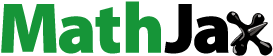
Abstract
No-till mechanized-transplanted rice was evaluated for different combinations of pre- and post-emergence herbicides to determine feasible, economically viable weed management options to control complex weed flora in rice fields. All pre-emergence herbicides significantly reduced the population of grassy weeds; of these, pendimethalin resulted in the greatest reductions (83%) at 15 days after transplanting (DAT). Among five post-emergence herbicide treatments, the combination of bispyribac-sodium (10%SP) + pyrazosulfuron (10%WP) was found to be the most effective in controlling all weed flora at both 35 and 55 DAT. The sequential application of pendimethalin (pre-emergence) followed bispyribac-sodium + pyrazosulfuron (post-emergence) resulted in significantly higher rice grain yield (4.4 t-ha−1) and relative gross-margin (417 USD-ha−1) than all other treatments. A strong negative correlation was observed between rice grain yield and weed biomass, and a strong positive correlation between rice grain yield and weed control efficiency. Our findings demonstrate the potential to combine pre- and post-emergence herbicides in no-till mechanized-transplanted rice; these findings have applications globally in regions where rice is established by no-till or mechanized transplanting.
1. Introduction
Rice (Oryza sativa) is a staple food and key source of calories for billions of people across Asia and there is a clear need to sustainably increase rice production within the region to increase global food security. Under traditional rice establishment practices, the soil is intensively tilled and puddled before the crop is manually transplanted. This puddled, transplanted rice (PTR) method of crop establishment is energy, water and labor intensive and contributes to the destruction of soil physical (soil percolation, soil structure, aggregation, compactness, etc.) and biological (microbes, fungi, carbon dynamics etc.) properties (Choudhary et al., Citation2018; M. Gathala et al., Citation2014; Gathala et al., Citation2020a, b). Tillage and puddling operations destroy soil aggregates, break capillary pores, and compact the soil, leading to the increased bulk density of surface layers (Gathala et al., Citation2017, Citation2011; Gupta et al., Citation2003) which in turn results in reduced plant root growth and thus poor nutrient and water absorption by the rice crop.
Alternative crop establishment methods which promote healthier soils are emerging, including dry direct seeded rice (DSR) and unpuddled transplanted rice (UPTR). In UPTR, seedlings are mechanically transplanted into untilled, saturated soil: this is a conservation agriculture (CA)-based technology where rice yields are not compromised and where less energy is used in rice production than in PTR (Bell et al., Citation2019; Chaki et al., Citation2021; Gathala et al., Citation2020b; Haque & Bell, Citation2019; Islam et al., Citation2019; Mitra et al., Citation2018). UPTR is well suited to agricultural environments where the onset of wet-season rains is abrupt and heavy, as occurs across many regions in Asia. In these regions, DSR may result in poor or even complete crop establishment failure (Haque & Bell, Citation2019; Islam et al., Citation2019). As an alternative to DSR or PTR, UPTR is a viable option where the soil is minimally disturbed, tillage costs and water requirements are reduced, and there is greater energy efficiency and profit without yield penalty (Gathala et al., Citation2020a; Islam et al., Citation2019; Mitra et al., Citation2018). In key regions where UPTR is an attractive establishment method, jute is a widespread crop prior to the rice crop. The jute leaf-fall contributes to softening of the soil prior to rice establishment, further reducing tillage requirements and increasing the viability of UPTR.
Under minimum-till UPTR weeds are not suppressed by pre-sowing tillage operations or by standing water as they are under traditional PTR management, and thus new methods of effective weed control are required to ensure rice yields are not compromised. While research has been conducted to understand the rice-based cropping agro-ecologies in which UPTR is a viable crop establishment option (Chaki et al., Citation2021; Rajiv et al., Citation2018; Sahu et al., Citation2019), there has been little investigation into weed management under UPTR, particularly in environments where the onset of the monsoon is sharp and intense.
Considerable weed pressure has been observed under UPTR early in the crop growing season, leading to significantly reduced rice yields when weeds are not effectively managed (Rahman, Citation2016). Traditional methods of in-crop weed control in rice (besides suppression with standing water) are manual removal and hoeing, but due both to the high cost of labor and to labor shortages which are acute at times critical for weeding, these methods cannot be relied upon (Chauhan, Citation2012; Kumar & Ladha, Citation2011).
Herbicides are an effective alternative weed management strategy as they require less labor and have the potential to provide useful weed control while reducing labor and production costs. Non-selective herbicides (e.g., glyphosate) have been used prior to crop establishment to control annual and biannual weeds, however they are less effective at complete weed removal than tillage operations (Carretta et al., Citation2021). The high initial efficacy of glyphosate, as well as the introduction of genetically modified crops, can both contribute to a decline in the use of other herbicide options and less investment by industry to discover new active ingredients (Green & Owen, Citation2011). Control of complex weed flora through a single pre- or post-emergence herbicide application is a very challenging task, and it is likely that the use of the same herbicides over a prolonged period may contribute to herbicide resistance in weeds (Kim, Citation1996). Applying several herbicides which have different active ingredients is to be encouraged for broad-spectrum weed control. The sequential application of pre-followed by post-emergence herbicides in rice has proved to be effective in controlling weed flora well without yield penalty (Ramesha et al., Citation2017; V. Singh et al., Citation2016; Zahan et al., Citation2018). At the same time, applying different herbicides in combination may reduce herbicide resistance (Mahajan & Chauhan, Citation2015). Chemical weed control has been shown to initially affect soil microbial populations, but this is followed by increased microbial population density and enzymatic activity as the crop progresses, particularly under UPTR (Pattanayak et al., Citation2022). Moreover, it has been observed that many reduced-tillage crop management practices promote small-seeded weeds (Chauhan, Citation2012), and we believe that this may also be true for UPTR.
