citation1994). Shukla et al. (Citation1994), Scheets (Citation2004) and Kusia et al. (Citation2015) had been reported that MCMV and SCMV also found to infect and cause mosaic disease in sugarcane, maize, sorghum, finger millet and weed species especially Poaceae in worldwide. This could confirm our assertion that the presence of weeds could serve as a reservoir of MCMV and SCMV for maize plant in high recorded of MLN severity. Similar to this finding, Kusia (Citation2014) evidently reported that wild grasses, domesticated grasses and cereal crops were found to host MCMV and SCMV either singly or dually although up to date no species of dicotyledons have been identified so far. To this, further study is required to bear out the role of weed species identified in this study whether they are alternative hosts of MCMV, SCMV and others causing MLN.
Regarding the relationships between WD and MLN severity, there were strong relationships between them. In severely infested plots there were higher MLN severities than well managed plots (HW + InA) in both cropping seasons. Likewise, the lower weed infestation resulted in the lower MLN severity since hand weeding practice exhibited high disturbance of alternate hosts and might have avoidable effects on disease development and insect infestations and this aided the crop to be strong and resistant to disease suppression. Trigiano, Windham, and Windham (Citation2008), Wangai et al. (Citation2012b) and Jones (Citation2018) reported that good field sanitation such as proper weed management can be eliminates alternative hosts for potential buildup of insect vectors and viral load and subsequently reducing MLN; however, lack of proper weed management practices could have resulted in offering alternative hosts to MCMV and SCMV (Karanja et al., Citation2018).
The management option such as HW + InA in this study was found to be effective against MLN. Insecticide application in combination with hand weeding also gave highest values of growth and yield related parameters measured than on the rest of treatments in both cropping seasons. This difference might have resulted from the variation in the combination of insecticide application along with hand weeding practice than lonely applied of either insecticide or hand weeding. The lower MLN severity and the higher growth and yield related parameters as a result of integrated management are in agreement with the research reports noted by ASARECA (Citation2013), Mahuku et al. (Citation2015) and Namikoye et al. (Citation2018). On the other hand, results obtained from the relationships analysis between MLN severity and grain yields showed there were strong associations between them; in this regard the higher MLN severity resulted in the lower grain yield.
Likewise, there was high NB and MRR on well managed plots, HW + InA, as compared to insecticide sprayed and hand weeding practice only. This might have been attributed to the high suppressing of the insect pest species and eliminations of weed species at the right time. In due, the high NB from HW + InA could be attributed to high yield and the low NB was attributed to low yield. HW + InA significantly reduced grain yield losses compared with insecticide sprayed and hand weeding only in both cropping seasons. This was because maize on the unmanaged plots botched to set grains due to less capable of leaves to functioned normal physiological processes and reduced ear size due to MLN during the growing periods. The grain yield loss computed in this study could not be solely accredited to MLN, considering other environmental factors, S. frugiperda and mechanical damaged due to wild animals and human involvement were important. Instead, the grain yield enhanced since the different management options, HW + InA, were used as compared to the yield of insecticide sprayed alone, hand weeding and unmanaged ones. Yield losses up to 100% due to MLN have been reported in maize crop in different parts of the world (Adams et al., Citation2014; Demissie et al., Citation2016; Mahuku et al., Citation2015; CitationWangai et al., Citation2012a, 2012b).
Previous studies indicated that MLN cannot be controlled by the use of insecticide or elimination of alternate hosts alone, and suggested that the most effective management option for MLN is came through the use of integrated approaches; combining of cultural practices and insecticidal management of insect vectors, use of biological control and resistant genotypes (ASARECA, Citation2013; Wangai et al., Citation2012b; Zhang et al., Citation2008). However, the overall results of this study revealed that the extent of MLN severity was relatively higher on hand weeding alone and unmanaged plots than HW + InA and insecticide sprayed only in both cropping seasons. As a result, insecticide alone and in combination with hand weeding practice was brought into being relatively safe to reduce insect pests’ effects, and such combination significantly lowered the number of insect pests and gave better management option in management of vector transmitted disease like MLN over the unmanaged one. Mahuku et al. (Citation2015) suggested that intensive integrated insect pest and cultural management practices could be useful for commercial production of virus-free maize crop.
