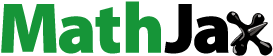
Abstract
Continuous conversion of land for different agricultural production purposes has resulted in degradation of soil resources. Rehabilitating soil quality through sustainable land management is one of the most pressing agenda in agriculture. Hence, this study was conducted to evaluate the trends of major soil quality after the introduction of A. decurrens tree-based farming in Guder watershed. Soil samples were collected from 5 land use types, 2 soil depths, and 3 slope positions with 3 replications and then analysed for selected soil physicochemical quality indicators. Selected soil physicochemical indicators were subjected to three-way ANOVA. The result revealed that the highest clay particles and soil bulk density were recorded at A. decurrens tree farmland and cropland soils, respectively. The maximum soil pH was recorded from natural forest (5.99), subsurface soil layer (5.56) and lower slope (5.57). The lowest soil organic carbon and total nitrogen contents were observed in cropland. Greater available phosphorus (13.58) mg kg−1 was obtained in the topsoil depth of A. decurrens tree farmland at the lower slope. Among the studied soil quality indicators total nitrogen, cation exchange capacity and soil pH were strongly and positively correlated with soil organic carbon. The finding of this study showed that shifting of cereal based into A. decurrens tree-based farming by smallholder farmers can improve the fertility and health of soils and then boost crop productivity. Therefore, the right integration of A. decurrens tree in the farming system can be an alternative approach to reverse soil quality deterioration in degraded agricultural landscapes of Ethiopia.
PUBLIC INTEREST STATEMENT
Land use conversion from cereal-based farming into Acacia decurrens tree-based farming system is now becoming the common practice in the North Western highlands of Ethiopia since the early 1990s. It has been grown extensively by smallholder farmers to rehabilitate their degraded farmlands and abandoned marginal lands. Since A. decurrens is one of the multi-purpose’s legume trees, it improves the soil fertility and enhances soil health. Besides, it has ecological benefits through increasing plantation forest and hence restorating soil quality. It has also creates incentives and job opportunities to the landless youth and diversifies income generation through producing and selling best quality charcoal. This, in turn, avoids unwise land use conversion into agricultural land and clearing of natural vegetation for fuel-wood. Overall, this finding illustrates the promising contributions of A. decurrens tree-based farming practices via restoring soil quality and improving soil fertility. Hence, further studies are recommended under different agro-ecological zones across the country and beyond to prove it for further use.
Competing Interests
The authors declares no competing interests.
1. Introduction
Land use change, deforestation and soil erosion are the most severe environmental problems and major causes of natural resource degradation in the highlands of Ethiopia (Birhan & Assefa, Citation2018; Gelaw et al., Citation2014; Girmay & Singh, Citation2012; Hurni, Citation1993). Declining of soil quality caused by inappropriate land use and poor soil management major environmental problems (Ayoubi et al., Citation2011; Gebreyesus, Citation2013). Inappropriate use of soil resources without considering their suitability is one of the drivers of land degradation (Göl et al., Citation2010; Tadele et al., Citation2013). Degradation of land is a serious problem that has a substantial effect on resource sustainability (Melku et al., Citation2019). Soil degradation is one part of land degradation, comprises physical, chemical, and biological degradation (Lal et al., Citation2003). It has resulted mainly from land use change and soil erosion.
According to Hurni (Citation1993) report, land degradation causes a 1–2 % annual decline of soil productivity from croplands in the Ethiopian highlands due to land degradation. Thus, soil fertility decline, soil acidity and soil erosion resulting from inappropriate land use practices become the major concern for agricultural soil quality in the Ethiopian highlands (Eyasu, Citation2017; Zelleke et al., Citation2010). Many studies demonstrated that soil degradation has mainly happened from anthropogenic land use for different purposes, and consequently, land use changes significantly affect soil quality (Andrews et al., Citation2004; Girmay & Singh, Citation2012; Zobeck et al., Citation2015). Besides, studies by Eyayu et al. (Citation2009) and Asmamaw and Mohammed (Citation2013) indicated that the conversion of forest land into cropland or rangeland results the decline of soil fertility and deterioration of soil quality in Ethiopia. Assessing the soil quality indicators to know the status of soil degradation due to land use change is desirable for the achievement of sustainable landscape management (Melku et al., Citation2019). Because, land use change has a significant impact on soil health by affecting their properties (Abiot & Ewuketu, Citation2017; Ozgoz et al., Citation2013).
The highlands of Ethiopia are characterized by a high level of degradation (Hurni et al., Citation2010). The same author also indicates that the major indicators of soil degradation are low soil organic carbon, low soil pH and high loss of soil through runoff. Guder watershed is part of the highlands of Ethiopia. In past decades, It also confronted major problems of severing land degradation (Hurni, Citation1993; Hurni et al., Citation2010) resulting from frequent cultivation and clearing of existing forests. These results sever soil erosion of the watershed. In addition, soil acidity is a serious problem of crop production in the study area (Birhanu et al., Citation2014). Leaching of cations and soil erosion are the main reasons for soil acidification. Hence, crop production is low owing to a decline in soil fertility emanating from erosion and acidity. Those problems cause the decline of soil quality leading to poor agricultural productivity and food insecurity.
However, the watershed has a remarkable land use change with A. decurrens tree farming system since 1990. This brought a tremendous land use shift from annual crop farming to A. decurrens tree-based farming. Acacia decurrens has been grown extensively by smallholder farmers in the highland agricultural landscape of study watershed (Achamyeleh et al., Citation2015). This farming system is considered as one approach of agroforestry that has a positive effect on restoring soil degradation through the improvement of soil chemical and physical properties and reduces soil erosion with its associated problems (Abiot & Ewuketu, Citation2017; Achamyeleh et al., Citation2015). Thus, A. decurrens tree-based farming is supposed to bring restoration of soil quality which may enhance soil fertility and crop productivity that helps to meet the goals of climate smart agriculture. Therefore, the level of soil degradation or resilience in A. decurrens tree-based system has not yet investigated in the highlands of Ethiopia. Additionally, there is no scientific evidence on the status of soil quality indicators and soil quality index as a result of practicing of A. decurrens tree-based farming after the tree harvest to convince smallholder farmers and policymakers for wider scale-up. Therefore, the present study was conducted to evaluate the effect of the introduction of A. decurrens tree-based farming system upon soil quality indicators in Guder watershed.
2. Materials and Methods
2.1. Description of the study area
The study was conducted in a highland area of Guder watershed, Fagita Lekoma district, Amhara National Regional State of Ethiopia. Guder watershed is located between 11°02ʹ00” to 11°08ʹ00” N latitude and 36°52ʹ00” to 36°58ʹ00” E longitude with altitude ranges from 2259 to 2634 m above sea level. Figure displays the location map of Guder watershed. The total area of the watershed is 7135 ha. According to (Food and Agriculture of the United Nations [FAO, Citation2015]) classification system Nitisols, Luvisols, and Vertisols are the major soil types with a coverage of 76, 18 and 6 percent in the study area. The study area soils are characterized with shallow, moderate to very deep in depth and clay loam to clay texture. The soil types varied from medium to high susceptible to erosion (Bireda, Citation2015).
