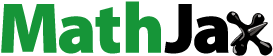
Abstract
In Ethiopia, the wastewater generated from coffee processing industries is often directly discharged to the river due to lack of monitoring facilities. This resulting in water contamination causing risks to entire ecological system and human well-being of the society needs an urgent attention by the environment specialists. Hence, the current research is aimed to investigate the potential of Moringa stenopetala seed powder for the improvement of physicochemical and bacteriological load of coffee processing wastewater. The optimization of adsorbent dose and contact time on the reduction of turbidity and COD were done by the standard method. The Moringa stenopetala seeds were collected from Dilla University campus and ground to fine powder. The obtained powder was characterized by scanning electron microscope and X-ray diffractometer and wastewater was collected from coffee processing site. The SEM and XRD results revealed that Moringa stenopetala seed powder having an amorphous morphology for retention of impurity load. After treatment with this seed powder, the physicochemical and biological quality of the wastewater was significantly improved and there is a 99.43% and 99.16% reduction of turbidity and COD were reached respectively at adsorbent dose of 80 mg L−1 and contact time of 60 min and 45 min respectively. This current research proved that Moringa stenopetala seed powder has a promising potential to improve physicochemical and biological quality of wastewater. Adopting this system can be economically, environmentally, and socially feasible to address wastewater problems. Further research on surface modification of this adsorbent is needed to enhance its removal efficiency.
PUBLIC INTEREST STATEMENT
In Ethiopia, the waste effluent from coffee processing industries is discharged into near rivers because of ignorance and due to lack of monitoring policy implementation. These unwanted activities of ignorance have been reached an alarming situation of environmental contamination that needs an urgent attention by environmental scientists. This results in an alarming rise in concentrations of toxic pollutants in the main water system and resulting in deterioration of water quality. There is an urgent need to safeguard the ecology and wellbeing of society by means of valuable research in discovery of safe, bulk that are easily available at cheap cost. Hence this research is focused on the pollutant removal capacities of Moringa stenopetala seed powder in effluent management generating from coffee processing units.
Competing interests
The authors declare no competing interests.
1. Introduction
Coffee is an important agricultural commodity in the world with a distinct aroma and a unique flavor. It undergoes a long process to make it ready for consumption. This process often entails the use of large quantities of fresh waterfreshwater which causes a rise in solid and liquid effluents (Padmapriya et al., Citation2013) into the environment. The wastewater generated from coffee processing unit contains organic impurities such as pectin, proteins, and sugars (Bello & Castillo, Citation1998). Chemical oxygen demand (COD) value is defined as an amount of dissolved oxidizable organic matter including nonbiodegradable matter present in it (Kalshetty et al., Citation2014). The COD value has been developed analogically to the BOD measurement, where BOD indicates the content of oxygen required for decomposition of organic compounds in wastewater by bacteria within the permissible level of 20 g L−1. COD value is an important tool to assess the water quality (Kitane et al., Citation2020). This test virtually measures all organic compounds that can be digested by using EPA method. By this method it is possible to remove 80% of the pollution load, with permissible level of 50 g L−1 (Jan & Ken, Citation2017). Water turbidity is caused by the presence of suspended particles of a different diameter, ranging from very small colloidal particles to large flocs, which scatter and absorbs electromagnetic radiation in IR and VIS regions. The particles may be of both mineral and organic origin in surface waters; however, organic suspended matter is usually observed in a wastewater treatment plant effluent (Zbigniew & Przemysław, Citation2016).
The socio-economic development is go with an increase in both the wastewater flows discharged and the degree of pollution of surface water, groundwater, and the environment generated by these discharges (Merghem et al., Citation2016). In Ethiopia, especially in the Gedeo Zone, Dilla area the wastewater generated from coffee processing activity is often directly discharged into the river system without any treatment as due to lack of monitoring facilities. This leads in deterioration of water quality (Alemayehu & Rani, Citation2008). Thus poses risks to ecological system and human well-being that deserves scientific attention. Hence, it is important to treat the wastewater from coffee processing activity before discharged to river system. In this regard, there are several technologies available to treat wastewater. However, adsorption methods are the most efficient and popular method. Diaz et al. (Citation1999) confirmed that there are several plant-based adsorbents that have been traditionally used to treat wastewater. Sisay and Ermias (Citation2018) reported that Moringa stenopetala (M. stenopetala) seed powder can be used as a substitute for wastewater treatment. However, there are no complete data on efficiency of M. stenopetala seed powder regarding its optimum dose and contact time required to efficiently remove turbidity and COD from coffee wastewater (CWW).
