Abstract
This study tested the effect of four improved fallow legumes; Leucaena leucophala, Cajanus cajan, Albizia lebbeck and Moringa oleifera (for 1 and 2 year period) on soil nitrogen, microbial dynamics and maize grain yield in the sub-humid zone of Ghana. The study was conducted at the University for Development Studies experimental station, at latitude 9° 25ꞌ N, longitude 0° 58ꞌ W and at 183 m asl. The layout of the experiment was a randomized complete block design (RCBD) with four replications. The pattern of decomposition for years 1 and 2 was similar, however, weight loss showed a decreasing pattern with sampling time. At 48 days, Cajanus lost 27% of its initial weight compared to Leucaena and Albizia (54%) and Moringa (94%). Albizia plots had the highest total microbial load, with bacteria being the dominant soil microorganism in all treatments. Maize yield was higher in the second year and in Albizia plot (1.72 kg/ha) while the control recorded the least yield (0.89 kg/ha) in the second year. We established that biomass amended plots improved soil N, microbial biomass and maize yield compared to control plots in both years 1 and 2. Microbial biomass and N mineralised were indicators of improved soil fertility and correlated positively with maize yield in the biomass amended plots. In conclusion, we recommend Albizia biomass as a good source of manure after 2 years of establishment under sub-humid condition.
PUBLIC INTEREST STATEMENT
Majority of the people in sub-Sahara African are smallholder rural farmers who depend on farming as their main source of livelihood. Unfortunately, the soils in these regions are low in fertility and have low organic matter. Inorganic fertilizers are relatively expensive and inaccessible to these farmers. An alternative is to explore on farm organic manure source. We provide this alternative by exploring application of leafy biomass from leguminous woody perennials (e.g., legume trees) which produce high amount of biomass and nitrogen. Generally, when the leafy biomass of these woody perennials are harvested and incorporated into soil, they decompose and release the nutrients into the soil. If farmers are able to synchronize the period of the biomass decomposition and nutrient release with crops growth, the crops can benefit from the released nutrients and the soil organic matter will be improved from the biomass applied.
Competing interests
The authors declare no competing interests.
1. Introduction
Soils of Sub-humid zone are characterised by low organic matter content because the traditional fallow period is insufficient to allow for regeneration of natural vegetation (Ngetich et al., Citation2012; Omari et al., Citation2018). This has affected soil nitrogen (N) fertility, microbial biomass and crop productivity due to the continuous depletion of soil nutrients (Atakora et al., Citation2019). Consequently, these regions are often perceived as food insecure (Ngetich et al., Citation2012). As a way of improving the soil N fertility, farmers have resorted to various methods through the use of inorganic fertilizers, animal manure and plant materials in the form of mulching, green manuring and biochar (Badu et al., Citation2019). However, chemical fertilizers are not environmentally friendly with the risk of toxic build-up of chemicals which may be deadly to plants and the environment (Zhao et al., Citation2016) and besides do not add organic matter to soil. Animal manure is usually difficult to access and biochar only acts as soil amendment and is more effective when applied with inorganic fertilizers (Badu et al., Citation2019).
The importance of maize in Ghana is based on the fact that it accounts for the largest planted area (740,000 ha) of all food crops, accounting for 55% grain output (Agyare et al., Citation2014; Shamsudeen et al., Citation2018). In addition, maize is a staple food and contains crude fat (3.21–7.22%), crude protein (9.69–15.30%), crude fiber (1.62–3.45%), and 69.659–74.549% carbohydrates (Demeke, Citation2018; Tajamul et al., Citation2016).
With current maize yield less than 2 t/ha, N has been considered the most determinant of its yield (Braimoh & Vlek, Citation2006; Mehmood, Citation2017; Ramírez et al., Citation2016). For example, Atakora et al. (Citation2019) suggested that because of the aggravated poor soil fertility in Sub-humid region of Ghana, the production of maize without N fertilization should be discouraged. Unfortunately, these inorganic N-based fertilizers are too expensive and beyond the reach of smallholder farmers. In addition, within the same study area, Atakora et al. (Citation2019) attempted to promote the use of inorganic N-based fertilizers to increase maize yield. However, significant amount of N20 emission was estimated regardless of the type (ammonia sulphate, Urea and NPK-60-40-40) and amount (60 to 120 kg N/ha) of inorganic N fertilizer that was applied to maize plant.
Therefore, there is an urgent need for a more environmentally sustainable method of producing maize and that can provide the required N, improve soil organic matter and microbial activities and reclaim the degraded lands characteristic of Sub-humid and semi-arid regions.
