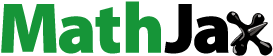
Abstract
Antibiotic and heavy metal-resistant pathogenic bacteria associated with fish from contaminated water can spread diseases. This study aims to identify antibiotics, lead, and silver nitrate-resistant bacteria in 180 fish samples from Lake Chivero using bacterial identification, disk-diffusion, and agar dilution methodologies. Of the identified 157 bacterial isolates, 46 (29.3%) were Staphylococcus, 35 (22.3%) were Escherichia coli, 26 (16.6%) were Aeromonas spp., 15 (9.6%) were Shigella spp., 13 (8.3%) were Bacillus cereus, 12 (7.6%) were Edwardsiella tarda, 7 (4.5%) were Proteus mirabilis, and 3 (1.9%) were Listeria spp. Gentamicin was the most effective, with 100% of the bacterial species susceptible, followed by neomycin (68.2%), tetracycline (56.7%), cloxacillin (52.2%), streptomycin (51%), erythromycin (32.6%), lincomycin (15.3%), and rifampicin (13.4%). For the eight taxa investigated, the multiple antibiotic resistance (MAR) index ranged from 0.50 to 0.88, with all values greater than 0.2. E. Coli and Proteus spp. had the highest MAR index values (0.88 each), followed by Bacillus, Aeromonas, and Staphylococcus (0.75 each), and Listeria, Edwardsiella, and Shigella (0.63 each). The isolated bacteria were multidrug-resistant, with varying resistance levels to lead and silver nitrate, with silver nitrate resistance rates ranging from 23.1–88.5% and lead resistance rates ranging from 30.8–69.2%. The multidrug resistance (MDR) pathogenic bacteria are found in fish from contaminated Lake Chivero, posing a health risk to humans. As a result, adequate waste disposal is required, as is multi-sectoral monitoring of antimicrobial resistance (AMR) and public education about the health risks associated with fish and fish-derived products.
PUBLIC INTEREST STATEMENT
Antibiotics and heavy metals water pollution has lately been a major cause of concern throughout the world, resulting in microbes that are resistant to a variety of medications. However, antibiotic- and heavy metal-resistant pathogenic bacteria linked with aquatic species like fish have the potential to transmit illnesses. As a result, this study examines the human pathogenic bacteria associated with Nile tilapia (Oreochromis niloticus) in a heavy metal and antibiotic-polluted environment (Lake Chivero) and assesses their resistance to lead, silver nitrate, and antibiotics. According to the findings, anthropogenic metals and antimicrobial residues have a crucial role in the development of resistance among bacteria linked with aquatic life. These drug-resistant microbes might have a significant influence on antibiotic therapeutic efficacy in aquatic organisms, humans, and other animals. To reduce the acquisition and spread of antibiotic resistance, thorough multi-sectoral monitoring, proper waste disposal, and public education are strongly advised.
1. Introduction
Fish and related by-products are essential foods for human nutrition, according to the Food and Agriculture Organization (FAO), because they provide affordable, high-quality animal protein (16–17% protein consumption), a significant amount of essential fatty acids (Omega-3 fatty acids), minerals, and vitamins (Food and Agricultural Organization [FAO], Citation2007;). On the other hand, fish are essential reservoirs and vectors for various bacterial genera, including Bacillus, Shigella, Pseudomonas, Staphylococcus, Salmonella, Vibrio, and Escherichia coli (Amande and Nwaka, Citation2013; FAO, Citation2007;). In healthy fish, bacteria from indigenous and non-indigenous sources have been identified. The presence of highly pathogenic bacteria poses a significant risk to human health, particularly to the immune-compromised, resulting from consuming raw/undercooked fish or using unsanitary handling procedures (M. Pal et al., Citation2016).
Most freshwater lakes in urban areas are contaminated with drug residues and heavy metals. Bacteria that have been exposed to different antibiotic residues and heavy metal concentrations over time have developed resistance mechanisms. Antibiotics have long been used as first-line therapy for bacterial infections, and they remain vital in modern medicine. Antimicrobial abuse (overuse/misuse) in human illness treatment and prevention, veterinary medicine, and aquaculture, on the other hand, increases the prevalence of antimicrobial resistance (Markey et al., Citation2013; Mohammadi et al., Citation2020, Citation2022). According to Gullberg et al. (Citation2011), prolonged antibiotic exposure increases selection pressure on bacterial populations even at sub-lethal antibiotic dosages. Heavy metals can be found in soil, water, and contaminants in various industries and mining operations. Water pollution caused by heavy metals has recently become a significant source of concern worldwide (Prabu, Citation2009). Furthermore, metals such as copper, arsenic, silver, mercury, and zinc have been used as antimicrobials in human and veterinary medicine (cure and prevention of post-weaning disorders; growth stimulators and diarrhea control in chickens) and disinfectants in clinical settings.
To adapt to metal and antibiotic exposure, bacteria have acquired several metals and antimicrobial resistance genes via vertical and horizontal gene transfer. Metal nanoparticles are used as therapeutic agents in human and veterinary medicine, and they are being promoted as a safe alternative to antimicrobial drugs (Bilal et al., Citation2017). Nonetheless, for decades, co-resistance (genetic linkage) of metal and antibiotic resistance determinants has been recognized (Pal et al., Citation2015; Pal et al., Citation2017; Yu et al., Citation2017). Antibiotic resistance may also occur due to co-selection via chromosomal metal resistance genes (Pal et al., Citation2015). Antimicrobial drugs and heavy metal co-selection can be aided by cross-resistance, co-regulation, biofilm formation, and co-resistance (Pal et al., Citation2017; Yu et al., Citation2017). The co-selection of resistance to metals and clinically significant antimicrobials is a potential health risk that warrants further investigation (Van Alen et al., Citation2018). Given the unprecedented levels of heavy metals, antimicrobial residues, and resistant bacteria in human-affected lakes like Lake Chivero (Magadza, Citation2003; Stepanauskas et al., Citation2005). Both informal and formal industries dump their wastes into rivers and lakes, and these aquatic environments serve as final sinks for most pollutants (Forsberg et al., Citation2012).
