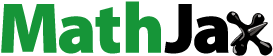
Abstract
Genetic and phenotypic parameters were estimated for various traits of Farta and their crosses with Washera sheep in northwest Ethiopia. Data collected from 2015 to 2020 on 4318 lambs were used. A number of models were evaluated, all contained the direct additive animal genetic effect and then, either the maternal genetic or permanent environment or both effects. The estimates of direct heritability for birth weight, weaning weight, pre-weaning daily gain, six-month weight, yearling weight and pre-weaning lamb survival were 0.08 ± 0.03, 0.20 ± 0.05, 0.15 ± 0.05, 0.24 ± 0.06, 0.29 ± 0.14 and 0.14 ± 0.04, respectively. The maternal heritabilities were 0.29 ± 0.05, 0.31 ± 0.05, 0.40 ± 0.05, 0.15 ± 0.57 and 0.02 ± 0.15 for weaning weight, pre-weaning daily gain, six-month weight, yearling weight and pre-weaning lamb survival, respectively. Genetic correlation between birth weight and pre-weaning lamb survival was negative (−0.36 ± 0.22), implying heavier lambs have low pre-weaning survival. For the other pairs of traits, genetic correlations ranging from 0.10 ± 0.16 between yearling weight and pre-weaning lamb survival to 0.98 ± 0.01 between weaning weight and pre-weaning daily gain were estimated. Overall, the present study showed that heritability is more likely to be overestimated if the maternal genetic and permanent environment effects are not taken into account. Parameters estimated in the current study can be used to design sheep breeding programs.
PUBLIC INTEREST STATEMENT
Indigenous sheep in Ethiopia are managed by smallholder farmers for diverse functions, such as income generation, food sources and other sociocultural benefits. Despite their diverse purposes, however, the average productivity of indigenous sheep is generally low due to the traditional husbandry practices implemented at the smallholder level. This implies developing and implementing an appropriate breeding program is vital to improving indigenous sheep productivity and enhancing their contribution to the livelihood development of smallholder farmers. As a first step to developing a breeding program, we estimated the (co)variance components and the genetic and phenotypic parameters of breeding objective traits of Ethiopian indigenous sheep. The different parameters estimated in the present study showed that a reasonable amount of genetic improvement can be achieved for Ethiopian indigenous sheep through selective breeding. The genetic and phenotypic parameters estimated in the present study can also be used as input to design breeding programs for other tropical sheep breeds.
1. Introduction
Knowledge of the genetic and phenotypic parameters of breeding objective traits is essential to designing livestock breeding programs. For instance, heritability could explain the extent of genetic influences on a particular trait while genetic correlations between traits would suggest the possible consequences of selection on one trait to the others. Normally, genetic and phenotypic parameters are characteristics of a particular population in which their estimates could vary across breeds and over time because of selection, differences in environmental factors and management practices (Jafari et al., Citation2014; Missanjo et al., Citation2013). One of the major challenges to designing and implementing breeding programs in developing countries like Ethiopia is the lack of breed-specific and up-to-date estimates of genetic and phenotypic parameters for the breeding objective traits. Because data recording system for livestock production is virtually absent, particularly in Ethiopia.
In Ethiopia, there are about 42.9 million sheep (Food and Agriculture Organization of the United Nations [FAOSTAT], Citation2020), which belong to the 14 indigenous breeds identified at the molecular level (Gizaw et al., Citation2007a). To the best of our knowledge, genetic parameter estimates are available only for three indigenous sheep breeds, namely Horro (Abegaz et al., Citation2002), Menz (Gizaw et al., Citation2007b) and Bonga (Areb et al., Citation2021). For the remaining 11 indigenous sheep breeds, however, estimates of genetic and phenotypic parameters are not available in the literature, among which are the Washera and Farta sheep breeds. Geographically, the two breeds are found in the northwest part of Ethiopia, but ecologically the latter inhabited the highland parts while the first-mentioned breed is distributed in the low- and mid-altitudes. Molecular studies conducted so far showed that the within-breed genetic diversity in Ethiopian indigenous sheep is very large (Amane et al., Citation2020; Gizaw et al., Citation2007a). In the referred sources, the Washera and Farta sheep breeds are among the indigenous sheep that possess high within-breed genetic variability showing their potential for genetic improvement through selection.