The study area is a portion of the moist subtropical agro-ecological zone of Ethiopia. It has a long dry season extending from November to April and wet season from May to October. The mean annual temperature varies between 10–24 ° C and receives a mean annual rainfall of 2365 mm (Ethiopia Metrology Agency [EMA], Citation2019). June, July, and August receive the largest shares of the annual rainfall. The major farming system of the watershed is crop-based livestock production. The dominant staple food crops grown are barley (Hordeum vulgare L.), teff (Eragrostis tef Zucc.), wheat (Triticum aestivum L.) and potato (Solanum tuberosum L.). Agricultural products are used as a source of food and cash income (Zerihun et al., Citation2016).
Recently, A. decurrens tree-based farming system is commonly experienced by the larger portion of smallholder farmers as the major source of income from charcoal production and crop production (Achamyeleh, Citation2015; Zerihun et al., Citation2016). Acacia decurrens tree was introduced and started since early 1990 for mainly fuelwood production in Fagita Lekoma district (Achamyeleh, Citation2015). Initially, smallholder farmers are planting this tree with teff crop with a spacing of 0.5 m by 0.5 m as Taungya system (Blanford, Citation1925). Wood products are harvested after five years’ age of the tree. Planting of A. decurrens tree has been widely implemented as a cash crop farming system and then the area coverage has tremendously expanded. These results a remarkable change of land uses from croplands to A. decurrens farming system (Achamyeleh et al., Citation2015). The products from the tree bring attractive economic benefits to smallholder farmers and help to improve soil fertility of the degraded farmlands. The economic return obtained from selling the tree product by converting into charcoal. As a result, smallholder farmers are inspired to plant this tree for a maximum of five years and they grow crops after harvesting of the tree products. Smallholder farmers have been initiated in the keeping of the stands of A. decurrens species in the degraded farmlands have become a dominant feature of the study area in Fagita Lekoma district, North-Western Ethiopia (Abiot & Ewuketu, Citation2017).
2.2. Soil sampling techniques
For this study, soil samples were collected through systematically stratified randomized sampling design modified from the procedure used by De Gruijter et al. (Citation2006), and Vågen and Winowiecki (Citation2013). Systematic stratification of the watershed was done based on land use type and slope position (De Gruijter et al., Citation2006). Five dominant land use types (A. decurrens tree farmland, cropland following A. decurrens tree harvest, cropland, grassland, and natural forest land), and soil depths (topsoil = 0–30 and subsurface = 30–50 cm) and slope positions (lower, middle, and upper) were considered as a factor. Therefore, sampling points were collected randomly with three replications at the same land use type, soil depth, and slope position. In A. decurrens tree farmland, soil samples were composed from farmlands particularly covered with A. decurrens trees at the age of five years before harvesting. Totally nighty soil samples were collected for soil analysis. The details of studied land use types in the Guder watershed are presented in Table .
Disturbed and undisturbed samples were collected for soil analyses. Soil samples were collected from each land use types and slope positions at soil depths increments and then pooled to obtain composite samples for physical and chemical analyses. Before sampling, surface litter was removed smoothly by hand. Auger was used for disturbed soil sampling. For soil bulk density determination, separately undisturbed soil samples were collected using core sampler (inner diameter = 5 cm and height = 5 cm) in five land use types and slope positions at each depth interval after the opening of a small pit with a dimension of 50 cm*50 cm *50 cm. Forty-five soil samples were collected from each topsoil and sub-surface soil sampling points.
Table 1. Description of land use types in Guder watershed
2.3. Preparation and laboratory analysis of soil samples
Composite topsoil and subsurface soil samples collected from the field were air-dried at room temperature, crushed and passed through a 2 mm sieve to remove stones, roots and other unwanted materials. Analysis of soil samples for soil physicochemical properties including soil texture, soil bulk density (SBD), soil moisture content (SMC), soil organic carbon (SOC), available phosphorus (AP), total nitrogen (TN), soil reaction (pH) and cation exchange capacity (CEC) were conducted at the soil and water laboratory of Adet Agricultural Research Center, Ethiopia.
Soil particle size distribution was determined by the Bouyoucous hydrometer method (Bouyoucos, Citation1962). Soil textural class was determined using the USDA textural triangle (United States Department of Agriculture [USDA], Citation2004). Bulk density was determined using the cylindrical metal core sampler method as outlined by Black (Citation1965). The initial weight of the core samples was determined. Then each sample was oven-dried at 105°C for 24 h oC to a constant weight, thereafter weighed. Henceforth, soil bulk density was calculated as the mass of oven-dried soil divided by the core volume of 102.1 cm3 (Black, Citation1965).
where SBD = soil bulk density (gcm−3), Ms = mass of soil after over dry (g), and Vb = bulk volume of the soil (cm3).
Soil moisture content was determined gravimetrically. Though volumetric method is more relevant than a gravimetric basis to express soil moisture content for agricultural purpose, it is also possible to convert gravimetric soil moisture content into volumetric moisture content by multiplying the gravimetric soil moisture content with dry soil bulk density and divided by 1 g cm−3 density of water (Hazelton & Murphy, Citation2007). Soil samples taken from the field were initially weighed followed by oven-drying for 24 hours at 105°C. Lastly, the weight of the oven-dried soil samples was measured. Hence, the gravimetric moisture content of the soil sample was determined following the method described in Hazelton and Murphy (Citation2007) as follows:
where SMC = gravimetric soil water content on a mass basis (%), Wwet = the weight of the wet soil sample (g), and Wdry = the weight of the dried soil sample (g).
The soil reaction (pH) was measured using a digital pH meter in the supernatant suspension of 1:2.5 soils to water ratio (McLean, Citation1982). The SOC determination was carried out by wet combustion according to Walkley and Black (Citation1934) procedure. In this method about 77% of the C is oxidized by potassium dichromate, so a correction factor of 100/77 = 1.3 is used during the calculation. The value of 77 % shows the ineffectiveness of combustion varies with the type of organic matter present. Total nitrogen was done through digestion, distillation and titration method of Kjeldhal method (Bremner & Mulvaney, Citation1982). Available phosphorus and CEC were determined by the Olsen method (Olsen & Sommers, Citation1982) and ammonium acetate extractable procedures (Houba et al., Citation1986).
2.4. Statistical analysis
The studied land use type, soil depth, and slope position were considered as independent variables (factors), whereas soil quality indicators were considered as the dependent variables. Before doing the ANOVA, all datasets on soil quality indicators were tested for normality and homogeneity of variance. Soil data result from the laboratory analysis were subjected to a three-way analysis of variance (ANOVA). After the means showed significant differences, mean comparisons were made by using Duncan`s multiple range test (DMRT) at a 5% level of significance (Duncan, Citation1955). Pearson’s correlation test was done to select the related soil physical and chemical indicators. Descriptive statistics, ANOVA, Pearson’s correlation test, and DMRT were conducted using R version of 3.5.0. and XLSTAT 2014 software (R Core Team, Citation2018).
3. Results
3.1. The effect of land use type, slope and soil depth on selected soil physical indicators
3.1.1. Particle size distribution and soil bulk density
There were statistically significant differences in particle size distribution among the land use types (p < 0.001) and soil depths (p < 0.05) and no significant differences among three factors and slope positions, respectively (Table ). Considering the main effects, the lowest clay content 18.1, 40.7 and 40.5 % were found from natural forest, topsoil layer and upper slope position, respectively. Whereas the highest clay content 54.6 and 48.2 % were found in A. decurrens tree farmland and lower slope position (Table ). Similarly, the lowest silt fraction 22.8, 26.9 and 26.7% were found in A. decurrens farmland, topsoil depth, and lower slope position, respectively (Table ). While the highest silt content 32.4 and 28.2 % were found from natural forest and lower slope positions. In addition, the lowest sand contents 22.6, 26.5 and 25.1 % were found from cropland, sub-surface soil layer, and lower slope position, respectively (Table ). Whereas, the highest sand contents 49.4 and 31.3 % were observed from soils of the natural forest and upper slope position, respectively.