Previous research has found that Lake Chivero is highly contaminated because it receives raw (via sewage bursting pipes) and insufficiently treated sewage (Moyo and Mtetwa, Citation2002), most likely containing antibiotic residues. Zaranyika et al. (Citation1993) found higher concentrations of copper, lead, nickel, and cadmium than the WHO threshold limits and Zimbabwean standards. Aquatic systems were identified as hotspots for antimicrobial, heavy metal, and biocide resistance by Banquero et al. (Citation2008). Antimicrobial resistance and MDR continue to pose severe threats to humanity and economic development (Azam et al., Citation2003). According to Zhao et al. Citation2017), heavy metals, biocides, and antibiotics may react to form less toxic by-products, and the overall combination of heavy metals, biocides, and antibiotics decreases toxicity to bacteria, giving rise to tolerant bacteria. Antimicrobial and heavy metal-resistant bacteria-infected fish may pose a public health risk by exposing human consumers to antibiotic-resistant bacteria (Seiler & Berendonk, Citation2012).
Despite the growing understanding of AMR acquisition, dissemination, and control in waterways, Zimbabwe has only a few studies on bacteria’s antimicrobial and heavy metal resistance profiles of environmental and animal origin. Gwenzi et al. (Citation2018), and Gufe et al. (Citation2019) investigated the origins, behavior, and health hazards of AMR genes in wastewater, as well as the resistance profiles of bacteria isolated from fish in Lake Chivero and Mufakose, Harare. This research aimed to identify human pathogenic bacteria on Nile tilapia (Oreochromis niloticus) from Lake Chivero and determine their resistance to lead, silver nitrate, and selected antibiotics. As a result, the study’s findings are critical for advising responsible authorities on better waste disposal methods, strategies to reduce the impact of antibiotic-resistant bacteria on public health, health risks associated with handling and consumption of undercooked/raw fish from contaminated sources.
2. Materials and methods
2.1. Study area
Fish samples were collected from Lake Chivero, located approximately 32 kilometers from Harare; the coordinates are 17053ʹ46 “S and 30049ʹ6 “E. (). The Lake was created in 1952 by damming the Manyame River, primarily to supply water to Harare. It is in the Zvimba district, in Zimbabwe’s natural region I, with a catchment area of 2136 km2. It has a surface area of 26 km2, a mean depth of 9 meters, is 1300 meters above sea level, and a water capacity of 2.5 × 108 m3 (Magadza, Citation2003).
3. Sample collection
A total of 180 fish samples were collected upon the arrival of boats on the shoreline before being handled by fish vendors. These fish samples were collected three times at the fisheries at Kamba Caravan Park station, Lake Chivero (). Gillnets set up overnight at various locations around the Lake were used to catch fish. Fish samples were placed in labeled sterile zip-lock bags in an ice-packed cooler box and transported to Harare’s Central Veterinary Laboratory, Bacteriology section. The samples were kept in a cold room (4–8 °C) for 1 hour before being analyzed.
3.1. Preparation of culture
Fish flesh [gills, skin (scales, fins, and tail), muscle, and internal organs] weighing 25 g was placed in Bijoux bottles containing 0.1% peptone water. Individual samples were homogenized for 1–2 min with a homogenizer (IKA, China) at 1200 rpm and incubated at 37 °C for 18–24 h for enrichment. This was done for each of the 180 fish samples. Pipetted (100 L) aliquots of the overnight enriched homogenates were then streaked on sheep blood agar (MAST, U.K.) and MacConkey agar (MAST, UK) and incubated at 37 °C for 18–24 h. Bacteria with different colony morphologies were subcultured onto sheep blood agar plates until pure cultures of the bacterial species were obtained.
3.2. Identification of bacteria
The bacteria were identified based on their phenotypic and biochemical characteristics. Gram staining was used to determine the Gram status of the bacteria, as described by Markey et al. (Citation2013), and biochemical characterization was done as described by Holt et al. (Citation1994). To identify the bacteria, oxidase, catalase, motility, indole, citrate, lysine decarboxylase, urease, and Triple Sugar Iron (TSI) tests were performed based on the Gram status of the isolates. Furthermore, sugar fermentation tests were performed to identify the bacteria at the species level. Sugars such as glucose, sucrose, sorbitol, xylose, lactose, inositol, rhamnose, mannitol, inulin, and mannose are examples of these sugars.
3.3. Antibiotic profiling
The antibiotic susceptibility of the detected isolates was assessed using the Kirby-Bauer agar-disk diffusion technique using Mueller Hinton Agar (MHA; OXOID, UK) plates (Bauer et al., Citation1966). The antibiotics employed were erythromycin (E, 15 g), streptomycin (S, 10 g), cloxacillin (CX, 5 g), tetracycline (T.E., 30 g), and neomycin (NE, 10 g). Briefly, a standardized inoculum of 0.5 MacFarland standard was prepared by picking a few colonies from well-isolated bacterial colonies (pure culture) and putting them into 0.85% saline and emulsifying them until turbidity similar to the 0.5 McFarland standard was attained. A sterile swab was immersed in the standardized bacterial suspension, and excess fluid was removed by pressing and rotating the swab against the tube’s inner walls above the fluid.