The integration of leguminous N fixing trees and shrubs with cereals such as maize in an Agroforestry system has proven useful in reclaiming degraded lands/soils, improve soil fertility by fixing N from the atmosphere into the soil and improving bioturbation (Rao, Citation2013). This has been possible through the decomposition of the biomass of the leguminous trees and/or shrubs used as in situ source of green manure. Therefore, we selected some of the most common leguminous trees and shrub available to smallholder farmers in the Sub-humid region of Ghana. These plants, Leucaena leucophala, Cajanus cajan, Albizia lebbeck and Moringa oleifera, are easily adapted and have the ability to restore the soil fertility by fixing nitrogen into the soil within a short period (Oyebamiji et al., Citation2017; Patel et al., Citation2015). For instance, the amount of N fixed by Leucaena; Albizia and Cajanus have been established (Kumar Rao & Dart, Citation1987; Mbaya et al., Citation1998).
Equally important is the dearth of research on pattern of changes in microbial biomass in soil amended with these tropical plant materials (Li et al., Citation2018) as soil microbes help in organic matter decomposition and release of nutrients.
Therefore, the present study was aimed to assess the decomposition, N-mineralization and soil microbial dynamics of the four leguminous plants. In addition, to determine the influence of improved short fallow system with each of the four (4) leguminous species on maize grain yield.
2. Materials and methods
2.1. Experimental site
The study was conducted at the University for Development Studies experimental station, Nyankpala, Ghana. The site lies within latitude 9° 25ꞌ N and longitude 0° 58ꞌ W, with an altitude of 183 m above sea level, in the Guinea Savannah agro-ecological zone of Ghana. The study area has a unimodal rainfall pattern which starts from May and ends in October.
The peak rainfall occurs between June and September (mean annual rainfall of 1,034 mm) with the dry season usually running from November to April.
The annual temperatures range between 25̊C and 32.4̊C. The soils are classified as Haplic Lixisol, which is a mixture of ferruginized ironstone brash and iron gravel stone (FAO/UNESCO, Citation1997).
3. Experimental design
The layout of the experiment was split plot with sub-plot as randomized completely block design (RCBD) with four (4) replications. The main plot was the fallow period and the sub-plot was the four leguminous species (Albizia lebbeck, Leucaena leucocephala, Cajanus cajan, and Moringa oleifera). Each sub-plot measured 5 × 5 m. Seeds of the legume plants, obtained from the Forest Research Institute of Ghana, were sown on each plot, by randomly allocating each treatment on the plots. At the end of each fallow period, the plot was cleared, soil samples taken for laboratory analysis. Leafy biomass from each plot was incorporated 2 weeks before planting the test crop. In the second week of June, test crop, a local improved maize (Zea mays, L var. Okomasa) was planted at a spacing of 80 × 50 cm and thinned to 2 plants per stand resulting in a plant population of 50,000 plants/ha. Sub-samples of the leguminous plant species were taken and used for conducting decomposition experiments to determine their decomposition rate, nitrogen mineralization, microbial biomass and microbial diversity. The above procedure was repeated at the end of the second-year improved fallow period.
4. Decomposition and nitrogen mineralization experiment
Decomposition studies were carried out for a period of 6 weeks, using the sampled biomass (chopped) of Leucaena leucocephala, Albizia lebbeck, Cajanus cajan and Moringa oleifera. The Litterbag (1 mm mesh) technique was used according to Kaba et al. (Citation2018). A total of 64 litterbags (4 species × 4 sampling periods × 4 replicates) were prepared each containing 20 g equivalent dry weight (D.W) of the leafy biomass of each species. The litterbags were randomly buried on the soil surface (0.5 cm depth).
At 7, 14, 28 and 42 days after burring, each of the treatments were retrieved, cleaned of all soil particles, dried to a constant weight at 60°C for 72 hours and weighed as their biomass (weight) remaining after decomposition.
Decomposition constants were calculated for the four species using the formula, Mt = Moe-kt where Mo is the initial mass, Mt is the mass of the litter at a certain time t after initial measurement, e is the natural logarithm, and K is the decomposition constant.
For the Nitrogen mineralization, the plastic bucket technique (Sierra, Citation2002) was used. The equivalents of 20 g D.W leafy biomass of each species were incorporated into a plastic bucket containing 6 kg of soil. The plastic buckets were arranged randomly in the field and stirred for uniform mixture. Watering and stirring was done to ensure uniform mixture. In each of the treatments, 5 g of the biomass were taken to Savannah Agricultural Research Institute (SARI) laboratory for determination of mineral N content, P, K, C and pH levels of each of the treatments upon retrieval. Nitrogen (N) was determined using the Micro-Kjeldal method, Phosphorus (P) by the Olsen method and Potassium (K) was determined in the Ammonium Acetate leachate by flame analysis using a Flame Photometer, while Carbon was determined by the Walkley-black method.