Table 2. Mean square error values from three-way ANOVA for selected soil physicochemical properties under different land use type
Table 3. Mean values (± SE) for soil properties under land use types, soil depth and slope position in Guder watershed
Table 4. Mean (±SE) of particle size distribution and SBD under different land use types
Variation in SBD due to differences in land use types, soil depths, and slope positions was significant (p < 0.01) (Table ). However, it was not affected by the interaction effects of land use type, soil depth, and slope. As a result of the main effects, the highest mean values of SBD were observed from cropland soils (1.24 g cm−3), sub-soil surface (1.19 g cm−3) and upper slope position (1.22 g cm−3) (Table ). Whereas the lowest SBD values were recorded for soils from soil samples of natural forest (1.0 g cm−3) and lower slope position (1.1 g cm−3).
3.1.1.1. Soil moisture content
Mean soil moisture content of soils in the studied area were significantly different (p ≤ 0.05) due to the interaction effects of land use type with soil depth, while variation as a result of the interaction of the three factors was not significantly different (p > 0.05) (Table ). Considering the interaction effects of land use types and soil depths, the highest (36.9 %) and the lowest (22.2 %) mean SMC values were recorded under the sub-surface soil layer of the CLFAD farmland and topsoil layer of the cropland, respectively (Table ).
Table 5. Mean (±SE) soil moisture content (%) at five land use type from two soil depths
Variations of SMC across soils of the studied area significantly (p ≤ 0.05) varied due to the interaction effects of land use and slope position (Table ). Accordingly, the highest (38.0 %) and the lowest (23.2 %) mean SMC values were recorded at the lower slope of the natural forest land and middle slope of the grassland, respectively (Table ).
Table 6. Mean (±SE) soil moisture content (%) due to the interaction effect of major land use types and slope positions
3.2. Land use, slope and soil depth effects on major soil chemical indicators
3.2.1. Soil reaction, soil organic carbon and total nitrogen
Soil pH (H2O) highly significantly varied across land use types (p < 0.001), and significantly affected with increasing soil depths (p < 0.05) and along with slope positions (p < 0.05) (Table ). Nevertheless, it did not show a significant difference (p > 0.05) due to the interaction of land use types and soil depth with slope positions. Accordingly, the lowest pH (H2O) values of 5.0, 5.5 and 5.4 were observed from the cropland (Figure ), sub-surface soil and upper slope position, respectively (Table ). In contrast, the highest pH (H2O) values 6.0 and 5.6 were observed from natural forest land (Figure , Table ) and lower slope positions, respectively (Table ). Soil pH was significantly and positively correlated with SOC, TN and CEC at r = 0.57**, 0.64** and 0.51**, respectively. However, it was correlated negatively with SBD (Table ). In general, the range of soil pH (H2O) of the studied area was between 4.0 and 7.1 (Table ).
Table 7. Mean (±SE) values of pH, SOC and TN under soil depths and slope positions
Table 8. Mean available phosphorus (mg kg−1) due to the interaction effects of land use types with soil depths along with slope positions in Guder watershed
Table 9. The interaction effects of land use types with soil depths on the distribution of available phosphorus (mg kg−1)
Table 10. The mean values of CEC (cmolc kg−1) due to the interaction effects of land use types with soil slope positions
Table 11. The interaction effects of land use types with soil depths along with soil depths on the distribution of CEC (cmolc kg−1)
Table 12. Pearson`s correlation matrix between selected soil physicochemical properties (soil quality indicators) in Guder watershed
Table 13. Summary of descriptive statistics for selected soil quality indicators in Guder Watershed
Figure 2. Variations of soil pH (H2O) under different land use types in Guder watershed
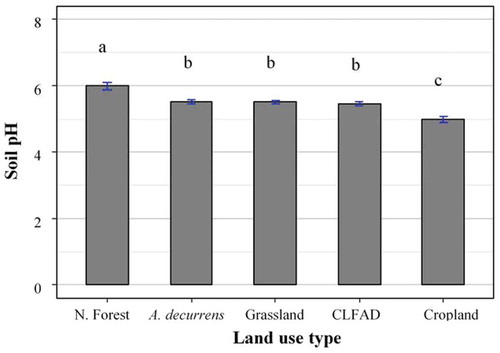
Analysis of variance revealed that the mean SOC content was highly significantly different across all land use types (p < 0.001), soil depths (p < 0.01) and significantly varied at three slope positions (p < 0.05) whereas there was no significant variation in SOC due to interaction effect of studied factors (Table ). In line with this, the highest mean SOC contents 7.3, 3.6 and 3.6 % were recorded in soils of natural forest land (Figure ), topsoil layer and lower slope position, respectively (Table ), while the lowest SOC content 1.8 and 2.7 % were observed from cropland and upper slope positions, respectively (Table ).
Figure 3. Distribution of soil organic carbon at different land use types in Guder watershed
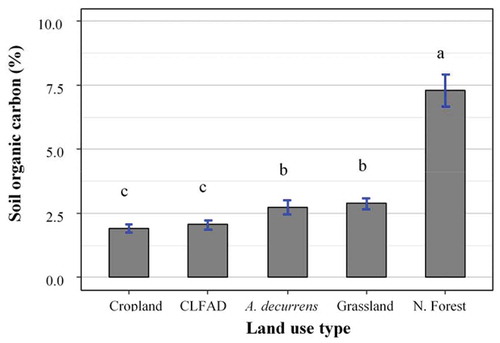
The SOC content of the study watershed was found in the decreasing order of natural forest > grassland > A. decurrens > CLFAD > cropland (Figure ). The result from this study also indicated that the SOC depletion of 74% and 35% were observed as a result of the conversion of the natural forest land into cropland and A. decurrens farmlands to CLFAD farmlands; respectively. Therefore, the change of land use from cropland into A. decurrens farmland was increased the content of SOC from 1.9 to 2.7 % (Table ).
Figure 4. Mean values soil organic carbon of different land use types with increasing of soil depths
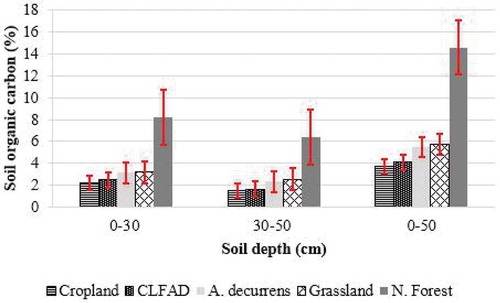
Similar to SOC, the mean total nitrogen content (TN) of the soils was also highly significantly affected by land use types (p < 0.001), soil depths (p < 0.01) and significantly affected by three slope positions (p < 0.05), while the interaction of factors was not affecting the TN (p > 0.05) (Table ). Thus, the highest mean TN contents 0.5 % was recorded from natural forest land (Figure , Table ) followed by 0.3 % for both topsoil and lower slope position (Table ). However, the lowest TN 0.1 and 0.2 % were observed for CLFAD farmlands and upper slope positions in the same order. The variations of TN content among the land use types, soil depths, and slope classes were in line with that of SOC content (Table ; Figure ). As a result, SOC and TN showed a strong positive correlation (r = 0.8; p < 0.001) (Table ). The SOC content of the study area was ranged from 0.7 to 13 % (Table ).