The inoculated plates were left to stand for 3–5 min to allow the agar to absorb excess moisture. An antibiotic disc dispenser (OXOID, UK) was removed from the refrigerator and left at room temperature for 15 min. Antibiotic discs (MAST, UK) were then dispensed onto the inoculated plates using a disc dispenser. The discs were gently pressed with sterile forceps to ensure complete contact with the agar. Within 15 min of application, the plates were inverted and incubated at 37°C for 24 h (Markey et al., Citation2013). As a control, the E. coli strain ATCC 7966 T was used. Antibiotic sensitivity against bacterial isolates was determined using a calliper to measure the zone of inhibition from the back of the plate to the nearest mm. Each isolate was classified as either sensitive (S), intermediate (I), or resistant (R) to each of the antibiotics tested (Clinical and Laboratory Standard Institute, Citation2018). The multiple antibiotic resistance (MAR) index was calculated for each isolate based on the disk diffusion method results. Each bacterium’s MAR index was calculated by dividing the number of antibiotics to which the isolate was resistant by the number of antibiotics to which the isolate had been exposed. When isolates are exposed to high-risk sources of animal or human pollution, where antimicrobial use is widespread, a MAR index value greater than 0.2 is found; when antibiotics are rarely or never used, a MAR index value less than 0.2 is found. If an isolate exhibited resistance to ≥3 antibiotics from three different classes, it was labeled as multi-drug resistant (MDR).
3.4. Heavy metal resistance testing
With some modifications, the agar dilution method was used to test bacterial resistance to heavy metals, as previously described by Timoney et al. (Citation1978). After autoclaving, a stock solution of lead (Pb) and silver nitrate was added to MHA, and the final concentrations of lead and silver nitrate were modulated in the range of (100; 200; 400; 800, and 1600) g/ml. Using sterile swabs, the bacterial suspension (prepared as described in the culture preparation section) was streaked onto MHA, embedded with lead and silver nitrate concentrations. For 24 h, the plates were inverted and incubated at 37 °C. The minimum inhibitory concentration (MIC) of each metal was determined by measuring the concentration of that metal that inhibited bacterial growth in each isolate. E. coli ATCC 25922 and S. aureus ATCC 33862 were used as reference strains. Because there are no established heavy-metal resistance breakpoints, the heavy metal concentrations used in this study to differentiate metal-resistant from metal-sensitive bacteria were based on the MIC of standard strains representing both Gram-negative and Gram-positive bacteria. Tolerant isolates had MICs for both lead and silver nitrate greater than 100 g/ml. The isolates were considered resistant if their MIC values exceeded the controls, E. coli ATCC 25922 and S. aureus ATCC 33862.
3.5. Data analysis
The isolates were classified based on the results of their biochemical tests. The identified bacteria’s prevalence and sample infestation rates were expressed as percentages (%). Each isolate was classified as either sensitive (S), intermediate (I), or resistant (R) to each of the antibiotics tested (Clinical and Laboratory Standard Institute, 2018). For each antibiotic tested, resistance rates were calculated.
4. Results and discussion
4.1. Isolation, identification
One hundred and fifty-seven (157) bacterial isolates from eight genera were isolated and identified from the 180 samples. shows how the isolated bacteria were divided into four major groups based on their cell shape (morphology), gram-staining reaction, primary biochemical, and sugar fermentation tests. 62.5 % (5/8) of the isolated bacteria were identified at the species level, while 37.5 % (3/8) were identified at the genus level. 46 (29.3%) of the 157 bacterial isolates were Staphylococcus, 35 (22.3%) E. coli, 26 (16.6%) Aeromonas spp., 15 (9.6%) Shigella spp., 13 (8.3%) Bacillus cereus, 12 (7.6%) Edwardsiella tarda, 7 (4.5%) Proteus mirabilis, and 3 (1.9%) Listeria spp (). Fresh fish and related products have been identified as potential sources and vehicles for foodborne disease transmission (Pal et al., Citation2015). The current study found Staphylococcus spp., E. coli, Aeromonas spp., Shigella spp., B. cereus, E. tarda, P. mirabilis, and Listeria spp. in fish samples. These findings are consistent with those of other local researchers (Hove et al., Citation2019; Manhondo et al., 2018; Sichewo et al., Citation2013), regional researchers (Anwar et al., Citation2012; Mhango et al., Citation2010; Shinkafi and Ukwaja, Citation2010; Tsfaye et al., Citation2018; Wamala et al., Citation2018), and international researchers (Mhango (Pech et al., Citation2017). According to) Gufe et al. (Citation2020), Coliforms are common in nature and do not always indicate faecal pollution. The presence of at least one member of the Enterobacteriaceae family, specifically faecal coliforms, was found in this study’s findings. Furthermore, the current study shows that the isolated bacteria were non-indigenous species, implying that the fish were recovered from anthropized waters (Mhango et al., Citation2010). According to Moyo and Mtetwa (Citation2002), Lake Chivero has received either raw (via sewage bursting pipes) or insufficiently treated sewage.
Table 1. Different groups, primary biochemical tests conducted, and bacteria identified in O. niloticus in Lake Chivero
Table 2. Prevalence percentages of the isolated bacteria
Because Lake Chivero receives municipal sewage outflows the high prevalence of coliforms, particularly E. coli (16.6 %), can be attributed to contamination from the surrounding waters (Magadza, Citation2003; Hove et al., Citation2019). Pathogenic and potentially opportunistic bacteria of faecal origin predominate in contaminated and nutrient-rich waters (Dalu et al., Citation2011). Fish can be used as a vehicle to spread these pathogens topically or by ingesting raw or undercooked fish (Haenen et al., Citation2013; Pal et al., Citation2015). Ambient temperatures may have provided a favorable environment for the growth of coliforms, spoiling the fish in a short period. The presence of E. coli is consistent with the findings of Gufe et al. (Citation2019), who examined 36 fish samples from informal markets in Mufakose, Harare, and discovered that E. coli was the most common bacteria (44 %). The same authors concluded that the increased prevalence could be attributed to the source of the fish, the handling by fish vendors, and the water used to sprinkle the fish to keep it fresh. Though E. coli is a human commensal, certain strains of the bacterium are pathogenic and cause gastrointestinal illnesses (Gweshe et al., Citation2020; Markey et al., Citation2013). Unfortunately, the current study could not characterize E. coli to understand the various serotypes. If E. coli and other coliforms exceed the acceptable limit (less than 10 or >1x101 CFU/ml; FAO,), they pose a significant risk to human health.
