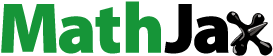
Abstract
The efficacies of ethanol and hexane crude extracts of shea nut cakes were assessed on Sitophilus zeamais Motsch and Prostephanus truncatus Horn. Phytochemical screening was performed using standard methods. The insecticidal activity of the extracts was assessed using contact toxicity, impregnated filter paper toxicity, and repellency tests. The phytochemical screening revealed the presence of alkaloids, phenols, saponins, glycosides, steroids, tannins, terpenoids, proteins, and reducing sugar in the shea nut cakes. Mortality of pests progressed with increased dosages from 53% to 100% within 24 hours after treatment at 500 to 2000 μg/mL for the extracts in contact toxicity. However, there was a significant difference (p < 0.05) in mortality between P. truncatus (100%) and S. zeamais (80%) at 2000 μg/mL with respect to shea nut cake II with successive Soxhlet extraction. Dose-dependent time of exposure was observed in impregnated filter paper toxicity for the extracts and the pests within 168 hours. All extracts of the shea nut cakes I and II exhibited some insecticidal activity with ethanolic extract of shea nut cake II exhibiting high activity against S. zeamais than P. truncatus. Whereas S. zeamais was more susceptible to the ethanolic extracts, P. truncatus was more susceptible to the hexane extracts. The results indicate that the shea nut cakes possess insecticidal activity against the two destructive maize pests and could be employed by peasant farmers to protect stored maize to enhance food security and ensure zero hunger.
PUBLIC INTEREST STATEMENT
Shea nut cakes are waste products from the processing of shea nuts into shea butter, and they are usually disposed of in the environment, becoming a menace. Some peasant farmers in the northern part of Ghana have been using the cakes as protectants for stored grains. Do the shea nut cakes possess the insecticidal activity as claimed by the farmers? If so, what phytoconstituents in them are responsible for the insecticidal activity of the cakes? Synthetic insecticides have many side effects on humans and the environment, hence there is a need to seek alternative insecticides. This article has proven that the shea nut cakes have insecticidal activity against two pests of maize, S. zeamais and P. truncatus, and that the shea nut cakes have phytochemicals that possess insecticidal activity and which can be exploited to synthesize novel insecticides that may be safer and more environmentally friendly.
1. Introduction
Maize is a very important staple food for growing populations in most parts of the world and is usually stored to provide food reserves as well as seeds for planting (Grote et al., Citation2021). In Ghana, maize ranks first in terms of area planted, and accounts for 50–60% of total cereal production, with the major producing areas located within the middle to the southern part of the country (Adu et al., Citation2014). Maize is one crop that suffers much destruction during storage in Ghana (Vowotor et al., Citation2013). Insect pests infest the grain during storage and transportation, particularly at the peasant farmer levels (Obeng-Ofori, Citation2007). Sitophilus zeamais and Prostephanus truncatus are the major destructive pests of storage maize that can cause a loss in value of maize within three months of storage, leading to a decline in quality, nutrition, and germination potential. Of an estimated total annual harvest of 250,000–300,000 tonnes of maize in Ghana, about 20–25%, is lost to S. zeamais alone (Abass et al., Citation2014; Obeng-Ofori & Amiteye, Citation2005). Mitigation of these postharvest losses requires the use of synthetic pesticides. However, its availability, accessibility, and affordability constitute a big challenge to farmers in Ghana, especially peasant farmers. Moreover, the lack of requisite knowledge on the quality and effective use of these synthetic pesticides by the farmers often causes undesirable side effects including leaving toxic residues on food, soil, and waterbodies. These have adverse effects on non-target insects and other beneficial organisms, and also contribute to the development of resistant strains of insects (Eze & Echezona, Citation2012). However, the use of locally available plant materials to protect stored grains, particularly maize against pest damage, is a common practice in traditional farm storage systems in most developing countries including Ghana (Boeke, Citation2002). The development of resistance by some pests to some of the existing synthetic pesticides and the potential health and environmental hazards have led to the search for botanicals as alternatives to synthetic pesticides (Eze & Echezona, Citation2012). Botanicals as biopesticides are readily available, affordable and biodegradable. Different plant materials commonly used to repel insect pest in the traditional farming system in Ghana as recommended by the ministry of food and agriculture includes Azadirachta indica (Neem tree), Lippia multiflora (Bush tea), Capsicum annuum (Chilli pepper), Cassia sophera (Coffee pod), Mitragyna inermis (False abura), Ocimum americana (American Basil tree), Chamaecrista nigricans (Partridge pea), Pleiocarpa mutica (Kanwene-taste bitter), Chromolaena odorata (Siam weed), Pterocarpus erinaceus (Barwood) and Vitellaria paradoxa (Shea tree; Obeng-Ofori, Citation2007). Some farmers in northern Ghana have been using shea nut cake, a waste product obtained after the extraction of shea butter from shea nut, as an insect-pest repellent on stored maize. However, no scientific validation has been performed on the shea nut cake’s insecticidal activity. Hence, the purpose of this study is to determine the chemical composition, and the toxicity and repellency effects of shea nut cake extracts against S. zeamais Motsch (Coleoptera: Curculionidae) and P. truncatus Horn (Coleoptera: Bostrichidae).