3.2.2. Available phosphorus
The available phosphorus (AP) showed a significant difference (p ≤ 0.05) as a result of the interaction of land use type, soil depth and slope position (Table ). The highest (13.6 mg kg−1) and the lowest (2.5 mg kg−1) mean values of AP were observed from the topsoil layer of the A. decurrens tree farmland soils at the lower slope position and sub-surface soil layers of cropland soils at the upper slope position, respectively (Table ).
The interaction effect of land use types with soil depths as highly significantly (p < 0.001) different on AP (Table ). The lowest AP value 3.5 mg kg−1 was found from the grassland soils of the topsoil layers, while the highest AP value 10.2 mg kg−1 of soil was observed from the topsoil layer of A. decurrens tree farmland (Table ). The result across all land uses from the top half meter soil depth indicated that a higher AP has observed in CLFAD and A. decurrens farmland than other land use types (Figure ). That is the lowest available phosphorus (4.9 mg ha−1) was recorded from cropland whereas A. decurrens farmland improves the available phosphorus from 4.9 to 6.6 mg ha−1 (Table ). The AP content of the studied watershed ranged from 1.7 to 16.6 mg kg−1 (Table ).
Figure 6. Mean values of available phosphorus across soils of different land use types at top half meter of the soil layer in Guder watershed
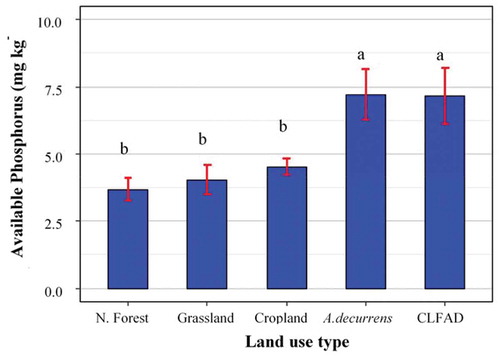
3.2.3. Cation exchange capacity
The cation exchange capacity (CEC) of the soils in the study site was varied significantly (p ≤ 0.01) as a result of the interaction effect of land use types with slope positions (Table ). The highest CEC (48.1) and the lowest value (30.4) cmolc kg−1 were found at the topsoil of the natural forest and the sub-surface layers of cropland, respectively (Table ). The correlation result was showed a strong positive correlation (r = 0.6**) of CEC with SOC (Table ). Similarly, the interaction effect of land use and soil depth was significantly different (p < 0.01) on soil CEC (Table ). Then, the lowest CEC value 29.5 cmolc kg−1 was registered in the cropland soils of the upper slope position, while the highest CEC value 49.6 cmolc kg−1 was recorded in natural forest soils of lower slope position (Table ). The land use change significantly affects available phosphorus. Soil CEC of the study area was ranged from 25.6 to 59.1 cmolc kg−1 (Table ).
4. Discussion
4.1. The effect of land use, slope and soil depth on selected soil physicochemical indicators
4.1.1. Physical soil quality indicators
4.1.1.1. Particle size distribution
Sand particles were higher in forest land soils compared to other land use types as a similar result is reported by Nahusenay and Kibebew (Citation2016). Slope gradient and soil depth with A. decurrens farming system have an effect on the variations of particle size distribution. The slope gradient differences have also an impact on particle size distribution due to the removal of the finer particles (mainly clay particles) by erosion at the upper slope areas while deposition of these particles occurs in the lower slope areas. On the other hand, land use change as a result of the planting of trees (A. decurrens) might have reduced erosion of fine particles and thus contribute for the high fine silt and clay fraction in the relatively lower slope position areas.
4.1.1.2. Soil bulk density
The lower SBD on natural forest could be related to the continuous addition of higher soil organic residues from forest trees litters on the surface soil layer in which the higher soil organic matter (SOM) content resulted increasing soil porosity, enhancing aggregates and fewer disturbances. This result is in accordance with the findings of Eyayu et al. (Citation2009) and Wondimagegn et al. (Citation2018), who reported that SBD under natural forest was lower than that of cropland and other land use types. On the other hand, higher SBD on cropland is due to continuous cultivation, which in turn lower SOC and thereby increased in SBD. Similarly, Yihenew et al. (Citation2015) and Berhanu (Citation2016) indicated a significantly higher bulk density under cropland as compared to other land uses in Northern and Central highlands of Ethiopia. Concerning, soil depth, SBD was found increasing downward for all land use types because of a decline in SOC with increasing soil depth (Table ). Similar findings were reported by Getahun et al. (Citation2014), Berhanu (Citation2016), and Tariku et al. (Citation2017) who indicated that the SBD is increased along with soil depth in a different part of Ethiopia. Moreover, the SBD was higher at soils of cropland followed by A. decurrens farmland in the study area.
The lowest SBD at the topsoil layer of the forest land could be attributed to the relatively high soil organic carbon derived from organic matter. In all the studied land use types, SBD increased parallel with increasing depth from topsoil to sub-surface soil layers. This is basically due to a decline in SOM contents along with the depth, poor aggregation, difficulty in root penetration and more compaction of the sub-surface compared to the topsoil layer. Eyayu et al. (Citation2009); Fantaw et al. (Citation2006) and Worku et al. (Citation2017) indicated that the increased SBD in the lower layer might be due to the compaction resulting from the weight of the topsoil layer. The SBD of the studied soils was found with the specified range as was suggested by White (Citation1997) who indicated that SBD is less than 1 g cm−3 in high SOM soils of natural forest land and it is largely affected by land use types, soil depths, and slope positions. Relatively the soil bulk density is increased under the cropland compared to A. decurrens farmland (Abiot & Ewuketu, Citation2017). The highest SMC under natural forest in the lower slope could be attributed to the addition of organic carbon. This finding is agreed with Fantaw (Citation2017) who found that higher available water content in the lower landscape position due to the combined effects of SOC and clay particles. In contrast, Bahilu et al. (Citation2014) observed that higher SMC in the upper slope position compared with lower slope due to the presence of trees and shrub covers which protects the soil from direct sunlight and makes the soil cool.
4.1.2. Soil chemical quality indicators
4.1.2.1. Soil reaction
As compared to the pH of forest land and A. decurrens farmlands, the pH of soils of cropland was lower that is similar to Birhanu et al. (Citation2014) who reported a similar result in Fagita Lakoma district, North Western Ethiopia. The soil reaction in cropland is generally characterized by lower levels of pH than other land use types (Alemayehu & Assefa, Citation2016). That is why 13.2% of Ethiopian soils are rated from strong to moderately acidic (pH<5.5) (Ermias et al., Citation2013). The relatively lower mean value of soil pH under the cropland might be due to either depletion and removal of basic cations as a result of continuous soil tillage and soil erosion that provides excess H ions to the soil solution that increases acidity. According to Achamyeleh et al. (Citation2015) and Yihenew et al. (Citation2015) land use change and soil management practices have remarkably influenced the soil pH which is consistent with the finding of this study.