The high prevalence of S. aureus (29.3 %) indicates unsanitary fish handling practices, as these species are found on human skin and in the surrounding waters. These results are slightly higher than Saito et al. (Citation2011) and Bujjamma and Padmavathi (Citation2015), who found 19.6 % and 24.47 % of all tested fish samples. Staphylococcus aureus can contaminate fish due to poor handling, putting consumers’ health at risk (Okonko et al., Citation2008) through food poisoning and life-threatening systemic conditions like osteomyelitis, septicaemia, meningitis, and pneumonia (Bukowski et al., Citation2010). Staphylococcus aureus is the most common cause of skin infections in patients and the second most common cause of bloodstream infections (Carratala et al., Citation2003). Other human opportunistic pathogens (P. mirabilis, E. tarda, B. cereus, and Listeria spp.) were identified in this study and are linked to human food poisoning manifested as diarrhea or vomiting (B. cereus), gastroenteritis (E. tarda), and human listeriosis (Listeria monocytogenes) (Cheesbrough, Citation2006). Infections of the urinary tract in young and older men (P. mirabilis). Aeromonas and E. tarda in fish samples were linked to poor water quality and high organic content in Lake Chivero (Magadza, Citation2003). As a result, this Lake poses a higher risk to aquaculture because some of the identified pathogens can cause significant fish disease, resulting in high mortalities and significant economic losses.
4.2. Antimicrobial susceptibility testing
A total of 157 bacterial isolates from eight genera were tested for sensitivity to eight (8) antibiotics from six (6) different classes. displays the antibiotic resistance rates of the identified bacteria, whereas depicts the overall bacterial susceptibility to the tested antibiotics.
Table 3. Isolated bacteria and their resistance rates
All isolates were resistant to at least one (1) of the antibiotics tested. In addition, all 157 isolates (100%) were susceptible to gentamicin. Bacterial resistance was most prevalent against Lincomycin and Rifampicin, with 79.6 % of isolates resistant to both. Although the rates of bacterial resistance to the antibiotics tested were not uniform, Listeria was an exception. It was susceptible to three antibiotics (tetracycline, gentamicin, and cloxacillin) and completely resistant to five (neomycin, streptomycin, erythromycin, lincomycin, rifampicin). Furthermore, E. coli had intriguing resistance patterns, with (>50%) resistance to five different antibiotics. Gentamicin was the most effective antibiotic, with 100 % of bacterial species sensitive (susceptible), followed by neomycin (68.2 %), tetracycline (56.7 %), cloxacillin (52.2 %), streptomycin (51 %), Erythromycin (32.6 %), lincomycin (15.3%), and rifampicin (13.4 %). Lincomycin and Rifampin had the lowest bacterial sensitivity. Because they were resistant to three antibiotics from three different classes, all the isolated bacteria were multidrug-resistant (). All of the identified genera were resistant to at least four of the antibiotics tested, and only two (25 %) were resistant to all seven antibiotics. Moreover, the MAR index of the eight tested genera ranged from 0.50 to 0.88, greater than 0.2. E. coli and Proteus spp. had the highest MAR index values (0.88 each), followed by Bacillus, Aeromonas, and Staphylococcus (0.75 each), Listeria and Shigella (0.63 each), while Edwardsiella recorded the least MAR OF 0.5 (). According to Hassan Citation1985, bacterial sensitivity to antibiotics varies with time and environment. All isolates were resistant to at least one (1) of the antibiotics tested in this study. Su et al. (Citation2011) reported that 98.5% of 203 Enterobacteriaceae isolates tested positive for resistance to at least one antibiotic. Similarly, Shah et al. Citation2012) isolated bacteria from marine and aquaculture environments and discovered similar results. This study found that all isolates were susceptible to gentamicin, consistent with other studies (Gufe et al., Citation2019; Citation2020; Gweshe et al., Citation2020; Manhondo et al., 2018). Lincomycin and Rifampicin had high percentages of resistance. Lincomycin is primarily active in veterinary medicine against gram-positive aerobic and anaerobic bacteria, but most gram-negative aerobes are resistant (McVey et al., Citation2013). This contradicts the findings of this study, which found that gram-positive bacteria (Listeria spp. and B. cereus) had higher resistance values, except S. aureus, which was moderately resistant. This is a significant finding with veterinary implications.
Antimicrobials (from humans, animals, and native bacteria) and other environmental stressors co-select for antimicrobial resistance may occur in water at lethal or sub-lethal doses, resulting in a new environment to which a bacterium must adapt (Cabello & Cabello, Citation2006). In the first case, lethal doses wipe out the susceptible population, leaving only the resistant type. Even at sub-lethal concentrations, antimicrobial resistance can occur (Gullberg et al., Citation2011). One generic effect of the resistant genes is to code for antimicrobial resistance in previously susceptible bacteria (Gwenzi et al., Citation2018).