2. Materials and methods
2.1. Sample collection and identification
The shea nut cakes (initial cake and final cake) were collected at Tung-teiya Shea Butter Extraction Women Association, Jisonayili in the Sagnarigu District of Northern Ghana (latitude: 9°16′ N–9°34′ N and longitude: 0° 36′ W–0° 57′ W) on the 21st of August 2020. The initial cake is the by-product obtained after the extraction of the crude butter by the women groups and after the first press by the mechanical expeller at the industry, while the final cake is the by-product after the crude butter is heated and skimmed off by the women groups and the second extraction by the mechanical expeller at the industries (Mumeen, Citation2013). The cakes were identified and authenticated by Mr Clifford Osafo Asare at the Department of Herbal Medicine, Faculty of Pharmacy and Pharmaceutical Sciences, Kwame Nkrumah University of Science and Technology (KNUST). A voucher specimen (KNUST/HM1/F/2021/001) was deposited in the herbarium for reference purposes. The conventional shea butter (CSB) produced by local women in Tung-teiya Shea Butter Extraction Women Association was also sampled.
2.2. Chemicals and reagents
The organic solvents were of analytical grade and procured from BDH Laboratory Supplies (England) which include ethanol, hexane, ethyl acetate, and methanol. A standard reference drug was purchased from Agricore Chemical Industry (China).
2.3. Preparation of plant materials
The collected shea nut cakes were air-dried at room temperature for three weeks in the Organic laboratory at the Department of Chemistry, KNUST. The dried cakes were pulverized into a coarse powder using a milling machine.
2.4. Extraction of plant materials
Successive and non-successive Soxhlet extraction methods were employed. In the successive extraction, hexane (nonpolar) and ethanol (polar) were utilized as solvents, but only ethanol was employed as solvent in the non-successive extraction. A mass of 1 kg (1000 g) of a pulverized shea nut cake was used in each method of extraction with 2.5 L of solvent used in each case. The nonpolar components in the cake (residual butter) were completely extracted with hexane in 15 hours while the polar components were completely extracted with ethanol in 21 hours for the successive extraction. In the non-successive extraction, the components were completely extracted in 18 hours. The filtrates were evaporated to dryness using a rotary evaporator (BUCHI Rotavapor R-114) at reduced temperature (50°C) and pressure. The concentrates were further air-dried to obtain the dried total crude ethanol and hexane extracts. The percentage yield of each extract was determined as the ratio of the weight of the dried extract to the weight of the crude pulverized shea nut cake used (%w/w).
2.5. Breeding of insects
The initial stock of S. zeamais was obtained from a naturally infested stock of maize cobs at Savannah Agricultural Research Institute in Nyankpala, Tamale. For P. truncatus, the initial stock was obtained at the insectary, Department of Crops and Soil Science, Kwame Nkrumah University of Science and Technology. Both insects were reared based on the method described by Babarinde (Citation2008) at the insectary, Department of Crop and Soil science, Kwame Nkrumah University of Science and Technology Kumasi. Adult pests (100) each of S. zeamais and P. truncatus of mixed sexes were placed in separate Kilner jars containing 500 g of sterilized grains (grains were sterilized in an oven at 65 °C for an hour) under ambient laboratory conditions (at 28 ± 2 °C temperature, 70 ± 5% relative humidity and 12 L:12D photo regime) to allow oviposition. After two weeks, the parent adults were removed by sieving to enable the emergence of the same age F1 progenies that were used for the different insecticidal assays.
2.6. Phytochemical screening of extracts
The pulverized sample and the crude extracts (ethanol and hexane) obtained were screened to assess the presence of phytoconstituents using the methods described by Trease and Evans (Citation2009).
2.7. Preparation of test solutions
A stock solution of 2000 μg/mL was prepared from each extract by dissolving 0.1 g of the extract in 50 mL of 95% ethanol or n-hexane. Concentrations of 1000 μg/mL and 500 μg/mL were prepared from the stock through serial dilution. Pirimiphos-methyl was used as a reference standard drug (RSD). Pirimiphos-methyl was chosen because it is one of the most effective, recommended and standard pesticides used against pests that attack grains.