The highest pH in the sub-surface soil layer could be the result of the accumulation of basic cations. Soil pH of subsurface depths had higher pH values than surface soil samples in all slope positions across the five land use types. This result is agreed with the findings with Tariku et al. (Citation2017) who indicated that an increasing trend of soil pH with increasing soil depth. According to Mohammed (Citation2003) and Yihenew et al. (Citation2015) soils in higher slopes had low pH values, this might be due to the washing away of solutes and basic cations. Soil pH was positively and significantly related to SOC, TN, and CEC which indicated that soil with higher pH has a significant nutrient availability for plant growth. According to the interpretation of pH suggested by Tekalign (Citation1991) soils of the study area ranged between strongly acidic to neutral.
4.1.2.2. Soil organic carbon
The lowest SOC was recorded in cropland and highest in the natural forest land and this result is in harmony with Yihenew et al. (Citation2015) and Nega and Heluf (Citation2009). Although a higher value of SOC was found at natural forest land, A. decurrens tree farmlands were sequestered relatively similar contributions with grassland into soil carbon pool of the study area. This is because of biomass transfer from A. decurrens tree into soil compared to cropland. This result was parallel with findings of Achamyeleh et al. (Citation2015) and Abiot and Ewuketu (Citation2017) who indicated that higher SOC was obtained from A. decurrens farmland compared to cropland.
According to Smith (Citation2007) land use determines the amount of carbon being sequestered into the soil and mainly in forest systems tend to have the largest input of C to the soil. Consequently, the forest land and A. decurrens tree farmlands were characterized with higher levels of SOC compared to other land use types that was in line with the finding of Aumtong et al. (Citation2009) and Don et al. (Citation2011) who reported that forest land had more SOC than other land use types. This could be attributed to the addition of biomass from trees. Thus, a higher amount of SOC indicated that healthy soil status with good soil structure (Food and Agriculture of the United Nations [FAO, Citation2012]). In addition, A. decurrens tree farmland soils contribute a significant amount of organic matter compared to cropland soils. On the contrary, lack of biomass addition to soil, removal of crop residue, and continuous tillage practices in cropland aggravate carbon losses from cropland (Baker et al., Citation2007). Moreover, the lower SOC in soils of the cropland could be a result of low C input from organic sources, high organic matter decomposition, and severe soil erosion.
Higher depletion of SOC was observed from the conversion of natural forest land into cropland. This finding is matched with other findings of Don et al. (Citation2011) who reported that 25% SOC losses due to the conversion of forest land to cropland. Besides, significant depletion of SOC from soils following the conversion of land use change has been reported in many studies
(Birhan & Assefa, Citation2017; Girmay et al., Citation2008; Hurni et al., Citation2010). Therefore, degradation of Ethiopian soil in the highlands is a well-recognized fact that resulted in a huge loss of fertile topsoil manifested with low soil C content. Although land use change causes depletion of soil carbon, conversion of the cropland into A. decurrens farmlands restored 30 % of SOC into soil pool in the study area. This finding is supported by Don et al. (Citation2011) who showed that the afforestation of degraded agricultural land is contributed by about 29 % SOC into the soil pool. Hence, the rehabilitation of infertile cropland is an entry point for the management of degraded agricultural landscapes of Ethiopian highlands. Generally, SOC of the present study area was in the range very low to high based on the rating of Tekalign (Citation1991).
4.1.2.3. Total nitrogen
The variations of TN content among the land use types, soil depths, and slope classes were in line with that of SOC content. Heshmati et al. (Citation2011) and Yihenew and Ayanna (Citation2013) reported that low TN content in soils of croplands as compared to natural forest lands that similar trend of finding with this research. This is because of low organic material input into the cropping system as a result of the complete removal of crop residue and livestock dung for animal feed and domestic energy, respectively. This finding could be related to the organic matter contents of the soil as looked in the previous presentations of the SOC. Most of the TN is found in organic form and plant litter might have contributed to relatively higher TN in natural forest soils. Besides, Tariku et al. (Citation2017) indicated that mineralization and nitrogen release was higher at the surface soil layer as these layers are exposed to temperature more than the sub-surface soil layers. However, disturbance due to land use change and continuous tillage might have been resulted in a reduction of TN in CLFAD land compared to natural forest land and cropland. Overall, TN was decreased with increasing soil depth in all land use types along topo-sequence downslope. This result is agreed with research findings by Duguma et al. (Citation2010), Abera and Taye (Citation2011), and Tariku et al. (Citation2017) who found a decreasing trend of TN content with increasing soil depths in Central Highlands of Ethiopia.
The higher TN on the topsoil layer (0–30 cm) was directly related to the addition of higher SOM on the surface soil. About 90–95% of nitrogen is derived from SOM sources in unfertilized soils (Miller & Donahue, Citation1997). As a result, SOC and TN showed a strong positive correlation and this finding is in line with the finding of Asmamaw and Mohammed (Citation2013) who obtained a significant relation of TN and SOC.
4.1.2.4. Available phosphorus
The result clearly showed the remarkable contribution of A. decurrens tree farming system into the soil phosphorus pool through the addition of SOM. This result is supported by the finding of Wakene and Heluf (Citation2003) who indicated that the dynamics of soil P affected by land use types through changes in vegetation cover, biomass production, and nutrient cycling. Similar studies by Nega and Heluf (Citation2009), Abebe & Endalkachew (Citation2012), Yihenew and Ayanna (Citation2013), and Nahusenay and Kibebew (Citation2016) indicated that SOM contributed for AP through the formation of organophosphate complexes that are more easily assimilated by plants and anion replacement of H2PO− from adsorption sites. However, earlier studies of Murphy (Citation1968) and Abebe & Endalkachew (Citation2012) showed that low availability of phosphorus in most Ethiopian soils is observed due to complete crop harvest and removal from the field, soil erosion, phosphorus fixation and low accumulation of SOM content in the cropland of agricultural landscapes which is agreed with the results of the present study.
The higher AP in A. decurrens farmland compared to cropland in which the plating of tree in acidic soils of the study area may reduce the fixation of AP and released from oxidation of SOM in which the results are in line with the findings of Tadele et al. (Citation2019) and Achamyeleh et al. (Citation2015). Accordingly, relatively higher values of AP were found in CLFAD farmland. This significantly increased AP could be attributed due to heated area soils during the preparation of charcoal after tree harvest as indicated by Tadele et al. (Citation2019). Available phosphorus had significantly increased in the heated soils as a result of charcoal making processes after harvesting of A. decurrens. The change of land use from cropland into A. decurrens and CLFAD farmland improves the level of soil AP from low to medium based on the rating of Tekalign (Citation1991). This finding concurs with Giardina et al. (Citation2000) who reported higher phosphorus amount for topsoil depth from studied soils after burning of forest biomass compared to unburned site soils, this indicated that a portion of the AP contained in tree biomass was transferred into soil during burning and the soil AP was higher due to diffusion process via soil heating. Available phosphorus of the study watershed is found in the range of low to high (Tekalign, Citation1991).
4.1.2.5. Cation exchange capacity
The higher value of CEC in the natural forest at lower slop could be related to the addition of SOM that provides negatively charged surfaces that play an important role in the exchange process. Whereas the soil CEC values in the cropland declined because of the removal of organic sources such as crop residue. This can be expressed and supported through a strong
positive correlation of CEC with SOC. The findings of the present study coincide with the results of Bewket and Stroosnijder (Citation2003) and Getahun and Bobe (Citation2015) who indicated that the highest and lowest CEC values were observed in soils of forest land and cropland, respectively. As compared to clay particles, SOM provides more negatively charged surfaces. Hence, SOM plays a vital role in the exchange of charges.
