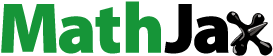
Abstract
This study was conducted to analyze the combining ability for yield and yield contributing traits of selected rice genotypes. Eighteen F2 populations developed by half-diallel mating design and seven parental lines were planted in randomized complete block design in three replications. Analysis of variance indicated that genotypes, general combining ability and specific combining ability mean square values were highly significant (p ≤ 0.001) for all measured traits which indicated the genetic diversity of the parents and the importance of both additive and nonadditive gene effects in the inheritance of the measured traits respectively. Parent P2 (WAC 18-WAT 15-3-1) followed by parent P1(WAB 1573-22-B-B-FKR 4-2-WAC 1-TGR 3-WAT9-1) were the best general combiners for days to flowering, number of effective tillers per hill, panicle length, flag leaf length and width, plant height, and grain yield per plant. Hybrid combinations with good mean performance, desirable SCA estimates and involving at least one of the parents with high GCA would be considered for favorable allele combinations. As a result cross combinations involving parent with good GCA (P2), WAC 18-WAT 15-3-1 x SR33701-HB3330-78 (2.54) had the high desirable grain yield followed by crosses, SR33701-HB3330-78 x SR33859-HB3324-93 (2.34), SR33701-HB3330-78 x SR33859-HB3324-133(2.43), and SR33859-HB3324-93 x SR33859-HB3324-133(1.48) which gave high SCA value in the required direction for grain yield. In general, the crosses showing significant and desirable combining ability effects were associated with better per se performance of their respective parents.
Kywords:
PUBLIC INTEREST STATEMENT
Rice production in Uganda started in 1942, mainly to feed the World War II soldiers. Most of rice production in Uganda is in the Eastern parts of the country, followed by the Western part of Uganda. This is due to the presence of lowland with high moisture content throughout the growing season. Lack of irrigation facilities, rice blast and rice yellow mottle virus are also a big constraint for rice production. As a result, farmers need variety which is resistant to these diseases, mature earlier and have good quality. To alleviate these bottle necks, the rice researchers doing on variety improvement through selection and hybridization with elite materials. These could be either using local elite materials or by introducing materials from different sources with the targeting the demand of different stakeholders. After subsequent evaluation, the materials would be evaluated by the growers, other stakeholders and also the variety releasing committee. If it fulfills the requirements, it will be released as variety for production.
1. Introduction
Rice (Oryza sativa L.) is most commonly produced in Asia, Africa, and Australia (Dogara & Jumare, Citation2014). It is the third most cultivated cereal crop in the world, after wheat and maize (FAO, Citation2014) and most common staple food for more than half of the world’s population. It provides up to 50% of the dietary caloric supply and a substantial part of the protein intake in Asia (Muthayya et al., Citation2014).
In Sub-Saharan Africa (SSA), rice consumption among urban dwellers has steadily grown, with a per capital consumption that has doubled since 1970 (Muthayya et al., Citation2014). From 2002 to 2007, rice production in Africa had increased by an average of 3.2% per year, and from 2007 to 2012 by 8.4% per year (CGIAR, Citation2013). Uganda’s production of rice increased by 5% over five years, from 214 thousand tones on 93 thousand hectares in 2010 to 237 thousand tones on 95 thousand hectares in 2014 (FAO, Citation2014). Dewina and Reno (Citation2010) reported that rice represented 0.42% of the total production of food crops in Uganda, ranking fifth after maize, cassava, bananas, and beans even though the production and productivity is limited by many factors.
The low production and productivity of the crop is due to many biotic and abiotic factors. So, to overcome the low productivity variety improvement and genetic study of the materials is the powerful mechanism. In line with these, combining ability analysis helps to identify the parents that have good combining ability of traits in the desirable direction for different targeted traits.
Identification of parents with good traits and studying its inheritance of the targeted traits to the progeny in different cross combination is vital to characterize the nature and magnitude of gene effects in the expression of different traits. Combining ability analysis gives an idea about the relative importance and magnitude of additive and nonadditive types of gene action in the expression of the traits (Griffing, Citation1956). As a result, this study was conducted to identify the best combining parents on the basis of their general and specific combining ability for yield and yield contributing traits quality improvement in in selected rice varieties.