2.8. Contact toxicity test by topical application
The contact toxicity bioassay followed the earlier method described by Babarinde (Citation2008). Ten adults of S. zeamais (3–7 days old) and P. truncatus (3–7 days old) were used for this study. The insects were chilled for three minutes to immobilize them and transferred into Petri dishes lined with moist filter paper. One microliter of each prepared concentration (500, 1000, and 2000 μg/mL) of ethanol and hexane extracts were applied to the dorsal surface of the thorax of each insect using a Hamilton syringe. Pirimiphos-methyl (1 µL) was used as a reference. 95% ethanol and n-hexane were used as control. The experiment was replicated four times. Insects that did not move by responding to three prods of a blunt probe were considered dead (Suthisut et al., Citation2011). Mortality was recorded 24 hours after treatment (HAT). Percentage mortality (PM) was determined using the formula:
2.9. Repellency test
The area preference method described by Mishra and colleagues (Mishra et al., Citation2012) was used to determine the repellent action of the extracts on S. zeamais and P. truncatus. Whatman No. 1 filter papers of 9 cm diameter were cut into two-halves, with one-half to be treated with extracts and the other half left untreated (control). Various concentrations (2000, 1000, and 500 μg/mL) of both the ethanol and hexane extracts prepared were uniformly applied to half of the filter paper discs with a dropping pipette. The other halves of the filter paper discs were treated with the appropriate solvent (ethanol for ethanol extracts and hexane for hexane extracts) and air-dried to evaporate the solvent completely (Ogendo et al., Citation2008). Pirimiphos-methyl was used as a reference standard drug (RSD). Full disks of filter papers were remade, by attaching treated halves to untreated halves of the same dimensions with cello tape. Each full filter paper was placed in a petri dish, and 10 adults of S. zeamais (3–7 days old) and P. truncatus (3–7 days old) of mixed sexes were released at the Centre of each filter paper and covered. For each shea nut cake extract, there were four replicates, and the number of insects present on control (Nc) and treated (Nt) strips were counted and recorded 24 hours after treatment (HAT). Percentage repellency (PR) values were calculated as:
Where:
Nc = number of insects on control side
Nt = number of insects on the extract side
2.10. Impregnated filter paper toxicity test
Whatman No. 1 filter papers of 9 cm diameter lined in different Petri dishes of similar diameter were carefully soaked with various concentrations (2000, 1000, and 500 μg/mL) of both ethanol and hexane extracts by means of dropping pipette. The solvents were allowed to evaporate completely, and 10 adults of S. zeamais (3–7 days old) and P. truncatus (3–7 days old) were introduced into each of the Petri dishes and covered. The control filter papers were separately soaked with ethanol and hexane in different Petri dishes. Mortality counts were taken 24, 72, 120, and 168 hours after treatment (HAT). Insects were considered dead when they did not move or did not respond to three prods of a blunt probe. Percentage mortality (PM) was calculated using the formula:
2.11. Data analysis
The experiments were set up in a completely randomized design. Data were subjected to analysis of variance (ANOVA) using GraphPad Prism 8.0.2 (263) for Windows (GraphPad Software, San Diego, CA, USA), and mean responses with standard errors were calculated to show variations between replicates. Data involving percentages underwent arcsine transformation before analysis. In all cases, mean separation was done using Tukey’s multiple comparison test at 5% probability level after ANOVA had indicated a significant difference.
3. Results
3.1. Extraction of plant materials
The yields of the extract in relation to the pulverized shea nut cakes materials were calculated as
Percentages. The results are shown in .
Table 1. Percentage yield of shea nut cake extracts
The percentage yields obtained with hexane as solvent were higher than those obtained with ethanol as solvent, for both the initial and final shea nut cakes during the successive extraction. The non-successive extraction method gave a higher yield of ethanolic extract than the successive extraction method. As the cakes contained some residual shea butter, the non-polar hexane solvent extracted them, leading to the high yield of the hexane extracts. This means that the final cake (SNC II) has a higher percentage residual shea butter content (33.26%) than the initial cake (13.41%).
3.2. Phytochemical screening
The pharmacological activity of a plant largely depends on the phytochemical composition of the plant (Trease & Evans, Citation2009). Screening for the presence of phytoconstituents in pulverized shea nut cake and the ethanol extracts for successive Soxhlet extraction revealed the presence of eight phytoconstituents from the 11 primary and secondary metabolites tested.
The hexane extracts from both shea nut cakes, SNC I and SNC II, have four phytoconstituents with three being common, that is saponins, tannins, and terpenes. The conventional shea butter from the local shea butter extractors contained only saponins.
3.3. Repellency test
The repellency action of extracts of various prepared concentrations against S. zeamais and P. truncatus was performed on filter paper, and the number of insects that were repelled by the extract was recorded 24 HAT. The treatments showed a significant difference (p < 0.05) in the repellency of S. zeamais and P. truncatus. Data are reported as means of four replicates, and they are presented in ).
All the extracts of hexane and ethanol obtained through successive and non-successive extraction methods exhibited some repellency potency. The Conventional shea butter (CSB) exhibited some amount of potency, and this increased with increasing concentration. The repellency potency of the extracts and CSB against the two pests S. zeamais and P. truncatus varied.3.4. Contact toxicity by topical application
The toxicities of the extracts and conventional shea butter (CSB) were examined on the two pests of maize, that is S. zeamais and P. truncatus by direct application on their thorax. The mortalities of these pests were recorded after 24 hours of treatment (HAT), and the percentage mortalities were determined. Data are presented as means of four replicates as depicted by ()).
The various extracts exerted varying contact toxicities against the pests. SE-SCN II exhibited the highest toxicity against S. zeamais at all concentrations. NE-SCN I exhibited the least toxicity against S. zeamais at the concentration 500 μg/mL but at higher concentrations, it was NE-SCN II that exhibited the least toxicity. The Conventional shea butter exhibited high contact toxicity against both pests with P. truncatus being more susceptible than S. zeamais. The contact toxicity of CSB increased with increasing concentration. The contact toxicity of CSB against S. zeamais was higher than all the ethanolic extracts except SE-SCN II. Against P. truncatus, the CSB had a higher contact toxicity against all the ethanolic extracts whether successive or non-successive.3.5. Filter paper impregnated toxicity test
The extracts of various concentrations were impregnated into filter papers that were tested separately on the two pests enclosed in Petri dishes, and their toxicities determined. The mortalities were recorded at 24, 72, 120, and 168 HAT. Data are presented as means of four replicates. depict the mortalities of S. zeamais and P. truncatus at a concentration of 500, 1000 and 2000 μg/mL of the extracts.
