Abstract
Nitrogen is the most limiting nutrient for growth of most crop plants in sub-Saharan Africa and legume crops largely depend on fixed nitrogen from indigenous nitrogen-fixing bacteria. The identification and symbiotic characterization of indigenous rhizobia is the basis for inoculants formulation for sustainable legume production in the face of climate change. The aim of this study was to evaluate the diversity of indigenous rhizobia responsible for cowpea nodulation in farmers’ fields across three Counties of Western Kenya. The symbiotic performance of abiotic stress-tolerant rhizobia in their homologous cowpea was also assessed. The results showed high genetic variability among the rhizobia isolates based on morphology and ERIC-PCR fingerprinting. Nine indigenous rhizobia isolates tolerant to extreme temperature, salinity and pH were tested for symbiotic efficiency in a glasshouse experiment. The rhizobia isolates were able to efficiently nodulate cowpea and showed wide variations in their symbiotic efficiency (SE). Five isolates (M2bii, B6ii, B2i, V5ii and V11i) showed significantly higher SE (p < 0.001) ranging from 114.6–179.6% compared to the commercial inoculant (CIAT 899) and N supplemented treatment. Further, six isolates with variability in symbiotic efficiencies were subjected to nifH symbiotic gene sequencing and confirmed their identities as Bradyrhizobium and Rhizobium. These isolates have potential for inoculant formulation. The results indicates the existence of indigenous rhizobia isolates which can tolerate environmental stresses and are symbiotically effective and thus can be developed into inoculants to improve the productivity of cowpea in Kenya.
PUBLIC INTEREST STATEMENT
Cowpea (Vigna unguiculata L. Walp) is a popular food grain legume playing a significant role in the economy of many African countries. The nitrogen fixing symbiosis of cowpea with rhizobia plays an important role in supplying sufficient N for legumes and subsequent non-leguminous crops. The isolation and characterization of elite local strains of rhizobia can provide a basis for the formulation of a rhizobia bio-inoculant. This study has revealed the presence of highly effective rhizobia in Kenyan soils that can be exploited for inoculants production as an alternative to the use of expensive chemical-based fertilizers. Majority of the indigenous rhizobial symbionts exhibited high nitrogen fixing efficiency which clearly demonstrated their potential for use in inoculants formulation to achieve maximum legume productivity.
1. Introduction
Nitrogen is a major mineral nutrient required for the growth and productivity of crop plants and its deficiency adversely affects the growth and yield of crops (Gothwal et al., Citation2008). Nitrogen is essential in plant cells for synthesis of proteins, enzymes, chlorophyll, DNA, RNA and growth regulators thus essential for plant growth and production of food and feed. Its deficiency causes reduced growth, leaf yellowing, reduced branching and small trifoliate leaves in legumes (Simon et al., Citation2014). Providing nitrogen to a crop by application of synthetic nitrate fertilizers is costly to the smallholder farmers. World consumption of nitrogen fertilizer was 119.4 million tons in 2018, of which Africa accounted for 8 percent of the consumption (www.fao.org). Other than consumption of non-renewable energy reserves, the environmental pollution through the escape of fertilizer nitrogen from the root zone is high as the fertilizers are not used efficiently by crops (Sessitch et al., Citation2013). Despite the dramatic increase in the application of synthetic fertilizers (N) to crops in the last 50 years from 12 Tg/year to current 104 Tg/year there has been remarkable decrease in yield with persistence cycle of poverty among many smallholder farmers. Chemical fertilizers are expensive and its imbalanced application can affect soil health. Hence application of beneficial N-fixing microorganisms as a substitute to chemical N fertilizer in an integrated approach is a viable strategy (Onyango et al., Citation2015). It is well known that biological N fixation is one way of converting elemental inert atmospheric N into plant usable form as organic compounds through its enzymatic reduction by N-fixing microbes such as rhizobia (Gothwal et al., Citation2008).
The legume-rhizobia symbiosis has a large impact on productivity of legumes and plays a significant role in fixation of atmospheric nitrogen (Silva et al., Citation2012). This symbiosis can provide an inexpensive way to enhance soil fertility and improve crop production in developing countries. The root nodule rhizobia approximately reduce 20 million tons of atmospheric nitrogen to ammonia which is 50–70% of the world biological nitrogen fixation (Simon et al., Citation2014). The nitrogen supplied through this symbiotic association is in readily assimilable forms of ammonia and in sufficient quantity to make mineral-N fertilization unnecessary. Encouraging the use of biological nitrogen fixation (BNF) serves a means to mitigate green-house gas emissions which lead to depletion of ozone layer. Rhizobia may also act as non-symbiotic plant growth promoting bacteria as in the case of economically important non-legume crops such as rice or wheat, which are the best studied examples that benefit from rhizobia as endophytes (Laranjo et al., Citation2014).
Cowpea (Vigna unguiculata L. Walp) is a popular food grain legume playing a significant role in the economy of many African countries. The annual global production is estimated over 3 million tons. The crop is adapted to heat, drought and low nutrient environment (Mariam et al., Citation2015). It is primarily grown by small-scale producers and represents one of the main subsistence crops because it can be used as grain and green pods with high protein content and green manure (Zilli et al., Citation2004). Small-scale farmers who are the major legume producers in Western Kenya rarely apply fertilizers during legume production due to their low income status (Laranjo et al., Citation2014). Hence, the crop is largely dependent on fixed nitrogen from native nitrogen fixers. The most limiting factor to productivity of cowpea is low nutrient availability, especially P and N in agricultural soils. However, cowpea is a promiscuous plant and can establish symbiosis with several species of rhizobia (Moreira et al., Citation2006), receiving most of its nitrogen from biological fixation. Symbiotic promiscuity is beneficial when legumes associate effectively with different rhizobia species allowing their adaptation to different environments. Bradyrhizobium has been shown to be the predominant microsymbiont of cowpea in agricultural soils. Several studies on the diversity of the nitrogen-fixing Leguminosae-associated nodulating bacteria have used cowpea as the trap plant species (Amanda et al., Citation2012).
The survival of rhizobia in nature depends on the genetic and physiological traits of the organisms in addition to the environmental conditions (Agrawal et al., Citation2012). Edaphic factors and prevailing environmental conditions such as soil pH, phosphorous level, temperature, salinity and soil moisture content have been reported to determine the diversity and distribution of native rhizobia populations (Ligiane et al., Citation2010). In the rhizobia-legume symbiosis, the process of N2 fixation is strongly related to the physiological state of the host legume. A competitive and persistent rhizobial strain is not expected to express its full capacity for nitrogen fixation if limiting factors (such as salinity, unfavorable soil pH, nutrient deficiency, mineral toxicity, temperature extremes, insufficient or excessive soil moisture and plant diseases) impose limitations on the vigor of the host plant (Hussein, Citation1999). Temperature affects root hair infection, bacteroid differentiation, nodule structure, and the functioning of the legume root nodule (Hussein, Citation1999; Simon et al., Citation2014). In addition, the relative activity of the rhizobia is altered by temperature, so that a bacterium that is highly effective at one temperature is less active at different temperatures (Monica et al. Citation2013). The favourable temperature range for root hair development and large number of infection is 15 to 20 °C. This is supported by the study by Lemaire et al. (Citation2015) which demonstrated that host genotype mainly determines rhizobial diversity although other ecological factors such as temperature and soil acidity were positively correlated with genetic variation of Mesorhizobia. In addition, the relationship between the host and environmental factors play a significant role in the distribution of rhizobia.