Therefore, this research is focused on the investigation of the potential of locally available M. stenopetala seed powder as a low-cost adsorbent for the treatment of physicochemical and bacteriological properties of CWW. It also investigates the amount of adsorbent dose and contact time for maximum reduction of turbidity and COD level was major concern in this work. In addition, an adsorption isotherm of the adsorbent was studied.
2. Materials and methods
2.1. Collection and preparation of plant material (adsorbent)
The good-quality dried M. stenopetala seeds were used for this study and were collected from Dilla University main campus and Dilla area, Gedeo Zone, South Nations, Nationalities and Peoples Regional State (SNNPR), Ethiopia. The collected M. stenopetala seeds were cleaned with distilled water and dried at room temperature. The wings and coat from the seeds were deshelled by hand and then grounded using mortar and pestle and were dried again. Finally, they were sieved with 20– 250 µm mesh size to obtain a consistent required powder size and then packed and stored in airtight container until used for adsorption treatment.
2.2. Characterization of Moringa stenopetala seed powder
The morphology and surface features of Moringa stenopetala seed powder were characterized by using scanning electron microscope (SEM- JEOL JSM 6360LA, Japan). SEM analysis was carried out at room temperature with an accelerating voltage of 5 kV. The crystal structure and amorphous nature of the prepared adsorbent was investigated by X-ray diffractometer (XRD) with 2θ scope of 10–80° using CuKα X-ray source. The prepared M. stenopetala seed powder was densely filled into aluminum sample holder. X-ray diffraction analysis of the samples was performed on-diffractometer with an operating conditions of 40 kV and 30 mA, with Cu-Kα radiation wavelength of Lambda = 1.5406 Å. The XRD spectra was analyzed using Origin 8.0 software and the crystalline grain size was calculated from the width of the peaks by taking tallest intense pick, using Scherrer’s formula given in EquationEquation (1)(1)
(1) .
2.3. Wastewater sampling and transportation
The CWW is discharged into Chichu River—a river that drains into another big river where people and livestock use for drinking purpose and the quality profile of water is still unknown. Standard procedure for examination of wastewater was followed (Steven & Pirner, Citation2005). Composite sampling technique according to the procedure explained by APHA (American Public Health Association) (Citation1998) was followed. Wastewater was collected carefully in sterile containers of polythene bottles from coffee processing sites of Gedeo Zone around Dilla town, SNNPR, Ethiopia. The sample bottles were stoppered and sealed with paraffin film. The samples were immediately brought to the laboratory for assessing various physicochemical and bacteriological qualities.
3. Experimental parts
3.1. Adsorption process
All the glassware, burettes and pipettes and other laboratory apparatus were cleaned with tap water thoroughly and next with distilled water and then rinsed with the sample solution before final use. Batch experiments were carried out using a series of 250 mL erlenmeyer flask to investigate the effect of adsorbent dose, and contact time. The adsorption treatment was done at room temperature (28 ± 1°C). Shaking of the mixture of sample and adsorbent was done using electrical shaker and allowed to settle for 60 minutes (optimum settling time). Stirring speed of the electrical shaker was kept constant for each run throughout the experiment for ensuring equal mixing. Each flask was filled with a known volume of sample with desired pH and mixing was initiated. The sample was withdrawn from the shaker at predetermined time intervals and was analyzed for some physicochemical and bacteriological investigation (García-Mateos et al., Citation2015).