As part of a genetic improvement program for Farta sheep, two research projects were launched in 2015. The first was based on crossbreeding between Washera and Farta, while the second was pure Farta sheep breeding through community-based selection. Both projects involve a pedigree-based performance recording scheme. In an attempt to optimize the selection program Abebe et al. (Citation2020); (Citation2021)) conducted extensive studies to identify breeding objective traits and subsequently estimate economic values. However, genetic and phenotypic parameter estimates are lacking for both breeds. Therefore, the present study aimed to contribute to the optimization of sheep breeding programs in northwest Ethiopia by estimating variance components, genetic and phenotypic parameters for body weight traits measured at different ages and pre-weaning lamb survival.
2. Materials and methods
2.1. Ethics statement
The present study does not require ethical approval as it used the existing datasets, which were collected from 2015 to 2020 in the Farta sheep genetic improvement research projects funded by Debre Tabor University, Ethiopia. The data contained growth performance and pre-weaning lamb survival records with pedigree information in which all phenotypic measurements were taken without impairing the animals’ welfare.
2.2. Study areas and data source
The present study was based on the Farta sheep genetic improvement program that has been running since 2015 in the Lay Gayient and Farta districts of the south Gondar zone, located in northwest Ethiopia. Both districts represent highland agroecology. Detailed descriptions of the two districts, including the sheep production system, are available in earlier work (Abebe et al., Citation2020). The genetic improvement program in the Lay Gayient district was based on crossbreeding between Washera rams and Farta ewes, while it was based on pure Farta sheep (both rams and ewes) breeding in the Farta district. In other words, the crossbreeding and pure breeding programs run in separate areas but with similar agroecology and management practices. In both cases, ram to ewe mating ratios ranging from 1:20 to 1:30 has been applied, with year-round mating and lambing. A ram in a particular flock is allowed to serve only for one year. The feeding is largely based on grazing on natural pasture, but residues from different crops are also used as feeds, particularly during dry seasons that occur from January to June. Breeding rams throughout the year and ewes during pregnancy are commonly supplemented with local grains and improved forage hay, such as a mixture of hairy vetch (Vicia villosa) grains either with its straws or hay from forage oats. Sheep deworming against internal parasites was performed quarterly, while vaccinations against pasteurellosis and sheep pox diseases were given on annual basis.
Data collection was carried out by trained enumerators under the close supervision of researchers. All animals were ear-tagged and birth weight was recorded within 24 hours after birth, otherwise, they would be missing but will have pedigree information and measurements for the subsequent periods. The average lamb weaning age was considered as three months (90 days), but recording dates were within the range of 80 to 102 days. Similarly, recording dates for six-month and yearling weights were in the range of 165 to 200 days and 300 to 365 days, respectively. Yearling weight in the present study is defined as the live weight measured at about 10–12 months of age in which replacement rams and ewes are expected to start the reproduction cycle. For all body weight traits, measurements were taken in the morning using a weighing scale calibrated with a 200-g precision. For pre-weaning lamb survival, the phenotype was recorded as 1 if lambs were available for measurement at the weaning age or zero otherwise.
For the current study, data collected from 2015 to 2020 were used. Data from crossbred and pure breed lambs were combined to estimate genetic and phenotypic parameters because a separate estimate was not possible due to the small dataset. After data editing, the number of lambs that have at least one record was 4318 lambs (3310 crossbred and 1008 pure breeds). The pedigree data contained 8185 animals of which 140 and 3840 were sires and dams, respectively. The number of records, means and coefficient of variation for the studied traits are given in Table .
Table 1. Number of records, mean (± standard deviation) and coefficient of variation for traits
2.3. Statistical analysis for fixed effects
For the studied traits, the fixed effects to be included in the final animal mixed models were identified using the univariate general linear model (GLM) procedure of SAS software (SAS, Citation2002). The fixed effects include the sex of lambs in 2 classes (male and female), birth type in 2 classes (single and twins), the birth season in 2 classes (1 = July to December, 2 = January to June), parity in 5 classes (1 to 5), birth year in 6 classes (2015 to 2020), flock in 19 classes (1 to 19), type and year of birth interaction, season and year of birth interaction, birth season and flock interaction, and birth year and flock interaction (Table ). Crossbred and pure breed lambs were kept in separate flocks, as a result, the genotype effect was fully confounded with flock effects thus becoming non-estimable. Further efforts were made to include the genotype effect by creating contemporary groups with birth season and year but no better model was found, thus the genotype as a fixed effect was not included in the models (co)variance components estimation.