Across the Eastern Gangetic Plain region of South Asia, farmers generally use only pre-emergence herbicides followed by limited in-crop hand weeding. Labour shortages are increasing in this region, and there is a need to identify the correct combination of herbicides which is most effective, and which can be recommended to smallholder farmers who are seeking to adopt CA-based crop establishment practices including UPTR.
In this present study, we hypothesise that it will be possible to examine the performance of sequential herbicide combinations under UPTR in the Eastern Gangetic Plains and to identify the most effective combination of pre- and post-emergence herbicides to suppress a range of weeds. This information will enable smallholder farmers to improve their rice crop productivity and increase their profitability. Our results will enable the recommendation of a regionally appropriate pre- and post-emergence herbicide combination for effective weed control under UPTR, which may also have benefits for other rice production methods in the region (e.g., PTR in rainfed ecologies, where weed pressure is very high and farmers are unable to manually control weeds). While the efficacy of these herbicide combinations has been well documented under DSR and in other agro-ecologies, little information is available on the optimum herbicide management strategy for weed control under UPTR and in the Eastern Gangetic Plains. The findings reported here have applications in other tropical rice-based agro-ecologies where UPTR is an appropriate rice establishment practice.
2. Materials and methods
2.1. Experimental site
The experiment was conducted during 2015 and 2016 in wet (rice growing) seasons at the research farm of Uttar Banga Krishi Viswavidyalaya (North Bengal Agricultural University), Cooch Behar, West Bengal, India, 26°24ʹ02.2” N, 89°23ʹ21.7” E, elevation 43 m above mean sea level. The soil of the experimental site was sandy loam and acidic (pH 5.54), well drained and with an organic carbon content of 0.89% (Sinha et al., Citation2019). The site is subtropical and humid with high annual precipitation of between 2800 and 3000 mm, of which 70–90% occurs during June to October. Minimum temperatures below 10°C occur in December and January, while maximum temperatures of around 35°C occur in April and May. Relative humidity is very high (70 to 100%) from May to December. The meteorological data pertaining to the period of experimentation are depicted in Figure . Prior to commencing the experiment, a uniform application of glyphosate was applied 10 days before rice seedlings were transplanted. There were no weeds present at transplanting in any plots.
3. Experimental details and management
The experiment was laid out in a two-factor randomized block design. Four pre-emergence weed management options were treated as factor 1 comprising a control (no pre-emergence herbicide applied) treatment and treatments with the application of one of three pre-emergence herbicides: pendimethalin 30 % EC (1000 g a.i. ha−1), oxadiargyl 80% WP (90 g a.i. ha−1) and pretilachlor 50% EC + safener (500 g a.i. ha−1). Five post-emergence weed management options were taken as factor 2: comprising the control (no post-emergence herbicide applied) treatment and the application of one of four post-emergence herbicides: bispyribac-sodium 10% SP (25 g a.i. ha−1), bispyribac-sodium+pyrazosulfuron 10% WP (20 g a.i. ha−1), fenoxaprop-p-ethyl 6.9% EC (56 g a.i. ha-1)+ethoxysulfuron 15% WDG (18 g a.i. ha−1) and fenoxaprop-p-ethyl followed by (fb) halosulfuron 75% WG (67 g a.i. ha−1). All of these herbicides are legal and available within the Eastern Gangetic Plain. Each experimental treatment was replicated thrice. Details of the treatment applications are shown in Table . Each experimental plot was 5 m long and 3.60 m wide (18 m2).
Table 1. Details of the experimental herbicide treatments
Herbicides may persist in the application area for some time, the duration over which they persist depends on the chemical nature, molecular stability and environmental factors.
The persistency of the pre-emergence herbicides used in this experiment (pendimethalin, pretilachlor and oxadiargyl) is very short under Indian conditions (Kumar & Ladha, Citation2011), as these herbicides undergo biotic degradation (by microbes or by plant enzymes) and abiotic degradation (sunlight or chemical degradation). The post-emergence herbicides used in this experiment are less toxic to humans and other animals and were applied at relatively low doses. These herbicides also do not persist long in the environment, particularly under South Asian climates (Choudhury et al., Citation2016). Well-targeted applications of effective herbicides (against which weeds have not developed resistance) are one of the best ways to minimise chemical applications while recognising that regional labor shortages mean some chemical use is inevitable in crop production.
The rice variety Naveen was used in the experiment, which has medium-long grains, a cropping-season duration of 115–120 days and a yield potential of 5.0 t ha−1. Rice seedlings were raised in a nursery on mats for 18 days prior to machine transplanting. At transplanting, the rice root mat was separated from its supporting polythene sheet and cut to size (610 mm by 225 mm) to fit the mechanical rice transplanter. Rice was transplanted on 2 July 2015 and 3 July 2016.
Seedlings were transplanted into untilled soil using an 8-row transplanter with 225 mm line-to-line spacing and 140 mm hill-to hill-distance. Two passes of the rice transplanter were required to fully plant out each experimental plot. The long-term historical annual precipitation has received more than 3200 mm, which is received mainly 90% during pre- and monsoon season (May to October), therefore farmers grow their monsoon season rice as a rainfed crop in the region. The crop received a good quantity of rainfall with the onset of south-west monsoon during June (622 mm in 2015 and 886 mm in 2016; Figure ), this rainfall before and during the rice-seedling transplanting period was effective for crop establishment as the soil was very soft and the residue of previous (wheat) crop had partially decomposed. Nutrients were applied at 80 kg ha−1nitrogen (as urea), 40 kg ha−1phosphorus (as P2O5), 40 kg ha−1 potassium (as K2O) and 25 kg ha−1 zinc (as ZnSO4). The nitrogen fertiliser was applied in three splits (i.e. 50% at transplanting, 25% at 21 days after transplanting and 25% at 42 days after transplanting), while the complete applications of the remaining fertilizers were applied immediately prior to rice transplanting.