Resistance is not limited to human-exploited environments; it occurs naturally in pristine settings (Davies and Davies, Citation2010). According to the same authors, Penicillinase, an enzyme that breaks down penicillin, was found in native bacteria in environment long before antibiotics were discovered. As a result, the current study’s high resistance rates could also be attributed to this phenomenon. Furthermore, resistance mechanisms differ between bacteria and antibiotics (Goel, 2015). Bacteria can develop antibiotic resistance by exchanging mobile genetic elements via horizontal gene transfer (Markey et al., Citation2013). According to Sidhu et al. (Citation2002), S. epidermidis serves as a reservoir for resistance genes and plasmids for the more pathogenic S. aureus. Bacteria with a broad host range can transfer and replicate genetic elements in various distantly related species (Gwenzi et al., Citation2018). According to McVey et al. (Citation2013) and Gweshe et al. (Citation2020), Shiga toxin-producing E. coli strains acquired the genes responsible for Shiga toxins from the bacterium Shigella dysenteriae, and this can transfer resistance genes to other bacteria.
According to McVey et al. (Citation2013), a biofilm is a structure of bacterial communities assembled as a protective strategy against the immune response and antibiotics. Antibiotic resistance is higher in bacteria found in biofilms than in free-living bacteria. Biofilms promote the exchange of genetic material between bacterial species within the biofilm and serve as antimicrobial barriers and reservoirs for antimicrobial-resistant bacteria (Balcázar et al., Citation2007). This, as well as other environmental stressors, could explain why E. coli had high resistance rates, as it produces a protease-mucinous complex that promotes biofilm formation (McVey et al., Citation2013). The high resistance of Listeria spp. to five antibiotics could be attributed to the small number of isolates found.
In this study, the MAR index values were relatively high, which could indicate antibiotic contamination of the environment and, as a result, is an indirect method of detecting antibiotics qualitatively because no quantification of antibiotic concentration is generally performed (Goel, 2015). These high figures indicate that the bacteria isolated came from a high-risk source where antibiotics are commonly used. It is not surprising that Lake Chivero is a contaminated hub, given its proximity to agricultural, residential, industrial, and municipal zones (Magadza, Citation2003). Bacteria may have developed resistance to a variety of antibiotics. These MAR strains may share mobilomes with non-MAR strains, making control difficult. Municipal effluents containing antibiotic residues, industrial effluents containing heavy metals, agricultural herbicides, pesticides, organic fertilizers, and antibiotic use in animal feed are just a few of the factors that cause bacteria to multi-select in order to adapt to the newly created harsh environment, resulting in widespread resistance. Prolonged bacteria exposure to these highly abused waters breeds MDR bacteria, also known as (superbugs), which are associated with decreased drug effectiveness, prolonged illnesses, high medical costs, and high mortality rates (Tanwar et al., Citation2014).
4.3. Heavy metal resistance testing
shows lead and silver nitrate resistance, ranging from 23.1 % for Bacillus spp. to 88.5 % for Aeromonas spp. The heavy metal resistance discovered could be attributed to anthropogenic heavy metal pollution from industry and mining operations, a significant issue in most cities. Among other heavy metals, lead was found in the water and tissues of Lake Chivero fish samples by Utete et al. (Citation2018). Several studies were conducted in Chivero, and these heavy metals generally inhibit microorganisms by blocking essential functional groups and interfering with membrane transport (Brock & Madigan, Citation1991; Huges and Poole, Citation1989). Furthermore, biofilm formation by resistant bacteria may be regarded as a heavy metal resistance strategy because it may limit the entry of metal ions into the bacterium (Balcázar et al., Citation2007).
Table 4. Tolerance of the isolated bacterial spp. to lead and silver nitrate
5. Conclusion
The connection between heavy metals and MDR has been underestimated; regrettably, as MDR has become a cause of morbidity and mortality in fish, animals and humans, there has been an increasing focus on explaining this co-selection association. Our data demonstrate that exposure to anthropogenic metals and antimicrobials residues may be critical to the co-selection of MDR and heavy metal resistance in bacteria, which might significantly affect the efficacy of antibiotics and treatments for bacterial infections in fish, humans and other animals. Modern agricultural practices must be upgraded as well in order to decrease antibiotic use while maintaining livestock health and welfare requirements. To minimize or eliminate the use of antibiotics in feed, empiric antibiotic administration, the use of other biocides, or the use of heavy metals in feed, improved rearing practices, proper sanitation, and improved nutrition (supplementation of eco-friendly natural strategies such as herbal preparations, probiotics, and prebiotics in livestock, or aquaculture) are necessary. The Harare and Norton municipalities and local companies should not discharge waste straight into Lake Chivero since this might endanger the health of fish, humans, animals, and the environment. Strict multi-sectoral monitoring, proper waste disposal, and public education are strongly advised to reduce AMR acquisition, dissemination, and associated consequences. Heavy metal resistance and MDR E. tarda, Aeromonas species, and other opportunistic pathogens can lead to fish death.
Disclosure statement
No potential conflict of interest was reported by the author(s).
Additional information
Funding
Notes on contributors
Claudious Gufe
Claudious Gufe, the first author, is an experienced Laboratory Technologist with a demonstrated history of working in the veterinary industry. Strong research professional with a Bsc Hononurs in Biological Sciences focused in Biological Sciences from Midlands State University and MSc in Tropical animal health, Institute of Tropical medicine, Antwerp Belgium, collaborating with University of Pretoria, South Africa. He is currently a PhD student.
Jerikias Marumure
Jerikias Marumure, the third author, a lecturer and researcher at Great Zimbabwe University. Completed his Bachelor of Science in Biological Sciences from the Bindura University of Science Education, and completed his Masters of Science in Applied Microbiology and Biotechnology from National University of Science and Technology in Zimbabwe. Currently a PhD student at University of South Africa, working on antibacterial resistance and the efficacy of antidiarrhoeal plants against cholera.
Bothwell Karembera, the second author, is biologist and a graduate of BSc honours Biological Sciences from the University of Zimbabwe.