3.2. Measurement of physicochemical and bacteriological parameters
The physicochemical properties and biological quality of wastewater were determined before and after treatment with M. stenopetala seed powder using specific methods at optimized experimental parameters (adsorbent dose and contact time). However, color, odor, temperature, and pH of the wastewater were measured on site. The pH of the sample was measured using a portable pH meter (Model HI9024, HANNA Instrument) calibrated with pH 4.0, 7.0, and 10.01 standard buffer solutions. Turbidity of sample was measured with portable turbidity meter (Model 0839, cole-parmer) calibrated with 0.5, 10, and 20 NTU standards. Total solid (TS), total suspended solid (TSS) and total dissolved solid (TDS), COD level, and bacteriological analysis which includes the Aerobic Mesophilic Bacteria (AMB), Total Coliform (TC), and Fecal Coliform (FC) were analyzed in laboratory according to the standard methods prescribed by (APHA [American Public Health Association], Citation1998; Brown et al., Citation1970) before and after adsorption treatment. The plate method technique was used to determine the number of colony-forming units per ml (cfu/mL) of AMB, FC and TC in wastewater. For AMB the plates were incubated at 37 °C for 24 hours using GALLEVKAMP incubator. After incubation, plates with colonies between 30 and 300 were considered for counting AMB. Similarly the TCs plates were incubated at 37°C for 24–48 hours. After incubation, plates with colonies between 30 and 300 were used for determining TC. The FCs culture media were incubated at 37°C for 24 hours. After incubation, purplish red colonies surrounded by reddish zone of precipitated bile were counted as FC.
4. Batch studies
4.1. Study of adsorbent dosages
The jar test was conducted for measuring coagulation activity and to find the optimum adsorbent dose and contact time. The initial mean values of turbidity and COD for wastewater were 785 NTU and 12,905 mg L−1 respectively. This study consists of batch experiments involving rapid mixing, slow mixing, and followed by sedimentation. The result was presented as shown in Figure .
Figure 1. Wastewater before and after treatment with different adsorbent dose (i.e. 20, 30, 40, 50, 60, 70, 80, 90, 100, and 110 mg L−1 adsorbent dose from left to right respectively).
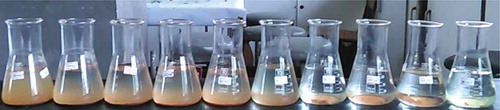
Wastewater sample of 250 mL each was kept with the M. stenopetala as an adsorbent of a series dose (20, 30, 40, 50, 60, 70, 80, 90, 100, and 110 mg L−1), in jar to see the effect of adsorbent dose and the beakers were mixed rapidly for 1 minute at 250 rpm; this was followed by 15 minutes of slowly mixing at 20 rpm to aid in floc formation. The flocculated suspensions were allowed to stand without disturbance for 60 minutes (optimum) for settling. The supernatants were sampled and analyzed for physicochemical and bacteriological parameters.
4.2. Effect of contact time
The effect of contact time on adsorption efficiency was examined with different time intervals 15 to 150 minutes. The sample was withdrawn with the predetermined 15-minute interval for physicochemical and bacteriological analysis.
4.3. Adsorption isotherms
The adsorption experiments were carried out by batch method. Percentage removal of turbidity and COD level were calculated by EquationEquation (2)(2)
(2) .
Where, Co and Ce are initial and final concentration of investigated physicochemical parameters.
The amount of organic matter adsorbed on the adsorbent (qe, mg.g−1) was calculated using EquationEquation (3)(3)
(3) . These data were evaluated by Langmuir and Freundlich adsorption isotherm equations, two equilibrium isotherm models usually used to interpret the efficiency of pollutant sorption.
Where, V is the volume of solution (L) in contact with the biosorbent and M is the mass (g) of the adsorbent.
The equilibrium of the adsorption process is often described by fitting the experimental points with models usually used for the representation of isotherm adsorption equilibrium.
4.4. Statistical analysis of data
Statistical analysis was performed to calculate the mean removal efficiency of M. stenopetala seed powder and to check whether there was a significant difference in concentration between each trial and to see the optimum values of experimental parameters by using t-test and the results were interpreted using Microsoft Excel and Origin Pro 8 SR0, 2007.
5. Result and discussions
5.1. Study of surface morphology for Moringa stenoptela seed powder
The surface morphology of M. stenopetala seed powder was studied for understanding surface characteristic of the powder using SEM. The Figure represents the SEM image of the powder. The surface morphology of the seed powder confirms the heterogeneous and relatively porous matrix of a material (Kebede et al., Citation2018). This structure facilitates the processes of adsorption, due to the interstices and, more importantly, to the presence of the protein component of the seed. Thus, based on these characteristics, it can be concluded that this material has an adequate morphological profile for retaining the particulate and organic matter in the wastewater.