Herbicides were applied with the use of a knapsack sprayer fitted with a flat-fan multi-nozzle boom (1-m width, configured with three flat-fan nozzles at 50-cm spacing, which covers a 1.5 m swath of spraying in one pass), using water as a carrier, and at rates of 500 L ha−1 for pre-emergence sprays and at 375 L ha−1 for post-emergence sprays. All herbicides were completely mixed with water prior to placement in the knapsack spray tank. Sprayer operators were provided with personal-protective equipment during spraying operations. No phytotoxicity was observed in this study.
Pre-emergence herbicides were sprayed onto treatment plots within two days of transplanting to impede weed-seed germination and post-emergence herbicides were applied at 15–25 days after transplanting (DAT), at the 3–5 leaf stage of weed plants. In each individual (sub-plot) treatment plot, four quadrats (0.5 m × 0.5 m; 0.25 m2) were established at 2 DAT. Weeds were measured three times during the crop season: at 15 DAT (i.e. just before post-emergence herbicide application), at 35 DAT (20 days after post-emergence herbicide application) and at 55 DAT. At each count, weed species were sorted into grass, broadleaf and sedge species. At 35 DAT weed biomass samples were taken from two randomly chosen quadrats in each plot, and at 55 DAT weed biomass was sampled from the remaining two quadrats. For biomass sampling, weeds were cut at ground level, washed with tap water, sun-dried, oven-dried at 70°C for 48 hours and then weighed.
Rice grain yield was measured at rice harvest over an area 3.6 m by 4 m, discarding 0.5 m area from all sides (14.40 m2 net area) and reported at 14% moisture. Rice grain yield is reported as the average yield over the two experimental seasons. The relative rice grain yield was the yield difference in each treatment plot compared to the complete control (i.e. no pre- or post-emergence herbicide applied) plot.
Weed control efficiency (WCE) was calculated based on weed biomass reduction using the equation:
3.1. Economic analysis
All management practices except herbicide application followed the same protocols in all treatments and so we did not calculate the total cost of crop production but assumed all other costs as fixed. Instead, we examined only the expense incurred in buying and applying herbicides, as well as any revenue earned or lost due to changes in rice grain yields resulting from herbicide use; the latter minus the former was the relative gross margin. Individual year data were used when calculating the relative herbicide cost and relative gross margin, which were calculated as the difference between the experimental and the control treatments.
3.2. Statistical analysis
Mean separations for different treatments under different parameters were performed using Tukey’s HSD (honest significant difference) test (P ≤ 0.05). Statistical analyses were conducted using JMP 16.0 software. The weed number and biomass data were transformed tolog10to satisfy the assumptions of analysis of variance to achieve a best fit normality distribution. As the experimental aim was to determine the most appropriate herbicide combination under no till UPTR, a full factorial ANOVA was run. The factors used in the ANOVA were year (Y), pre-emergence (PH), Y × PH, post-emergence (PO), Y × PO, PH × PO, Y × PH × PO and replication (i.e. year). However, for analysis of weed data on 15 DAT, only one factor (PH) was considered as post-emergence herbicides were applied after 15 DAT. We observed relationships between grain yield and weed biomass and between grain yield and WCE and fitted linear equations to them to describe their trends.
4. Results
4.1. Weed flora
The weed flora observed were the grasses Cynodon dactylon L., Echinochloa colona L., Digitaria ciliaris L., Dactyloctenium aegyptium L., Eleusine indica L. and Leptochlova chinensis L.; the sedges Cyperus rotundus L., Cyperus iria L., Cyperus difformis L., and Fimbristylis miliacea L.; and the broad-leaf weeds Ludwigia octovalvis, Ageratum conyzoides L., Eclipta alba L. and Enhydra fluctuans. During the initial period (1–20 DAT), grassy weeds were predominant, with some sedges present, while broad-leaf weeds, especially L. parviflora, emerged at 20–25 DAT and were present in control plots throughout the remainder of both rice growing seasons (individual weed species data not collected).
4.2. Weed density
4.2.1. Grasses
The density of grassy weeds was significantly affected at 35 and 55 DAT by both pre- and post-emergence herbicide applications (Table ). At 15 DAT when post-emergence herbicides were not applied, the weed density also varied significantly under different pre-emergence treatment. The grass weed population was lowest with the pre-emergence herbicide pendimethalin; in this treatment, grass weeds were 83, 80 and 68% lower at 15, 35 and 55 DAT, respectively, than in the control treatment. Other pre-emergence herbicides (oxadiargyl and pretilachlor) were also effective in reducing grassy weed populations compared to the no-pre-emergence herbicide treatment, but these had 39% to 47% higher grassy weed populations than the pendimethalin treatment at 55 DAT.
Table 2. Effect of pre- and post-emergence herbicides on weed density and weed biomass at different crop growth stages under unpuddled mechanically transplanted rice
All post-emergence herbicide applications significantly reduced the number of grassy weeds compared to the no-post-emergence herbicide treatment (Table ) at both 35 and 55 DAT. Among the post-emergence herbicides, bispyribac + pyrazosulfuron was 39% more effective in reducing grassy weeds at 35 DAT than all other post-emergence options; it was also more effective than bispyribac alone at 55 DAT when fenoxaprop + ethoxysulfuron was equally effective.
The interaction effects of pre- and post-emergence treatments were only significant at 55 DAT in grassy weeds. In general, the pre-emergence herbicide pendimethalin in combination with any post-emergence herbicide option was effective at reducing grassy weed populations (Figure ). The most effective combination was pendimethalin in combination with fenoxaprop fb halosulfuron and bispyribac + pyrazosulfuron and the least effective combination was Oxadiargyl fb bispyribac and pretilachlor fb fenoxaprop + halosulfuron.