The dependence of rhizobia nitrogen fixation in legume production is often affected by several environmental stresses such as drought, soil salinity and acidity. Despite the important role played by rhizobia in nitrogen fixation, there is limited information on the effect of abiotic stresses (increased soil acidity and salinity, and temperature) on indigenous rhizobia populations and their functionality in farmers’ fields. The identification and selection of effective indigenous rhizobia strains can contribute to the improvement of symbiotic nitrogen fixation under wide range of environmental stresses. Research efforts to evaluate the diversity of the rhizobial community aiming to obtain new efficient rhizobial strains have been conducted in the eastern and coastal regions of Kenya (Mathu et al. Citation2012; Ndungu et al., Citation2018). Despite the presence of highly diverse cowpea-nodulating rhizobia in Kenya, little is known about their diversity, especially in western Kenya. Knowledge of the morpho-genetic characterization of the indigenous rhizobia in Western Kenya will help develop ecological strategies to ensure survival in the prevailing environmental conditions as well as effects of future climate change. Increased interest in the utilization of symbiotic N fixing bacteria as biofertilizers in agriculture has prompted studies of their diversity. Improving knowledge on the genetic diversity of symbiotic bacteria is important in understanding the role of biological N fixation in improving agricultural productivity. The objective of this study was to determine the morpho-genetic diversity of rhizobia populations from agricultural soils of Western Kenya and evaluate the symbiotic efficiencies of isolates tolerant to extreme environmental conditions (acidity, salinity and high temperature).
2. Materials and methods
2.1. Source of root nodules
Root nodules were collected from a local cowpea variety (K80) grown in agricultural farms of three Counties [Kakamega (0.33 N, 34.71E), Vihiga (0.038 N, 34.71E) and Busia (0.46 N, 34.10E)] in Western Kenya. The three counties were chosen for their contrast in the amounts of rainfall, annual temperatures and predominant soils. The selection of cowpea variety (K80) by farmers was based on farmers’ preference. The selection criterion of the farms to collect root nodules was based on the history of having grown cowpea for the last two years and no prior rhizobia inoculations.
2.2. Sampling of root nodules and soil
The root nodules of cowpea used in this study were collected from 30 farmers’ fields, with 12, 11 and 7 fields from Kakamega, Busia and Vihiga Counties, respectively. All nodules were collected at flowering stage of the plants. Five plants were dug from each farmer’s field, the nodules detached and placed in plastic vials containing silica gel and transferred to the laboratory at the Department of Biochemistry, University of Nairobi for rhizobia isolation. Soil samples were collected at the time of as nodule collection by digging at 20 to 30 cm depth. The composite soil samples were placed in plastic bags and transferred to the laboratory for chemical analysis. The reference strain Rhizobium tropici CIAT 899 was obtained from the microbiological Resource Centre (MIRCEN), University of Nairobi.
2.3. Soil analysis
Soil samples were air dried, crushed using a mortar and pestle and passed through a 0.5 cm mesh to remove any plant or grass fragment. The prepared soil samples were analyzed at the Department of Soil Science Laboratories, University of Nairobi. Soil pH was evaluated using a Pye-Unican pH meter Model 290-MK2 (Mettler-Toledo Inc, Canada) at a soil to water ratio of 1:2.5 (Okalebo et al., Citation2002). A modified Walkley-Black dry combustion method was used to determine the percentage of organic C in the soil samples (Nelson & Sommers, Citation1982). Total N was evaluated using the macro-Kjedahl method as modified by Anderson and Ingram (Citation1989) while available P was analyzed by the Bauschand Lomb supertonic spectrophotometer after extraction using the Bray P1 method (Bray & Kutz, Citation1945) and exchangeable K was extracted using ammonium acetate and evaluated using flame photometry as described by Anderson and Ingram (Citation1993). Soil texture was analyzed using hydrometer method as described by Bouyocous (Citation1962).
2.4. Isolation of cowpea rhizobia
The collected intact nodules were washed under running tap water to remove adhering soil particles. The nodules were immersed in sterile glass beaker containing 70% ethanol for 1 minute then transferred to 3% (v/v) sodium hypochlorite solution for 4 minutes and finally rinsed five times with sterile distilled water (Somasegaran & Hoben, Citation1994). The surface sterilized nodules were crushed with a pair of flame-sterilized blunt-tipped forceps in a 100 µl of sterile distilled water. A loopful of the nodule suspension was streaked on yeast extract mannitol agar (YEMA) plates then incubated at 28 °C in the dark for 10 days. After growth, a single colony was picked and purified by re-streaking on fresh YEMA medium.
The bacterial isolates were named using the site of collection as prefix (M = Mumias in Kakamega, B = Busia and V = Vihiga) followed by the field number and the roman numbers indicates the number of isolates from each field.
2.5. Biochemical and morphological characterization of the isolates
For initial identification and characterization, biochemical and morphological tests were used (Aynalem et al., Citation2018). The biochemical tests including Gram staining (Somasegaran & Hoben, Citation1994) growth on YEMA with Congo red dye and growth on YEMA with bromothymol blue (Vincent, Citation1970) were performed. For morphological characterization, the isolated colonies were sub-cultured on YEMA plates and incubated for 3–10 days at 28 °C. The colony morphology was evaluated and parameters recorded were colour, form and elevation, margins, size and mucus production. The mucous morphology analysis was based on type and elasticity of mucus (Aynalem et al., Citation2018).
2.6. Abiotic stress tolerance of cowpea nodulating bacteria isolates
The isolates were tested for pH, temperature and salinity tolerance. YEMA plates whose pH had been adjusted to pH 4, 6, 8 and10 using 0.1 N HCl for acid and 0.1NaOH for alkaline pH before autoclaving were streaked with rhizobia isolates. The plates were incubated at 28 °C for 10 days and the control was at pH 7. The growth rate was evaluated as described by Aynalem et al. (Citation2018). Temperature tolerance was tested by incubating plates streaked with rhizobia isolates at 20, 25, 30, 35, 40 and 45 °C for 10 days and growth rate evaluated (Rhitu et al., Citation2012). Tolerance to salinity was assessed by determining the growth of the isolates on YEMA plates supplemented with 0, 1, 3, and 5% (w/v) NaCl. Control was at 0.01% (w/v) NaCl. The plates were streaked with rhizobia isolates and incubated at 28 °C for 10 days and the growth rate was evaluated (Rhitu et al., Citation2012).