5. Conclusion
The results of the study showed disease incidence, severity; and growth and yield related parameters were varied between the two cropping years. Among treatments evaluated the lowest MLN disease severities were recorded in HW + InA and insecticide spray alone plots in both cropping seasons. Similarly, the highest yield was recorded in HW + InA followed by insecticide spray alone. The relationship analysis exhibited there was a relation between insect pests’ number and WD. This implies that insects and/or weed species might have an effect on the disease initiation and development of MLN. Overall, results showed that HW + InA was found to be better management option and reduced MLN and increased grain yields in both 2016 and 2017 cropping seasons. Thus, it is better to use management option as it gave relatively higher defense against MLN and resulted in higher grain yields and economic returns as compared to the unmanaged one. Therefore, dissemination and popularization of HW + InA to the growers will be very important to increase production and productivity of the maize makes use of it as an alternative management option. Consequently, sustainable disease management has to be focused on a system approach with integrated disease management practices through suppressing of the vectors and elimination of the alternate host during the growing period. Further extensive studies are suggested for evaluation of different management options under different agro-ecological conditions to come up with suitable conclusive management option. Moreover, research is necessary to confirm the role of insect pests and weeds as vector and alternative hosts for MCMV, SCMV, MDMV and WSMV causing MLN, respectively.
Acknowledgements
The authors would like to acknowledge Southern Agricultural Research Institute, SARI, Ethiopia, for financing and providing all the necessary facilities to the study. We express our heartfelt appreciation and gratitude to our staff members at Arba Minch Agricultural Research Center for their assistance in providing us technical support and data collection. We are very thankful to Habtamu Terefe (PhD) (Haramaya University, School of plant science) and Gediyon Tamiru (MSc) (Hawasa Agricultural Research Center, SARI) who helped us in one way or another for the accomplishment of this study.
Additional information
Funding
Notes on contributors
Getachew Gudero Mengesha
Getachew Gudero Mengesha (MSc, BSc) is a plant pathology researcher and staff of the Arba Minch Agricultural Research Center of Southern Agricultural Research Institute, Ethiopia. He has experienced in conducting field experiments in different crops in collaboration with colleagues and alone. He has also experienced in undertaking a comprehensive field survey studies to understand the interaction and association of different biophysical factors to decrease or increase plant disease epidemics. Currently, he has designed and carried out many epidemiological studies under both controlled and natural conditions. He takes account for integrated disease management option and recommend subsistence farmers and private owners who involved in crop production systems to exploit the integrated management option to manage the plant diseases and keep going the productivity of the crops. He is involved in regional, national and international projects focusing on crop protection and production systems in collaboration with colleagues and alone.
References
- Abendroth, L., Elmore, R., Hartzler, R. G., McGrath, C., Mueller, D. S., Munkvold, G. P., … Tylka, G. L. (2009). Corn field guide (pp. 26). Extension and Outreach Publications. Retrieved from http://lib.dr.iastate.edu/extension_pubs/26
- Adams, I. P., Harju, V. A., Hodges, T., Hany, U., Skelton, A., & Rai, S. (2014). First report of maize lethal necrosis disease in Rwanda. New Disease Report, 29: 22. doi:10.5197/j.2044-0588.2014.029.022
- ASARECA. (2013). Workshop to develop a strategic plan for maize lethal necrosis disease for eastern and central Africa. Nairobi, Kenyahttp://www.ndrs.org.uk/article.php?id=029022
- Beyene, Y., Gowda, M., Suresh, L. M., Mugo, S., Olsen, M., Oikeh, S. O., … Prasanna, B. M. (2017). Genetic analysis of tropical maize inbred lines for resistance to maize lethal necrosis disease. Euphytica, 213,23.