Figure 2. Effects of pre- and post-emergence herbicides on weed density in mechanized unpuddled transplanted rice for grassy weeds at 55 days after transplanting (DAT). Treatments with the same letter are not significantly different (p = 0.05) from each other. PH1 = no pre-emergence, PH2 = pendimethalin, PH3 = oxadiargyl, PH4 = pretilachlor; PO1 = no post-emergence, PO2 = bispyribac, PO3 = bispyribac+pyrazosulfuron, PO4 = fenoxaprop+ethoxysulfuron, PO5 = fenoxaprop fb halosulfuron.
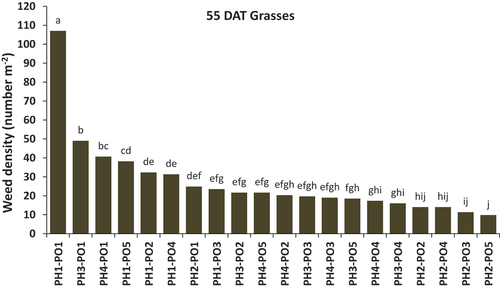
4.2.2. Sedges
The prevalence of sedges was influenced by pre- and post-emergence herbicide applications at 35 and 55 DAT (Table ). At 15 DAT both pendimethalin and pretilachlor were equally more effective in reducing sedges than oxadiargyl or the control treatment without pre-emergence herbicide; the former treatments reduced sedges by 22% over the latter. At 35 DAT, all pre-emergence herbicide treatments performed equally in controlling sedge density over the no-pre-emergence-herbicide control. The performance of the pre-emergence herbicides in controlling the density of sedges at 55 DAT was highest in pendimethalin, followed by oxadiargyl, then pretilachlor and finally the treatment with no pre-emergence herbicide. The most effective post-emergence herbicide treatment to reduce the density of sedges was fenoxaprop fb halosulfuron at both 35 and 55 DAT over the remainder of the post-emergence herbicide treatments. At 55 DAT, the bispyribac + pyrazosulfuron was also highly effective in reducing the sedge population relative to bispyribac alone and fenoxaprop + ethoxysulfuron. The sedge density at both 35 and 55 DAT was significantly lower in all post-emergence herbicide treatments compared to that observed in the treatment with no post-emergence herbicide.
Interactions between pre- and post-emergence herbicide treatments significantly affected the prevalence of sedges at both 35 DAT and 55 DAT (Figure ). Effective sedge reduction was observed when any pre-emergence herbicide was followed by the post-emergence herbicide fenoxaprop fb halosulfuron at both crop stages (Figure ). In general, the combination of pretilachlor as a pre-emergence herbicide with any of the post-emergence herbicide treatments resulted in poor control of sedges.
Figure 3. Effects of pre- and post-emergence herbicides on weed density in mechanized unpuddled transplanted rice for sedges weeds at 35 (top) and 55 (bottom) days after transplanting (DAT). For each graph treatments with the same letter are not significantly different (p = 0.05) from each other. PH1 = no pre-emergence, PH2 = pendimethalin, PH3 = oxadiargyl, PH4 = pretilachlor; PO1 = no post-emergence, PO2 = bispyribac, PO3 = bispyribac+pyrazosulfuron, PO4 = fenoxaprop+ethoxysulfuron, PO5 = fenoxaprop fb halosulfuron.
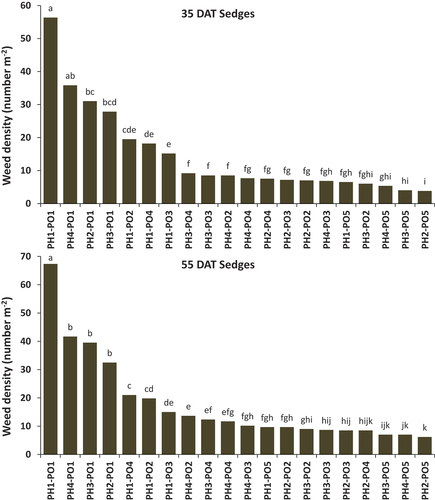
4.2.3. Broadleaf weeds
Pre-emergence herbicide applications reduced the population of broadleaf weeds at 15 DAT, 35 DAT and 55 DAT (Table ). For all crop growth stages, the three pre-emergence herbicide treatments reduced the broadleaf weed populations as compared to control (i.e. no pre-emergence herbicide) treatment by 26%, 35% and 29% at 15, 35 and 35 DAT, respectively. At 55 DAT, the application of pendimethalin resulted in lower broadleaf weed density than the application of pretilachlor. The maximum reduction in broadleaf weeds by post-emergence herbicides alone occurred with the bispyribac + pyrazosulfuron treatment compared to the rest of the post-emergence herbicide treatment sat both 35 and 55 DAT. The fenoxaprop fb halosulfuron treatment was the least effective in reducing the density of broadleaf weeds compared to all other post-emergence herbicide treatments, but it was more effective than the no-post-emergence herbicide treatment.
At both 35 and 55 DAT interactions between pre- and post-emergence herbicides were found significant with regard to the density of broadleaf weeds. Figure shows that the most effective control of broadleaf weeds was achieved by applying any of the three pre-emergence herbicides followed by bispyribac + pyrazosulfuron or bispyribac alone. Post-emergence herbicide application alone was not particularly effective in reducing broadleaf weeds as compared to combination with a pre-emergence herbicide.
Figure 4. Effects of pre- and post-emergence herbicides on weed density in mechanized unpuddled transplanted rice for broadleaf weeds at 35 (top) and 55 (bottom) days after transplanting (DAT). For each graph treatments with the same letter are not significantly different (p = 0.05) from each other. PH1 = no pre-emergence, PH2 = pendimethalin, PH3 = oxadiargyl, PH4 = pretilachlor; PO1 = no post-emergence, PO2 = bispyribac, PO3 = bispyribac+pyrazosulfuron, PO4 = fenoxaprop+ethoxysulfuron, PO5 = fenoxaprop fb halosulfuron.