Table 2. Least square means of the studied traits adjusted for various fixed effects
3. Genetic analysis models
For all the studied traits, (co)variance components and heritability were estimated following a univariate analysis using the ASReml software package (Gilmour et al., Citation2009). With the same fixed effects, the genetic and phenotypic correlations between a pair of traits were calculated using bivariate analysis. The random effects of direct additive animal genetic, maternal genetic and permanent environment factors were evaluated for all traits using the following four linear mixed models. Fixed effects included in the genetic models for each trait are given in Table .
Table 3. Fixed effects included in the genetic analysis model for the studied traits
Where, Y is a vector of observation for the studied traits; b, a, pe, m and e are vectors of fixed, direct additive animal genetic, permanent environment, maternal genetic and random residual effects, respectively; X is an incidence matrix that relates the observations of each trait with the different fixed effects shown in Table ; Z1,Z2 and Z3 are incidence matrices relating the observations to the corresponding additive animal genetic, permanent environment and maternal genetic effects, respectively; Cov(a, m) is the covariance between additive animal genetic (a) and maternal genetic (m) effects, which was assumed to be zero.
In addition to the four linear mixed models, the logit transformed form of model 4 was applied to estimate (co)variance components and heritability for pre-weaning lamb survival. Comparison among linear models was performed using the log-likelihood ratio test (LRT). However, comparing linear and logit models using LRT is not recommended. Thus, the suitability of linear and logit models for pre-weaning lamb survival data analysis was evaluated using a 10-fold cross-validation technique in the same procedure as described by Everett-Hincks et al. (Citation2014).
4. Results and discussion
4.1. Fixed effect analysis
The least-square means (LSM) of lamb traits estimated at the various levels of fixed effects are presented in Table . The sex of lambs was a significant source of variation for the weight measured at birth, weaning and six-month of age in which male lambs were significantly heavier than females (P < 0.001). Such variations are assumed to be linked to sex-related factors, like the physiological and hormonal differences that exist between males and females. Variation in body weight between males and females has been widely reported in different sheep breeds (Gamasaee et al., Citation2010; Jafari et al., Citation2014; Taye et al., Citation2010; Yavarifard et al., Citation2015). Dam parity, which reflects the age of ewes, exerted significant influences on birth weight in which lambs born from older ewes were relatively heavier than those obtained from younger ewes. However, the effects of parity for other traits were not significant (P > 0.05).
The birth type of lambs showed significant influences in all traits except for yearling weight in which single-born lambs performed better than twins. Especially at earlier ages, maintaining the nutritional requirement of lambs is dependent on maternal nourishing potential. Although the milk production capacity of Ethiopian indigenous sheep is unknown, it is assumed to be very small and may not be adequate to support higher litter sizes. This coupled with several environmental factors, such as climate variability and lack of quality feed, the performances of lambs born as twins and higher are expected to be relatively low. The influence of birth type observed in the present study agrees with multiple literature sources (e.g., Gamasaee et al., Citation2010; Kamjoo et al., Citation2014; Vatankhah & Talebi, Citation2008). The two-way Interactions of birth season, birth year and the flock had shown significant effects in all traits except for the pre-weaning lamb survival (P < 0.01). As main effects, however, all the three factors had significant influences on the pre-weaning lamb survival (P < 0.001). Although flocks are under a genetic improvement program, the major feed source is grazing on the natural pasture in which its productivity varies with seasons and across years. It has been reported that seasonal fluctuation in the availability of feed is one of the leading constraints in livestock production in Ethiopia (Ayele et al., Citation2021; Duguma & Janssens, Citation2021).