- Birch, C. J. (1996). Testing the performance of two maize simulation models in a range of environments. Environmental Software, 11(9), 1.8. doi:10.1016/S0266-9838(96)00031-7
- Bockelman, D. L., Claflin, L. E., & Uyemoto, J. K. (1982). Host range and seed-transmission studies of maize chlorotic mottle virus in grasses and corn. Plant Disease, 66(3), 216–218. doi:10.1094/PD-66-216
- Braidwood, L., Quito-Avila, D. F., Cabanas, D., Bressan, A., Wangai, A., & Baulcombe, D. C. (2017). Maize chlorotic mottle virus exhibits low divergence between differentiated regional subpopulations. Scientific Report, 8, 1173. doi:10.1038/s41598-018-19607-4
- Brault, V., Uzest, M., Monsion, B., Jacquot, E., & Blanc, S. (2010). Aphids as transport devices for plant viruses. Comptes Rendus Biologis, 333, 524–538. doi:10.1016/j.crvi.2010.04.001
- Cabanas, D., Watanabe, S., Higashi, C. H. V., & Bressan, A. (2013). Dissecting the mode of maize chlorotic mottle virus transmission (Tombusviridae: Machlomovirus) by Frankliniella williamsi (Thysanoptera: Thripidae). Journal of Economic Entomology, 106(1), 16–24. doi:10.1603/EC12056
- Campbell, C. L., & Madden, L. V. (1990). Introduction to plant disease epidemiology (1st ed., pp. 532). New York: John Wiley and Sons.
- Castillo, J., & Hebert, T. T. (1974). New virus disease affecting maize in Peru [Nueva enfermedad virosa afectando al maiz en el Peru]. Fitopatologia, 9, 79–84.
- International Maize and Wheat Improvement Center (CIMMYT). (1988). From agronomic data to farmer recommendations: An economics training manual. Completely revised edition (pp. 79). Mexico. DF: CIMMYT. ISBN 968-6127-18-6.
- CSA (Central Statistical Agency). (2015). Agricultural sample survey, 2014/2015. Report on area and production of crops (Private peasant holdings, main season). Central Statistical Agency, Volume 1, No. 578. Addis Ababa, Ethiopia. pp: 10–14.
- CSA (Central Statistical Agency). (2017). Agricultural sample survey, 2016/2017. Report on area and production of crops (Private peasant holdings, main season). Central Statistical Agency, Volume 1, No. 584. Addis Ababa, Ethiopia. pp: 10–18.
- Das, T. K. (2008). Weed science. Basics and application (pp. 901). New Delhi: Jain brothers.
- De Groote, H., Wangare, L., Kanampiu, F., Odendo, M., Diallo, A., Karaya, H., & Friesen, D. (2008). The potential of a herbicide resistant maize technology for Striga control in Africa. Agricultural System, 97, 83–94. doi:10.1016/j.agsy.2007.12.003
- Demissie, G., Deressa, T., Haile, M., Bogale, G., Dida, M., & Chibsa, T. (2016). Prevalence, distribution and impact of maize lethal necrosis disease (MLND) in Ethiopia. Pest Management Journal of Ethiopia, 18, 37–49.
- FAOSTAT. (2015). Statistical databases and data-sets of the food and agriculture organization of the United Nations. Retrieved from http://faostat.fao.org/site/339/default.aspx.