Figure 3. Mortality of (a) S. zeamais and (b) P. truncatus at 500 μg/mL in impregnated filter paper toxicity test.

4. Discussions
4.1. Extraction of bioactive compounds
The purpose of the successive Soxhlet extraction was to use hexane to extract all the residual shea butter from the shea nut cake before using ethanol to extract the phytoconstituents. This is to ensure that any bioactivity determined for the ethanol extract under successive extraction could be attributable to the phytoconstituents in the shea nut cake but not shea butter. As observed, using ethanol to extract phytoconstituents directly from the initial cake yielded 10.50, but it yielded only 2.53 after shea butter had been extracted. This means that of the 10.50% yield of the ethanol extract obtained under the non-successive extraction, 7.97% is due to the presence of residual shea butter and other non-polar components in it that could not be extracted by the hexane. The ethanol extracts of the two shea nut cakes, successive and non-successive, had seven phytoconstituents in common, that is glycosides, phenols, proteins, saponins, steroids, tannins, and terpenes. However, reducing sugars were present in the initial cake but absent in the final cake and its extracts. Conversely, alkaloids were absent in the initial cake but present in the final cake and its extracts. The results obtained in this research is corroborated by earlier works reported by Mumeen (Citation2013).
Natural products from plants continue to be important in the search for novel insecticides and have straightforward applications as pest control agents (Aslan et al., Citation2004; Sparks et al., Citation2017). The class of bioactive phytoconstituents such as terpenes, phenolics, and alkaloids had long been known and used for the protection of field crops and stored commodities against insect attack (Sparks et al., Citation2017). Essential oils from plant and animal origins also have a history for their insect-pest control in traditional botanical insecticides (Arnason & Sims, Citation2012). Hence, the presence of terpenes, alkaloids, and phenols in the SNC samples could be responsible for the insecticidal activities of the SNC (Osei Akoto et al., Citation2020; Sparks et al., Citation2017).
Plant extracts or essential oils have received more attention as biopesticides (Adil et al., Citation2015; Babarinde et al., Citation2014; El-aziz et al., Citation2007; Mondal & Khalequzzaman, Citation2009; Osei Akoto et al., Citation2020; Regnault-roger & Hamraoui, Citation1993). Available research findings have proven the potential of natural products as good alternatives to synthetic pesticides in the fight against storage pest (Aslan et al., Citation2004; Babarinde et al., Citation2014; Gerwick & Sparks, Citation2014).
4.2. Repellency test
The various extracts exerted varying repellency potency against the pests. Against S. zeamais, the ethanolic extract of shea nut cake II obtained through successive extraction (SE-SCN II) exhibited the highest potency, while hexane extract of shea nut cake II (H-SCN II) exhibited the least repellency potency at higher concentrations of 1000 μg/mL and 2000 μg/mL. Again, SE-SCN II exhibited the highest potency against P. truncatus with the hexane extract of shea nut cake I (H-SCN I) exhibiting the least potency. Hence, the ethanol extract of SNC II obtained from successive Soxhlet extraction method (SE-SCN II) proved to be the most potent against both S. zeamais and P. truncatus maize pests. This extract (SE-SCN II) was obtained after hexane had been used to extract all the residual shea butter from the shea nut cake II. Toxicities and repellencies of oil components from living organisms against insect-pest of maize are well established (Tapondjou et al., Citation2005). Obeng-Ofori et al. (Citation1998) reported on the toxicity repellency of camphor, a major component of essential oil of Ocimum kilimandscharicum, against Sitophilus granarius, S. zeamais, Tribolium castaneum and P. truncatus. Important essential oils obtained from the leaves of V. paradoxa (shea tree) have been found useful in fighting insect-pest on food products (Buxton et al., Citation2019). Vegetable oil from V. paradoxa seeds has been found to have insecticidal properties against D. maculatus infestation (Ogban, Citation2019) and was utilized to protect dried fish, and this corroborates the results of this study on the insecticidal property of CSB against S. zeamais and P. truncatus. The repellency results in Figure (a and b) indicate that the response of the two pests to the extracts was dosage-dependent within the 24 hours after treatment. In each treatment of the insect pests, except the control, there was a significant difference (p < 0.05) in repellency of the dosages as high percentage repellencies were recorded at the highest concentrations (2000 μg/mL) of each extract. The reference standard drug, Pirimiphos-methyl, gave 100% repellency towards each insect within 12 HAT. The highest repellency action was exhibited by SE-SNC II against S. zeamais and P. truncatus. The extracts showed different levels of repellencies, and this may be attributed to the differences in the volatile bioactive components needed for repelling insects. These volatile components did not escape because the repellency chambers were closed (Babarinde et al., Citation2014) leading to high repellence of insects. Also, the percentage repellencies of extracts recorded against P. truncatus follow a similar trend, except that there are less significant differences in repellencies observed. The highest percentage repellency against P. truncatus was 73.33, which is less than 86.67 for S. zeamais at 2000 μg/mL. Hence, S. zeamais was more susceptible to the repellency potency of the extracts than P. truncatus. Generally, the extracts exerted significant levels of repellencies towards S. zeamais than P. truncatus. This can be attributed to the different levels of stimulation of respiration in the insects by the bioactive components in the extracts based on the insect’s morphological differences. Phytochemicals characterized by the presence of triterpenes, long chain double bonds, flanking hydroxyls, acetoxyls and carbonyls are mostly biologically active against insects (Aslan et al., Citation2004; Babarinde & Adeyemo, Citation2010; Black et al., Citation1994). The presence of some phytoconstituents including steroids, terpenes and tannins in the ethanolic extracts of SNC may be responsible for the significant repellencies of these extracts against the tested insects (Sparks et al., Citation2017). In general, S. zeamais can be said to be more susceptible to the repellency potency of the extracts than P. truncatus.