2.7. ERIC-PCR genomic fingerprinting of rhizobia isolates
Based on the results of morphological clustering, 48 isolates (24, 15 and 9 isolates from Kakamega [Mumias], Busia and Vihiga, respectively) from different subclusters of the dendrogram (data not shown) were selected for molecular characterization with the ERIC-PCR markers. Total genomic DNA of the rhizobia isolates and the reference strain CIAT 899 was extracted according to the method described by Niste et al. (Citation2013). The quality and quantity of isolated DNA was checked by 1% (w/v) agarose gel electrophoresis and spectrophotometer (Hitachi), respectively.
The intergenic repetitive sequences of the genomic DNA were amplified using the enterobacterial repetitive intergenic consensus (ERIC) primers (ERIC-1 5ʹ ATG TAA GCT CCT GGG GAT TCA C and ERIC-2 5ʹ AAG TAA GTG ACT GGG GTG AGC G 3ʹ) (Versalovic et al., Citation1994). Polymerase chain reaction (PCR) amplification was performed in a 20 µl total reaction containing 4 µl of PCR pre-mix, 2 µl genomic DNA, 1 µl of forward and reverse primer and 12 µl nuclease free water. The amplification was performed in a Bio-Rad thermal cycler. The PCR temperature profile used was 94 ºC for 5 minutes followed by 35 cycles of 94 ºC for 30 seconds, 52 ºC for 1 minutes, 72 ºC for 1 minute with a final extension of 72 ºC for 8 minutes. The amplified products were confirmed on 2% (w/v) agarose gel electrophoresis stained with ethidium bromide (1 mg/ml) and photographed under ultraviolet (UV) transilluminator.
The sizes of ERIC amplified fragments were estimated by comparison with the molecular weight marker. ERIC fingerprints were recorded as binary data i.e. 1 and 0 for presence and absence of a band. All data was scored for two independent experiments. A simple matching coefficient was calculated to construct a similarity matrix and the unweighted pair group mean arithmetic (UPGMA) algorithm was used to perform hierarchical cluster analysis and to construct a dendrogram using NTSYS-pc package (Rohlf Citation1990).
2.8. Evaluation of symbiotic effectiveness of abiotic stress-tolerant rhizobia isolates
Nine isolates exhibiting tolerance to abiotic stress (pH, temperature and salinity) were selected for studying their symbiotic efficiency. Rhizobia isolates M2bii, M7b, B2i, B6ii, V4 and V11i displayed tolerance to low pH (acidity), B2i and V5ii showed tolerance to high temperatures and V9i was tolerant to high salinity. Each isolate was authenticated as root nodulating bacteria by inoculating 1 ml of 72 hour pure YEM broth culture on the host plant (cowpea K80) grown in a controlled environment in 2 kg plastic plant pots filled with sterilized soil. The seeds were sterilized as per the method described by Amanda et al. (Citation2012). The pots were arranged in a complete randomized design (CRD) with five replicates. Treatments without chemical nitrogen fertilizer and inoculation served as negative (C-) control while treatments without inoculation plus nitrogen fertilizer applied at a rate of 70 µg N mL−1 in form of KNO3 served as positive (C+) control. This solution was supplied to the positive control plants once every week (Somasegaran & Hoben, Citation1994). Plants were supplied with tap water after every 2 days and fertilized with quarter strength N-free nutrient solution once a week (Aynalem et al., Citation2018). The experiment was carried out in a glasshouse with a 12 hour photoperiod. After 50 days of growth, the plants were harvested and the roots were scored for nodulation. The plants were oven dried at 70 ºC for 48 hours and nodule number (NN), nodule dry matter (NDM), root dry matter (RDM) and shoot dry matter (SDM), were determined. Total nitrogen content (SNC) per plant was analyzed using the Kjedahl method (Anderson & Ingram, Citation1993). Symbiotic efficiency (SE) was evaluated by comparing each of the indigenous rhizobial isolates with chemical nitrogen applied controls (plant N content in inoculated pots/plant N content in N application) × 100 (Beck et al., Citation1993). The relative effectiveness of each rhizobial isolate was estimated as described by Kawaka et al. (Citation2014).
2.9. Sequencing and phylogenetic analysis of nifH symbiotic gene of potent cowpea rhizobia
The PCR-amplified products of nif gene of six selected efficient cowpea rhizobia were sequenced using automated DNA sequencer (ABI model 377, Applied Biosystems) as per the manufacturer’s instructions. The nifH primer pair [PolF (TGCGAYC-CSAARGCBGACTC)/PolR (ATSGCCATCATYTCRCCGGA)] was described previously (Poly et al. Citation2001). Polymerase chain reaction (PCR) amplification was performed in a Bio-Rad thermal cycler under the following conditions: 94 ºC for 5 minutes followed by 35 cycles of 94 ºC for 45 seconds, 60 ºC for 1 minute, 72 ºC for 2 minute with a final extension of 72 ºC for 5 minutes. The quality of PCR products were confirmed on 1.5% (w/v) agarose gel electrophoresis stained with ethidium bromide (1 mg/ml) and photographed under ultraviolet (UV) transilluminator. The same nifH primer pair was used for sequencing of the amplicons. The sequences obtained were checked for quality, aligned pair-to-pair, and were before the BLAST using Bioedit software package. The consensus sequences generated were compared with those deposited in the National Center for Biotechnology Information (NCBI) GenBank using the nucleotide BLAST (BLASTn) tool (Altschul et al. Citation1990). The nifH consensus sequences were aligned using online toll CLUSTAL-W (Thompson et al. Citation1994). Phylogenetic trees were constructed using MEGA version 7.0 (Kumar et al., Citation2016). The nifH sequences obtained in this study were deposited in the GenBank database under the accession numbers of MW218434—MW218435 and MT948182—MT948185.
2.10. Statistical analysis
All data on NN, NDM, RDM, SDM, SNC and SE were subjected to analysis of variance (ANOVA), with means compared by the Turkey’s test, at 5% probability (p < 0.01) using the GeneStat program Version 15.
3. Results
3.1. Soil characteristics from the study sites
The physio-chemical properties of soils varied across farmers’ fields in the three study sites (). The soil pH values were generally acidic and ranged from 4.9 to 6.7. The nitrogen levels in all the study sites were very low measuring 0.13%, 0.17% and 0.11% for Mumias in Kakamega, Busia and Vihiga, respectively. Potassium levels were high with a range of 1.3–1.7 cmolkg−1. Organic carbon was moderately low ranging from 1.09 to 1.71%. Available P levels were considerably low, with Vihiga and Busia Counties recording the lowest (8.7 ppm) and highest (9.5 ppm) levels, respectively. The textural classes of the study sites sandy clay-loam for Mumias and Vihiga and sandy clay for Busia.