5. Microbial biomass estimation
Microbial biomass was determined at 12 and 24 months. From the four plots of each of the treatments, soil sample representative of the different slope positions in each plot was randomly collected using soil auger to a depth of 0–20 cm and bulked into a composite soil sample for each treatment. The composite soil samples were oven-dried and sieved through a 2 mm-strainer (screen). Samples were placed in an ice chest containing ice blocks and transported to the microbiology laboratory.
6. Sample preparation
Ten grams of each soil sample was weighed into each sampling bottle containing 90 ml of peptone water making a dilution of 10⁻1. This was then agitated vigorously for 2 minutes to obtain homogeneity. One milliliter (1 ml) of the sample inoculum was pipetted from the 10⁻1 dilution into a separate tube containing 9 ml of peptone water to make a dilution of 10⁻2. The liquids were carefully mixed by aspirating 10 times with a sterile pipette and again 1.0 ml transferred into another dilution tube containing 9 ml of dilution peptone water and mixed. This would now contain 10⁻3. These dilutions were then ready for inoculation.
7. Sample analysis
Sterile plates of plate count agar were labeled 10⁻1, 10⁻2 and 10⁻3 and 0.1 ml (100 µl) of the various dilutions inoculated accordingly. The inoculum was promptly spread on the surface of the plates using sterile glass spreader (Drigalsky spatulas).
The plates were allowed to dry for 15 minutes before incubating in an inverted position for at 35–37ºC for 48 hours. Colonies were then counted and enumerated. All colonies in three plates corresponding to one dilution and showing between 30 and 300 colonies were counted.
Averages of the replicates were calculated and multiplied by their dilution factor. This was then reported as the colony-forming unit per gram. Overcrowded colonies were not counted and were reported as “Too Numerous to Count” (TNTC).
8. Isolation and identification of fungi
For enumeration and isolation of yeast molds, same serial dilutions were prepared and inoculated on Dichloran Rose-Begal Chloramphenicol agar (DRBC). Plates were incubated in a covered box at room temperature for 5 days and inspected after every 2 days.
Different characteristic features of the isolated fungi were observed and used in their identification according to Richardson and Simpson (Citation2011).
9. Identification of bacteria
9.1. Preparation of smears
A drop of physiological saline was placed on a clean grease-free glass slide. A sterile inoculating loop was used to transfer a colony to the slide and emulsified to make a smear. The slides were left to air-dry before heat fixing using the flame of a Benson burner to properly adhere the smear on the slides as well as preserve microorganisms and prevent smears from being washed from the slides. Smears were allowed to cool before staining. The smears were examined microscopically with oil immersion objective to report the gram status and morphology of bacteria.
10. Biochemical test
Catalase test and coagulate test were used to confirm Staphylococcus aureus, indole test was used to confirm Eschrichia coli and oxidase test was used to confirm Pseduomounas auruginosa. Haemolysis on blood agar was used to confirm Streptococci species.
11. Data analysis
Before analyses, the entire data were subjected to normality test to satisfy conditions for ANOVA and other parametric analyses. Analysis of variance (ANOVA) was performed using GenStat Ninth Edition to find out the differences in mineralization, microbial load and maize yield among the four species. Where F-test was significant at P < 0.05, Tukey’s Honestly Significant Difference (HSD) test (p < 0.05) was used to separate the means. Where shown, data are averages ± standard deviation (s.d.) of the four replicates. The decomposition and mineralization rates at the various data points were used to run a regression analysis to find the relationship between decomposition and mineralization of nutrients among the various treatments per day.
12. Result
12.1. Initial soil and microbial analysis
The soil was moderately acidic with a pH value of 5.20, with very low soil total nitrogen, organic carbon and available phosphorus (Bray-1 P). However, it was relatively high in exchangeable potassium and the microbial (fungi and bacteria) count showed a total of 422 microbial loads (Table ).
Table 1. Initial properties of soil at the farming for the future experimental site, UDS Nyankapala campus within the Guinea-Savannah ecological zone
12.2. Biomass Decomposition and nitrogen mineralization
The initial C: N ratio of the biomass used for the decomposition in years 1 and 2 were similar for all the plant species (average of 11). Similarly, rate of decomposition in both years 1 and 2 years were not significantly different. However, among the plant species, there were significant (p < 0.05) differences in their rate of decomposition as weight lost showed a decreasing pattern with sampling time (Figure ).
Figure 1. Amount of initial biomass remaining in the litter bags during the decomposition experiment (Bars indicate standard errors; means are averages of years 1 and 2).