4.3. Contact toxicity test
To exert activity in contact toxicity, insecticide molecules have to enter the body and cross several barriers before arriving at their target site(s) of action (Smagghe & Tirry, Citation2001). However, the physiological responses of each insect species to the same crude plant extract were not the same (Regnault-roger & Hamraoui, Citation1993). The extracts could diffuse more into the target site in the body of P. truncatus than S. zeamais causing higher toxicity in it. The mechanisms of toxicities of terpenoids such as pulegone, linalool and 1,8 cineole against weevils are based on their reversible competitive inhibition of acetylcholinesterase by apparently binding the hydrophobic site of the enzyme’s active center (Kordali et al., Citation2006; Ryan & Byrne, Citation1988). The bioactive components in the SNC could have exhibited a similar mechanism of action towards the two pests, especially P. truncatus. Interestingly, the extracts of shea nut cake II for both successive and non-successive extraction methods (SE-SCN II and NE-SCN II) showed the presence of the same phytochemicals (), but the extract from successive extraction exhibited the highest contact toxicity against S. zeamais, whereas the extract from the non-successive extraction exhibited the least at higher concentrations. This means that the differences in the contact toxicity are not attributable to the presence of the phytochemicals only but may also be due to factors such as difference in the actual concentrations of the phytochemicals in the extracts, phytochemicals in SE-SCN II acting synergistically to enhance activity, and presence of residual shea butter in NE-SCN II. S. zeamais is more susceptible to the ethanol extract obtained after the removal of residual shea butter (SE-SCN II). This clearly indicates that the potency of SE-SCN II is not attributable to the presence of residual shea butter. Hence, the type of extraction method employed has a significant impact on the toxicity of the extract obtained. The hexane extracts for both shea nut cakes I and II (H-SCN I and H-SCN II) exhibited the highest contact toxicity against P. truncatus at the concentrations of 500 μg/mL and 1000 μg/mL, with the hexane extract of shea nut I (H-SCN I) exhibiting the highest toxicity against P. truncatus at 2000 μg/mL. This strong activity may be due to the presence of the phytochemicals saponins, tannins and terpenes as well as residual shea butter in the extracts. Whereas residual shea butter reduces repellency potency against the pests, it increases contact toxicity against the pests. Hence, P. truncatus is more susceptible to the non-polar extracts than the polar extracts. In general, P. truncatus can be said to be significantly more susceptible to the contact toxicity of the extracts than S. zeamais. However, the hexane extracts of both shea nut cakes I and II, H-SCN I and H-SCN II, which had residual shea butter in addition to important phytochemicals, had greater toxicities against P. truncatus than the CSB at lower concentrations. The CSB showed the presence of only saponins. The oily and non-volatile nature of the shea butter seems to play a vital role in contact toxicity. Of the two shea nut cakes, the ethanol extract of shea nut cake II (SE-SCN II) was found to have a higher contact toxicity than the ethanol extracts of shea nut cake I (SE-SCN I) at all concentration levels against both S. zeamais and P. truncatus. Hence, the polar components of the extracts of SNC II were more active than those of SNC I. The difference could be due to the absence of alkaloids in the SE-SCN I.
Table 2. Phytochemical screening of shea nut cate (SNC) and the extracts
4.4. Filter paper impregnated toxicity test
The extracts with residual oil components (H-NSC I, H-NSC II) and CSB generally showed less significant or non-mortalities within the first 24 HAT compared to the other extracts in almost all the dosages prepared. This can be attributed to the different phytoconstituents in the extracts and the mode of action of the extracts in the impregnated filter toxicity test. The ethanolic extracts of both SNC I and II, which were obtained from successive Soxhlet extraction (SE-SNC I and SE-SNC II), exhibited the highest potency against both S. zeamais and P. truncatus at all dosage levels. The potencies of the two extracts SNC 1 and SNC II varied with dosage and time of application. Hence, the two ethanolic extracts obtained by successive Soxhlet extraction and containing many phytoconstituents exhibited the highest toxicity against both S. zeamais and P. truncatus in the filter paper impregnated toxicity test. The SNC extracts obtained with hexane as solvents (H-SNC I and H-SNC II) exhibited varying toxicities that were generally lower than those obtained with the ethanolic extracts. Their toxicities varied with dosage and time of application. The toxic effect exerted by a certain quantity of a chemical depends on its intrinsic reactivity with the target site(s) of action and on the time-course of its concentration at the target site (Smagghe & Tirry, Citation2001). The time-course for the extract’s concentration in S. zeamais could be lesser than that in P. truncatus leading to high mortalities in S. zeamais. The complexity of the chemical composition of essential oils or extracts might lead to complex activity, probably with synergism or antagonism phenomena at the site of action (Regnault-roger & Hamraoui, Citation1993). Plant essential oils have been found to interfere with basic metabolic, biochemical, physiological and behavioral functions of insects. They either function via the neurotoxic mode of action and interference with the neuromodulator octopamine (E. E. Enan, Citation2005) or GABA-gated chloride channels (Citation2012; Priestley et al., Citation2003). Whereas some monoterpenes bind to octopamine receptors (E. Enan, Citation2001), others bind to acetylcholinesterase (E. E. Enan, Citation2005). The terpenes found in all the SNC extracts in this study may be exerting their insecticidal activity via one or more of the aforementioned modes of action.