5.2. XRD pattern for M. stenopetala seed powder
The Figure shows the X-ray diffraction patterns of M. stenopetala seed powder and most probable diffraction peaks is at around scattering angle (2θ) of 21.48°. Due to the high amount of oils and proteins present in the composition of the material (around 69% by weight), the X-ray pattern shows a poorly resolved peak indicates a predominance of amorphous material. According to the Scherer calculation, the M. stenopetala seed powder particle size obtained was less than 2 nm (0.81 nm) which is considered as microspores amorphous domain or less crystalline structure. This shows high porosity with small particle size and high surface area that provides greater number of adsorption sites with higher adsorption efficiency.
5.3. Physicochemical and bacteriological effect of M. stenopetala treatment
The results of this study (Tables , and ) showed that, at optimum condition, the use of M. stenopetala seed powder not only diminishes physicochemical parameters of water pollutant but also harmful bacteria. The M. stenopetala seed powder binds to the dissolved solids and ions in the water and makes them sink to the bottom. The optimum adsorbent dose of 80 mg L−1 powder showed maximum reduction of turbidity and COD level 99.43% and 99.22% were achieved at optimum contact time 60 minutes and 45 minutes respectively. The amounts of total dissolved solids in the water are also a rough indicator of mineral content (APHA [American Public Health Association], Citation1998). Before treatment the coffee wastewater had innumerable number of bacterial colonies but after treatment with plant material the number of bacteria got reduced. At the end of the 90th minute there is no bacterial colony at all.
Table 1. Freundlich and Langmuir adsorption isotherm constants
Table 2. Removal efficiency of physical parameters from coffee processing wastewater through adsorption by M. stenopetala seed powder along with world health organization (WHO) permissible limits discharged to water system
Table 3. Removal efficiency of chemical parameters of coffee processing wastewater through adsorption by M. stenopetala seed powder along with WHO permissible limits discharged to the water system
Table 4. Removal efficiency of bacterial load of coffee processing wastewater through adsorption by M. stenopetala seed powder along with WHO permissible limits discharged to the water system
5.4. Effect of M. stenopetala treatment on physical parameters
The results revealed that colour of the coffee effluent was dark brown before treatment and become light greenish brown after treatment with M. stenopetala seed powder and remained same even after optimum dose reached as shown in Figure ) and Table . The reason could be the color of the seed powder. The unpleasant odor was changed to pleasant after 15 minutes treatment as presented in Table . It could be due to the phytochemicals of the M. stenopetala seed powder. Colour is very important factor for aquatic life for making food from sun rays. The photosynthetic activity is found to be reduced due to dark coloration. Dark color will affect other parameters like temperature, dissolved oxygen (DO), and biological oxygen demand (BOD) (Kalshetty et al., Citation2014). Odor of water is caused both by chemical agents like hydrogen sulfide, free chlorine, ammonia, phenols, alcohols, esters, hydrocarbons, and biological agents such as algae, fungi, and other microorganisms (Kalshetty et al., Citation2014). Initial pH of wastewater was acidic and significantly increased after adsorption treatment. Interestingly, the temperature of the coffee effluent remained the same before and after treatment. All physiological activities and life processes of aquatic organisms are generally influenced by water temperature (Kalshetty et al., Citation2014), because it affects the biochemical reactions in aquatic organisms. The pH is most important in determining the corrosive nature of water. The lower the pH value higher is the corrosive nature of water. The uses of M. stenopetala seed as natural coagulant significantly increase the pH of CWW almost to the neutral see Table . The possible mechanism may be during fermentation process volatile fatty acids (VFAs) and H2 can be produced in which both produce NH3 leads to pH increase (Jules et al., Citation2008). Subsequently, after treatment of 75 minutes there is no pH increase observed. In addition, the microorganisms present in wastewater treatment system use organic materials as an energy and carbon source for the synthesis of new cells, respiration, and mobility. Thus, absence of organic materials gives a negative result as indicated by growth limitation or inhibition (Gray, Citation2004). It is likely that since the treatment was able to enhance the reduction of the organic materials in coffee wastewater thus leading to a decrease in the availability of food resource; this resulted in an inhibition of the growth of microorganism such as the acid-producing bacteria which caused coffee wastewater become neutral or more alkaline (Radojević & Bashkin, Citation1999). In general, this treatment showed a significant improvement in pH value from 3.7 to 7.1; this remained well within the standard value for wastewater discharge (pH 6.8–8.5). The result indicates that the proposed system effectively increased the final pH values of treated CWW without any additional chemical additives.