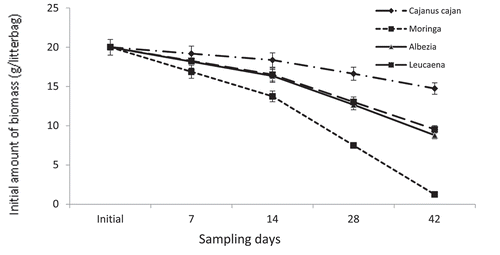
Except for Leucaena and Albizia biomass which had similar pattern of decomposition at every sampling stage, Cajanus and Moringa were significantly (p < 0.05) different, with Moringa having the highest weight loss at every sampling stage while Cajanus took the longest time to lose its initial weight. For example, at 28 days, Cajanus had lost 3.4 g (17%) of the initial weight compared to 35%, 36% and 63% in Leucaena, Albizia and Moringa, respectively (Figure ).
On the last day of recuperation (48 days), Leucaena and Albizia lost similar amount of their initial weight (average 54%) but Cajanus had lost only 27% while and Moringa lost 94% of the initial weight (Figure ).
Biomass decomposition and N released were significantly (p < 0.05) and positively related in both years for all the four plant species. The coefficient of determination (R2) range between 74% and 87% for year 1 and 64% and 86% for year 2 (Figures –5).
The amount of biomass required to decompose and release the required 75 kg N/ha for the maize plant varied among the plant species and in each year. The amount of biomass decreased in the second year for all the plant species.
For instance, the amount of Albizia, Cajanus, Leucaena and Moringa biomass required (to produce 75 kg N/ha) in year 1 was 1,233.3 kg, 428 kg, 1297 kg and 2760 kg D.W per hectare, respectively (Figures –5). However, these quantities reduced to 1162 kg, 328 kg,1252 kg and 2625 kg, respectively, in the second year 2 (Figures –5).
12.3. Microbial load and diversity after biomass application
Different types of microbes were identified before biomass incorporation, at year 1 and at year 2 after biomass application. The E. Coli, Staplylococcus aureus, Aspergillus niger and Paecilonyes spp were common in all the three stages, while Penicilium spp was only found at the initial stage (Table ).
Table 2. Types of microorganisms present before biomass application (Initial stage) at the farming for the future site, University for Development Studies, Guinea Savannah zone of Ghana
The plant species contributed significantly to soil microbial loads (bacteria and fungi) after they were applied to the maize plant. The highest total microbial load was recorded for Albizia and this was significantly (p < 0.05) higher than Cajanus but not Moringa and Leucaena (Table ). The microbial community structure was significantly (p < 0.05) influenced by the different plant biomass and the year of incorporation, with year 2 producing more microbes than year 1 (Table ).
Table 3. Effect of different plant biomass at different ages of plant on soil microbial organisms in the Guinea Savannah zone of Ghana
Cajanus biomass had the highest fungi abundance and was significantly (p < 0.05) higher than Moringa and Leucaena but similar to that of Albizia (Table ). Pseudomonas aeruginosa was however found at the initial sampling stage and year 2 but not in year 1.
Towards year 2, new microbial species were identified (Table ), for example, Streptococcus spp, Rhizopus, Trichoderma and Yeast were identified in year 2 plot but not in year one or the initial stage (Tables and ).
Table 4. Types of microorganisms present in year 1 after biomass application, at the farming for the future site, University for Development Studies in the Guinea Savannah zone of Ghana
Table 5. Types of microorganisms present in year 2 after biomass application at the farming for the future site, University for Development Studies, Guinea Savannah zone of Ghana
12.4. Yield of maize as influenced by the different biomass application
Maize grain yield between Years 1 and 2 was significantly different, except the control which had similar yield (Year 1 = 0.89 t/ha and Year 2 = 0.91). Generally, maize yield in year 2 was significantly (P < 0.05) higher than yield in year 1 (Table ) for all the biomass amended plots.
Table 6. Grain yield of maize in both first and second years. at the farming for the future, University for Development Studies in the Savannah zone of Ghana
After 12 months of leguminous trees and shrub growth, maize grain yield was highest in Albizia plot (1.72 kg/ha) while the control plot recorded the least maize grain yield (0.89 kg/ha). However, maize grain yield from Leucaena and Moringa were similar, but were significantly higher than the control plot (Table ).
In year 2, Albizia and Leucaena had the highest maize grain yield (average of 2.37 kg/ha) followed by Moringa and Cajanus (average of 1.9 kg/ha) (Table ).