4.2. Heritability estimates
Variance components and heritability of traits estimated using different models are shown in Table . For each trait addressed in the present study, estimates of variance components and heritability vary across models, highlighting the importance of addressing various random effects while estimating heritability and other parameters. Following the LRT evaluation, model 2 is found to be the best for lamb birth weight, while model 3 is assumed to be a better model to describe variations in weaning weight, pre-weaning daily gain and six-month weight. For yearling weight, model 4 provides separate estimates for the maternal genetic and permanent environment components, thus regarded as the best model. Following a 10-fold cross-validation evaluation, logit transformed model 4 showed a relatively higher prediction error variance (0.0045) than its linear form (0.0001). In addition, correlation in the logit model was about 0.974 while it was around 0.966 in the linear model. Considering the relatively lower prediction error variance but with nearly similar correlation estimates, the linear form of model 4 is assumed to be better than the logit for parameter estimation in pre-weaning lamb survival. Everett-Hincks et al. (Citation2014) also reported better performances of linear models than logit for genetic parameter estimation using binary data.
Table 4. Variance components, heritability estimates and log-likelihood values of traits
For those models that have the same log-likelihood (LL) values, the principle of parsimony and the conceptual insightfulness of models were taken into account. A model that contains fewer variables is considered as parsimonious, while the one that reveals the fundamental characteristics of the variables of interest is deemed conceptually insightful (Daganzo et al., Citation2012; Vandekerckhove et al., Citation2015). For instance, models 3 and 4 had the same LL values for weaning weight, pre-weaning daily gain and six-month weight but model 3 contained fewer variables, thus more preferred. For yearling weight and pre-weaning lamb survival traits, model 4 provided separate estimates for the maternal genetic and permanent environmental random effects implying that it was conceptually more insightful than the other models that contained fewer variables and the same LL values.
In all models, the additive genetic variance estimated for yearling weight was much higher than the body weights measured at earlier ages. This suggests that a higher genetic gain can be realized on body weight traits by applying selection at about a yearling age. The trend of the additive genetic variance observed in the present study agrees with the findings of Gizaw et al. (Citation2007b) and Gamasaee et al. (Citation2010). It was also observed that maternal genetic and permanent environmental variances were increased with the ages of the animal. Usually, after weaning the dependence of lambs on their dam is expected to be low and so does the maternal effect. However, maternally inherited genes and other effects during the earlier ages such as those related to milk production may have lifelong influences on the phenotype of the offspring (Rashidi et al., Citation2008). In general, it is suggested that response to selection may not only be dependent on the additive genetic variance but could also be influenced by the genetic and non-genetic effects of the dam, thus need to be taken into account during genetic evaluation (Jafari et al., Citation2014; Martínez et al., Citation2016).
The direct heritability of birth weight estimated using model 2 was about 0.08, which is very low but found within the range of literature estimates. For example, Gizaw et al. (Citation2007b) reported a direct heritability of about 0.46 for birth weight in Menz sheep using the animal genetic effect as a random variable. However, Mohammadi et al. (Citation2015) found a direct heritability of 0.09 for Lori sheep using a model similar to model 4 of the present study, while as low as 0.04 was reported by Rashidi et al. (Citation2008) for Karmani sheep in Iran. The variations in heritability estimates across literature may be attributed to the differences in breed performance and dissimilarities in the types of fixed and random effects included in the models.
The direct and maternal heritabilities of weaning weight estimated using model 3 were about 0.20 and 0.29, in the order mentioned. These estimates are in close range with the studies of Baneh et al. (Citation2010) and Behzadi et al. (Citation2007). Through an extensive review, Safari et al. (Citation2005) reported average heritability values of weaning weight that ranges from 0.18 to 0.23, which are close to the direct heritability estimated in the present study. For pre-weaning daily gain, direct and maternal heritability estimates obtained in model 3 were 0.15 and 0.31, respectively. The direct heritability of pre-weaning daily gain obtained in the present study is similar to a value of 0.15 estimated by Ehsaninia (Citation2021). In addition, Jawasreh et al. (Citation2018) obtained a direct heritability of about 0.19 for Awassi sheep in Jordan, which is close to the estimates of the current study.