Researchers are still in dispute as to the exact physiological mechanism of action of plant oils in causing mortality to insects when they are exposed to these oils. Some intimate that the oils cause death of the insect by interfering in their normal respiration leading to suffocation (Don-Pedro, Citation1989), while others have indicated that the oils have no effect on mortality or longevity (Singh et al., Citation1978). The toxicity of CSB in this study is more likely due to interference in normal respiration leading to suffocation since the CSB contained only one phytoconstituent and yet exhibited higher potency in destroying P. truncatus than the SNC extracts which contained more phytochemicals. Again, the toxicity of CSB was less than the SNC extracts in the repellency test since there was no direct contact of the CSB with the insects to cause greater mortalities. The mechanism of action involved in the toxicity by topical application and filter paper impregnated toxicity tests are dissimilar since P. truncatus was far more susceptible to the extracts with residual shea butter (H-SNC I and H-SNC II) in toxicity by topical application at all dosages than S. zeamais which is rather more susceptible to these extracts in the filter paper impregnated toxicity test. The insecticidal properties of SNC extracts clearly suggest and confirm the insecticidal potential of SNC as an alternative pest control measure.
5. Conclusion
The extracts of SNC showed remarkable insecticidal potency against S. zeamais and P. truncatus. Both tested insects were susceptible to all the SNC extracts, but P. truncatus was more susceptible to residual oil-containing extracts in the toxicity by topical application. S. zeamais was more susceptible to the ethanolic extracts of the shea nut cakes in the repellency and filter paper impregnated toxicity test. The type of extraction method employed has an effect on the insecticidal activity of the extract. The results authenticate the use of shea nut cake by some local farmers for the protection of their stored maize. The SNC contains promising bioactive phytocomponents that possess insecticidal properties, and these could serve as lead compounds for the development of the next generation of insecticides. Further studies are ongoing in our laboratory towards isolation, characterization, identification, determination of biological activities, and structural elucidation of the isolates from the shea nut cake.
Acknowledgements
The authors acknowledge Mr Kwabena Yaw James of the Savannah Agricultural Research Institute in Nyankpala, Tamale, and Mrs Josephine Mends Asante at the insectary, Department of Crop and Soil Science, KNUST-Kumasi, for providing the pests and technical support.
The authors are grateful to the Department of Chemistry and the Chemical Ecology Laboratory KNUST-Kumasi for the use of their facilities for this study.
Disclosure statement
No potential conflict of interest was reported by the author(s).
Additional information
Funding
Notes on contributors
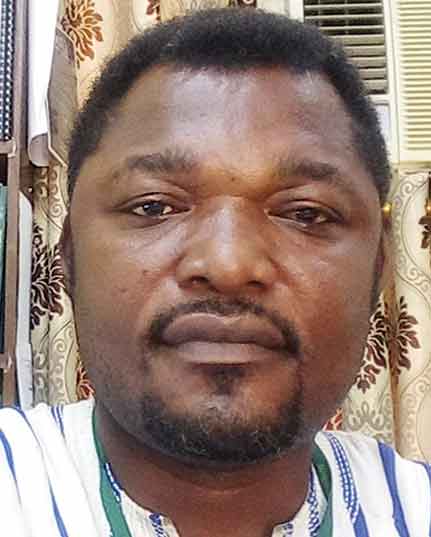
Akwasi Acheampong
Akwasi Acheampong is a Professor in Chemistry with research interests covering Organic and Natural Products and Chemical Ecology. He is in charge of the Chemical Ecology Laboratory in the Department of Chemistry of KNUST. He is excited about unearthing biologically active compounds from nature that may serve as lead compounds for the development of novel drugs to address the health challenges of humanity, identifying semiochemicals and phytochemicals to protect crops from herbivory feeding and stored grains from pest attacks.
Abdullai Alhassan Naazo
Abdullai Alhassan Naazo is an MPhil graduate from the Department of Chemistry and a member of the Chemical Ecology laboratory.
Jonathan Osei-Owusu
Dr. Jonathan Osei-Owusu is a Chemical Ecologist and a lecturer at the University of Environmental and Sustainable Development, Somanya, Ghana.
Emmanuel Quartey
Emmanuel Quartey and Abdul Malik Abdul Fatawu are members of the Chemical Ecology laboratory engaged in research.
References
- Abass, A. B., Ndunguru, G., Mamiro, P., Alenkhe, B., Mlingi, N., & Bekunda, M. (2014). Post-harvest food losses in a maize-based farming system of semi-arid Savannah area of Tanzania. Journal of Stored Products Research, 57, 49–17. https://doi.org/10.1016/j.jspr.2013.12.004
- Adil, B., Tarik, A., Kribii, A., Ounine, K., Aterials, M., & Ethods, M. (2015). The study of the insecticidal effect of Nigella sativa essential oil against Tuta absoluta larvae. International Journal of Engineering Science Technologies, 4(10), 88–90.