2. Materials and methods
2.1. Experimental material
The experiment was conducted at National Crop Resources Research Institute (NaCRRI) Namulonge in Uganda on seven rice varieties for population development. The genotypes are from two sources, four genotypes are from AfricaRice and three genotypes were from KAFACI (Korea-Africa Food and Agriculture Cooperation Initiative) as described in Table.
Table 1. Origin and pedigree of selected genotypes used for population development
The parental genotypes were planted in buckets and staggered four times at one-week intervals to synchronize flowering dates of the varieties. A half-diallel mating design was used to generate F1 populations and later advanced to F2 level so as to increase the amount of seeds. The seven parental genotypes with the respective18 crosses were evaluated at NaCRRI in the screen house in a 4 × 7 alpha lattice design in three replications. A spacing of 20 cm between rows and between blocks and 20 cm between plants were used. All agronomic practices including weeding and fertilizer applications were performed as the local recommendations.
2.2. Data collection and analysis
Data for days of 75% flowering, number of effective tillers per hill, panicle length, flag leaf length, flag leaf width, plant height were collected accordingly on five randomly selected plants for each plot, while grain yield was recorded by separately harvesting the three middle rows. The data were then analyzed using GenStat (12th edition, Payne et al., Citation2009) alpha lattice restricted maximum likelihood (ReML) algorithm. The genotypes were considered a fixed effect while blocks and replications were random effects. In order to select a good combination of parents, coefficient of genetic determination both in broad and in narrow sense, general combining ability and specific combining ability, additive, and dominance variance were calculated using Griffing (Citation1956) method II model I. The statistical model used was as follows:
is the grand mean,
and
are GCA effects of the ith and jth parents respectively,
is the SCA effect for the crosses between the ith and jth parents, Rk is replication means effects, and
is experimental error.
The GCA and SCA variance components were calculated by using σ2gca and σ2sca coefficients and also weighted mean of the respective parents were used to estimate the mean of the missing crosses. A two tailed t-test were used to determine the significance of the GCA and SCA effects. Baker’s ratio was also computed to estimate the parental performance associated with variance of GCA and SCA effects using the following formula; Baker’s ratio = 2σ2GCA/(2 σ2GCA + σ2SCA). In addition to this, broad sense coefficient of genetic determination (BSCGD), and narrow sense coefficient of genetic determination (NSCGD) estimates for each measured traits were computed as follows: BSCGD = CGD–BS = (2σ2GCA + σ2SCA)/(2σ2GCA + σ2SCA + σ2error/r) and NSCGD = 2σ2GCA/(2σ2GCA + σ2SCA + σ2error/r) (Abney et al., Citation2000; Jacquard, Citation1983).
3. Result and discussion
3.1. Analysis of variances
The analysis of variance for yield and yield components presented in Table which revealed that a highly significant differences (p ≤ 0.01) among the tested genotypes for all measured traits and also for GCA and SCA. The significant general and specific combining ability (Table ) indicated that the importance of both additive and nonadditive gene actions in the expression of these characters. The relative magnitude of GCA is higher for all traits except grain yield which indicated the predominant effect of additive gene actions for the control of days to maturity, number of effective tillers per hill, panicle length, flag leaf length, flag leaf width, and plant height. It was further supported by ratio (σ2GCA/σ2SCA) being higher than one and degree of additive gene action.
Table 2. Analysis variance and mean square estimates for agronomic traits of rice
Chakraborty et al. (Citation2009) was also reported similar results that the additive gene actions plays a higher portion for the control of grains per panicle, plant height, days to flowering, flag leaf length, panicle length, number of effective tillers per hill and grain yield. Gnanamalar and Vivekanandan (Citation2013), was also reported similar study that predominance of additive gene actions for the control of different agronomic traits in rice. On the other hand, Zeinab et al. (Citation2014) reported the higher significant contribution of SCA variance effects for the control of number of tillers per hill, panicle length and 100-grain weight which showed preponderance of nonadditive gene action in the inheritance of the traits. The predominance of non-additive gene action was observed for grain yield which was illustrated by the variance of SCA was higher than variance of GCA. This result corresponds with the findings of different scholars which stated the predominance of non-additive gene action for grain yield, Saidaiah et al. (Citation2010), Hasan et al. (Citation2013), and Bano and Singh (Citation2019).