Zakio Makuvara
Zakio Makuvara, the corresponding author, is a lecturer and researcher at Great Zimbabwe University. Completed his Bachelor of Science in Biological Sciences and Statistics from the University of Zimbabwe, and completed his Masters of Science in Applied Microbiology and Biotechnology from National University of Science and Technology in Zimbabwe. Currently a PhD student at University of South Africa, working on antimalarial resistance and the efficacy of antimalarial plants against malaria.
References
- Amande, T. J., & Nwaka, S. U. (2013). Bacterial flora of African catfish (Clarias gariepinus) harvested from ponds in Uyo, South-South Nigeria. Journal of Environmental Science, Toxicology and Food Technology, 5(3), 72–14. https://doi.org/10.9790/2402-0537276
- Anwar, N., Bayleyegn, M., & Eshetu, Y. (2012). Occurrence and distribution of bacterial pathogens of fish in the southern gulf of Lake Tana, Bahir Dar, Ethiopia. Livestock Research for Rural Development, 241, 149.
- Azam, K., Basher, M. Y., Ali, M., Asaduzzaman, M., & Hossain, M. M. (2003). Comparative study of organoleptic, microbiological, and biochemical qualities of four selected dried fish in summer and winter. Pakistan Journal of Biological Science, 6(24), 2030–2033. https://doi.org/10.3923/pjbs.2003.2030.2033
- Balcázar, J. L., Vendrell, D., de Blas, I., Ruiz-Zarzuela, I., Gironés, O., & Múzquiz, J. L. (2007). Quantitative detection of Aeromonas salmonicida in fish tissue by real-time PCR using self-quenched, fluorogenic primers. Journal of Medical Microbiology, 56(3), 323–328. https://doi.org/10.1099/jmm.0.46647-0
- Banquero, F., Martínez, J. L., & Canton, R. (2008). Antibiotics and antibiotic resistance in water environments. Current Opinion in Biotechnology, 19(3), 260–265. https://doi.org/10.1016/j.copbio.2008.05.006
- Bauer, A. W., Kirby, W. M. M., Sherris, J. C., & Turck, M. (1966). Antibiotic susceptibility testing by a standardized single disk method. American Journal of Clinical Pathology, 45(4_ts), 493–496. https://doi.org/10.1093/ajcp/45.4_ts.493
- Bilal, M., Rasheed, T., Iqbal, H. M. N., Hu, H., Wang, W., & Zhang, X. (2017). Macromolecular agents with antimicrobial potentialities: A drive to combat antimicrobial resistance. International Journal Biology Macromolecules, 103, 554–574. https://doi.org/10.1016/j.ijbiomac.2017.05.071
- Brock, T. P., & Madigan, M. T. (1991). Biology of microorganisms (6th ed.), pp. 340. Prentice.
- Bujjamma, P., & Padmavathi, P. (2015). Prevalence of Staphylococcus aureus in fish samples of local domestic fish market. International Journal of Current Microbiology and Applied Sciences, 4(5), 427–433.
- Bukowski, M., Wladyka, B., & Dubin, G. (2010). Exfoliative toxins of Staphylococcus aureus. Toxins, 2(5), 2245–2268. https://doi.org/10.3390/toxins2051148
- Cabello, F. C., & Cabello F. C. (2006). Heavy use of prophylactic antibiotics in aquaculture, a growing problem for human and animal health and for the environment. Environmental Microbiology, 8(7), 1137–1144. https://doi.org/10.1111/j.1462-2920.2006.01054.x
- Carratala, J., Roson, B., Fernandez-Sabe, N., Shaw, E., Del Rio, O., Rivera, A., & Gudiol, F. (2003). Factors associated with complications and mortality in adult patients hospitalized for infectious cellulitis. European Journal of Clinical Microbiology and Infectious Diseases, 22(3), 151–157. https://doi.org/10.1007/s10096-003-0902-x
- Cheesbrough, M. (2006). District laboratory practice in tropical countries (2nd ed.). Cambridge University Press.
- Clinical and Laboratory Standard Institute., (2018).
- Dalu, T., Barson, M., & Nhiwatiwa, T. (2011). Impact of intestinal microorganisms and protozoan parasites on drinking water quality in Harare, Zimbabwe. Journal of Water, Sanitation and Hygiene for Development, 1(3), 153–163. https://doi.org/10.2166/washdev.2011.049
- Davies, J., & Davies, D. (2010). Origins and evolution of antibiotic resistance. Microbiology and Molecular Biology Reviews, 74(3), 417–433. https://doi.org/10.1128/MMBR.00016-10
- Food and Agricultural Organization. (2007). Social issues in small-scale fisheries: How a human rights perspective can contribute to respective fisheries. In Presentation to FAO committee of fisheries no 27.