Table 1. Chemical characteristics of soil from the three study sites in Western Kenya
3.2. Isolation, biochemical and morphological characterization of rhizobia isolates
A total of 54 isolates of rhizobia were obtained from root nodules of cowpea from three Counties in Western Kenya. The highest number of isolates (28) was from Mumias in Kakamega followed by 17 from Busia and 09 from Vihiga Counties. All the isolates were similar in appearance on YEMA media (Supplementary Figure 1). When incubated at 28 °C, 68% of the isolates were fast-growing, while 32% were slow-growing. The colonies appeared white, elevated i.e. raised or convex, circular with smooth margin, mucoid, glistening and 1–5 mm in diameter after 10 days of culture on YEMA media (Supplementary Table 1).
Upon growth on YEMA with Congo red dye, the rhizobia colonies appeared white to pale pink, elevated, gummy and shiny with entire margins. On YEMA with bromothymol blue, both fast and slow growers formed circular and raised colonies. Different isolates gave different reactions on this media classifying the isolates into three groups. Of the 54 rhizobia isolates, 28 (51.6%) turned the medium from green to blue (Supplementary Figure 1) and were classified as alkaline producers. Twenty two (40.7%) of the rhizobia isolates turned the media yellow and were classified as acid producers, while four (7.4%) had a neutral reaction.
3.3. Screening of abiotic stress tolerance of cowpea rhizobia isolates
All the rhizobia isolates were observed to have a wide range of pH profile for their growth and were able to tolerate significant growth in the pH range from 4–10.0 (). The temperature tolerance range varying from 20 to 45 °C was tested for all the isolates. All the isolates obtained from soils of the three Counties exhibited optimum growth at 25 °C and 35 °C. Rhizobia isolates showed variations in growth, below and beyond the range of temperature tested. Thirty four (62.96%) isolates were able to grow at low temperature of 20 °C. Twenty (37.04%) isolates (M3aii, M4bi, M6a, M6b, M7a, M10, M12, M13i, M14i, M14ii, B2i, B2ii, B2iii, V2, V4, V5ii, V7, V9, V10 andV11i) grew up to a temperature of 40 °C, and only 5 (0.09%) isolates (M7a, M14i, B2i, B2iii and V5ii) could grow at 45 °C ().
Table 2. Salinity, pH and temperature tolerance of the rhizobia isolates
The salt tolerance profile of the rhizobia isolates is presented in . All the isolates grew in media without NaCl and also in media supplemented with 0.1% NaCl concentration. Forty nine (90.74) isolates grew in media supplemented with 3% NaCl concentration, while only seventeen (31.48%) were able to grow at salt concentration of 5%. None of the rhizobia isolates from Busia County grew in media supplemented with 5% NaCl (). Three isolates namely V5ii, V9 and V10 (from Vihiga), while 14 isolates namely M1bi, M1bii, M3ai, M3aii, M4ai, M4ai, M4bi, M6a, M6b, M7b, M9, M10, M13ii and M14i (from Kakamega) were tolerant to 5% NaCl.
3.4. ERIC-PCR fingerprinting analysis
Forty eight rhizobia isolates were amplified using ERIC primers and produced distinct and reproducible banding patterns. The sizes of the amplified DNA bands ranged from 75–5000 bp and the total number of fragments was 1–10 depending on the isolate. The ERIC-PCR fingerprints showed that all the rhizobia isolates showed unique band profiles, indicating that these rhizobia are different isolates.
The UPGMA dendrogram constructed from the banding patterns grouped the rhizobia isolates into two clusters A and B (). The isolates in clusters A and B were each separated into two sub-clusters at a similarity level of 57% and 67%, respectively. The clusters and sub-clusters were heterogeneous, and consisted of isolates from all the three Counties. The isolates in the dendrogram were neither grouped based on the geographical regions where root nodules were collected nor by their tolerance to abiotic stresses.
Figure 1. A dendrogram of ERIC-PCR fingerprints of rhizobia isolates nodulating cowpea variety K80 in soils from Western Kenya and the reference strain CIAT 899 constructed using unweighted pair group method arithmetic mean (UPGMA) and the Jaccard’s similarity coefficient with the software NTSYSPC program version 2.10. The bacterial isolates were named using the site of collection as prefix (M = Mumias in Kakamega, B = Busia and V = Vihiga) followed by the field number and the roman numbers indicates the number of isolates from each field
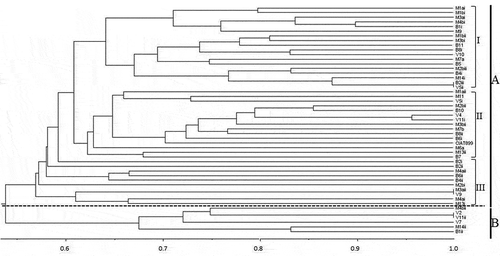
3.5. Symbiotic effectiveness of abiotic stress-tolerant rhizobia isolates
Nine isolates with different degrees of tolerance to abiotic stresses were selected including M2bii, M7b and V11i (both acid and salinity tolerant); B2ii (both acid and high temperature tolerant); and B2i, B6ii, V4, V5ii and V9i (acid, high salinity and high temperature tolerant). These isolates constituted suitable candidates which were further evaluated for their symbiotic properties. All the nine rhizobia isolates and the reference strain CIAT 899 formed root nodules with the host cowpea plants and were authenticated as root nodulating bacteria. These rhizobia isolates showed variations in host plant nodulation ranging from 2 to 94 nodules per plant. Inoculation of host cowpea plants with rhizobia isolates increased NN and NDM (). Highly significant differences (p < 0.001) were observed on NN, NDM, RDM, SDM, SNC and SE among the isolates tested.
Table 3. Symbiotic effectiveness of abiotic stress-tolerant rhizobia isolates from cowpea (variety K80) evaluated by nodule number per plant (NN), nodule dry matter (NDM), root dry matter (RDM), shoot dry matter (SDM) and total nitrogen content in shoots (SNC) at 50 days after plant emergence under greenhouse conditions
The highest NDM was obtained for plants inoculated with V4 and V11i isolates as compared to reference strain CIAT 899. The RDM values were higher than the reference strain CIAT 899 except for M2bii and V9 rhizobia isolates. The highest SDM was obtained in plants inoculated with all the isolates as compared with the reference strain CIAT 899. The SDM used as an indicator of relative effectiveness indicated that B6ii was the most effective with 179.6% (). The isolate V9i was found to be the least effective with 83.1% being lower than reference strain. The SE values for five isolates namely B6ii, V11i, V5ii, M2bii and B2i with values of 179.6, 143.4, 127.3, 123.2 and 114.8%, respectively, were higher than the reference strain CIAT 899 with a value of 109.3%. However, the SE values for isolates V4, M7b, B2ii and V9i were lower than that obtained from the chemical nitrogen control plants.