A High coefficient of genetic determination in the broad sense (0.9), (0.54), (0.87), (0.85), (0.71), and (0.97) and also high Baker’s ratio of 0.6, 0.5, 0.5, 0.6, 0.6, and 0.5 for days to flowering, number fertile tillers per hill, panicle length, flag leaf length, flag leaf width, and plant height respectively. This indicating primarily additive inheritance among crosses for the measured traits. The high Baker’s ratio also implies that selection in early generations can be effective. Therefore, methods such as pedigree selection, modified pedigree, or mass selection can be useful (Fehr, Citation1987).
3.2. General combining ability
Estimates of the effects of general combining ability which refers to the average performance of the parents in a series of crosses of rice for yield and yield contributing traits are presented in Table . All the parents had a highly significant GCA effects except parent P6. All the parents which are sourced from AfricaRice had a good general combining ability as compared to those obtained from KAFACI. Genotype (WAC 18-WAT 15-3-1) was the best combining parent for all measured traits. For days to flowering an early flowering variety is highly preferred in areas experiencing shortage of rainfall. African crop production is mainly dependent on rainfed production, which is suffering with erratic rainfall. Due to this, development of early flowering and maturing variety would be a better option.
Table 3. General combining ability effects of seven parents for different agronomic traits
Table 4. Specific combining ability effects of 18 hybrids for different agronomic traits
Variety or crosses with negative GCA and SCA effects for days to flowering could be considered desirable for early maturing crop development. In this study, genotypes SR33701-HB3330-78 followed by SR33859-HB3324-133 had significant negative GCA for days flowering good combining nature. Genotype SR33701-HB3330-78 was also the best source parent for number of effective tillers per hill. Genotypes WAB 1573-22-B-B-FKR 4-2-WAC 1-TGR 3-WAT9-1, WAC 18-WAT 15-3-1, and FAROX 521-357-H1 were exhibited the positive and higher GCA effects for panicle length. In addition to this, parent WAC 18-WAT 15-3-1 had also good combining ability for flag leaf length and flag leaf width. Parents from AfricaRice (WAB 1573-22-B-B-FKR 4-2-WAC 1-TGR 3-WAT9-1, WAC 18-WAT 15-3-1, and WAB2135-WAC B-2-TGR 2-WAT1-1) had a good general combining ability for plant height for tallness, while those from KAFACI (SR33701-HB3330-78, SR33859-HB3324-133 and SR33859-HB3324-93) had showed good general combining ability for dwarfness. Genotypes from AfricaRice (WAB 1573-22-B-B-FKR 4-2-WAC 1-TGR 3-WAT9-1, WAC 18-WAT 15-3-1, WAB2135-WAC B-2-TGR 2-WAT1-1, and FAROX 521-357-H1) had generally a good general combining ability for grain yield.
3.3. Specific combining ability and mean performances
Specific combining ability should be used in combination with hybrid means and GCA of the respective parents for better hybrid selection. Many research results stated that the hybrid combinations with mean performance, desirable SCA estimates and involving at least one of the parents with high GCA would be considered for favorable allele combinations Manivannan and Ganesan (Citation2001) and Gnanasekaran et al. (Citation2006).
The specific combining ability of the crosses are presented in Table 4. Hybrids with significant negative SCA value was good for the development of early maturing rice genotypes. From the total of eighteen crosses only cross combination of WAC 18-WAT 15-3-1 x WAB2135-WAC B-2-TGR 2-WAT1-1, WAB 1573-22-B-B-FKR 4-2-WAC 1-TGR 3-WAT9-1 x WAC 18-WAT 15-3-1, WAB2135-WAC B-2-TGR 2-WAT1-1 x FAROX 521-357-H1, WAB2135-WAC B-2-TGR 2-WAT1-1 x SR33859-HB3324-93, WAB 1573–22-B-B-FKR 4-2-WAC 1-TGR 3-WAT9-1 x SR33859-HB3324-133, and WAB 1573-22-B-B-FKR 4-2-WAC 1-TGR 3-WAT9-1 x WAB2135-WAC B-2-TGR 2-WAT1-1 had showed a significant (P ≤ 0.01) negative of −14.37, −5.4, −3.16, −2.13, −1.32, and −1.06 respectively. Similar to days to flowering, high negative SCA values for plant height is desirable. As a result, from this study all the cross combinations showed anon significant SCA value.