- Forsberg, K. J., Reyes, A., Wang, B., Selleck, E. M., Sommer, M. O. A., & Dantas, G. (2012). The shared antibiotic resistome of soil bacteria and human pathogens. Science, 337(6098), 1107–1111. https://doi.org/10.1126/science.1220761
- Gufe, C., Hodobo, C. T., Mbonjani, B., Majonga, O., Marumure, J., Musari, S., Jongi, G., Makaya, P. V., & Machakwa, J. (2019). Antimicrobial profiling of bacteria isolated from fish sold at informal market in Mufakose, Zimbabwe. International Journal of Microbiology, 2019, 1–7. https://doi.org/10.1155/2019/8759636
- Gufe, C., Marumure, J., Hodobo, T., Jongi, G., Chirau, D., Musari, S., Katsande, P., Mbonjani, B., Ncube, R., Museredza, R., Gadaga, B., Sibanda, Z., & Munyanyi, T. (2020). Isolation and antibiotic susceptibility profiling of enteric bacteria from homemade and industrial made meat pies sold in Harare CBD, Zimbabwe. Scholars Academic Journal of Biosciences, 8(1), 1–6. https://doi.org/10.36347/sajb.2020.v08i01.001
- Gullberg, E., Cao, S., Berg, O. G., Ilback, C., Sandegren, L., Hughes, D., & Andersson, D. I. (2011). Selection of resistant bacteria at very low antibiotic concentrations. PLoS Pathology, 7(7), e1002158. https://doi.org/10.1371/journal.ppat.1002158
- Gwenzi, W., Musiyiwa, K., & Mangori, L. (2018). Sources, behaviour, and health risks of antimicrobial resistance genes in wastewaters: A hotspot reservoir. Journal of Environmental Chemical Engineering, 8(1), 102220. https://doi.org/10.1016/j.jece.2018.02.028
- Gweshe, W. M., Muteveri, T., Gufe, C., Marumure, J., & Hodobo, T. C. (2020). Antimicrobial-resistant shiga-toxin producing Escherichia coli Isolated from ready-to-eat meat products and fermented milk sold in the formal and informal sectors in Harare, Zimbabwe. Journal of Pure Applied Microbiology, 14(2), 1157–1165. https://doi.org/10.22207/JPAM.14.2.11
- Haenen, O. L. M., Evans, J. J., & Berthe, F. (2013). Bacterial infections from aquatic species: Potential for and prevention of contact zoonoses. Revue Scientifique Et Technique, 32(2), 497–507. https://doi.org/10.20506/rst.32.2.2245
- Hassan H S. (1985). Sensitivity of Salmonella and Shigella to antibiotics and chemotherapeutic agents in Sudan. American Journal of Tropical Medicine and Hygiene, 88(4), 243–7.
- Holt, J. G., Krieg, N. R., Sneath, P. H. A., Staley, J. T., & Williams, S. T. (1994). Bergey’s manual of determinative bacteriology (9th ed.). Williams and Wilkins, Co.
- Hove, I., Gufe, C., Barson, M., Sibanda, S., & Makaya, P. V. (2019, October-November). The bacterial flora on selected organs of Oreochromis niloticus in Lake Chivero, Zimbabwe. South Asian Research Journal of Biology and Applied Biosciences, 1(3). https://doi.org/10.36346/sarjbab.2019.v01i03.003
- Huges, M. N., & Poole, R. K. (1989). Metals and microorganisms. Chapman and Hall Inc.
- Magadza, C. H. D. (2003). Lake chivero: A management case study. Lakes & Reservoirs: Research and Management, 8(2), 69–81. https://doi.org/10.1046/j.1320-5331.2003.00214.x
- Markey, B. K., Leonard, F. C., Archambault, M., Cullinane, A., & Maguire, D. (2013). Clinical veterinary microbiology (2nd ed.). Elsevier.
- McVey, S. D., Kennedy, M., & Chengappa, M. M. (2013). Veterinary microbiology (3rd ed.). Wiley-Blackwell.
- Mhango, M., Mpuchane, S. F., & Gashe, B. A. (2010). Incidence of indicator organisms, opportunistic and pathogenic bacteria in fish. African Journal of Food Agriculture, Nutrition and Development, 10(10), 4203–4215. https://doi.org/10.4314/ajfand.v10i10.62898
- Mohammadi, G., Rafiee, G., El Basuini, M. F., Van Doan, H., Ahmed, H. A., Dawood, M. A. O., & Abdel-Latif, H. M. R. (2020). Oregano (Origanum vulgare), St john’s-wort (hypericum perforatum), and lemon balm (Melissa officinalis) extracts improved the growth rate, antioxidative, and immunological responses in Nile tilapia (Oreochromis niloticus) infected with Aeromonas hydrophila. Aquaculture Reports, 18(November), 100445. https://doi.org/10.1016/j.aqrep.2020.100445
- Mohammadi, G., Hafezieh, M., Karimi, A. A., Azra, M. N., Van Doan, H., Tapingkae, W., Abdelrahman, H. A., & Dawood, M. A. O. (2022). The synergistic effects of plant polysaccharide and pediococcus acidilactici as a synbiotic additive on growth, antioxidant status, immune response, and resistance of Nile tilapia (Oreochromis niloticus) against Aeromonas hydrophila. Fish & Shellfish Immunology, 120(January), 304–313. https://doi.org/10.1016/j.fsi.2021.11.028
- Moyo, N., & Mtetwa, S. (2002). Water quality management and pollution control. In R. Hirji, P. Jonhson, P. Maro, & M. T. Chiuta (Eds.), Defining mainstream environmental sustainability in water resources management in southern africa (pp. 137–176). SADC/IUCN/SARPC/World Bank, Maseru/Harare/Washington.
- Okonko, I. O., Ogunjobi, A. A., Fajobi, E. A., Onoja, B. A., Babalola, E. T., Adedeji, A. O., & Ogun, A. (2008). Microbial studies on frozen shrimps processed in Ibadan and Lagos, Nigeria. Scientific Research and Essays, 3(11), 537–564.
- Pal, C., Bengtsson-Palme, J., Kristiansson, E., & Larsson, D. G. (2015). Co-occurrence of resistance genes to antibiotics, biocides and metals reveals novel insights into their co-selection potential. BMC Genomics, 16(1), 964. https://doi.org/10.1186/s12864-015-2153-5
- Pal, M., Ketema, A., Mulu, S., Dutta, Y., & Anberber, M. (2016). Microbial quality of fish and fish products. Beverage & Food World, 43(2), 46–49.
- Pal, C., Asiani, K., Arya, S., Rensing, C., Stekel, D. J., Larsson, D. G. J., & Hobman, J. L. (2017). Metal resistance and its association with antibiotic resistance. Advance Microbiology Physiology, 70(2017), 261–313. https://doi.org/10.1016/bs.ampbs.2017.02.001
- Pech, Z. G. H., Chavez, C. M. R., & Reynoso, F. L. (2017). Pathogenic bacteria in Oreochromis niloticus var. stirling tilapia culture. Fisheries and Aquaculture Journal, 8(2), 197.