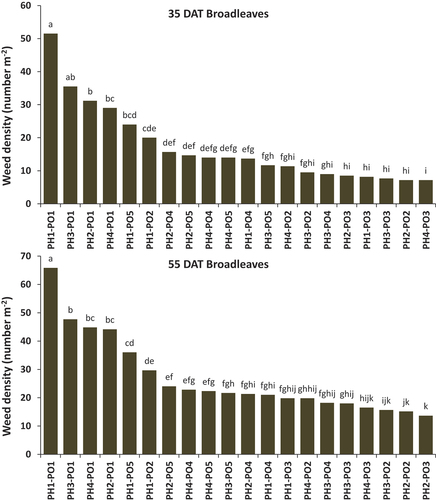
4.2.4. Weed and rice crop biomass
The weed biomass at 35 and 55 DAT followed the same trends as weed density: maximum weed biomass was recorded under the no-pre-emergence herbicide treatment (64.4 g m−2averaged across the two sampling dates) and minimum under the pendimethalin treatment (38.3 g m−2): i.e. the latter treatment produced 40% lower weed biomass (Table ). Pendimethalin also resulted in 12% lower weed biomass than either other pre-emergence herbicide, oxadiargyl or pretilachlor. In contrast, net rice crop biomass (Table ) was highest in the pendimethalin treatment (8.94 t ha−1) and lowest in the no-pre-emergence herbicide treatment (7.46 t ha−1).
Table 3. Individual and interaction effects of pre- and post-emergence herbicides on crop biomass and weed control efficiency (mean of two years’ data) under unpuddled mechanically transplanted rice
The no-post-emergence herbicide treatment produced higher weed biomass than any of the post-emergence herbicide treatments at both 35 and 55 DAT (Table ). Weed biomass under bispyribac + pyrazosulfuron treatment was significantly lower than under all other post-emergence herbicide treatments at both crop stages (35 and 55 DAT). The fenoxaprop fb halosulfuron treatment was less effective in reducing weed biomass than either bispyribac alone or fenoxaprop + ethoxysulfuron, at both 35 and 55 DAT. In sequential application, pendimethalin(pre-emergence) followed by bispyribac + pyrazosulfuron (post-emergence) had significantly produced the lowest weed biomass (33.6 g m−2) than rest of the herbicide combinations (Figure ). The rice crop biomass at harvest was 12% higher in the bispyribac + pyrazosulfuron treatment (Table ); this treatment achieved the highest rice yield (4395 kg ha−1) among all post-emergence herbicide treatments (Table ). The lowest rice crop biomass was observed in the no-post-emergence herbicide treatment. The harvest index (HI) varied significantly among all pre- and post-emergence herbicide treatments. Among the pre-emergence herbicide treatments, HI was highest in the pendimethalin treatment and lowest in the no-pre-emergence herbicide treatment (Table ). The application of bispyribac alone as a post-emergence achieved the highest HI. The interaction effect of pre-emergence and post-emergence on crop biomass was highest in the combination of pendimethalin with bispyribac + pyrazosulfuron (10.55 t ha−1) followed by pretilachlor with bispyribac + pyrazosulfuron (9.2 t ha−1).
Figure 5. Effects of pre- and post-emergence herbicides on weed biomass in mechanized unpuddled transplanted rice for broadleaf weeds at 55 days after transplanting (DAT). Treatments with the same letter are not significantly different (p = 0.05) from each other. PH1 = no pre-emergence, PH2 = pendimethalin, PH3 = oxadiargyl, PH4 = pretilachlor; PO1 = no post-emergence, PO2 = bispyribac, PO3 = bispyribac+pyrazosulfuron, PO4 = fenoxaprop+ethoxysulfuron, PO5 = fenoxaprop fb halosulfuron.
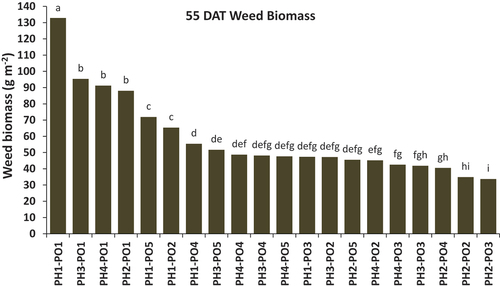
Table 4. Individual and interaction effects of pre- and post-emergence herbicides on rice grain yield and gross margins (mean of two years’ data) under unpuddled mechanically transplanted rice
4.3. Weed control efficiency and rice grain yield
Significant interactions at both later sampling dates (35 and 55 DAT) between pre- and post-emergence herbicides on weed control efficiency (WCE; Table ) were recorded. In general, a sole application of either pre- or post-emergence herbicide did not control weeds effectively as the sequential application of both pre- and post-emergence herbicides. Maximum WCE (e.g. 78.85 % and 74.62% at 35 and 55 DAT, respectively) was achieved with the sequential application of any of the three pre-emergence herbicides followed by bispyribac + pyrazosulfuron as a post-emergence herbicide, for both sampling dates. The highest WCE in pre-emergence herbicides alone was recorded with pendimethalin application (average across the two sampling dates of 66%), while the lowest WCE was in the treatment with no pre-emergence herbicide applied (42% averaged across the two sampling dates). Among the post-emergence herbicides, bispyribac + pyrazosulfuron achieved the maximum WCE of 72.6% and 68.7% at 35 and 55 DAT, respectively, while the remaining post-emergence herbicides had lower WCE than bispyribac + pyrazosulfuron but were more effective than the treatment without any post-emergence herbicide.