A high negative and desirable SCA were recorded for cross combinations of SR33859-HB3324-93 x SR33859-HB3324-133 (−23.6), WAB 1573-22-B-B-FKR 4-2-WAC 1-TGR 3-WAT9-1 x SR33859-HB3324-133(−10.76), and SR33701-HB3330-78 x SR33859-HB3324-133 (−10.1). Unlike the above two traits a high SCA value is desirable for the traits of number of effective tillers per plant, panicle length, flag leaf length, flag leaf width and grain yield. Out of the eighteen crosses only one cross, WAB2135-WAC B-2-TGR 2-WAT1-1 x FAROX 521-357-H1 showed a positive significant (p ≤ 0.05) SCA value for number of effective tillers per hill, while a positive non-significant SCA were recorded on seven crosses as presented in Table 4. Crosses combinations of WAB2135-WAC B-2-TGR 2-WAT1-1 x SR33859-HB3324-93 (3.5), FAROX 521-357-H1 x SR33859-HB3324-93(3.17), SR33701-HB3330-78 x SR33859-HB3324-93 (3.07), SR33701-HB3330-78 x SR33859-HB3324-133 (2.61), FAROX 521-357-H1 x SR33859-HB3324-133 (2.60), and WAC 18-WAT 15-3-1 x SR33859-HB3324-133(2.49) had exhibited a high and positive significant (p ≤ 0.001) SCA values for panicle length.
Most of the cross combinations revealed anon significant SCA value for flag leaf length and flag leaf width. Only the following cross combinations, WAB 1573-22-B-B-FKR 4-2-WAC 1-TGR 3-WAT9-1 x SR33701-HB3330-78 (4.53) and WAC 18-WAT 15-3-1 x SR33859-HB3324-133(4.21) WAC 18-WAT 15-3-1 x SR33859-HB3324-93(0.26) and FAROX 521-357-H1 x SR33859-HB3324-93(0.16) were revealed desirable SCA values for flag leaf length and for flag leaf width respectively. Except cross combinations of WAB 1573-22-B-B-FKR 4-2-WAC 1-TGR 3-WAT9-1 x WAC 18-WAT 15-3-1(−0.17), WAB 1573-22-B-B-FKR 4-2-WAC 1-TGR 3-WAT9-1 x WAB2135-WAC B-2-TGR 2-WAT1-1(−2.47) and WAC 18-WAT 15-3-1 x SR33859-HB3324-133(−0.53) the remaining fifteen cross combinations showed a significant desirable SCA value for grain yield. For grain yield the following cross combinations, WAC 18-WAT 15-3-1 x SR33701-HB3330-78 (2.54), SR33701-HB3330-78 x SR33859-HB3324-93 (2.34), SR33701-HB3330-78 x SR33859-HB3324-133(2.43) and SR33859-HB3324-93 x SR33859-HB3324-133(1.48) were gave high SCA value in the required direction.
4. Conclusion
Effective crossing approaches should consider the characteristics of the material before incorporating to the breeding program. Studying combining ability effects has great importance in plant breeding as an effective means of selecting potential parents for hybridization and specific crosses for further exploitation. From this study parents which are sourced from AfricaRice had a good general combining ability than genotypes obtained from KAFACI for most characters included in the study.
Parent P2 (WAC 18-WAT 15-3-1) followed by parent P1(WAB 1573–22-B-B-FKR 4-2-WAC 1-TGR 3-WAT9-1) were the best general combiners in the desired direction for days to flowering, number of effective tillers per hill, panicle length, flag leaf length and width, plant height, and grain yield per plant while, crosses involving with this parent, WAC 18-WAT 15-3-1 x SR33701-HB3330-78 (2.54 t/ha) had the high desirable grain yield followed by crosses, SR33701-HB3330-78 x SR33859-HB3324-93 (2.34 t/ha), SR33701-HB3330-78 x SR33859-HB3324-133(2.43 t/ha), and SR33859-HB3324-93 x SR33859-HB3324-133(1.48) which gave high SCA value for grain yield. As a result, these crosses combinations would be advanced and selection can start at early generation.