13. Discussion
The present study tested the hypothesis that improved short fallow system (1 or 2 years) of leguminous plant species will improve soil N, microbial biomass and yield of maize compared to natural fallow system in the sub-humid zone of Ghana. Some soil properties before the establishment of the improved fallow with leguminous plants showed low N (0.002%), organic carbon (0.420%) and microbial count (Table ). This was not too surprising as earlier research by Fosu et al. (Citation2007) confirmed this to be symptomatic of soils in sub-humid and semi-arid zones of Ghana.
At the end of year 1 fallow period, amount of biomass produced by Albizia (9.4 t/ha), Leucaena (5.9 t/ha), Moringa (2 t/ha) and Cajanus (6 t/ha) increased over 60% at the end of year 2 fallow period. This was significantly higher than what has been reported for the same species and other legume species in Ghana and elsewhere (Kaba & Abunyewa, Citation2019; Marroquín et al., Citation2005). The trend of biomass decomposition was similar in both year 1 and 2, however, among the species, they varied in their decomposition pattern and N mineralization despite their low (average of 10) C:N ratio (Figures –5). This pattern is inconsistent with the general view that total N and low C:N ratio (<25) exhibit consistent influence on biomass decomposition and N mineralization (Kaba et al., Citation2018).
However, we can attribute the differences in the decomposition to the initial N:P ratio of the biomass, which is believed to be optimum for decomposer microorganisms when it is in the range of 10–15 (Swift et al., Citation1979).
For instance, the N:P ratio for Moringa was the lowest (9.5) followed by Leucaena and Albizia (average of 11) while Cajanus had the highest of 16.5. It was therefore not surprising that on the last day of recuperation (48 days), Leucaena and Albizia lost similar amount of their initial weight (average 54%) but Cajanus had lost only 27% while and Moringa lost 94% of the initial weight (Figure ).
In agreement with Jacob et al. (Citation2010) and Prescott (Citation2005) a positive correlation between N concentrations and litter decomposition, as seen in Cajanus species (Figure ), do not necessarily imply that litter quality controls litter decay. The regression analysis between biomass decomposition and N mineralization to establish the relationship between quantities of biomass decomposed and the expected quantity of N released into the soil for maize uptake showed that it will be better for farmers to consider 2 year improved fallow system with any of the leguminous plant as less amount of biomass will be required as organic manure to supply the 75 kgN needs of the maize.
For instance, from the equations in Figures –5, the amount of Albizia, Cajanus, Leucaena and Moringa leafy biomass required (to produce 75 kg N/ha) in year 1 was 1233.3 kg, 428 kg, 1297 kg and 2760 kg/ha D.W, respectively. However, these quantities reduced to 1162 kg, 328 kg, 1252 kg and 2625 kg/ha, respectively, in the second year 2.
An analysis of the soil also showed that the microbial biomass changed over time, with more number of soil microbes being (Table ). There have been similar reports of a substantial increase in microbial biomass following organic matter applications (Sall et al., Citation2006). For example, Sall et al. (Citation2006) measured up to 400% increase in soil microbes in soils amended with biomass from the leguminous plant, Faidherbia albida compared to unamended soils. This contradicts earlier suggestions (Puri & Ashman, Citation1998) that microbial biomass are usually constant throughout the year in spite of changing soil conditions and nutrient availability. This certainly influenced the rate of decomposition and N mineralization among the plant species in both years (Table ). For example, Cajanus had the lowest rate of decomposition and N release, interestingly; it also recorded the lowest number of microbial biomass in both years, hence stressing the importance of micro organisms in nutrient release from decomposing organic matter.
This observation however disagrees with reports by Marvin et al. (Citation2011) that N mineralization is predominantly controlled by biochemical processes that are independent of microbial activity; hence, microbial biomass may not be ideal indicators of soil N supply. However, in line with Halmi et al. (Citation2018) our findings revealed that microbial biomass is an indicator of soil fertility and had a strong positive link with N-mineralization after the application of the plant biomass in both year 1 and 2.
In addition, in agreement with Lazcano et al. (Citation2013) and Mbuthia et al. (Citation2015) but inconsistent with Ngosong et al. (Citation2010) the plants' biomass amended soils significantly increased bacteria (96%) microbes than fungi in both year 1 and 2 (Table ). Li et al. (Citation2018) also reported that the microbial community composition at the experimental site after organic matter application was dominated by bacteria (60%). The differences in microbial community structure influenced the decomposition and N mineralization. Among the amended plots, Cajanus had the lowest number of bacteria, and in turn, took the longest time to decompose its initial biomass and release N.
In stressing the importance of bacteria during decomposition, Lazcano et al. (Citation2013) and Lipson et al. (Citation2009) described that bacteria had higher growth rates, were the most sensitive microbial groups, had a much shorter turnover time than fungi and can react faster to the environmental changes in soil. Our observation should not be too surprising as Mbuthia et al. (Citation2015) have indicated that lower fungal biomass relative to bacteria is typical in cultivated soils and it has been attributed to different factors such as intensive physical disturbance, and altered amount of the nutrient inputs as compared to undisturbed soils.