Rice grain yields differed significantly between the pre- and post-emergence herbicide treatments along with their interactions. Among the pre-emergence herbicide treatments, those with pendimethalin recorded a significantly higher grain yield (3.64 t ha−1 averaged across two seasons): this was 18% and 23% higher than the rice grain yields achieved under oxadiargyl or pretilachlor, respectively (Table ). The treatment with no pre-emergence herbicide produced the lowest rice grain yield over the two seasons (2.68 t ha−1). Rice grain yields differed under all post-emergence herbicide treatments over the two seasons: yields were highest under bispyribac + pyrazosulfuron (3.64 t ha−1), then under bispyribac, fenoxaprop fb halosulfuron, fenoxaprop + ethoxysulfuron, with rice under the no post-emergence herbicide treatment achieving the lowest yield (2.13 t ha−1).
In terms of interactions between pre- and post-emergence herbicides, the treatment with pendimethalin followed by bispyribac + pyrazosulfuron achieved the highest rice grain yield (4.40 t ha−1); this was significantly higher than all other treatment combinations except pendimethalin with bispyribac. In general, pendimethalin as a pre-emergence herbicide followed by any of the post-emergence herbicides achieved a higher rice grain yield than treatments with any other pre-emergence herbicides: this treatment combination achieved 18%, 24% and 36% higher rice yields than the treatments with oxadiargyl, pretilachlor or no-pre-emergence herbicide, respectively. Rice yield increased 174% under the sequential application of pendimethalin followed by bispyribac + pyrazosulfuron compared to yields from the absolute control treatment (no pre- or post-emergence herbicide). This also produced 10% higher rice grain yield than the next highest yielding treatment, pendimethalin followed bispyribac alone (Table ).
At both 35 and 55 DAT and across all herbicide treatment combinations, strong relationships (~R2 = 0.63) were observed between rice grain yield and both weed biomass and weed control efficiency (Figure ). Most weed biomass observations were between 20 and 60 g m−2 at 35 DAT and between 30 and 100 g m−2 at 55 DAT. Rice yield was expressed as a function of weed biomass at 55 DAT using the equation: y = −20.344x+4278.8, where y is rice yield and x is weed biomass at 55 DAT. To interpret this equation, when weed biomass production was 20 g m−2, rice grain yield decreased by 0.7 t ha−1, which was a loss of 14% in rice yield compared to when weeds were well controlled (i.e. when weed biomass was found to be nil). Further, when weed biomass increased to 40 or 80 g m−2, rice yields decreased by 1.3 and 2.2 t ha−1, and was 27% and 47% lower, respectively, than when weeds were ideally controlled. Similarly, a linear equation describing the relationship between rice grain yield and weed control efficiency (WCE) showed that when WCE was lower than 62% the rice grain yield was significantly reduced. To achieve high rice grain yields, weeds should be well controlled until at least maximum tillering and panicle initiation, i.e. between transplanting and 40–55 DAT.
4.4. Relative rice grain yields and relative gross margins
Both relative rice grain yield and relative gross margins (i.e. relative to the absolute control where no herbicides were applied) were significantly influenced by pre- and post-emergence herbicide application treatments and their interactions. The relative rice grain yields followed the same trends as the rice grain yields described in Section 3.3. A mean maximum rice grain yield (mean of 2.79 t ha−1over two seasons) was achieved relative to the absolute control in the treatment with pendimethalin followed by bispyribac + pyrazosulfuron (Table ). Pendimethalin alone as a weed control treatment produced an additional 0.95 t ha−1 rice grain over the absolute control; this was 62.5% higher than that achieved under either the oxadiargyl or pretilachlor sole pre-emergence treatments. The application of bispyribac + pyrazosulfuron as a post-emergence treatment increased relative rice grain yields by 2.03 t ha−1over the absolute control; this was 9.2%, 45.4%, 27.8% and 285% higher than rice yields achieved in the bispyribac, fenoxaprop + ethoxysulfuron, fenoxaprop fb halosulfuron and no-post-emergence herbicide treatments, respectively.
After the two-year experiment, the additional cost incurred due to the application of only pre-emergence herbicides varied between 5.27 and 17.57 USD ha−1; this was lowest in the pretilachlor treatment and the highest in the pendimethalin treatment. Similarly, for the post-emergence herbicides, the additional cost incurred varied between 10.41 and 75.14 USD ha−1and was lowest under fenoxaprop + ethoxysulfuron and highest under fenoxaprop fb halosulfuron. In the sequential herbicide treatments, the additional cost varied between 15.68 and 92.70 USD ha−1and was lowest under the pretilachlor followed by fenoxaprop + ethoxysulfuron treatment and highest under the pendimethalin followed by fenoxaprop fb halosulfuron treatment (Table ).
The relative mean gross margins across two crop seasons varied from 88.86 to 148.75 USD ha−1 in the pre-emergence only herbicide treatments, with the pendimethalin treatment having the highest gross margin and oxadiargyl having the lowest. In the post-emergence only herbicide treatments relative gross margins varied between 145 and 230 USD ha−1, with maximum gross margins under bispyribac + pyrazosulfuron treatment.
Relative gross margins increased considerably when pre- and post-emergence herbicides were used in combination. When pendimethalin was combined with bispyribac + pyrazosulfuron, the relative gross margin increased to 417 USD ha−1, which was significantly higher than the gross margin achieved under any other sequential herbicide combination examined except pendimethalin with bispyribac.
5. Discussion
5.1. Weed flora
In a similar sub-Himalayan plain environment, Maity and Mukherjee (Citation2009) also observed a greater presence of C. dactylon, C. rotundus and Ludwigia spp. under no-till UPTR. The increase in weed species observed here under UPTR relative to PTR has also been observed under untilled mechanized rice establishment (commonly direct seeded rice) in many other studies in South Asia, where new weed species such as C. dactylon, L. chinensis, D. cillaris, C. rotondus, and Ludwigia spp. have been observed (Kumar & Ladha, Citation2011; Gathala et al., Citation2013; Chhokar et al., Citation2014; Hossain et al., Citation2020). This shift in weed dynamics may be a result of a more plant-favourable environment arising out of improved soil health in the absence of tilling, puddling and soil compaction, which contributes to increasing weed seed density and prevalence (Kumar & Ladha, Citation2011; Chhokar et al., Citation2014; Hossain et al., Citation2020).