According to Gebeyaw (Citation2007) deforestation, leaching of cations, low addition of crop residue in the soil, continuous cropping, and soil erosion have contributed to the depletion of basic cations and resulted in low CEC on the cropland compared to other adjust land uses in highlands of Ethiopia. Higher CEC value of the soil is a sign of substantial buffering capacity against induced change and indicator of high nutrient reserves. Based on the CEC ratings suggested by Hazelton and Murphy (Citation2007) soil of Guder watershed was rated as moderate to very high.
5. Conclusions
The results of this research showed that studied soil quality indicators were significantly affected by land use changes as a result of the introduction of A. decurrens tree under the topsoil and subsurface soil depths as well as slope position of the watershed. The finding of this study showed that A. decurrens plantation is an appropriate land use intervention to enhance the soil quality indicators including soil pH, soil organic carbon, total nitrogen, cation exchange capacity and available phosphorus. The result also indicated that cropland following A. decurrens tree harvest as a Taungya system is an alternative land use management to increase soil pH, soil organic carbon and available phosphorus contents and then improves the soil quality. However, soils of cropland showed less soil pH, soil organic carbon, total nitrogen, cation exchange capacity, and available phosphorus while soil bulk density was increased and this land use showed severe deterioration of the soil quality indicators. As a result, cropland results in low soil fertility that could lead to unsustainable agriculture. Considering the slope of the area, soil organic carbon contents, pH and total nitrogen significantly increased downslope.
Restoration of soil quality in the landscapes of study watershed helps to improve the resilience capacities of soils to climate change effects including drought. Thus, efforts to maintain soil quality by increasing soil organic matter levels in soils by planting trees in agricultural landscapes should be encouraged and promoted. Hence, the new and innovative form of A. decurrens tree-based farming system practiced by smallholder farmers could be considered as a climate-smart landscape management approach to restore soil quality.
Authors’ contributions
All authors have made their equal contributions in designing the research proposal, analysing data and producing this manuscript.
correction
This article has been republished with minor changes. These changes do not impact the academic content of the article.
Acknowledgements
The authors are greatly thankful to African Center of Excellence for Climate Smart Agriculture, Haramaya University, Ethiopia for providing financial support. We also appreciate the smallholder farmers in Guder watershed for allowing us to collect soil samples on their farmland.
Disclosure statement
The authors have declared no conflict of interest.
Additional information
Funding
Notes on contributors

Zerfu Bazie
Zerfu Bazie is a researcher at Adet Agricultural Research Center, Ethiopia. His research interest focuses on the generation of climate smart soil management technologies that increase agricultural productivity and reduce the emission of greenhouse gases as well as enhances the resilient of smallholder farmers during climate shocks.
Samuel Feyssa
Samuel Feyssa (PhD) is an Assistant Professor at the School of Natural Resources Management and Environmental Sciences, Haramaya University, Ethiopia. His area of research work focuses on soil fertility management, soil genesis, and plant nutrition. He advises many postgraduate students at Haramaya University.
Tadele Amare
Tadele Amare (PhD) is a Senior Researcher in Soil and Water Management at Adet Agricultural Research Center, Ethiopia. He engaged in several research activities as principal investigator. He has a good number of publications. His researches focus on sustainable land management, increasing crop productivity through prudent plant nutrients application, modeling on crop responses to plant nutrients, integrated soil fertility management, and spectroscopic based soil analysis.
References
- Abebe, N., & Endalkachew, K. (2012). The contribution of coffee agro-ecotype to soil fertility in Southwestern Ethiopia. African Journal of Agricultural Research, 7(1), 74–25.
- Abera, Y., & Taye, B. (2011). Effect of land use on soil organic carbon and nitrogen in soils of Bale, Southeastern Ethiopia. Tropical and Subtropical Agroecosystems, 14(1), 229–235.
- Abiot, M., & Ewuketu, L. (2017). Effects of Acacia decurrens (Green wattle) tree on selected soil physico-chemical properties North Western Ethiopia. Research Journal of Agriculture and Environmental Management, 6(5), 95–103.
- Achamyeleh, K. (2015). Integration of Acacia decurrens (J.C. Wendl.) Willd. into the farming system, its effects on soil fertility and comparative economic advantages in North Western Ethiopia [MSc Thesis]. Bahir Dar University.
- Achamyeleh, K., Enyew, A., Hailemariam, K., Zerihun, N., Tsugiyuki, M., Atsushi, T., & Haregewyn, N. (2015). Acacia decurrens tree-based farming system for improving land restoration and income: A case study of farmers’ practice in Northwesters Ethiopia. https://doi.org/10.13140/RG.2.1.4672.6165
- Alemayehu, A., & Assefa, A. (2016). Effects of land use changes on the dynamics of selected soil properties in northeast Wellega, Ethiopia. Soil, 2(1), 63–70. https://doi.org/10.5194/soil-2-63-2016
- Andrews, S. S., Karlen, D. L., & Cambardella, C. A. (2004). The soil management assessment framework. Soil Science Society of America Journal, 68(6), 1945–1962. https://doi.org/10.2136/sssaj2004.1945
- Asmamaw, L., & Mohammed, A. (2013). Effects of slope gradient and changes in land use/cover on selected soil physico- biochemical properties of the Gerado catchment, North-Eastern Ethiopia. International Journal of Environmental Studies, 70(1), 111–125. https://doi.org/10.1080/00207233.2012.751167
- Aumtong, S., Magid, J., Bruun, S., & de Neergaard, A. (2009). Relating soil carbon fractions to land use in sloping uplands in northern Thailand. Agriculture, Ecosystems and Environment, 131(3–4), 229–239. https://doi.org/10.1016/j.agee.2009.01.013
- Ayoubi, S., Khormali, F., Sahrawat, K. L., & Rodrigues de Lima, A. C. (2011). Assessing impacts of land use change on soil quality indicators in a loessial soil in Golestan Province, Iran. Journal of Agricultural Science and Technology, 13(5), 727–742.
- Bahilu, B., Mulugeta, L., & Alemayehu, R. (2014). Soil fertility status as affected by different land use types and topographic positions: A case of delta sub-Watershed, Southwestern Ethiopia. Journal of Biology, Agriculture and Healthcare, 4(27), 91–105.
- Baker, J. M., Ochsner, T. E., Venterea, R. T., & Griffis, T. J. (2007). Tillage and soil carbon sequestration: What do we really know? Agriculture. Ecosystems and Environment, 118(1–4), 1–5. https://doi.org/10.1016/j.agee.2006.05.014
- Berhanu, S. (2016). Assessment of soil fertility status of Vertisols under selected three land uses in Girar Jarso District of North Shoa zone, oromia national regional state, Ethiopia. Environmental Systems Research, 5(1), 18. https://doi.org/10.1186/s40068-016-0069-y
- Bewket, W., & Stroosnijder, L. (2003). Effects of agroecological land use succession on soil properties in Chemoga watershed, Blue Nile basin, Ethiopia. Geoderma, 111(1–2), 85–98. https://doi.org/10.1016/S0016-7061(02)00255-0
- Bireda, A. (2015) GIS and remote sensing-based land use/land cover change detection and prediction in Fagita Lekoma woreda, Awi zone, North Western Ethiopia [MSc. Thesis]. Adis Abeba University.