- Adu, G. B., Abdulai, M. S., Alidu, H., Nustugah, S. K., Buah, S. S., Kombiok, J. M., … Etwire, P. M. (2014). Practices for maize in Ghana. AGRA/CSIR.
- Arnason, J. T., & Sims, S. R. (2012). Natural products from plants as insecticides. Encyclopedia of Life Support Systems (EOLSS). , 1–8. http://www.eolss.net/sample-chapters/c06/e6-151-13.pdf
- Aslan, I., Özbek, H., Çalmaşur, Ö., & Şahin, F. (2004). Toxicity of essential oil vapours to two greenhouse pests, Tetranychus urticae Koch and Bemisia tabaci Genn. Industrial Crops and Products, 19(2), 167–173. https://doi.org/10.1016/j.indcrop.2003.09.003
- Babarinde, S. A. (2008). Comparative bioactivity of three Khaya species (Meliaceae) against Callosobruchus maculatus Fabricius (Coleoptera : Bruchidae). Journal of Entomological Research, 10(1), 27–35. https://doi.org/10.1080/03235400802246952
- Babarinde, S. A., & Adeyemo, Y. A. (2010). Archives of phytopathology and plant protection toxic and repellent properties of Xylopia aethiopica (Dunal) A . richard on Tribolium castaneum herbst infesting stored millets, Pennisetumglaucum (L.) R . Archives of Phytopathology and Plant Protection, 43(8), 810–816. https://doi.org/10.1080/03235400802246952
- Babarinde, S. A., Akinyemi, A. O., Usman, L. A., Odewole, A. F., Sangodele, A. O., Olasupo, O., & Olalere, O. D. (2014). Toxicity and repellency of Hoslundia opposita Vahl (Lamiaceae) leaves‘ essential oil against rust-red flour beetle, Tribolium castaneum Herbst (Coleoptera: Tenebrionidae). Natural Product Research, 28(6), 365–371. https://doi.org/10.1080/14786419.2013.866115
- Black, B. C., Hollingworth, R. M., Ahammadsahib, K. I., Kukel, C. D., & Donovan, S. (1994). Insecticidal action and mitochondrial uncoupling activity of AC-303,630 and related halogenated pyrroles. Pesticide Biochemistry and Physiology, 50(2), 115–128. https://doi.org/10.1006/pest.1994.1064
- Boeke, S. J. 2002. Traditional African plant products to protect stored cowpeas against insect damage Graduate thesis.
- Buxton, T., Takahashi, S., Doh, A. E., Baffoe-ansah, J., Owusu, O., & Kim, C. (2019). Insecticidal activities of cinnamic acid esters isolated from Ocimumgratissimum L . and Vitellaria paradoxa Gaertn leaves against Tribolium castaneum Hebst (Coleoptera : Tenebrionidae). Pest Management Science, 76(1), 257–267. https://doi.org/10.1002/ps.5509
- Don-Pedro, K. N. (1989). Mechanisms of action of some vegetable oils motsch (Coleoptera : Curculionidae) on wheat. Journal of Stored Products Research, 25(4), 217–223. https://doi.org/10.1016/0022-474X(89)90027-1
- El-aziz, A., Omer, E. A., & Sabra, A. S. (2007). Chemical composition of Ocimumamericanum essential oil and its biological effects against, Agrotis ipsilon, (Lepidoptera : Noctuidae). Journal of Agriculture & Biological Sciences, 3(6), 740–747.
- Enan, E. (2001). Insecticidal activity of essential oils: Octopaminergic sites of action. Toxicology & Pharmacology,130(3), 325-335. https://doi.org/10.1016/S1532-0456(01)00255-1
- Enan, E. E. (2005). Molecular and pharmacological analysis of an octopamine receptor from American cockroach and fruit fly in response to plant essential oils, 171, 161–171. https://doi.org/10.1002/arch.20076
- Eze, S., & Echezona, B. C. (2012). Agricultural pest control programmes, food security and safety. African Journal of Food, Agriculture, Nutrition and Development, 12(5), 1–11. https://doi.org/10.18697/ajfand.53.10385
- Gerwick, B. C., & Sparks, T. C. (2014). Natural products for pest control: An analysis of their role, value and future. Pest Management Science, 70(8), 1169–1185. https://doi.org/10.1002/ps.3744
- Grote, U., Fasse, A., Nguyen, T. T., & Erenstein, O. (2021). Food security and the dynamics of wheat and maize value chains in Africa and Asia. Frontiers in Sustainable Food Systems, 4, 1–17. https://doi.org/10.3389/fsufs.2020.617009
- Khater, H. F. (2012). Ecosmart biorational insecticides : Alternative insect control strategies. Advances in Integrated Pest Management, 17–60.
- Kordali, S., Aslan, I., Çalmaşur, O., & Cakir, A. (2006). Toxicity of essential oils isolated from three Artemisia species and some of their major components to granary weevil, Sitophilus granarius (L.) (Coleoptera: Curculionidae). Industrial Crops and Products, 23(2), 162–170. https://doi.org/10.1016/j.indcrop.2005.05.005
- Mishra, B. B., Tripathi, S. P., & Tripathi, C. P. M. (2012). Repellent effect of leaves essential oils from Eucalyptus globulus (Myrtaceae) and Ocimum basilicum (Lamiaceae) against two major stored grain insect pests of coleopterons. Journal of Natural Sciences Research, 10(2), 50–54. http://sciencepub.net
- Mondal, M., & Khalequzzaman, M. (2009). Ovicidal activity of essential oils against red flour beetle, Tribolium castaneum (Coleoptera: Tenebrionidae). Journal of Bio-Science, 17(2), 57–62. https://doi.org/10.3329/jbs.v17i0.7102
- Mumeen, A. 2013. Biochemical and microbiological analysis of shea nut cake. Doctoral Dissertation, Kwame Nkrumah University of Science and Technology.