3.6. Molecular identification of the isolates
Based on the variability of symbiotic efficacy, the sequence of nif gene of the six rhizobia isolated from cowpea were sequenced and analyzed for molecular identification using NCBI BLASTn tool based on the sequence match with earlier reported sequence of type strains. Results revealed the greatest sequence similarity with genus Bradyrhizobium and Rhizobium. Three groups were identified (). Group A consisted of B6ii and M7b rhizobia isolates which showed similarity with reference strain Bradyrhizobium sp. Strain TUTMGSA126 (MK184071.1) with Bradyrhizobium elkani strain IAR16B (JQ810099.1) placed in a neighbour branch. Three rhizobia isolates (V5ii, B2ii and V9i) of group B were observed in the same clade with reference strain of Bradyrhizobium sp. CCBAU33118 (KC356324.1). Group C consisted one isolate (V11i) was placed with reference strain Rhizobium sp. GV20 (LC461109.1).
Figure 2. Maximum-likelihood phylogenetic tree based on nifH gene sequences of cowpea rhizobia isolates. The significance of each branch is indicated by the bootstrap value calculated for 1000 replicates. Scale bar indicates 5% substitution per site. GenBank accession numbers for the nucleotide sequences are given in parenthesis, B6ii (MT948182), M7b (MT948183)V5ii (MT948184), V11i (MT948185), B2ii (MW218434) and V9i (MW218435)
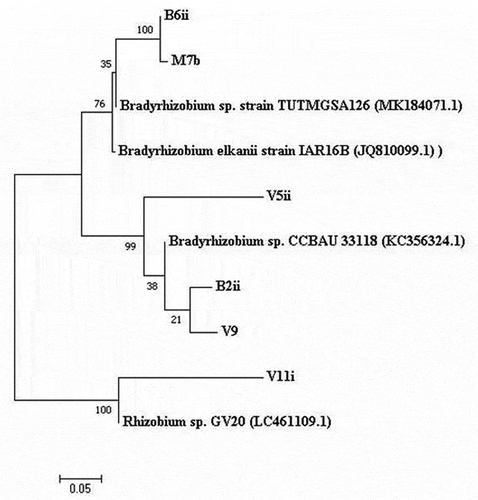
4. Discussion
In this study the pH of soils varied across the farms from 4.9–6.7 and this would be due to leaching as a result of high precipitation and the rate of fertilization. This is supported by the findings of Koskey et al. (Citation2017). Lower pH increases solubility of Al, Mn, and Fe in soil bringing about toxicity to plants by slowing or stopping root growth. Kawaka et al. (Citation2014) reported that legumes require neutral or slightly acidic soil especially when they depend on symbiotic nitrogen fixation for growth. The soils nitrogen and organic carbon levels are lower than the recommended values by Okalebo et al. (Citation2002), thus considered infertile. Soils from Vihiga had low pH of 4.9 and possibly one of the reasons for the low number of isolates obtained as low pH has a detrimental effect on the growth of most rhizobia strains. Soil pH below 5.5 and high nitrogen values inhibit nodulation and nitrogen fixation (Koskey et al., Citation2017, Citation2018).
Bacteria nodulating cowpeas have been reported to be widely distributed in Africa (Ndungu et al., Citation2018; Ondieki et al. Citation2017). In this study, a total of 56 cowpea nodulating bacteria were isolated from three Counties of Western Kenya. Growth of the isolates on YEMA media produced the standard morpho-cultural characteristics of Rhizobium species. All the isolates obtained in this study absorbed Congo red dye (white to pale pink) and showed a Gram negative reaction during incubation, which are the typical characteristics of cowpea nodulating rhizobia (Harpreet et al., Citation2012; Kawaka et al., Citation2014). All slow growing Rhizobium species are alkali producers which turn YEMA media supplemented with bromothymol blue dye from green to blue while fast growing are acid producers and turn the dye in the media yellow (Somasegaran & Hoben, Citation1985). In this study, 28 isolates turned the media blue indicating that they are slow and alkaline producers, while 22 isolates were fast and acid producers. This indicates that the test isolates represented both fast and slow growing rhizobia nodulating cowpea and this is in agreement with the findings in other studies (Costa et al., Citation2014; Kawaka et al., Citation2014; Shraddha et al., Citation2013). The bacteria symbionts of cowpea from soils of three different agroecological zones of Western Kenya exhibited marked morphological variability as evidenced by the colony size, texture, shape, colour and growth rate.
Changes in soil salinity, temperature and pH are important parameters to characterize new isolates of rhizobia. These abiotic stress parameters have been shown to affect rhizobial growth and symbiotic performance within the host plants (Koskey et al., Citation2017). The isolates in this study showed wide variation with respect to their pH, salinity and temperature requirements. The optimum pH for rhizobia growth was observed to be between 6 and 8. Majority of the isolates (77.78%) showed significant growth at pH 4 and this is in line with findings of Ali et al (Citation2009) and Wood et al. (Citation1988). These isolates were classified as fast-growing strains and are important candidates for acidic soils. This report is in agreement with findings of Boakye et al. (Citation2016) that showed rhizobia tolerant to pH as low as 4 exist and have the ability to nodulate Vigna unguiculata.
With respect to temperature tolerance, all the isolates grew optimally at 25 °C and 35 °C and these results are in agreement with the findings of Harpreet et al. (Citation2012) and Rodrigues et al. (Citation2006). Five isolates including M7a, M14i, B2i, B2iii and V5ii were able to grow at extreme temperatures (45 °C) and these isolates were high temperature tolerant can be considered as important candidates to develop inoculants as biofertilizer. Tolerance to soil salinity is complex and involves the bacteria’s ability to tolerate and adapt to the changing environmental conditions. In this study, a decrease in growth of rhizobia was observed with increasing NaCl concentrations. This observation is similar to the findings by Ali et al (Citation2009), Nagales et al. (Citation2002), and Thrall et al. (Citation2008) who reported that NaCl concentration may have negative effect on rhizobia as a result of direct toxicity and osmotic stress. Seventeen isolates (31.48%) tolerated salt concentrations of up to 5% indicating that these isolates could potentially offer a possibility for developing inoculants for cowpea production. The highest production of exopolysaccharide by the isolates tolerant to extreme abiotic stresses in this study could be an adaptive characteristic in providing protection to the rhizobia against soil salinity, temperature and pH fluctuations in the soil. Rhizobia nodulating cowpea with the ability to withstand harsh and extreme environmental conditions could be potential candidates for the development of commercial inoculants.
This study evaluated the genetic diversity of the rhizobial symbionts of cowpea from three Counties in Western Kenya, using ERIC-PCR fingerprinting. The ERIC-PCR profiles revealed significant amounts of amplicons that are variable in number and size, indicating high genetic variation among rhizobia nodulating cowpea in Kenyan soils. The high genetic diversity among the rhizobia isolates obtained from a single cowpea variety may be attributed to the promiscuous nature of cowpea plant since several strains from Rhizobium genera have the ability to nodulate this plant species. These findings support previous reports which showed high genetic diversity of rhizobia nodulating cowpea in other parts of Kenya (Mathu et al., Citation2012; Ndungu et al., Citation2018) as well as elsewhere including Ghana, Botswana, South Africa and Brazil (Amanda et al., Citation2012; Pule-Meulenberg et al., Citation2010). Furthermore, the fact that some ERIC-PCR clusters were heterogenous (comprising isolates from different geographical regions) suggests that, while the distribution of rhizobial types may be shaped by edaphic and climatic factors, some isolates probably exhibited wider adaptation and could therefore occur in contrasting environments. These findings are consistent with a report by Ndungu et al. (Citation2018), which showed that bacteria symbionts of cowpea from contrasting locations in Kenya shared closer genomic relatedness.