- Birhan, A., & Assefa, A. (2017). Spatial variability of selected soil properties in relation to land use and slope position in Gelana sub-watershed, Northern highlands of Ethiopia. Physical Geography, 39(3), 230–245. https://doi.org/10.1080/02723646.20171380972
- Birhan, A., & Assefa, A. (2018). Land use/land cover changes and their environmental implications in the Gelana sub-watershed of Northern highlands of Ethiopia. Environmental Systems Research, 6(1), 7. https://doi.org/10.1186/s40068-017-0084-7
- Birhanu, A., Enyew, A., & Argaw, M. (2014). Impact of land use types on soil acidity in the highlands of Ethiopia : The case of Fageta Lekoma district. Environmental Sciences, 2(8), 124–132. http://dx.doi.org/10.15413/ajes.2013.035
- Black, C. A. (1965). Methods of soil analysis, part II. Amer. Soc. Inc. Pub.
- Blanford, H. R. (1925). Regeneration with the assistance of Taungya in Burma. Indian Forest records xi, Part III, 41.
- Bouyoucos, G. J. (1962). Hydrometer method improved for making particle size analyses of soils 1. Agronomy Journal, 54(5), 464–465. https://doi.org/10.2134/agronj1962.00021962005400050028x
- Bremner, J. M., & Mulvaney, C. S. (1982). Nitrogen-Total 1. Methods of soil analysis. Part 2. Chemical and microbiological properties, (methodsofsoilan2). Madison, Wisconsin: American Society of Agronomy, Soil Science Society of America.
- De Gruijter, J., Brus, D., Bierkens, M., & Knotters, M. (2006). Sampling for natural resource monitoring. Springer.
- Don, A., Schumacher, J., & Freibauer, A. (2011). Impact of tropical land-use change on soil organic carbon stocks a meta-analysis. Global Change Biology, 17(4), 1658–1670. https://doi.org/10.1111/j.1365-2486.2010.02336.x
- Duguma, L., Hager, H. E., & Sieghardt, M. (2010). Effects of land use types on soil chemical properties in smallholder farmers of central highland Ethiopia. Ecology (Bratislava), 29(1), 1–14. https://doi.org/10.4149/ekol_2010_01_1
- Duncan, D. B. (1955). Multiple range and multiple F tests. Biometrics, 11(1), 1–42. https://doi.org/10.2307/3001478
- EMA. (2019). Records of weather data from 2008 to 2018 in Bahir Dar branch. Ethiopia Metrological Agency.
- Ermias, A., Shimelis, H., Mark, L., & Fentahun, M. (2013). Aluminum tolerance in cereals: A potential component of integrated acid soil management in Ethiopia. Ethiopia Journal of Natural Resources, 13(1), 43–66.
- Eyasu, E. (2017). Characteristics of Nitisol profiles as affected by land use type and slope class in some Ethiopian Highlands. Environmental Systems Research, 6(1), 20. https://doi.org/10.1186/s40068-017-0097-2
- Eyayu, M., Heluf, G., & Tekalign, M. (2009). Effects of land-use change on selected soil properties in the Tera Gedam catchment and adjacent agroecosystems, north-west Ethiopia. Ethiopian Journal of Natural Resources, 11(1), 35–62.
- Fantaw, Y. (2017). Effect of landscape positions on soil properties in an agricultural land a transect study in the main rift valley area of Ethiopia effect of landscape positions on soil properties in an agricultural land a transect study in the main Rift Valley area of Et. Journal of Science and Development, 5(1), 21–31.
- Fantaw, Y., Ledin, S., & Abdu, A. (2006). Soil property variations in relation to topographic aspect and vegetation community in the south-eastern highlands of Ethiopia. Forest Ecology and Management, 232(1–3), 90–99. https://doi.org/10.1016/j.foreco.2006.05.055
- FAO. (2012). Global Agro‐ecological Zones. IIASA. Food and Agricultural Organization of the United Nations.
- FAO. (2015). World Reference Base for Soil Resources. The international soil classification system for naming soils and creating legends for soil maps. (World Soil Resources 2014 Reports No. 106). Food and Agricultural Organization of the United Nations.
- Gebeyaw, T. (2007). Soil Fertility Status As Influenced By Different Land Uses in Maybar Areas of South Wello Zone, North Ethiopia [MSc Thesis]. Haramaya University.
- Gebreyesus, B. (2013). Soil quality indicators response to land use and soil management systems in Northern Ethiopia’s Catchment. Land Degradation & Development, 27(2), 438–448. http://doi.org/10.1002/ldr.2245
- Gelaw, A., Singh, B. R., & Lal, R. (2014). Soil organic carbon and total nitrogen stocks under different land uses in a semi-arid watershed in Tigray, Northern Ethiopia. Agriculture, Ecosystems and Environment, 188, 256–263. https://doi.org/10.1016/j.agee.2014.02.035
- Getahun, B., & Bobe, B. (2015). Impacts of land use types on selected soil physico-chemical physico chemical properties. Science, Technology and Arts Research Journal, 4(4), 40–44. https://doi.org/10.4314/star.v4i4.6
- Getahun, H., Mulugeta, L., Fisseha, I., & Feyera, S. (2014). Impacts of land uses changes on soil fertility, carbon and nitrogen stock under smallholder farmers in central highlands of Ethiopia: Implication for sustainable agricultural landscape management around Butajira area. New York Science Journal, 7(2), 27–44.
- Giardina, C. P., Sanford, R. L., & Døckersmith, I. C. (2000). Changes in soil phosphorus and nitrogen during the slash-and-burn clearing of a dry tropical forest. Soil Science Society of America Journal, 64(2014), 399–405. https://doi.org/10.2136/sssaj2000.641399x
- Girmay, G., & Singh, B. R. (2012). Changes in soil organic carbon stocks and soil quality: Land-use system effects in northern Ethiopia. Acta Agriculturae Scandinavica, Section B Soil and Plant Science, 62(6), 519–530. https://doi.org/10.1080/09064710.2012.663786
- Girmay, G., Singh, B. R., Mitiku, H., Borresen, T., & Lal, R. (2008). Carbon Stocks in Ethiopian soils in relation to land use and soil management. Journal of Land Degradation and Development, 19(4), 351–367. https://doi.org/10.1002/ldr.v19:4
- Göl, C., Çakir, M., Edis, S., & Yilmaz, H. (2010). The effects of land use/land cover change and demographic processes (1950–2008) on soil properties in the Gökçay catchment, Turkey. African Journal of Agricultural Research, 4(13), 1670–1677. https://doi.org/10.5897/AJAR09.716
- Hazelton, P., & Murphy, B. (2007). Interpreting soil test results: What do all the numbers mean? (2nd ed.). CSIRO Publishing.
- Heshmati, M., Arifin, A., Shamshuddin, J., Majid, N. M., & Ghaituri, M. (2011). Factors affecting landslides occurrence in agro-ecological zones in the Merek catchment, Iran. Journal of Arid Environments, 75(11), 1072–1082. https://doi.org/10.1016/j.jaridenv.2011.06.011
- Houba, V. J. G., Novozamsky, I., Huybregts, A. W. M., & Van der Lee, J. J. (1986). Comparison of soil extractions by 0.01M CaCl2, by EUF and by some conventional extraction procedures. Plant and Soil, 96(3), 433–437. https://doi.org/10.1007/BF02375149
- Hurni, H. (1993). World soil erosion and Conservation: Land degradation, famine, and land resource scenarios in Ethiopia (In Piment). World Soil Erosion and Conservation.