In general, maize yield was higher in amended plots compared to the control; likewise, higher yield was recorded in year 2 than in year 1 (Table ). This observation reiterates the important role that biomass from leguminous plants play in releasing N to improve soil organic matter quality and increase crop yield (Li et al., Citation2018; Oyebamiji et al., Citation2017). Similar to the findings of Haider et al. (Citation1991) there was a strong relationship between microbial biomass, N mineralization and maize grain yield in both cropping years (Table ). Among the plant species, however, grain yield was highest in Albizia amended plots in both year 1 and 2 (Table ). This confirms an earlier study in Nigeria by Oyebamiji et al. (Citation2017) who showed that Albizia decomposed and mineralized N faster for maize uptake and improve yield under Semi-humid/arid environment.
The present study envisages that when leguminous trees and shrubs are used in improved fallow systems with maize in an Agroforestry system, and their biomass used as source of green manure, they could play alternative or supplementary role to improve soil microbial activities and release N essential to sustain soil fertility for improving maize yield under sub-humid and semi-arid conditions, this benefit could be much higher if biomass application is done after 1 year of establishment.
14. Conclusion
The present study contributes to efforts being made to develop farming system that is sustainable under sub-humid and semi-arid conditions. We established that the leguminous plant biomass improved the soil N, increased microbial biomass and maize yield compared to the control plots in both years 1 and 2. In addition, these parameters (N, Microbes and yield) improved significantly when the land was used for maize cultivation after 2 years of planting the leguminous trees and shrub.
Among the plant species, Albizia biomass was the most efficient in decomposing, releasing N, improving microbial biomass and increasing maize yield. This was followed by Leucaena and Moringa biomass, while Cajanus biomass came last in the sub-humid dry land farming conditions. However, Cajanus provided grain that serves as food making it attractive to the farmer as an option for short fallow improved system under sub-humid condition.
Conflict of interest
The Authors declare that they have no conflict of interest.
Summary of group key research activities
The Department of Agroforestry conducts research that involve integration of trees/shrubs into traditional agriculture cropping systems. The idea is to assess how these woody perennials can contribute to improve the soil fertility, improve microclimate and yield of the associated annual crops. In doing this, we utilize multipurpose trees, especially legume species. We envisage that when the right woody perennials are selected and integrated with crops, they could help reclaim degraded lands/soils, improve soil fertility, reduce greenhouse gas emission and help farmers reduce the application of inorganic fertilizers, protect the environment, reduce cost of production and improve profitability of farming. The group also has research interest in climate change impact on farmers and their adaptation and mitigation measures.
Additional information
Funding
References
- Agyare, W. A., Asare, I. K., Sogbedji, J., & Clottey, V. A. (2014). Challenges to maize fertilization in the forest and transition zones of Ghana. African Journal of Agricultural Research, 9(6), 593–14. https://doi.org/10.5897/AJAR12.1698
- Atakora, W. K., Kwakye, P. K., Weymann, D., & Brüggemann, N. (2019). Stimulus of nitrogen fertilizers and soil characteristics on maize yield and nitrous oxide emission from ferric luvisol in the Guinea Savanna agro-ecological zone of Ghana. Scientific African, 6, e00141. https://doi.org/10.1016/j.sciaf.2019.e00141
- Badu, E., Kaba, J. S., Abunyewa, A. A., Dawoe, E. K., Agbenyega, O., & Barnes, V. R. (2019). Biochar and inorganic nitrogen fertilizer effects on maize (Zeamays L.) nitrogen use and yield in moist semi-deciduous forest zone of Ghana. Journal of Plant Nutrition, 42(19), 2407–2422. https://doi.org/10.1080/01904167.2019.1659347
- Braimoh, A. K., & Vlek, P. L. G. (2006). Soil quality and other factors influencing maize yield in northern Ghana. Soil Use and Management, 22(2), 165–171. https://doi.org/10.1111/j.1475-2743.2006.00032.x
- Demeke, K. H. (2018). Nutritional quality evaluation of seven maize varieties grown in Ethiopia. Biochemistry and Molecular Biology, 3(2), 45–48. https://doi.org/10.11648/j.bmb.20180302.11
- FAO/UNESCO. (1997). Soil map of the world, revised legend (World Soils Resource Report No. 60). FAO. 41 pp.