Acknowledgments
The author gratefully acknowledges Alliance for Green Revolution in Africa (AGRA) project for the financial support and National Crop Resource Research Institute (NaCRRI) of Uganda; especially rice research team for their strong collaboration and hosting this study. Ethiopian Institute of Agricultural Research had also highly accredited for giving study leave and Fogera National Rice Research and Training Center (FNRRTC) is also highly acknowledged for hosting for the author for his internship during studying period.
Additional information
Funding
Notes on contributors
Zelalem Zewdu
This work is part of masters degree in plant breeding and seed systems at Makerere University. The materials were obtained from Korea-Africa Food and Agriculture Cooperation (KAFACI) Initiative project. The author evaluated the parental lines for their agronomic performance, grain yield and disease resistance. The rosses were made with elite Ugandan materials to determined genetic inheritance of the martials for different targeted traits. After he accomplished his study, currently he is working in the National Rice Research Program of Ethiopia as Rice Breeder.
References
- Abney, M., McPeek, M. S., & Ober, C. (2000). Estimation of variance components of quantitative traits in inbred populations. American Society of Human Genetics, 66(2), 629–10.
- Bano, D. A., & Singh, S. P. (2019). Combining ability studies for yield and quality traits in aromatic genotypes of rice (Oryza sativa. L.). https://doi.org/10.5958/0975-928X.2019.00044.9
- CGIAR. (2013). Consultative group for international agricultural research Africa rice center.
- Chakraborty, R., Chakraborty, S., Dutta, B. K., & Paul S. B., 2009. Combining ability for yield and yield components in bold grain rice (Oryza sativa L.) of Assam. Acta Agron., 58(1), 9–14.
- Dewina, H., & Reno, S. (2010). Staple food price in Uganda. https://ageconsearch.umn.edu/record/58553
- Dogara, A. M., & Jumare, A. I. (2014). Origin, distribution and heading date in cultivated rice. International Journal of Plant Biology Research, 2, 2–6.
- FAO. (2014). Food and Agriculture Organization of the United Nations statistics division.
- Fehr, W. R. (1987). Principles of cultivar development, theory and technique. Iowa state university, Macmillan publishing company.
- Gnanamalar, R. P., & Vivekanandan, P. (2013). Combining ability analysis of grain quality traits in rice (Oryza sativa L.). Asian Journal of Plant Science and Research, 3(2), 145–149.
- Gnanasekaran, M., Vivekanandan, P., & Muthuramu, S. (2006). Combining ability and heterosis for yield and grain quality in two-line rice (Oryza sativa L.) hybrids. Indian Journal of Genetics, 66(1), 6–9.
- Griffing, B. (1956). Concept of general and specific combining ability in relation to diallel crossing system. Australian Journal of Biological Sciences, 9, 463–493.
- Hasan, M., Kulsum, U., Lipi, L., & Akm, S. (2013). Combining ability studies for developing new rice hybrids in Bangladesh. Bangladesh Journal of Botany, 42(2), 215–222. https://doi.org/10.3329/bjb.v42i2.18022
- Jacquard, A. (1983). Heritability: One word, three concepts. Biometrics, 39(2), 465–477.
- Manivannan, N., & Ganesan, J. (2001). Line × tester analysis over environments in sesame. Indian Journal of Agricultural Research, 35(4), 225–258.
- Muthayya, S., Sugimoto, J. D., Montgomery, S., & Maberly, G. F. (2014). An overview of global rice production, supply, trade, and consumption. Annals of the New York Academy of Sciences, 1324, 7–14.
- Payne, R. W., Murray, D. A., Harding, S. A., Baird, D. B., & Soutar, D. M. (2009). An introduction to GenStat for windows (12th ed.). VSN International.
- Saidaiah, P. S., Kumar, S., & Ramesha, M. S. (2010). Combining ability studies for development of new hybrids in rice over environments. Journal of Agricultural Sciences, 2(2), 225–233.
- Zeinab, M., Nadali, B., & Jelodar, N. B. (2014). Genetic dissection of some important agronomic traits in rice using line × tester method. International Journal of Advanced Biological and Biomedical Research, 2(1), 181–191.