- Prabu. (2009). Impact of heavy metal contamination of Akaki river of Ethiopia on soil and metal toxicity on cultivated vegetable crops. Electronic Journal of Environmental, 8(9), 818–827.
- Saito, E., Yoshida, N., Kawano, J., Shimizu, A., & Igimi, S. (2011). Isolation of Staphylococcus aureus from raw fish in relation to culture methods. Journal of Veterinary Medical Science, 73(3), 287–292. https://doi.org/10.1292/jvms.10-0198
- Seiler, C., & Berendonk, T. (2012). Heavy metal driven co-selection of antibiotic resistance in soil and water bodies impacted by agriculture and aquaculture. Frontiers in Microbiology, 3(December), 399. https://doi.org/10.3389/fmicb.2012.00399
- Shah S Q, Colquhoun D J, Nikuli H L, Sørum H. (2012). Prevalence of antibiotic resistance genes in the bacterial flora of integrated fish farming environments of Pakistan and Tanzania. Environ Sci Technol, 46(16), 8672–9. https://doi.org/10.1021/es3018607
- Shinkafi, S. A., & Ukwaja, V. C. (2010). Bacteria associated with fresh tilapia fish (Oreochromis niloticus) sold at Sokoto central market in Sokoto, Nigeria. Nigerian Journal of Basic and Applied Sciences, 18(2), 217–221.
- Sichewo, P. R., Gono, R. K., & Jvmn, S. (2013). Isolation and identification of pathogenic bacteria in edible fish: A case study of Fletcher dam in Gweru, Zimbabwe. International Journal of Scientific Research, 2(9), 227–269−.
- Sidhu, M. S., Heir, E., Leegaard, T., Wiger, K., & Holck, A. (2002). Frequency of disinfectant resistance genes and genetic linkage with β-lactamase transposon tn 552 among clinical staphylococci. Antimicrobial Agents Chemotherapy, 46(9), 2797–2803. https://doi.org/10.1128/AAC.46.9.2797-2803.2002
- Stepanauskas, R., Glenn, T. C., Jagoe, C. H., Tuckfield, R. C., Lindell, A. H., & McArthur, J. V. (2005). Elevated microbial tolerance to metals and antibiotics in metal-contaminated industrial environments. Environmental Science & Technology, 39(10), 3671–3678. https://doi.org/10.1021/es048468f
- Su, Y. C., & Liu, C. (2007). Vibrio parahaemolyticus: A concern of seafood safety. Food Microbiology, 24(6), 549–558. https://doi.org/10.1016/j.fm.2007.01.005
- Tanwar J, Das S, Fatima Z, Hameed S. (2014). Multidrug resistance: an emerging crisis. Interdisciplinary Perspectives on Infectious Diseases, 2014, 541340. https://doi.org/10.1155/2014/541340
- Timoney, J. F., Port, J., Giles, J., & Spanier, J. (1978). Heavy-metal and antibiotic resistance in the bacterial flora of sediments of New York bight. Applied and Environmental Microbiology, 36(3), 465–472. https://doi.org/10.1128/aem.36.3.465-472.1978
- Tsfaye, S., Kasye, M., Chane, M., Bogale, B., & Abebeagre, Z. (2018). Preliminary survey of gram-negative bacterial pathogens from commonly caught fish species (Oreochromis niloticus, Cyprinus carpio and clarias gariepinus) in Lake Hayiq, Ethiopia. Fish Aquatic Journal, 9(1), 238. https://doi.org/10.4172/2150-3508.10000238
- Utete, B., Phiri, C., Mlambo, S. S., Maringapasi, N., Muboko, N., Fregene, T. B., & Kavhu, B. (2018). Metal accumulation in two contiguous eutrophic peri-urban lakes, Chivero and Manyame, Zimbabwe. African Journal of Aquatic Science, 43(1), 1–5. https://doi.org/10.2989/16085914.2018.1429249
- Van Alen, S., Kaspar, U., Idelevich, E. A., Köck, R., & Becker, K. (2018). Increase of zinc resistance in German human derived livestock-associated MRSA between 2000 and 2014. Veterinary Microbiology, 214, 7–12. https://doi.org/10.1016/j.vetmic.2017.11.032
- Wamala, S. P., Mugimba, K. K., Mutoloki, S., Mdegela, E. Ø., Byarugaba, R., & Dk, S. H. (2018). Occurrence and antibiotic susceptibility of fish bacteria isolated from Oreochromis niloticus (Nile tilapia) and Clarias gariepinus (African catfish) in Uganda. Fisheries and Aquatic Sciences, 21(6), 1–10. https://doi.org/10.1186/s41240-017-0080-x
- Yu, Z., Gunn, L., Wall, P., & Fanning, S. (2017). Antimicrobial resistance and its association with tolerance to heavy metals in agriculture production. Food Microbiology, 64(Jun), 23–32. https://doi.org/10.1016/j.fm.2016.12.009
- Zaranyika, M. F., Mtetwa, L., Zvomuya, S., Gongoraand, G., & Mathuthu, A. S. (1993). The effect of industrial effluent and leachate from landfills on the levels of selected trace heavy metals in the waters of upper and middle Mukuvisi River in Harare, Zimbabwe. Bulletin in Chemical Society of Ethiopia, 7(1), 1–10.
- Zhao Z, Wang J, Han Y, Chen J, Liu G, Lu H, Yan B and Chen S. (2017). Nutrients, heavy metals and microbial communities co-driven distribution of antibiotic resistance genes in adjacent environment of mariculture. Environmental Pollution, 220, 909–918. https://doi.org/10.1016/j.envpol.2016.10.075