- Fosu, M., Kühne, R. F., & Vlek, P. L. G. (2007). Mineralization and microbial biomass dynamics during decomposition of four leguminous residues. Journal of Biological Sciences, 7(4), 632–637. https://doi.org/10.3923/jbs.2007.632.637
- Haider, J., Marumoto, T., & Azad, A. K. (1991). Estimation of microbial biomass carbon and nitrogen in Bangladesh soils. Soil Science and Plant Nutrition, 37(4), 591–599. https://doi.org/10.1080/00380768.1991.10416927
- Halmi, A., Farid, M., Hasenan, S. N., Simarani, K., & Abdullah, R. (2018). Linking soil microbial properties with plant performance in acidic tropical soil amended with biochar. Agronomy, 8(11), 255. https://doi.org/10.3390/agronomy8110255
- Jacob, M., Karin, V., Andrea, P., & Frank, M. T. (2010). Leaf litter decomposition in temperate deciduous forest stands with a decreasing fraction of beech (Fagus sylvatica). Oecologia, 164(4), 1083–1094. https://doi.org/10.1007/s00442-010-1699-9
- Kaba, J. S., & Abunyewa, A. A. (2019). New aboveground biomass and nitrogen yield in different ages of gliricidia (Gliricidia sepium Jacq.) trees under different pruning intensities in moist semi-deciduous forest zone of Ghana. Agroforestry Systems. https://doi.org/10.1007/s10457-019-00414-3(0123456789)
- Kaba, J. S., Zerbe, S., Zanotelli, D., Abunyewa, A. A., & Tagliavini, M. (2018). Uptake of nitrogen by cocoa (Theobroma cocoa L.) trees derived from soil decomposition of gliricidia (Gliricidia sepium Jacq.) shoots. Acta Horticulturae, 1217(1217), 263–270. https://doi.org/10.17660/ActaHortic.2018.1217.33
- Kumar Rao, J. V. D. K., & Dart, P. J. (1987). Nodulation, nitrogen fixation and nitrogen uptake in pigeon pea (Cajanus cajan (L.) Millsp) of different maturity groups. Plant and Soil, 99(2–3), 255–266. https://doi.org/10.1007/BF02370872
- Lazcano, C., GoÂmez-BrandoÂn, M., Revilla, P., & DomõÂnguez, J. (2013). Short-term effects of organic and inorganic fertilizers on soil microbial community structure and function. Biology and Fertility of Soils, 49(6), 723–733. https://doi.org/10.1007/s00374-012-0761-7
- Li, J., Wu, X., Gebremikael, M. T., Wu, H., Cai, D., Wang, B., Li, B., Zhang, J., Li, Y., & Xi, J. (2018). Response of soil organic carbon fractions, microbial community composition and carbon mineralization to high-input fertilizer practices under an intensive agricultural system. PloS One, 13(4), e0195144. https://doi.org/10.1371/journal.pone.0195144
- Lipson, D. A., Monson, R. K., Schmidt, S. K., & Weintraub, M. N. (2009). The trade-off between growth rate and yield in microbial communities and the consequences for under-snow soil respiration in a high elevation coniferous forest. Biogeochemistry, 95(1), 23–35. https://doi.org/10.1007/s10533-008-9252-1
- Marroquín, I. M., Vargas, J. H., Velázquez, A. M., & Etchevers, J. B. (2005). Aboveground biomass production and nitrogen content in Gliricidia sepium (Jacq.) Walp. Under several pruning regimes. Interciencia, 30(3), 151–158. http://www.redalyc.org/articulo.oa?id=33910206
- Marvin, S. L., Whalen, J. K., Ziadi, N., & Zebarth, B. J. (2011). Nitrogen dynamics and indices to predict soil nitrogen supply in humid temperate soils. Advances in Agronomy, 112, 55–102. Academic press. https://doi.org/10.1016/B978-0-12–385538-1.00002-0
- Mbaya, N., Mwange, K. N., & Luyindula, N. (1998). Nitrogen fixation in Acacia auriculiformis and Albizia lebbeck and their contributions to crop-productivity improvement (No. IAEA-TECDOC–1053).
- Mbuthia, L. W., Acosta-Martínez, V., DeBruyn, J., Schaeffer, S., Tyler, D., Odoi, E., & Eash, N. (2015). Long term tillage, cover crop, and fertilization effects on microbial community structure, activity: Implications for soil quality. Soil Biology & Biochemistry, 89, 24–34. https://doi.org/10.1016/j.soilbio.2015.06.016
- Mehmood, A. N. (2017). Nitrogen management and regulation for optimum NUE in maize – A mini review. Cogent Food & Agriculture, 3(1), 1348214. https://doi.org/10.1080/23311932.2017.1348214
- Ngetich, F. K., Shisanya, C. A., Mugwe, J., Mucheru-Muna, M., & Mugendi, D. (2012). The potential of organic and inorganic nutrient sources in sub-Saharan African crop farming systems. In Soil fertility improvement and integrated nutrient management – A global perspective. InTech.