The direct heritability of six-month weight estimated using model 3 was about 0.24 and is found within the range of previous findings. For instance, Baneh et al. (Citation2010) reported a heritability of 0.36 for six-month weight using a model similar to model 3 of the present study. For the same trait, direct heritability estimates of 0.16 (Mohammadi et al., Citation2015) and 0.19 (Vatankhah & Talebi, Citation2008) were also reported for the different Iranian sheep breeds. Estimates of maternal heritability obtained in the present study for the six-month weight (0.40) and the preceding traits were relatively larger than most literature estimates (e.g., Baneh et al., Citation2010; Behzadi et al., Citation2007; Kamjoo et al., Citation2014). In the current study, the maternal genetic variance is more likely to be inflated given the inefficiency of model 4 to partition the genetic and non-genetic components of maternal effects for all traits except for yearling weight and pre-weaning lamb survival (Table ). This could further be linked to the small dataset used in the present study, particularly dams with their own records were very small.
For yearling weight, the direct heritability was about 0.29 in model 4, which agrees with the study of Abegaz et al. (Citation2002), who reported direct heritability estimates ranging from 0.23 to 0.31 using various models for Horro sheep breed in Ethiopia. However, estimates higher than the present study were also found in the literature, such as 0.56 (Gizaw et al., Citation2007b) and 0.43 (Gamasaee et al., Citation2010). Such disparity among studies could imply that heritability is a population parameter that could vary across breeds due to differences in genetic and non-genetic effects. The maternal heritability of yearling weight obtained in the present study was about 0.15 but with a high standard error (0.57), indicating that the estimate is not different from zero. In the literature, maternal heritability estimates ranging from 0.08 to 0.17 were reported for yearling weight by Abegaz et al. (Citation2002), Behzadi et al. (Citation2007), and Gamasaee et al. (Citation2010).
For pre-weaning lamb survival, the direct and maternal heritability estimates were 0.14 and 0.02, respectively, but the latter has a standard error of 0.15, implying the estimate is not different from zero. In the literature, heritability estimates for lamb survival traits are generally low, for instance, about 0.03 based on a review by Safari et al. (Citation2005). The low heritability of lamb survival is an indication that the direct additive genetic effect on this trait is minimal, instead, large variations are due to the non-genetic component of the dam and other environmental effects. Thus, proper lamb management practice might be a better option to improve pre-weaning lamb survival.
5. Genetic and phenotypic correlations
The genetic and phenotypic correlations estimated between traits using a bivariate analysis are given in Table . All the correlations were positive except for the genetic correlation between birth weight and lamb survival, which was about −0.36. Although it is weak, a negative genetic correlation between these two traits implies that lambs with higher body weight have low pre-weaning survival. Similar to the present study, Everett-Hincks et al. (Citation2014) reported a genetic correlation of about −0.28 between lamb birth weight and pre-weaning lamb survival in New Zealand sheep. The same authors stated that directional selection for heavier birth weight could impair the survivability of lambs. Nel et al. (Citation2021) also found a negative genetic correlation between birth weight and the survival of lambs up to weaning ages. The low survival of heavier lambs in the present study may be linked to the large influences of environmental factors (Table ) because lambs up to weaning age are largely dependent on their dam to satisfy their nutritional requirements. Importantly, the genetic correlations of pre-weaning lamb survival with traits other than birth weight were all positive, suggesting that good lamb management practices are vital to reducing pre-weaning lamb losses.
Table 5. Genetic (above) and phenotypic (below) correlations estimated for the different traits
Genetic correlations of birth weight with weaning weight (0.15) and six-month weight (0.17) were low, even estimates were accompanied by large standard errors. However, it was moderately high (0.6) between birth weight and yearling weight. The highest genetic correlation (0.98) was estimated between weaning weight and pre-weaning daily gain, while the corresponding estimates of six-month weight with weaning weight (0.64), pre-weaning daily gain (0.70) and yearling weight (0.77) were moderately high. Literature estimates of genetic correlations between body weight traits measured at different ages vary among studies. For example, Lôbo et al. (Citation2009) reported genetic correlations of 0.89 and 0.59 between birth weight and weights at weaning and yearling ages, respectively, while Gamasaee et al. (Citation2010) found values of 0.29 and 0.14 for the same traits in the order mentioned. The latter authors also reported genetic correlations of 0.85 and 0.60 between yearling weight and the weights at weaning and six-month ages, respectively, while Behzadi et al. (Citation2007) obtained estimates of 0.92 and 0.94 for the same pair of traits appeared in the stated order. Inconsistencies in the estimates of genetic correlations between body weight traits among studies might be attributed to the variations in breed performances and the diverse environmental factors across areas. This further highlights the importance of estimating genetic parameters at the breed level, especially when designing genetic improvement programs.