More than 99.43% reduction of turbidity was observed in this study at optimum adsorbent dose and contact time (80 mg L−1 and 60 minutes respectively). The predominant mechanism for high adsorption between coagulation active components in M. stenopetala and particles of suspension may be due to permit inter-particle bridging. Also the efficiency of adsorption depends on the adsorbent surface area, surface morphology, pore size distribution, polarity, and functional groups attached to the adsorbent surface (Ali et al., Citation2016). This indicates that naturally dried M. stenopetala seed contains more active ingredients with more surface area available for adsorption and inter-particulate bridging (Joseane et al., Citation2013).
5.5. Chemical parameters removal efficiency of M. stenopetala
From Table it was observed the initial chemical characteristic of coffee wastewater is high. The initial COD value of 12,905 mg L−1 was far higher than the standard value discharge to the water system. COD values conveyed the amount of dissolved oxidizable organic matter including non–biodegradable matter present in it (Kalshetty et al., Citation2014). It is obvious that high value of COD is often associated with the strength of organic contents in wastewater which indicates the higher polluting power to the ecosystem (Penn et al., Citation2009). The high COD values in CWW, therefore constitute a high concentration of organic matters. As shown in Table all the investigated parameters were reduced gradually after treatment with M. stenopetala seed power. More than 99.16 % reduction of COD was observed in this study at optimum adsorbent dose and contact time (80 mg L−1 and 45 min) respectively. The obtained result was below the WHO guideline permissible limits discharged to the water system for industrial wastewater. The results also suggested that COD removal is associated with the reduction of organic materials suspended in wastewater which also indicated that powdered M. stenopetala seed has a high ability to bind the organic matters suspended in CWW. The use of powdered M. stenopetala seed certainly enhanced the purification process, thus significantly decreases COD values in the CWW. The probable explanation for this phenomenon is due to the high absorption of the organic materials onto the adsorbent active site. As shown in Table the initial electrical conductivity (EC) of coffee wastewater is high and it is far higher than the standard value discharge to the water system. EC values measure the mobility of dissolved ions in a solution and correlate with the total dissolved solids (TDS) contents. As time increase EC values significantly decrease, this may be due to the dissolved ions adsorbed to the active site of the adsorbent surface.
5.6. Bacteriological parameters removal efficiency of M. stenopetala
Microbiologically, the results revealed that, before treatment the coffee effluent has innumerable number of bacterial colonies but after treatment with M. stenopetala the number of bacteria got reduced. After 90 minutes of treatment almost all plates did not exhibit bacterial growth (colony) see Table . The reduction observed in the bacterial population of wastewater treated with M. stenopetala seed powder was attributed to the antibacterial properties of the bioactive ingredient in M. stenopetala (Padmapriya et al., Citation2015).
5.7. Effect of experimental parameters (adsorbent dose and contact time)
The effect of adsorbent dose was investigated on removal efficiency of turbidity and COD by keeping other parameters constant. As shown in Figure ), the percent removal efficiency was increased as adsorbent dose increases from 20 to 80 mg L−1 and becomes constant at higher doses for removal of turbidity and COD. This is because at higher dose of adsorbent, due to increased surface area, more adsorption sites are available causing higher removal. However, the increase of the dose of powdered M. stenopetala seed after optimum dose reached did not remove turbidity and COD in CWW. The one-way ANOVA confirmed that an increase in doses of M. stenopetala seed powder did not significantly differ from each other in terms of turbidity as well as COD removal (P > 0.05). This could be probably the system has been reached adsorption-desorption equilibrium position. This suggests that the adsorbed species/solute may either block the access to the internal pores or cause particles to aggregate and thereby resulting in the reduction of availability of active sites for adsorption reported by SivaKumar et al. (Citation2017). The influence of contact time on removal efficiency of turbidity and COD was investigated in the range of 15–150 minutes at the optimized dose (Figure )). Maximum removal of turbidity and COD was occurred within 60 and 45 minutes of adsorption treatment respectively. After 60 and 45 minutes the increase in the percentage removal of turbidity and COD are not significant respectively. This could be probably the adsorption-desorption equilibrium is reached. Similar trends have been reported in literature on the extent of removal of manganese (II) ions (SivaKumar et al., Citation2017). Similar COD removal trend was reported by Chatouia et al. (Citation2017) from vegetable oil refinery wastewaters using ferric chloride as a coagulant.