- FAOSTAT. (2017). Production crops E all data. Food and agriculture organization of the United Nations. Retrieved from http://faostat3.fao.org/download/Q/*/E
- Fatma, H. K., Tileye, F., & Ndakidemi, P. A. (2016). Insights of maize lethal necrotic disease: A major constraint to maize production in East Africa. African Journal of Microbiology Research, 10(9), 271–279. doi:10.5897/AJMR2015.7534
- Fentahun, M., Feyissa, T., Abraham, A., & Kwak, H. R. (2017). Detection and characterization of maize chlorotic mottle virus and sugarcane mosaic virus associated with maize lethal necrosis disease in Ethiopia: An emerging threat to maize production in the region. European Journal of Plant Pathology, 149, 1011–1017. doi:10.1007/s10658-017-1229-2
- Gibson, L., & Benson, G. (2002). Origin, History, and Uses of Corn (Zea mays), Iowa State University, Department of Agronomy. Retrieved from http://www.agron.iastate.edu
- Gomez, K. A., & Gomez, A. A. (1984). Statistical procedures for agricultural research (2nd ed., pp. 680). John Wiley and Sons, Inc.
- Gordon, D. T., Bradfute, O. E., Gingery, R. E., Nault, L. R., & Uyemoto, J. K. (1984). Maize chlorotic mottle virus. description of plant viruses, 284 [ed. by association of applied biologists]. Retrieved from http://www.dpvweb.net/dpv/showdpv.php?dpvno=284
- Guadie, D., Abraham, A., Tesfaye, K., Winter, S., Menzel, W., & Knierim, D. (2018a). First report of maize yellow mosaic virus infecting maize (Zea mays) in Ethiopia. Plant Disease, 102(5), 1044. doi:10.1094/PDIS-08-17-1290-PDN
- Guadie, D., Knierim, D., Winter, S., Tesfaye, K., & Abraham, A. (2018b). Survey for the identification and geographical distribution of viruses and virus diseases of maize (Zea mays L.) in Ethiopia. European Journal of Plant Pathology. doi:10.1007/s10658-018-1568-7
- SAS Institute Inc. (2009). SAS/Stat guide for personal computers, version 9.2 edition. Cary, North Carolina, USA: SAS Institute Inc.
- James, W. C., & Shih, C. S. (1973). Size and shape of plots for estimating yield losses from cereal foliage diseases. Experimental Agriculture, 9, 63–71. doi:10.1017/S0014479700023693
- James, W. C., & Teng, P. S. (1979). The quantification of production constraints associated with plant diseases. Applied Biology, 4, 201–267.
- Jensen, S. G. (1985). Laboratory transmission of maize chlorotic mottle virus by three species of corn root worms. Plant Disease, 69(10), 864–868. doi:10.1094/PD-69-864
- Jiang, X. Q., Wilkinson, D. R., & Berry, J. A. (1990). An outbreak of maize chlorotic mottle virus in Hawaii and possible association with thrips. Phytopathology, 80, 1060.
- Jones, D. (2018). Early season maize lethal necrosis disease (MLND) and fall armyworm update. Thinking Agronomy Newsletter. Retrieved from https://cropnuts.com/maize-lethal-necrosis-disease/
- Karanja, J., Derera, J., Gubba, A., Mugo, S., & Wangai., A. (2018). Response of selected maize inbred germplasm to maize lethal necrosis disease and its causative viruses (Sugarcane Mosaic Virus and Maize Chlorotic Mottle Virus) in Kenya. The Open Agriculture Journal, 12, 215–225. doi:10.2174/1874331501812010215
- Kawano, K. (1990). Harvest index and evaluation of major food crop cultivars in the tropics. Euphytica, 46, 195–202. doi:10.1007/BF00027218
- Kiruwa, F. H., Tileye, F., & Patrick, N. (2016). Insights of maize lethal necrotic disease: A major constraint to maize production in East Africa. African Journal of Microbiology Research, 10(9), 271–279. doi:10.5897/AJMR2015.7534