In addition to the genetic relationship, correlations between traits were examined at the phenotypic level. Accordingly, the phenotypic correlations estimated in the current study ranged from 0.03 between birth weight and pre-weaning lamb survival to about 0.97 between weaning weight and pre-weaning daily gain. For most traits, the phenotypic correlation is lower than the genetic correlation. Overall, the results of the current study showed that due to the positive genetic and phenotypic correlations between body weight traits, selection on one of the traits is more likely to have a favorable correlated response on the other trait.
6. Conclusion
In the current study, the genetic and phenotypic parameters estimated for traits of Farta and their crosses with Washera sheep are in general within the range of values reported in the literature. It was also shown that models that take into account the direct additive animal genetic effect together with either the permanent environment or maternal genetic or both effects were found to be more appropriate to explain the variation in the studied traits. However, considering only the direct additive animal genetic effect was shown to inflate estimates of heritability, particularly for six-month and yearling weights. The additive genetic variance increased with the ages of the animal, implying that higher genetic gain can be obtained via selection for higher yearling weight. The maternal genetic variance was also higher in the later ages, suggesting that selection only based on the additive genetic effect may not be sufficient. Except for lamb birth weight, estimates of direct heritability for the studied traits are reasonably adequate for genetic improvement through selection. The positive genetic correlations observed between the studied traits, except between birth weight and pre-weaning lamb survival, imply that selection on one of the traits could potentially improve the other too. Overall, the genetic and phenotypic parameters obtained in the present study can be used as inputs to design breeding programs not only for Ethiopian indigenous sheep but also for other sheep breeds in developing countries where data is limited to estimate breed-specific parameters.
Disclosure statement
No potential conflict of interest was reported by the author(s).
Data availability statement
The data that support the findings of this study are available from the corresponding author, ASA, upon reasonable request.
Additional information
Funding
Notes on contributors
Abiye Shenkut Abebe
Abiye Shenkut Abebe is a PhD student in animal genetics and breeding at Bahir Dar University, Bahir Dar, Ethiopia. His research focus is designing breeding programs for small ruminants.
Kefyalew Alemayehu
Kefyalew Alemayehu (PhD) is a professor in animal genetics and breeding at Bahir Dar University. He is also a nature and wildlife management specialist. He has been advising many MSc and PhD students in animal genetics and breeding at Bahir Dar University. He is also an associate editor for the Journal of Agriculture and Environmental Sciences at Bahir Dar University.
Solomon Gizaw
Solomon Gizaw (PhD) is a senior researcher at the International Livestock Research Institute (ILRI), Addis Ababa, Ethiopia. He authored many Journal articles in animal breeding and genetics and contributed a lot to the development of the field.
Anna Maria Johansson
Anna Maria Johansson (PhD) is a researcher in animal breeding and genetics at the Swedish University of Agricultural Sciences.
References
- Abebe, A. S., Alemayehu, K., Johansson, A. M., Gizaw, S., & Clegg, S. R. (2020). Breeding practices and trait preferences of smallholder farmers for indigenous sheep in the northwest highlands of Ethiopia: Inputs to design a breeding program. PLoS ONE, 15(5), e0233040. https://doi.org/10.1371/journal.pone.0233040
- Abebe, A. S., Alemayehu, K., Gizaw, S., & Johansson, A. M. (2021). Economic values for traits of indigenous sheep managed under a low-input production system in north-western highlands of Ethiopia: Input to design of breeding programmes. Animal Science Papers and Reports, 39(4), 367–14. http://a192.fsi.pl/year-2021-vol-39-no-4
- Abegaz, S., Negussie, E., Duguma, D., & Rege, J. E. O. (2002). Genetic parameter estimates for growth traits in Horro sheep. Journal of Animal Breeding and Genetics, 119(1), 35–45 doi:10.1046/j.1439-0388.2002.00309.x.