5.2. Weed density and biomass
This study demonstrated the potential of pendimethalin in particular as a pre-emergence herbicide under no-till UPTR to effectively control grass and some broadleaf weeds. All three pre-emergence herbicides, pendimethalin, oxadiargyl and pretilachlor, were more effective at reducing the density of grass weeds than of sedges or broadleaf weeds in the first 15 DAT. Combining these herbicides with post-emergence herbicides has not been trialled before in this region, and this approach is an innovative one to test new management options for farmers. Pendimethalin reduced grassy weeds population by 83%, 80% and 68% during 15, 35 and 55 DAT as compared to no herbicides treatment. Pre-emergence herbicides restrict the germination of weed seeds lying within 2–3 cm of the soil surface as they inhibit cell division during germination (Rao et al., Citation2007; Hossain et al., Citation2020). Pendimethalin had a greater effect on grasses and higher overall efficacy and longer residual effects in fields, which were wet but without standing water than either oxadiargyl or pretilachlor, both of which require highly saturated soils or even standing water for optimum weed control (Chhokar et al., Citation2007; Mahajan & Chauhan, Citation2015; V. Singh et al., Citation2016). Reductions in the density of grasses like Echinochloa have also been previously observed with the application of a group of pre-emergence herbicides including pendimethalin (Khaliq & Matloob, Citation2012; Hossain et al., Citation2021; Chatterjee et al., Citation2021). The effectiveness of pendimethalin as a pre-emergence herbicide in reducing the density of grass weeds has further been observed more widely across South Asia (Rao et al., Citation2007; M. Singh et al., Citation2015). Most pre-emergence herbicides lack efficacy against sedge weeds, in particularly those which multiply through rhizomes and stolon rather than by cell division; thus, the pre-emergence herbicides tested here were less effective against sedges (Rao et al., Citation2007; M. Singh et al., Citation2015; Hossain et al., Citation2020; Chatterjee et al., Citation2021).
No pre-emergence herbicide alone was effective at preventing the establishment of weeds at later sampling dates due to the relatively short-lived persistence of pre-emergence herbicides under hot and moist conditions (Saha et al., Citation2021). Post-emergence herbicides were also able to reduce weed density: in particular, the bispyribac alone or bispyribac + pyrazosulfuron treatments not only controlled both grassy and broadleaf weeds well but also effectively controlled sedges, particularly Cyperus iria which is a major sedge weed in the region (Jat et al., Citation2021; Saha et al., Citation2021). In our experiment, bispyribac + pyrazosulfuron and bispyribac alone reduced the grass weed density by 66–67%; while the broadleaf weed density was reduced by 79 and 66% during 35 and 55 DAT, respectively. This ability of bispyribac-based treatments to suppress many different weed types is attributed to the wide spectrum range of weed flora targeted: bispyribac alone controls grass weeds well, and also to some extent sedges and broadleaf weeds; when combined with a sulfonylurea compound such as pyrazosulfuron it also effectively control sedges and broadleaf weeds in their early growth stages (Gill et al., Citation2013; Saha et al., Citation2021; V. Singh et al., Citation2016). The post-emergence herbicide fenoxaprop + ethoxysulfuron failed to control sedges; however, this herbicide was reasonably effective at controlling broadleaf weeds. Another sulfonylurea compound, halosulfuron, was very effective at controlling all sedge weeds, including rhizome-propagated plants: consequently, the treatment fenoxaprop fb halosulfuron resulted in a significant reduction in sedge density which has been previously observed in South Asia (Saha et al., Citation2021).
Our results demonstrate that either pre-emergence or post-emergence herbicides alone was not able to control weed flora effectively: weed control efficiency was less than 60% in these treatments relative to combinations of pre- and post-emergence herbicides (Table ). The effectiveness of sequential application of herbicides in managing weed flora under mechanized rice establishment in both no-till and tilled experiments was reported by various studies (Jat et al., Citation2021; Kaur & Singh, Citation2015; Kumar & Ladha, Citation2011; Mahajan & Chauhan, Citation2015; Rao et al., Citation2007; Saha et al., Citation2021; M. Singh et al., Citation2015; V. Singh et al., Citation2016).
The combined application of appropriate pre- and post-emergence herbicides, applied at correct weed growth stages, is key to effectively control weeds under no-till mechanized UPTR. We observed maximum weed biomass reduction across all weed flora at both 35 and 55 DAT with the sequential application of pendimethalin as a pre-emergence herbicide followed by bispyribac + pyrazosulfuron as a post-emergence herbicide. Lower weed density at all sampling times was the primary reason for this reduced weed biomass. Mahajan et al. (Citation2009) observed that the sequential application of pendimethalin followed by bispyribac effectively controlled weeds in mechanized dry direct-seeded rice, while other studies observed the lowest weed biomass in treatments with the sequential application of pendimethalin followed by bispyribac + azimsulfuron, i.e. bispyribac combined with another sulfonylurea compound (Mahajan & Chauhan, Citation2013; R.G. Singh et al., Citation2010; Saha et al., Citation2021). The combination of pendimethalin followed by fenoxaprop + ethoxysulfuron also provided good control of mixed weed flora as the addition of pendimethalin broadened both the duration of weed control and the spectrum of weeds targeted, while the sulfonylurea compound effectively controlled both sedges and broadleaf weeds. The suppression of late weed flushes increased the effectiveness of sulfonylurea compounds such as pyrazosulfuron to reduce weed density and increase weed control efficiency in transplanted rice, as has been previously reported by Pal et al. (Citation2012) and Saha et al. (Citation2021).