- Kusia, E. S. (2014). Characterization of maize chlorotic mottle virus and sugarcane mosaic virus causing maize lethal necrosis disease and spatial distribution of their alternative hosts in Kenya (MSc Thesis). Pan African University, Institute for Basic Sciences Technology and Innovation. Retrieved from http://hdl.handle.net/123456789/166
- Kusia, E. S., Subramanian, S., Nyasani, J. O., Khamis, F., Villinger, J., Ateka, E. M., & Pappu, H. R. (2015). First report of lethal necrosis disease associated with co-infection of finger millet with maize chlorotic mottle virus and sugarcane mosaic virus in Kenya. Plant Disease, 99(6), 899–900. doi:10.1094/PDIS-10-14-1048-PDN
- Mahuku, G., Lockhart, B. E., Wanjala, B., Jones, M. W., Kimunye, J. N., Stewart, L. R., & Redinbaugh, M. G. (2015). Maize lethal necrosis (MLN), an emerging threat to maize-based food security in sub-Saharan Africa. Phytopathology, 105(7), 956–965. doi:10.1094/PHYTO-12-14-0367-FI
- Mariki, A. (2017). Distribution of maize lethal necrosis disease, its causal viruses and alternative hosts in the north- central regions of Tanzania (MSc Thesis in Crop Science). Makerere University, Kampala, Uganda. p. 90. Retrieved from https.//hdl.handle.net/10568/81213
- Miano, D. W. (2014). Maize lethal necrosis disease: A real threat to food security in the eastern and central Africa region. Nairobi, Kenya. Retrieved from http://www.nuruinternational.org
- Ministry of Agriculture and Natural Resources (MoANR) & Ethiopian Agricultural Transformation Agency (EATA). (2016). Soil fertility status and fertilizer recommendation atlas of the southern nations, nationalities and peoples’ regional state, Ethiopia (pp. 196–197). Addis Ababa, Ethiopia.
- Mudde, B., Olubayo, F. M., Miano, D. W., Asea, G., Kilalo, D. C., Kiggundu, A., … Adriko, J. (2018). Distribution, incidence and severity of maize lethal necrosis disease in major maize growing agro-ecological zones of Uganda. Journal of Agricultural Science, 10(6), 72–85. doi:10.5539/jas.v10n6p72
- Namikoye, E. S., Kariuki, G. M., Kinyua, Z. M., Githendu, M. W., & Kasina, M. (2018). Cropping system intensification as a management method against vectors of viruses causing maize lethal necrosis disease in Kenya. East African Agricultural and Forestry Journal. doi:10.1080/00128325.2018.1456298
- Nault, L. R., Styer, W. E., Coffey, M. E., Gordon, D. T., Negi, L. S., & Niblett, C. L. (1978). Transmission of maize chlorotic mottle virus by chrysomelid beetles. Phytopathology, 68, 1071–1074. doi:10.1094/Phyto-68-1071
- Nelson, S. J., Brewbaker, A., & Hu, J. (2011). Maize chlorotic mottle virus. Plant Disease, 79, 1–6.
- Niblett, C. L., & Claflin, L. E. (1978). Corn lethal necrosis - a new virus disease of corn in Kansas. Plant Disease Reporter, 6(1), 15–19.
- Redinbaugh, M. G., & Zambrano-Mendoza, J. L. (2014). Control of virus diseases in maize. In G. Lobenstein & N. Katis (Eds.), Control of plant virus disease: Seed-propagated crops (pp. 391–429). Waltham, MA USA: Academic Press.
- Robert, G. D., & James, H. T. (1991). Principles of statistics. A biomerical approach. (2nd ed., pp. 633). New York, NY: McGraw-Hill College. ISBN-10: 0070610282; ISBN-13: 978-0070610286.
- Scheets, K. (2004). Maize chlorotic mottle. In H. Lapierrre & P. A. Signoret (Eds.), Viruses and virus diseases of Poaceae (Gramineae) (pp. 642–644). Paris: Institute National de la Recherche Agronomique.
- Shukla, D. D., Ward, C. W., & Brunt, A. A. (1994). The potyviridae. Wallingford, UK: CAB International. ISBN: 0851988644.