- Amane, A., Belay, G., Nasser, Y., Kyalo, M., Dessie, T., Kebede, A., Getachew, T., Entfellner, J. D., Edea, Z., Hanotte, O., & Tarekegn, G. M. (2020). Genome-wide insights of Ethiopian indigenous sheep populations reveal the population structure related to tail morphology and phylogeography. Genes & Genomics, 42(10), 1169–1178. https://doi.org/10.1007/s13258-020-00984-y
- Areb, E., Getachew, T., Kirmani, M. A., Ssilase, T., & Haile, A. (2021). Estimation of co-variance components, genetic parameters, and genetic trends of reproductive traits in community-based breeding program of Bonga sheep in Ethiopia. Animal Bioscience, 34(9), 1451–1459. https://doi.org/10.5713/ajas.20.0413
- Ayele, J., Tolemariam, T., Beyene, A., Tadese, D. A., & Tamiru, M. (2021). Assessment of livestock feed supply and demand concerning livestock productivity in Lalo Kile district of Kellem Wollega Zone, Western Ethiopia. Heliyon, 7(10), e08177. https://doi.org/10.1016/j.heliyon.2021.e08177
- Baneh, H., Hafezian, S., Rashidi, A., Gholizadeh, M., & Rahimi, G. (2010). Estimation of genetic parameters of body weight traits in ghezel sheep. Animal Biosciences, 23(2), 149–153. https://doi.org/10.5713/ajas.2010.90266
- Behzadi, M. R. B., Shahroudi, F. E., & Van Vleck, L. D. (2007). Estimates of genetic parameters for growth traits in Kermani sheep. Journal of Animal Breeding and Genetics, 124(5), 296–301 doi:10.1111/j.1439-0388.2007.00672.x.
- Daganzo, C. F., Gayah, V. V., & Gonzales, E. J. (2012). The potential of parsimonious models for understanding large scale transportation systems and answering big picture questions. EURO Journal on Transportation and Logistics, 1(1–2), 47–65. https://doi.org/10.1007/s13676-012-0003-z
- Duguma, B., & Janssens, G. P. J. (2021). Assessment of livestock feed resources and coping strategies with dry season feed scarcity in mixed crop–livestock farming systems around the gilgel gibe catchment, Southwest Ethiopia. Sustainability, 13(19), 10713. https://doi.org/10.3390/su131910713
- Ehsaninia, J. (2021). Estimates of (co)variance components and genetic parameters for pre-weaning body weight traits and Kleiber ratio in Sangsari sheep breed. Italian Journal of Animal Science, 20(1), 918–927. https://doi.org/10.1080/1828051X.2021.1908860
- Everett-Hincks, J. M., Mathias-Davis, H. C., Greer, G. J., Auvray, B. A., & Dodds, K. G. (2014). Genetic parameters for lamb birth weight, survival and death risk traits. Journal of Animal Science, 92(7), 2885–2895 doi:10.2527/jas2013-7176.
- Food and Agriculture Organization of the United Nations. (2020). FAOSTAT statistical database. Retrieved January 25, 2022, from https://www.fao.org/faostat/en/#data/QCL
- Gamasaee, V. A., Hafezian, S. H., Ahmadi, A., Baneh, H., Farhadi, A., & Mohamadi, A. (2010). Estimation of genetic parameters for body weight at different ages in Mehraban sheep. African Journal of Biotechnology, 9(32), 5218–5223 https://www.ajol.info/index.php/ajb/article/view/92154.
- Gilmour, A. R., Cullis, B. R., & Thompson, R. (2009). ASReml update: What’s new in release 3.00. VSN International Ltd.
- Gizaw, S., Van Arendonk, J. A. M., Komen, H., Windigcd, J. J., & Hanottee, O. (2007a). Population structure, genetic variation and morphological diversity in indigenous sheep of Ethiopia. Animal Genetics, 38(6), 621–628. https://doi.org/10.1111/j.1365-2052.2007.01659.x
- Gizaw, S., Lemma, S., Komen, H., & Van Arendonk, J. A. M. (2007b). Estimates of genetic parameters and genetic trends for live weight and fleece traits in Menz sheep. Small Ruminant Research, 70(2–3), 145–153. https://doi.org/10.1016/j.smallrumres.2006.02.007
- Jafari, S., Hashemi, A., Darvishzadeh, R., & Manafiazar, G. (2014). Genetic parameters of live body weight, body measurements, greasy fleece weight, and reproduction traits in Makuie sheep breed. Spanish Journal of Agricultural Research, 12(3), 653–663 http://dx.doi.org/10.5424/sjar/2014123-4564.