Inoculation of legumes with elite rhizobial strains is a common agricultural practice used to promote biological nitrogen fixation and increase crop yields in the tropics (Lindstrom et al. Citation2010). However in field conditions, inoculated strains of rhizobia are at a survival disadvantage by virtue of their low competitive ability for legume nodulation in comparison to indigenous strains. Moreover, a major advantage of the indigenous strains is their adaptability to local and diverse edapho-climatic conditions (Ligiane et al., Citation2010).
Symbiotic efficiency tests were carried out to select the best nitrogen fixing strains among the obtained abiotic stress-tolerant rhizobia isolates. The candidate isolates were obtained from three agro-ecological zones differing in soil types and pH, precipitation (rainfall) and temperature and consequently may display adaptability to environmental stresses present in those regions. The glasshouse results showed that inoculation with the selected abiotic-stress tolerant rhizobia significantly enhanced cowpea nodulation, shoot dry matter and total nitrogen in shoots. The shoot dry matter and total nitrogen content in the shoots were used to estimate the symbiotic efficiency of the rhizobia isolates (Beck et al., Citation1993). The results showed significantly high variability (p < 0.001) in the symbiotic effectiveness induced by the indigenous rhizobia of cowpea from soils in three agro-ecological zones of Western Kenya. All the isolates induced nodulation and had SE ranging from 83.17 to 179.6%. These findings suggest that indigenous isolates enhanced nitrogen fixation, which consequently increased shoot dry matter and nitrogen accumulation in the shoots. These results are in agreement with the study by Kawaka et al. (Citation2014) who reported SE ranging from 67 to 170% when common beans were inoculated with rhizobia from soils of Western Kenya.
The shoot dry matter of the legumes grown in nitrogen-free sand is a good indicator of the symbiotic effectiveness of the rhzobial symbionts (Ereso, Citation2017). It was clear from our results that all the indigenous rhizobia isolates tested were highly effective (SE > 80%). Five isolates fixed more nitrogen than the commonly used commercial reference CIAT 899. These results suggest the presence of highly effective rhizobial populations responsible for cowpea nodulation in Kenyan soils. Chidebe et al. (Citation2017) also isolated effective rhizobia from root nodules of cowpea grown in Mozambique. These reports indicate that there are effective rhizobia in Kenyan soils that can be exploited in cowpea production especially in abiotic stressed farmlands. These isolates could be developed and formulated into inoculants for cowpea in Kenya.
5. Conclusions
Rhizobia nodulating cowpeas in soils of Western Kenya are phenotypically and genetically diverse. The study has also revealed the presence of highly effective rhizobia in Kenyan soils that can be exploited for inoculants production as an alternative to the use of expensive chemical-based fertilizers. Majority of the indigenous rhizobia symbionts exhibited high nitrogen fixing efficiency than the commercial inoculants CIAT 899. The highly effective rhizobia that nodulated cowpea in this study, clearly demonstrate their potential for use in inoculants formulation.
Supplemental Material
Download MS Word (111.5 KB)Acknowledgements
The authors express their gratitude to the Department of Biochemistry, University of Nairobi for the provision of the glasshouse, laboratory space and reagents and consumables. We also thank the Department of Land Resource Management and Agricultural Technology, University of Nairobi for provision of soil chemistry laboratory space. Smallholder cowpea farmers in Kakamega, Busia and Vihiga Counties are acknowledged for providing free access to their farms. The authors also acknowledge the help of John Kimotho and Anne Owiti in laboratory analysis of samples.
Disclosure statement
The authors declare that the study was conducted in the absence of any financial or commercial relationships that could be seen as a potential conflict of interest.
Supplementary material
Supplemental data for this article can be accessed here.
Additional information
Funding
Notes on contributors
Evans N. Nyaboga
Ms. Carolyn Odori is currently pursuing a Master of Science degree in Molecular Biology and Bioinformatics at the Department of Biochemistry, Jomo Kenya University of Agriculture and Technology (JKUAT), Kenya. She works on “Phenotypic and genotypic characterization of cowpea nodulating bacteria and evaluation of the symbiotic efficiencies of strains tolerant to abiotic stresses”. Drs. Jane Ngaira, Johnson Kinyua and Evans N. Nyaboga are her supervisors. Research reported here are the results of her MSc project. Drs. Jane Ngaira and Johnson Kinyua are senior lecturers and molecular biology specialists currently working with Jomo Kenya University of Agriculture and Technology (JKUAT). Dr. Evans N. Nyaboga is a senior lecturer and molecular biologist at the Department of Biochemistry, University of Nairobi. Ms. Carolyn Odori carried out her research project at the Department of Biochemistry, University of Nairobi and Dr. Evans N. Nyaboga trained her on isolation and genetic characterization of rhizobia as well as evaluation of symbiotic effectiveness of rhizobia.
References
- Agrawal, P. K., Agrawal, S., Singh, U., Katiyar, N., & Verma, S. K. (2012). Phenotypic characterization of Rhizobia from legumes and its application as a bioinoculant. Journal of Agricultural Technology, 8(2), 681–15. http://www.ijat-aatsea.com
- Ali, S. F., Rawat, L. S., Meghvansi, M. K., & Mahna, S. K. (2009). Selection of stress-tolerant rhizobial isolates of wild legumes growing in dry regions of Rajasthan. ARPN Journal of Agricultural and Biological Sciences, 4, 1.
- Altschul, S. F., Gish, W., Miller, W., Myers, E. W., & Lipman, D. J. (1990). Basic local alignment search tool. Journal of Molecular Biology, 215(3), 403–410. doi:10.1016/s0022-2836(05)80360-2
- Amanda, A. G., Paula, D. J., Rafaela, S. A., Ligiane, A. F., Karina, B. S., & Fatima, M. (2012). Genetic and symbiotic diversity of nitrogen-fixing bacteria isolated from agricultural soils in the Western Amazon by using cowpea as the trap plant. Applied and Environmental Microbiology, 78(18), 6726–6733. https://doi.org/10.1128/AEM.01303-12
- Anderson, J. M., & Ingram, J. S. (1989). Tropical soil biology and fertility: A handbook of methods of analysis. CAB International.
- Anderson, J. M., & Ingram, J. S. (1993). Tropical soil biology and fertility: A handbook of methods (2nd ed.). CAB International.