- Singh, M., Ashutosh, S., Upadhyaya, P. E., & Rao, G. E. (2005). Transmission studies on an indian isolate of sugarcane mosaic potyvirus. Sugar Technology, 7(2–3), 32–38. doi:10.1007/BF02942526
- Smale, B. D., & Jayne, T. (2011). Maize revolutions in sub-Saharan Africa (World Bank Policy Research Working Paper, No. 5659). Retrieved from http://documents.worldbank.org/curated/en/475801468209965095/pdf/WPS5659.pdf
- Stoud, A., & Parker, T. (1989). Weed identification guide for Ethiopia (pp. 278). FAO publication.
- Tang, L., Wu, J., Ali, S., & Ren, S. (2013). Establishment of the baseline toxicity data to different insecticides for Aphis craccicirora Koch and R. maidis (Fitch). (Homoptera: Aphidian) by glass tube residue film technique. Pakistan Journal of Zoology, 45(2), 411–415.
- Terefe, H., & Getachew, G. (2019). Distribution and association of factors influencing emerging maize lethal necrosis disease epidemics in Southern Ethiopia. Cogent Food and Agriculture, 5, 1683950. doi:10.1080/23311932.2019.1683950
- Tewabech, T., Getachew, A., Fekede, A., & Dagne, W. (2002). Maize pathology research in Ethiopia: A review. pp. 97–105. In: N. Mandefro, D. Tanner, & S. Twumasi-Afriyie. (eds.), Enhancing the Contribution of Maize to Food Security in Ethiopia. Proceedings of the 2nd National Maize Workshop of Ethiopia. Addis Ababa, Ethiopia: EARO and CIMMYT. doi:10.1038/sj/jim/7000217
- Trigiano, R. N., Windham, M. T., & Windham, A. S. (2008). Plant pathology: Concepts and laboratory exercise (Vol. 21, pp. 269). Boca Raton: CRC Press.
- Uyemoto, J. K. (1983). Biology and control of maize chlorotic mottle virus. Plant Disease, 67(1), 7–10. doi:10.1094/PD-67-7
- Uyemoto, J. K., Bockelman, D. L., & Clafin, L. E. (1980). Severe outbreak of maize lethal necrosis disease in Kansas. Plant Disease, 64, 99–100. doi:10.1094/PD-64-99
- Wangai, A., Kinyua, Z. M., Otipa, M. J., Miano, D. W., Kasina, J. M., Leley, P. K., & Mwangi, T. N. (2012b). Maize (corn) Lethal Necrosis (MLN) disease. KARI Information Brochure. Retrieved from http://www.disasterriskreduction.net/fileadmin/user_upload/drought/docs/1%20%20Maize%2Lethal%20Necrosis%20KARI.pdf
- Wangai, A. W., Redinbaugh, M. G., Kinyua, Z. M., Mahuku, G., Sheets, K., & Jeffers, D. (2012a). First report of maize chlorotic mottle virus and maize lethal necrosis in Kenya. Plant Disease, 96(10), 1582–1583. doi:10.1094/PDIS-06-12-0576-PDN
- Wheeler, B. J. (1969). In Mitton, P. J. (Ed.), An introduction to plant diseases (pp. 374). John Wiley and Sons, Inc.
- Xie, L., Zhang, J. Z., Wang, Q. A., Meng, C. M., Hong, J. A., & Zhou, X. P. (2011). Characterization of maize chlorotic mottle virus associated with maize lethal necrosis disease in China. Journal of Phytopathology, 159, 191–193. doi:10.1111/jph.2011.159.issue-3
- Zhang, M. Q., Rao, G. P., Gaur, R. K., Ruan, M. H., Singh, M., Sharma, S. R., … Singh, P. (2008). Characterization, diagnosis and management of plant viruses. Industrial Crops and Products, 1, 111–144.
- Zhao, M., Ho, H., Wu, Y., He, Y., & Li, M. (2014). Western flower thrips (Frankliniella occidentalis) transmits maize chlorotic mottle virus. Journal of Phytopathology, 162, 532–536. doi:10.1111/jph.2014.162.issue-7-8