- Hurni, H., Abate, S., Amare, B., Berhanu, D., Ludi, E., Portner, B. Y., & Gete, Z. (2010). Land degradation and sustainable land management in the highlands of Ethiopia. North-South perspectives.
- Lal, R., Iivari, T., & Kimble, J. M. (2003). Soil degradation in the United States: Extent, severity, and trends. CRC Press.
- Landon, J. R. (1991). Booker tropical soil manual: A handbook for soil survey and agricultural land evaluation in the tropics and subtropics. Longman Scientific and Technical.
- McLean, E. O. (1982). Soil pH and lime requirement. In A. L. Page, R. H. Miller, & D. R. Keeney (Eds.), Methods of Soil Analysis, Part 2 (pp. 199–224). American Society of Agronomy.
- Melku, D., Awdenegest, M., & Kebede, A. (2019). Effects of land uses on soil quality indicators: The case of Geshy subcatchment, Gojeb River Catchment, Ethiopia. Applied and Environmental Soil Science, 1–11. https://doi.org/10.1155/2019/2306019
- Miller, R. W., & Donahue, R. L. (1997). Soils in our environment (7th ed.). Prentice-Hall of India.
- Mohammed, S. (2003). The effect of organic matter on runoff, soil loss and crop yield at Anjeni, West Gojjam. Alemaya University.
- Murphy, H. F. (1968). A report on the fertility status and other data on some soils of Ethiopia (No. Experiment Station Bulletin No. 44). College of Agriculture, Dire Dawa, Ethiopia.
- Nahusenay, A., & Kibebew, K. (2016). Effects of land use, soil depth and topography on soil physicochemical properties along the toposequence at the Wadla Delanta Massif, Northcentral highlands of Ethiopia. Environment and Pollution, 5(2), 57–71. https://doi. 10.5539/ep.v5n2p57
- Nega, E., & Heluf, G. (2009). Influence of land use changes and soil depth on cation exchange capacity and contents of exchangeable bases in the soils of Senbete watershed, Western Ethiopia. Ethiopian Journal of Natural Resources, 11(2), 195–206.
- Olsen, S. R., Cole, C. V., Watanabe, F. S., & Dean, L. A. (1954). Estimation of available phosphorus in soils by extraction with sodium carbonate. U.S. Department of Agriculture.
- Olsen, S. R., & Sommers, L. E. (1982). Phosphorus analysis. In D. R. A. L. Miller & R. H. Keeney (Eds.), Method of soil analysis, Part 2 (pp. 403–427). Madison.
- Ozgoz, E., Gunal, H., Acir, N., Gokmen, F., Birol, M., & Budak, M. (2013). Soil quality and spatial variability assessment of land use effects in a typic Haplustoll. Land Degradation and Development, 24(3), 277–286. https://doi.org/10.1002/ldr.v24.3
- R Core Team. (2018). R: A language and environment for statistical computing. R Foundation for Statistical Computing. https://www.R-project.org/
- Smith, P. (2007). Land use change and soil organic carbon dynamics. Nutrient Cycling in Agroecosystems, 81(2), 169–178. https://doi.org/10.1007/s10705-007-9138-y
- Tadele, A., Anteneh, A., & Asmare, W. (2019). The implication of A. decurrens plantations on Soil properties in Fageta Lekoma district. Proceedings of Amhara region agricultural research institute, Bahir Dar, Ethiopia.
- Tadele, A., Hergarten, C., Hurni, H., Wolfgramm, B., Birru, Y., & Yihenew, G. S. (2013). Prediction of soil organic carbon for Ethiopian highlands using soil spectroscopy. ISRN Soil Science, 1–11. https://doi.org/10.1155/2013/720589
- Tariku, N., Haile, K., Abiyot, L., Mulugeta, S., & Habtamu, T. (2017). Variation in soil properties under different land use types managed by smallholder farmers along the toposequence in southern Ethiopia. Geoderma, 290, 40–50. https://doi.org/10.1016/j.geoderma.2016.11.021
- Tekalign, T. (1991). Soil, plant, water, fertilizer, animal manure and compost analysis (No. Working document No. 13). International Livestock Research Center for Africa, Addis Ababa, Ethiopia.
- USDA. (2004). Soil Survey Laboratory Methods Manual, Soil Survey Investigation Report No. 42 Version 4.0 (4 No. 42). United States Department of Agriculture.
- Vågen, T. G., & Winowiecki, L. A. (2013). Mapping of soil organic carbon stocks for spatially explicit assessments of climate change mitigation potential. Environmental Research Letters, 8(1), 15011. https://doi.org/10.1088/1748-9326/8/1/015011
- Wakene, N., & Heluf, G. (2003). Forms of phosphorus and status of available micronutrients under different land-use systems of Alfisols in Bako area of Ethiopia. Ethiopian Journal of Natural Resources, 5(1), 17–37.
- Walkley, A., & Black, I. A. (1934). An examination of the Degtjareff method for determining soil organic mat-ter and a proposed modification of the chromic acid titration method. Soil Science, 37(1), 29–38. https://doi.org/10.1097/00010694-193401000-00003
- White, R. E. (1997). Principles and practices of soils science: The soil is the natural resource. Cambridge University Press.
- Wondimagegn, A., Fantaw, Y., & Karltun, E. (2018). Soil organic carbon variation in relation to land use changes : The case of Birr watershed, upper Blue Nile River Basin, Ethiopia. Journal of Ecology and Environment Research, 46, 16. https://doi.org/10.1186/s41610-018-0076–1
- Worku, K., Debela, A., & Shanko, D. (2017). Characterization of the existing soil management in relation to some selected physico-chemical properties at Bulehora Woreda, West Guji Zone. International Journal of Agricultural Research, Innovation and Technology, 7(2), 43–48. https://doi.org/10.3329/ijarit.v7i2.35321
- Yihenew, G. S., & Ayanna, G. (2013). Effects of different land use systems on selected physicochemical properties of soils in Northwestern Ethiopia. Journal of Agricultural Science, 5(4), 112–120. https://dx.doi.org/10.5539/jas.v5n4p112.
- Yihenew, G. S., Fentanesh, A., & Solomon, A. (2015). The effects of land use types, management practices and slope classes on selected soil physico-chemical properties in Zikre watershed. Environmental Systems Research, 4(3), 0–6. https://doi.org/10.1186/s40068-015-0027-0
- Zelleke, G., Agegnehu, G., Abera, D., & Rashid, S. (2010). Fertilizer and soil fertility potential in Ethiopia: Constraints and opportunities for enhancing the system. International food policy research institute (IFPRI).
- Zerihun, N., Tsunekaw, A., Enyew, A., Nigussie, H., Tsubo, M., Nohmi, M., Tsegaye, D., & Aklog, D. (2016). Factors affecting small-scale farmers’ land allocation and tree density decisions in an Acacia decurrens based taungya system in Fagita Lekoma District, North-Western Ethiopia. Small-Scale Forestry, 15(3), 219–233. https://doi.org/10.1007/s11842-016-9352-z
- Zobeck, T. M., Steiner, J. L., Stott, D. E., Duke, S. E., Starks, P. J., Moriasi, D. N., & Karlen, D. L. (2015). Soil quality index comparisons using Fort Cobb, Oklahoma, watershed-scale land management data. Soil Science Society of America Journal, 79(1), 224–238. https://doi.org/10.2136/sssaj2014.06.0257