- Aynalem, B., Tadele, T., Yelkal, B., & Seble, W. Y. (2018). Phenotypic characterization and symbiotic effectiveness test of chickpea (Cicer arietinum L.) rhizobia isolated from Dejen and Aneded Districts, East Gojjan zone, Amahara region, Ethopia. African Journal of Biotechnology, 17(23), 730–738. https://doi.org/10.5897/AJB2018.16434
- Beck, D. P., Materon, L. A., & Afandi, F. (1993). Practical rhizobium legume technology manual (Vol. 19). Technical Manual International Center for Agricultural Research in the dry areas (ICARDA).
- Boakye, E. Y., Innocent, Y. D., Seth, K. A. D., & Samuel, K. O. (2016). Characterization and diversity of rhizobia nodulating selected tree legumes in Ghana. Symbiosis, 69(2), 89–99. https://doi.org/10.1007/s13199-016-0383-1
- Bouyocous, G. J. (1962). Hydrometer method improved for making particle size analyses of soils. Agronony Journal, 53(5), 464–465. https://doi.org/10.2134/agronj1962.00021962005400050028x
- Bray, L. N., & Kutz, L. N. (1945). Determination of total organic and available forms of phosphorous in soils. Soil Science, 59(1), 39–45. https://doi.org/10.1097/00010694-194501000-00006
- Chidebe, I. N., Jaiswal, S. K., & Dakora, F. D. (2017). Distribution and phylogeny of microsymbionts associated with cowpea (Vigna unguiculata L. Walp) nodulation in three agroecological regions of Mozambique. Applied and Environmental Microbiology, 84(2), e01712–e01717. https://doi.org/10.1128/AEM.01712-17
- Costa, F. M., Schiavo, J. A., Brasil, M. S., Leite, J., Xavier, G. R., & Fernandes Jr, P. I., Jr. (2014). Phenotypic and molecular fingerprinting of fast growing rhizobia of field-grown pigeonpea from the eastern edge of the Brazilian Pantanal. Genetics and Molecular Research Journal, 13(1), 469–482. https://doi.org/10.4238/2014.January.21.16
- Ereso, T. (2017). Symbiotic effectiveness of rhizobia from chickpea (Cicer arietinum L.) and, phenotypic and symbiotic characterization of rhizobia nodulating faba bean (Vicia faba L.) from Southern Ethiopia. Research Journal of Microbiology, 7, 280–296.
- Gothwal, R., Nigam, V., Mohan, M., Sasmal, D., & Ghosh, P. (2008). Screening of nitrogen fixers from rhizospheric bacterial isolates associated with important desert plants. Applied Ecology and Environmental Research, 6(2), 101–109. https://doi.org/10.15666/aeer/0602_101109
- Harpreet, K., Poonam, S., Navprabhjot, K., & Gill, B. S. (2012). Phenotypic and biochemical characterization of Bradyrhizobium and Ensifer spp. isolated from soybean rhizosphere. Bioscience Discovery, 3(1), 40–46.
- Hussein, H. (1999). Rhizobium-legume symbioses and nitrogen fixation under severe conditions and in an arid climate. Microbiology and Molecular Biology Reviews, 63, 968–989.
- Kawaka, F., Mathews, M. D., Peter, A. O., Omwoyo, O., John, M., Newton, O., Morris, M., Alice, A., Dative, M., & John, M. (2014). Symbiotic efficiency of native rhizobia nodulating common bean (Phaseolus vulgaris L.) in soils of Western Kenya. International Scholarly Research Notes, 2014, 8. https://doi.org/10.1155/2014/258497
- Koskey, G., Simon, W. M., Ezekiel, M. N., Jacinta, M. K., Omwoyo, O., & John, M. M. (2017). Potential of native Rhizobia in enhancing nitrogen xixation and yields of climbing beans (Phaseolus vulgaris L.) in contrasting environments of Eastern Kenya. Frontiers in Plant Science, 8, 443. https://doi.org/10.3389/fpls.2017.00443
- Koskey, G., Simon, W. M., Jacinta, M. K., Omwoyo, O., John, M. M., & Ezekiel, M. N. (2018). Genetic characterization of mid-altitude climbing bean (Phaseolus vulgaris L.) varieties. Frontiers in Microbiology, 9, 968. https://doi.org/10.3389/fmicb.2018.00968
- Kumar, S., Stecher, G., & Tamura, K. (2016). MEGA7: Molecular evolutionary genetics analysis version 7.0 for bigger datasets. Molecular Biology and Evolution, 33(7), 1870–1874. https://doi.org/10.1093/molbev/msw054
- Laranjo, M., Ana, A., & Solange, O. (2014). Legume growth –promoting rhizobia. An Overview of the Mesorhizobium Genus. Microbiological Research, 169(1), 2–17. doi: 10.1016/j.micres.2013.09.012
- Lemaire, B., Oscar, D., Samson, B. M., Chimphan, C., & Stirton, C. (2015). Symbiotic diversity, specificity and distribution of Rhizobia in native legumes of the core cape subregion (South Africa). FEMS Microbiology Ecology, 91(2), 1–17. https://doi.org/10.1093/femsec/fiu024
- Ligiane, A. F., Pedro, M., Jacqueline, S. S., Karina, B. S., & Fatima, M. (2010). Diversity and efficiency of Bradyrhizobium strains isolated from soil samples collected from around Sesbania virgata roots using cowpea as trap species. Revista Brasileira De Ciencia Do Solo, 34(4), 1113–1123. https://doi.org/10.1590/S0100-06832010000400011
- Lindström, K., Murwira, M., Willems, A., & Altier, N. (2010). The biodiversity of beneficial microbe-host mutualism: the case of rhizobia: the case of rhizobia. Research in Microbiology, 161, 453–463. doi:10.1016/j.resmic.2010.05.005
- Mariam, A., Nazar, N. B., Salah, H. M., & Youness, A. A. (2015). Symbiotic and phenotypic characteristics of Rhizobia nodulating cowpea (Vigna unguiculata L. Walp) grown in arid region of Libya (Fezzan). Journal of Environmental Science and Engineering, 4, 227–239. doi: 10.17265/2162-5263/2015.05.001
- Mathu, S., Laetitia, H., Pieter, P., Matiru, V., Mwirichia, R., & Lasueur, D. (2012). Potential of indigenous bradyrhizobia versus commercial inoculants to improve cowpea (Vignaunguiculata L. walp.) and green gram (Vigna radiata L. wilczek.) yields in Kenya. Soil Science and Plant Nutrition, 58(6), 750–763. https://doi.org/10.1080/00380768.2012.741041
- Monica, N., Vidican, R., Pop, R., & Rotar, I. (2013). Stress factors affecting symbiosis activity and nitrogen fixation by Rhizobium cultured in vitro. ProEnvironment, 6, 42–45.