5.8. Adsorption isotherm
The equilibrium relationships between adsorbent and adsorbate are described by the adsorption isotherms. The adsorption curves were applied to both the Langmuir and Freundlich equations. The Freundlich isotherm model assumes that the adsorption occurs on heterogeneous surfaces, is often expressed as:
This equation can be linearized as follows:
Where KF is Freundlich isotherm constant (lg−1) and n is Freundlich isotherm exponent (dimensionless).
Values of KF and n were calculated from the intercept and slope of plots lnqe vs lnCe and a straight line indicates the confirmation of the Freundlich isotherm for adsorption, see Table . The value of n should be greater than one confirming good adsorption capacity of the adsorbent (Table ) otherwise desorption becomes predominant. According to (Bhatt et al., Citation2012), if a value of n = 1, the adsorption is linear, for n < 1, the adsorption is chemisorption and for n > 1 the adsorption is a favorable physical adsorption. Langmuir isotherm, which assumes that a monolayer of adsorbate is formed on a relatively regular adsorbent surface, using the partially protonated groups of the adsorbent.
The Langmuir isotherm has been successfully applied to many real sorption processes and is expressed as follows:
Where qe is the amount adsorbed at equilibrium (mgg−1), Ce the equilibrium concentration (mg L−1), b a constant related to the energy or net enthalpy of adsorption (Lmg−1), and Q0 the mass of adsorbed solute required to saturate a unit mass of adsorbent (mgg−1). Q0 represents a practical limiting adsorption capacity when the surface is fully covered with the adsorbate and allows the comparison of adsorption performance particularly in the cases where the adsorbent did not reach its full saturation in experiments. The Langmuir equation can be described by the linearized form as follows:
A plot of (Ce/qe) vs Ce gives a straight line with slope 1/Qo and intercepts 1/Qob. Qo and b can be determined from this plot (Table ). The essential characteristics of Langmuir isotherm can be expressed in terms of a dimensionless constant, separation factor, or equilibrium parameter, RL, which is defined by:
According to Abdel and Moamen (Citation2011), RL value indicates the adsorption nature to be either unfavorable if RL>1), linear if RL = 1, favorable if 0< RL<1 and irreversible if RL = 0. From the data calculated in Table , the adsorption of COD and turbidity on to M. stenopetala seed powder confirmed by the high correlation coefficient R2 suggested that Langmuir models provide good model and best fitted the adsorption systems. The higher values of R2 (> 0.95) achieved for the studied adsorption isotherms models show that the adsorption process of COD and turbidity from treated wastewater by prepared M. stenopetala seed powder in this work can be well explained by the models of Langmuir and Freundlich isotherms.
6. Conclusion
It is concluded from the investigated physicochemical and bacteriological analysis of the CWW that all parameters were much more than the prescribed limits. M. stenopetala seed powder was effective in removal of physicochemical impurities from the wastewater. Likewise, M. stenopetala was effective in the inhibition of bacterial contamination. In removal of turbidity and COD at first the adsorption is very rapid as there are lots of free sites for the adsorption to take place. The 99.43% and 99.16% reduction of turbidity and COD was reached respectively at adsorbent dose of 80 mg L−1 and contact time of 60 min and 45 min respectively. The data for COD removal fit well with Langmuir isotherm model whereas adsorption by M. stenopetala seed powder can be modeled by both Freundlich and Langmuir isotherms. Thus it can be concluded that seeds of M. stenopetala can be used for the removal of organic matter and improves the physicochemical and bacteriological parameters of the wastewater. The present study work explored the development in the field of wastewater treatment technology at low cost and easy available material and explored the potential application of M. stenopetala seed powder for industrial wastewater treatment. Adopting this system can be economically, environmentally, and socially feasible to address wastewater problems of coffee industry. Further research on surface modification of this adsorbent is needed to enhance its removal efficiency.
Acknowledgements
The authors are very thankful to office of the vice president for research and technology transfer of Dilla University (DU) for financial support.