- Ngosong, C., Jarosch, M., Raupp, J., Neumann, E., & Ruess, L. (2010). The impact of farming practice on soil microorganisms and arbuscular mycorrhizal fungi: Crop type versus long-term mineral and organic fertilization. Applied Soil Ecology, 46(1), 134–142. https://doi.org/10.1016/j.apsoil.2010.07.004
- Omari, R. A., Bellingrath-Kimura, D. S., Fujii, Y., Sarkodee-Addo, E., Appiah Sarpong, K., & Oikawa, Y. (2018). Nitrogen mineralization and microbial biomass dynamics in different tropical soils amended with contrasting organic resources. Soil Systems, 2(4), 63. https://doi.org/10.3390/soilsystems2040063
- Oyebamiji, N. A., Babalola, A. O., & Aduradola, A. M. (2017). Decomposition and nitrogen release patterns of Parkiabiglobosa and Albizialebbeck Leaves with nitrogen fertilizer for maize production in Sudan Savanna Alfisol of Nigeria. Journal of Tropical Forestry and Environment, 7(1), 1. https://doi.org/10.31357/jtfe.v7i1.3022
- Patel, D. P., Das, A., Kumar, M., Munda, G. C., Ngachan, S. V., Ramkrushna, G. I., & Somireddy, U. (2015). Continuous application of organic amendments enhances soil health, produce quality and system productivity of vegetable-based cropping systems in subtropical Eastern Himalayas. Experimental Agriculture, 51(1), 85–106. https://doi.org/10.1017/S0014479714000167
- Prescott, C. E. (2005). Do rates of litter decomposition tell us anything we really need to know? Forest Ecology and Management, 220(1–3), 66–74. https://doi.org/10.1016/j.foreco.2005.08.005
- Puri, G., & Ashman, M. R. (1998). Relationship between soil microbial biomass and gross N mineralisation. Soil Biology & Biochemistry, 30(2), 251–256. https://doi.org/10.1016/S0038-0717(97)00117-X
- Ramírez, M. V., Rubilar, R. A., Montes, C., Stape, J. L., Fox, T. R., & Lee Allen, H. (2016). Nitrogen availability and mineralization in Pinus radiatastands fertilized mid-rotation at three contrasting sites. Journal of Soil Science and Plant Nutrition, 16(1), 118–136. https://doi.org/10.4067/S0718-95162016005000009
- Rao, D. L. N. (2013). Soil biological health and its management. In Soil health management: Productivity-sustainability-resource management (pp. 55–83). FDCO, New Delhi.
- Richardson, A. E., & Simpson, R. J. (2011). Soil microorganisms mediating phosphorus availability update on microbial phosphorus. Plant Physiology, 156(3), 989–996. https://doi.org/10.1104/pp.111.175448
- Sall, S. N., Masse, D., Ndour, N. Y. B., & Chotte, J. L. (2006). Does cropping modify the decomposition function and the diversity of the soil microbial community of tropical fallow soil? Applied Soil Ecology, 31(3), 211–219. https://doi.org/10.1016/j.apsoil.2005.05.007
- Shamsudeen, A., Nkegbe, P. K., & Donkoh, S. A. (2018). Assessing the technical efficiency of maize production in northern Ghana: The data envelopment analysis approach. Cogent Food & Agriculture, 4(1512390), 1. https://doi.org/10.1080/23311932.2018.1512390
- Sierra, J. (2002). Nitrogen mineralization and nitrification in a tropical soil: Effects of fluctuating temperature conditions. Soil Biology & Biochemistry, 34(9), 1219–1226. https://doi.org/10.1016/S0038-0717(02)00058-5
- Swift, M. J., Heal, O. W., Anderson, J. M., & Anderson, J. M. (1979). Decomposition in terrestrial ecosystems (Vol. 5). Univ of California Press.
- Tajamul, R. S., Prasad, K., & Kumar, P. (2016). Maize – A potential source of human nutrition and health: A review. Cogent Food & Agriculture, 2(1166995), 1. https://doi.org/10.1080/23311932.2016.1166995
- Zhao, J., Ni, T., Li, J., Lu, Q., Fang, Z., Huang, Q., Zhang, R., Li, R., Shen, B., & Shen, Q. (2016). Effects of organic–inorganic compound fertilizer with reduced chemical fertilizer application on crop yields, soil biological activity and bacterial community structure in a rice–wheat cropping system. Applied Soil Ecology, 99, 1–12. https://doi.org/10.1016/j.apsoil.2015.11.006