- Moreira, F. S., Cruz, L., De Faria, S. M., Marsh, T., Martinez-Romero, E., Pedrosa, F. O., Pitard, R. M., & Young, J. P. W. (2006). Azorhizobium doebereinerae sp. nov. microsymbiont of Sesbania virgata (Caz.) Pers. Systematics and Applied Microbiology, 29(3), 197–206. https://doi.org/10.1016/j.syapm.2005.09.004
- Nagales, J., Campos, R., Ben-Abdelkhalek, H., Olivares, J., Lluch, C., & Sanjuan, J. (2002). Rhizobium tropici genes involved in free-living salt tolerance are required for the establishment of efficient nitrogen fixing symbiosis with phaseolus vulgaris. Molecular Plant-Microbe Interactions, 15(3), 225–232. https://doi.org/10.1094/MPMI.2002.15.3.225
- Ndungu, S. M., Messer, M. M., Ziegler, D., Gamper, H. A., Meszaros, E., Thuita, M., Vanlauwe, B., Frossard, E., & Thonar, C. (2018). Cowpea (Vigna unguiculata L. Walp) hosts several widespread bradyrhizobial root nodule symbionts across contrasting agro-ecological production areas in Kenya. Agriculture, Ecosystem and Environment, 261, 161–171. https://doi.org/10.1016/j.agee.2017.12.014
- Nelson, D. W., & Sommers, L. E. (1982). Total carbon, organic carbon and organic matter. In A. L. Page, R. H. Miller, & D. R. Keeney (Eds.), Methods of soil analysis. Part 2 chemical and microbiological properties (pp. 301–312).
- Niste, M., Roxana, V., Rodica, P., & Ioana, R. (2013). Stress factors affecting symbiosis activity and nitrogen fixation by rhizobia cultured in vitro. ProEnvironment, 6, 42–45
- Okalebo, R. K., Gathua, W., & Woomer, P. L. (2002). Laboratory methods of soil analysis. A working manual, TSBR-CIAT and SACRED Africa (2nd ed.).
- Ondieki, K. D., Nyaboga, E. N., Wagacha, M. J., & Mwaura, F. B. (2017). Morphological and Genetic Diversity of Rhizobia Nodulating Cowpea (Vigna unguiculata L.) from Agricultural Soils of Lower Eastern Kenya. International Journal of Microbiology, 9. https://doi.org/10.1155/2017/8684921
- Onyango, B., Beatrice, A., Regina, N., Koech, P., Skilton, R., & Francesca, S. (2015). Morphological, genetic and symbiotic characterization of root nodule bacteria isolated from Bambara groundnuts (Vigna subterranea L. Verdc) from soils of Lake Victoria basin, Western Kenya. Journal of Applied Biology and Biotechnology, 3, 1–10.
- Poly, F., Ranjard, L., Nazaret, S., Gourbière, F., & Monrozier, L. J. (2001) Comparison of nifH gene pools in soils and soil microenvironments with contrasting properties. Applied Environmental Microbiology, 67(5), 2255–2262.
- Pule-Meulenberg, F., Belane, A. K., Krasova-Wade, T., & Dakora, F. D. (2010). Symbiotic functioning and Bradyrhizobial biodiversity of cowpea (Vigna unguiculata L. Walp.) in Africa. BMC Microbiology, 10, 89. https://doi.org/10.1186/1471-2180-10-89
- Rhitu, R., Prasanta, K., Trilocham, M., & Aqbal, S. (2012). Phenotypic and molecular characterization of indigenous rhizobia nodulating chickpea in India. Indian Journal of Experimental Biology, 50, 340–350.
- Rodrigues, C. S., Laranjo, M., & Oliveira, S. (2006). Effect of heat and pH stress in the growth of chickpea mesorhizobia. Current Microbiology, 53(1), 1–7. https://doi.org/10.1007/s00284-005-4515-8
- Rohlf, F. J. (1990). NTSYS-pc. In: Numerical Taxonomy and Multivariate Analysis System. New York: Exeter Software.
- Sessitch, A., Mehboob, I., Naveed, M., & Zahir, Z. A. (2013). Potential of rhizosphere bacteria for improving Rhizobium-Legume symbiosis. In Plant microbe symbiosis: Fundamentals and advances (pp. 305–349).
- Shraddha, B., Vyas, R. V., Shelat, H. N., & Sneha, J. M. (2013). Isolation and identification of root nodule bacteria of mung bean (Vigna radiata L.) for biofertilizer production. International Journal Research of Pure Applied Microbiology, 4, 127–133.
- Silva, F. V., Simões-Araújo, J. L., Silva, J. P., Xavier, G. R., & Rumjanek, N. G. (2012). Genetic diversity of rhizobia isolates from amazon soils using cowpea (Vigna unguiculata) as trap plant. Brazilian Journal of Microbiology, 43(2), 682–691. https://doi.org/10.1590/S1517-83822012000200033
- Simon, Z., Kelvin, M., Amare, G., & Patrick, A. N. (2014). Isolation and characterization of nitrogen fixing rhizobia from cultivated and uncultivated soils of Northern Tanzania. American Journal of Plant Science, 5(26), 4050–4067. https://doi.org/10.4236/ajps.2014.526423
- Somasegaran, P., & Hoben, H. J. (1985). Methods in legume-rhizobium technology NifTAL project and MIRCEN. Department of Agronomy, 2nd Soil Science Hawaii Institute Tropical Agriculture Human research (pp. 1–52). Univ. Hawaii Manoa.
- Somasegaran, P., & Hoben, H. J. (1994). Collecting nodules and isolating rhizobia. In Handbook of rhizobia: Methods in legume-rhizobium technology (pp. 13).
- Thompson, J. D., Higgins, D. G., & Gibson, T. J. (1994). CLUSTAL W: improving the sensitivity of progressive multiple sequence alignment through sequence weighting, position-specific gap penalties and weight matrix choice, position-specific gap penalties and weight matrix choice. Nucleic Acids Research, 22(22), 4673–4680. doi:10.1093/nar/22.22.4673
- Thrall, P. H., Bever, J. D., & Slattery, J. F. (2008). Rhizobial mediation of Acacia adaptation to soil salinity: Evidence of underlying trade-offs and tests of expected pattern. Journal of Ecology, 96(4), 746–755. https://doi.org/10.1111/j.1365-2745.2008.01381.x
- Versalovic, J., Schneider, M., De Bruijin, F. J., & Lupski, J. R. (1994). Genomic fingerprinting of bacteria using repetitive sequence-based polymerase chain reaction. Methods in Molecular and Cellular Biology, 5, 25–40.
- Vincent, J. M. (1970). A Manual for the practical study of root-nodule bacteria. In I.B.P handbook (pp. 15, 164). Blackwell Scientific.
- Wood, M., Cooper, J. E., & Bjourson, A. J. (1988). Response of Lotus rhizobia to acidity and aluminium in liquid culture and in soil. Plant Soil, 107(2), 227–231. https://doi.org/10.1007/BF02370551
- Zilli, J. E., Valisheski, R. R., Freire, F. R., & Rumjanek, M. C. P. (2004). Assessment of cowpea rhizobium diversity in Cerrado areas of northeastern Brazil. Brazilian Journal of Microbiology, 35(4), 281–287. https://doi.org/10.1590/S1517-83822004000300002