- Jawasreh, K., Ismail, Z. B., Iya, F., Castañeda-Bustos, V. J., & Valencia-Posadas, M. (2018). Genetic parameter estimation for pre-weaning growth traits in Jordan Awassi sheep. Vet World, 11(2), 254–258. https://doi.org/10.14202/vetworld.2018.254-258
- Kamjoo, B., Baneh, H., Yousefi, V., Mandal, A., & Rahimi, G. (2014). Genetic parameter estimates for growth traits in Iran-Black sheep. Journal of Applied Animal Research, 42(1), 79–88. https://doi.org/10.1080/09712119.2013.822806
- Lôbo, A. M. B. O., Lôbo, R. N. B., Paiva, S. R., de Oliveira, S. M. P., & Facó, O. (2009). Genetic parameters for growth, reproductive and maternal traits in a multibreed meat sheep population. Genetics and Molecular Biology, 32(4), 761–770. https://doi.org/10.1590/S1415-47572009005000080
- Martínez, R. A., Dassonneville, R., Bejarano, D., Jimenez, A., Even, G., Mészáros, G., & Sölkner, J. (2016). Direct and maternal genetic effects on growth, reproduction, and ultrasound traits in zebu Brahman cattle in Colombia. Journal of Animal Science, 94(7), 2761–2769 doi:10.2527/jas.2016-0453.
- Missanjo, E., Imbayarwo-Chikosi, V., & Halimani, T. (2013). Estimation of genetic and phenotypic parameters for production traits and somatic cell count for jersey dairy cattle in Zimbabwe. International Scholarly Research Notices, 2013. Article ID 470585. https://doi.org/10.1155/2013/470585
- Mohammadi, K., Abdollahi-Arpanahi, R., Amraei, F., Mohamadi, E. M., & Rashidi, A. (2015). Genetic parameter estimates for growth and reproductive traits in Lori sheep. Small Ruminant Research, 131, 35–42. https://doi.org/10.1016/j.smallrumres.2015.07.029
- Nel, C. L., Swan, A. A., Dzama, K., Scholtz, A. J., & Cloete, S. W. P. (2021). Genetic parameters and trends for lamb survival following long-term divergent selection for number of lambs weaned in the Elsenburg Merino flock. Animal Production Science, 61(18), 1965–1981. https://doi.org/10.1071/AN21198
- Rashidi, A., Mokhtari, M. S., Jahanshahi, A. S., & Abadi, M. R. M. (2008). Genetic parameter estimates of pre-weaning growth traits in Kermani sheep. Small Ruminant Research, 74(1–3), 165–171. https://doi.org/10.1016/j.smallrumres.2007.06.004
- Safari, E., Fogarty, N. M., & Gilmour, A. R. (2005). A review of genetic parameter estimates for wool, growth, meat and reproduction traits in sheep. Livestock Production Science, 92(3), 271–289. https://doi.org/10.1016/j.livprodsci.2004.09.003
- SAS. (2002). Version 9.00. SAS Institute Inc.
- Taye, M., Abebe, G., Gizaw, S., Lemma, S., Mekoya, A., & Tibbo, M. (2010). Growth performances of Washera sheep under smallholder management systems in Yilmanadensa and Quarit districts, Ethiopia. Tropical Animal Health and Production, 42(4), 659–667 doi:10.1007/s11250-009-9473-x.
- Vandekerckhove, J., Matzke, D., & Wagenmakers, E. J. (2015). Model comparison and the principle of parsimony. In J. R. Busemeyer, Z. Wang, J. T. Townsend, & A. Eidels (Eds.), The Oxford handbook of computational and mathematical psychology (pp. 300–319). Oxford University Press.
- Vatankhah, M., & Talebi, M. A. (2008). Heritability estimates and correlations between production and reproductive traits in Lori-Bakhtiari sheep in Iran. South African Journal of Animal Science, 38(2), 110–118 https://www.ajol.info/index.php/sajas/article/view/4116.
- Yavarifard, R., Ghavi Hossein-Zadeh, N., & Shadparvar, A. A. (2015). Estimation of genetic parameters for reproductive traits in Mehraban sheep. Czech Journal of Animal Science, 60(6), 281–288. https://doi.org/10.17221/8242-CJAS