Additional information
Funding
Notes on contributors
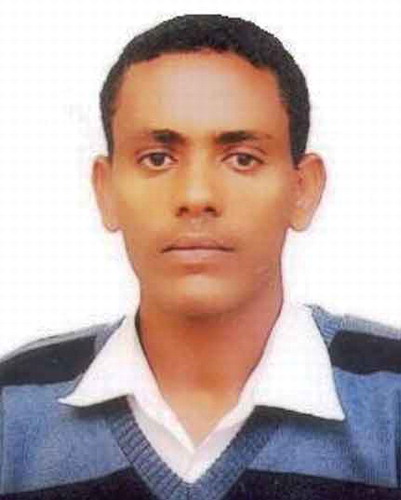
Workineh Mengesha Fereja
Workineh Mengesha Fereja is currently a staff member of Chemistry Department and Vice Director of Energy and Environment Research Center at Dilla University, Ethiopia. His specialization is in Environmental Sanitation. He worked on environmental analysis, the physical-chemical behavior and partitioning of organic micro-pollutants in/between the different environmental matrices. Mr. Fereja has been working research on the application of the current technologies for the quantification, prevention, and remediation of environmental pollution where the focus area is waste/wastewater treatment technology. Part of his research results have been published in international journal, Journal of Applied Chemical Science International, Sivakumer, Workineh M. Fereja, & Mohalakshmi (2017). Prosopisnjuliflora carbon and commercial activated carbon in the pollutant reduction technology. 8(1): 1-5. The experience of the applicant is ranging from environmental analysis combine preventive and remediation technology. So the manuscript submitted for publication is one of the projects among the research activities in our lab group.
References
- Abdel, G., & Moamen, I. 2011. Evaluation of synthetic zeolite as engineering passive permeable reactive barrier. [Degree of Master of philosophy]. Cairo University.
- Alemayehu, H., & Rani, D. (2008). Effect of effluent generated from coffee processing plant on the water bodies and human health in its vicinity. Journal of Hazard Materials, 152(1), 259–14. https://doi.org/10.1016/j.jhazmat.2007.06.094
- Ali, R. M., Hamad, H. A., Hussein, M. M., & Malash, G. F. (2016). Potential of using green adsorbent of heavy metal removal from aqueous solutions: Adsorption kinetics, isotherm, thermodynamic, mechanism and economic analysis. Ecological Engineering, 91, 317–332. https://doi.org/10.1016/j.ecoleng.2016.03.015
- APHA (American Public Health Association). (1998). Standard methods for the examination of water and wastewater (20th ed.). Washington D.C.
- Bello, M. R., & Castillo, R. M. F. (1998). Start-up of an anaerobic hybrid (UASB/filter) reactor treating waste water from a coffee processing plant. Journal of Science Direct, 4(5), 219–225. https://doi.org/10.1006/anae.1998.0171
- Bhatt, A. S., Sakaria, P. L., Vasudevan, M., Pawar, R. R., Sudheesh, N., Bajaj, H. C., & Mody, H. M. (2012). Adsorption of an anionic dye from aqueous medium by organo clays: Equilibrium modeling, kinetic and thermodynamic exploration. Royal Society of Chemistry Advances, 2(23), 8663–8671. https://doi.org/10.1039/C2RA20347B
- Brown, E., Skougstad, M. W., & Fishman, M. J. (1970). Methods for collection and analysis of water samples for dissolved minerals and gases (Techniques of Water Resources Investigations of the U.S. Geological Survey). United States government printing office.
- Chatouia, M., Lahsaini, S., Aguelmous, A., Souabia, S., Bahlaoui, M. A., & Jada, A. (2017). Removal of oil and grease from vegetable oil refinery wastewaters by coagulation-flocculation process. Moroccan Journal of Chemistry, 5(4), 683–690. http://revues.imist.ma/?journal=morjchem&page=login
- Diaz, A., Rincon, N., Escorihuela, A., Fernandez, N., Chacin, E., & Forster, C. F. (1999). A preliminary evaluation of turbidity removal by natural coagulants indigenous to Venezuela. Process Biochemistry, 35(3–4), 391–395. https://doi.org/10.1016/S0032-9592(99)00085-0
- García-Mateos, F. J., Ruiz-Rosas, R., Marqués, M. D., Cotoruelo, L. M., Rodríguez-Mirasol, J., & Cordero, T. (2015). Removal of paracetamol on biomass-derived activated carbon: Modeling the fixed bed breakthrough curves using batch adsorption experiments. Chemical Engineering Journal, 279, 18–30. https://doi.org/10.1016/j.cej.2015.04.144
- Gray, N. F. (2004). Micro-organisms and pollution control. Biology of wastewater treatment (Vol. 4). Imperial College Press.