- Obeng-Ofori, D. (2007). The use of botanicals by resource poor farmers in Africa and Asia for the protection of stored agricultural products. Stewart Postharvest Review, 3(6), 1–8. https://doi.org/10.2212/spr.2007.6:10
- Obeng-Ofori, D., & Amiteye, S. (2005). Efficacy of mixing vegetable oils with pirimiphos-methyl against the maize weevil, Sitophilus zeamais motschulsky in stored maize. Journal of Stored Products Research, 41(1), 57–66. https://doi.org/10.1016/j.jspr.2003.11.001
- Obeng-Ofori, D., Reichmuth, C. H., Bekele, A. J., & Hassanali, A. (1998). Toxicity and protectant potential of camphor, a major component of essential oil of Ocimumkilimandscharicum, against four stored product beetles. International Journal of Pest Management, 44(4), 203–209. https://doi.org/10.1080/096708798228112
- Ogban, E. I. (2019). Insecticidal value of four vegetable oils against Dermestes maculatus DeGeer on smoked African mud cat fish, Clarias gariepinus Burchell. Journal of Entomology and Zoology Studies, 7(3), 563–568.
- Ogendo, J. O., Kostyukovsky, M., Ravid, U., Matasyoh, J. C., Deng, A. L., Omolo, E. O., Kariuki, S. T., & Shaaya, E. (2008). Bioactivity of Ocimumgratissimum L. oil and two of its constituents against five insect pests attacking stored food products. Journal of Stored Products Research, 44(4), 328–334. https://doi.org/10.1016/j.jspr.2008.02.009
- Osei Akoto, C., Acheampong, A., Boakye, Y. D., Naazo, A. A., & Adomah, D. H. (2020). Anti-inflammatory, antioxidant, and anthelmintic activities of Ocimumbasilicum (Sweet Basil) fruits. Journal of Chemistry, 2020, 9. https://doi.org/10.1155/2020/2153534
- Priestley, C. M., Williamson, E. M., Wafford, K. A., & Sattelle, D. B. (2003). Thymol, a constituent of thyme essential oil, is a positive allosteric modulator of human GABA A receptors and a homo-oligomeric GABA receptor from Drosophila melanogaster. British Journal of Pharmacology, 140(8), 1363–1372. https://doi.org/10.1038/sj.bjp.0705542
- Regnault-roger, C., & Hamraoui, A. (1993). Influence d ’ huiles essentielles aromatiques sur Acanthoscelides obtectus Say, bruche du haricot (Phaseolus vulgaris L .). ACTA BOTANICA GALLICA, 8078(140), 217–222. https://doi.org/10.1080/12538078.1993.10515584
- Ryan, M. F., & Byrne, O. (1988). Plant-insect coevolution and inhibition of acetylcholinesterase. Journal of Chemical Ecology, 14(10), 1965–1975. https://doi.org/10.1007/BF01013489
- Singh, S. R., Luse, R. A., Leuschner, K., & Nangju, D. (1978). Oil treatment for the control of callosobruchus maculatus during cowpea storage (F .). Journal of Stored Products Research, 14(2–3), 77–80. https://doi.org/10.1016/0022-474X(78)90001-2
- Smagghe, G., & Tirry, L. (2001). Insect midgut as a site for insecticide detoxification and resistance. In L. Ishaaya (Eds.), Biochemical sites of insecticide action and resistance (pp. 293–321). Springer. https://doi.org/10.1007/978-3-642-59549-3_14
- Sparks, T. C., Hahn, D. R., & Garizi, N. V. (2017). Natural products, their derivatives, mimics and synthetic equivalents: Role in agrochemical discovery. Pest Management Science, 73(4), 700–715. https://doi.org/10.1002/ps.4458
- Suthisut, D., Fields, P. G., & Chandrapatya, A. (2011). Contact toxicity, feeding reduction, and repellency of essential oils from three plants from the ginger family (Zingiberaceae) and their major components againstSitophilus zeamais and Tribolium castaneum. Journal of Economic Entomology, 104(4), 1445–1454. https://doi.org/10.1603/EC11050
- Tapondjou, A. L., Adler, C., Fontem, D. A., Bouda, H., & Reichmuth, C. (2005). Bioactivities of cymol and essential oils of Cupressussempervirens and Eucalyptus saligna against Sitophilus zeamais motschulsky and Tribolium confusum du val. Journal of Stored Products Research, 41(1), 91–102. https://doi.org/10.1016/j.jspr.2004.01.004
- Trease, G. E., & Evans, W. C. (2009). Pharmacognosy (16th ed.). W.B. Sanders Company Ltd.
- Vowotor, K. A., Mensah-bonsu, A., Mutungi, C., & Affognon, H. (2013). Postharvest losses in Africa – Analytical review and synthesis : The case of Ghana. In C. Mutungi & H. Affognon (Eds.), African insect science for food and health (pp. 121). ICIPE.