- Jan, C. V. E., & Ken, C. (2017). Review of coffee waste water characteristics and approaches to treatment. Research Gate. https://www.researchgate.net/publication/2380840988
- Joseane, D. P. T., Guilherme, F. L., & Ricardo, F. Z. (2013). Coagulant and natural polymer Perspective for Treatment of Water. Plastic and Polymer Technology, 2(3), 55–62.
- Jules, B. V., Nidal, M., & Grietie, Z. V. (2008). Anaerobic waste treatment. In J. B. Van (Ed.), Biological waste treatment: Principles, modeling and design (pp. 401–442). IWA publishing.
- Kalshetty, B. M., Hobha, S. N., Kalashetti, M. B., & Gani, R. (2014). Water quality of river tungabhadra due to the discharge of industrial effluent at Harihar, District Davanagere, Karnataka State, India. American Journal of Advanced Drug Delivery, 2(1), 120–132.
- Kebede, T. G., Alemayehu, A., Simiso, D., Thabo, T. I., & Nkambule, M. M. N. (2018). Study on adsorption of some common metal ions present in industrial effluents by Moringa stenopetala seed powder. Journal of Environmental Chemical Engineering, 6(1), 1378–1389. https://doi.org/10.1016/j.jece.2018.01.012
- Kitane, M., Ihihi, A., Bahloul, A., El, K. K., & Benqlilou, C. (2020). New Eco-friendly process tanning by concentrated recovered from olive oil mill wastewater. Moroccan Journal of Chemistry, 8(2), 439–445. http://revues.imist.ma/?journal=morjchem&page=login
- Merghem, K. A., El, H. H., Mokhtari, O., Alnedhary, A. A., Belkhir, H., Dssouli, K., Ait, N. F., Elkhadir, G., & Chetouani, A. (2016). Quality assessment and potential reuse of treated wastewater by activated sludge (Sana’a city, Yemen). Moroccan Journal of Chemistry, 4(3), 731–742.
- Padmapriya, R., Jenny, A., & Thirunalasundari, T. (2013). Coffee waste management-An overview. International Journal of Current Research, 9(1), 83–91.
- Padmapriya, R., Jenny, A., & Thirunalasundari, T. (2015). Treatment of coffee effluent by Moringa oleifera seed. International Journal of Current Microbiology and Applied Sciences, 4(1), 288–295. https://www.researchgate.net/publication/330900463
- Penn, M. R., Pauer, J. J., & Mihelcic, J. R. (2009). Biochemical oxygen demand. In A. Sabljic (Ed.), Environmental and ecological chemistry, Isle of Man (Vol. 2, pp. 278). UNESCO-EOLSS.
- Radojević, M., & Bashkin, N. V. (1999). Practical environmental analysis. The Royal Society of Chemistry.
- Sisay, T., & Ermias, H. (2018). Moringa stenopetala seed cake powder as biosorbent for wastewater treatment and its adsorption isotherm and thermodynamic study. Food Science and Quality Management, 80(1), 9–15.
- SivaKumar, K. K., Fereja, W. M., & Mahalakshmi, S. (2017). Prosopis juliflora carbon and commercial activated carbon in the pollutant reduction technology. Journal of Applied Chemical Science International, 8(1), 1–5. https:/www:ikprress.org/index.php/JACSI/article/view/4092
- Steven, M., & Pirner, S. (2005). Standard operating procedures for field Sampler. State of South Dakota Department of Environment and Natural Sources Water Resources Assistance Program, 1, 10–40.
- Zbigniew, M., & Przemysław, K. (2016). Turbidity measurements as a tool of monitoring and control of the SBR effluent at the small wastewater treatment plant – Preliminary study. Archives of Environmental Protection, 42(3), 33–36. https://doi.org/10.1515/aep-2016-0030