5.3. Weed control efficiency and rice crop yields
All sequential herbicide combination treatments resulted in better rice yield performance than was achieved under the application of a pre-emergence or post-emergence herbicide alone. This is a result of weed control over both a longer period of time and a diverse weed flora leading to higher weed control efficiency (WCE) and lower weed biomass in each of the sequential treatment combinations than in the single-herbicide treatments. Sequential application of herbicides has helped to reduce crop-weed competition at critical rice crop growth periods (i.e. initial 6 weeks during active plant tillering) by keeping weeds below a critical level; this process enables the rice crop to utilize all resources and promotes effective rice growth (Ahmed et al., Citation2021; Mahajan et al., Citation2014; Mukherjee et al., Citation2008). The most critical growth stage of rice-weed crop competition is two to six weeks after transplanting: this is the active tillering period for the rice crop, which determines the rice yield. The highest rice grain yield, achieved under the pendimethalin followed by bispyribac + pyrazosulfuron treatment, was achieved due to 78% higher WCE in this treatment than in the absolute control treatment, which reduced weed density and biomass by 64% and 45% during 35 and 55 DAS, respectively, and facilitated higher relative rice grain yield gain (2.8 t ha−1). Effective weed control was achieved at initial plant growth stages under pendimethalin, while weed control around tillering resulted from the bispyribac + pyrazosulfuron, which reduced weed-crop competition and promoted rice biomass and grain productivity. Higher rice grain yields with increased WCE have been reported elsewhere with sequential applications of pendimethalin followed by bispyribac alone or mixed with pyrazosulfuron (Kaur & Singh, Citation2015; Khaliq et al., Citation2012; Saha et al., Citation2021; V. Singh et al., Citation2016; Walia et al., Citation2012). While conducting research on alternate crop establishment techniques of rice, Patra et al. (Citation2018) showed higher yields under PTR during initial years, while UPTR recorded maximum grain yields (4616 kg ha−1) in later years, being at par with PTR (4606 kg ha−1). However, the yield was significantly reduced under DSR (2750 kg ha−1) due to poor crop establishment following high rainfall early in the cropping season. In our experiment, we also observed similar yields under UPTR with appropriate pre- and post-emergence herbicides in combination and under PTR.
The sequential herbicide application combinations showed strong correlations (~R2 = 0.63) between rice grain yield and both weed biomass and WCE (Figure ). Equations relating rice grain yield and WCE indicate that when WCE was less than 62% the rice grain yield was significantly reduced thus to achieve high rice grain yields it is critical that rice fields should be weed-free up to maximum tillering and panicle initiation, i.e. between transplanting and 40–55DAT, as these are the growth stages of rice critical to determining the ultimate yield. Strong positive relationships between rice grain yield and WCE and strong inverse relationships between rice grain yield and weed biomass have also been reported in many studies previously (Walia et al., Citation2012; Kaur & Singh, Citation2015; Mahajan & Chauhan, Citation2015; Hossain et al., Citation2020; Saha et al., Citation2021).
5.4. Gross margins
Relative rice gross margins were a function of rice grain yield and herbicide costs. While the sequential application of pendimethalin followed by bispyribac + pyrazosulfuron increased herbicide costs, this treatment also achieved maximum relative gross margins, 18% and 29% higher over next best treatment combinations viz., pendimethalin followed by bispyribac alone and pendimethalin followed by fenoxaprop + ethoxysulfuron due to better weed control efficiency, which facilitated rice plant growth and ultimately resulted in higher grain yield. The application of pre- or post-emergence herbicides alone was cheaper but ultimately resulted in lower relative gross margins than the sequential treatments, indicating that low weed control efficiencies depressed net rice grain yields. Higher gross margins in rice cultivation were mostly associated with higher weed control efficiencies as observed in various studies (Kumar & Ladha, Citation2011; Mahajan & Chauhan, Citation2015; Saha et al., Citation2021; V. Singh et al., Citation2016).
6. Conclusions
While UPTR has been previously shown to be a sustainable, energy-efficient crop establishment practice under which similar yields to those under PTR can be achieved, there remain challenges of increased weed pressure, diversity, and prevalence. In research, we have demonstrated that effective weed control across a range of weed flora is achievable under no-till UPTR using innovative combinations of pre- and post-emergence herbicides. Weeds were most effectively managed with the sequential combination of pendimethalin (pre-emergence) followed by bispyribac + pyrazosulfuron (post-emergence). There was an increase in production costs associated with herbicide use; however, using herbicides also increased rice grain yields and gross margins, which were highest in the treatments where weeds were well controlled relative to control treatments. Our findings are relevant and applicable in other rice-producing agro-ecologies where no-till UPTR is a promising alternative to traditional PTR. Future research could include integrating herbicide use with mechanized weed management strategies, weed control efficiency on the individual weed flora and herbicide efficiency at different moisture regimes; this may reduce dependency on herbicides alone.
Acknowledgements
This research was conducted as part of the Sustainable and Resilient Farming Systems Intensification in the Eastern Gangetic Plains (SRFSI) project (CSE/2011/077) funded by the Australian government through the Australian Centre for International Agricultural Research and the Department of Foreign Affairs and Trade. We are grateful to Uttar Banga Krishi Viswavidyalaya for facilitating the field trials, laboratory analyses and providing support staff. Thoughtful comments from two anonymous reviewers improved this manuscript.
Disclosure statement
The authors declare that they have no known competing financial interests or personal relationships that could have appeared to influence the work reported in this paper.
Additional information
Funding
References
- Ahmed, S., Alam, M. J., Hossain, A., Islam, A. K. M. M., Awan, T. H., Soufan, W., Qahtan, A. A., Okla, M. K., & El Sabagh, A. (2021). Interactive effect of weeding regimes, rice cultivars, and seeding rates influence the rice-weed competition under dry direct-Seeded condition. Sustain, 13(1), 317. https://doi.org/10.3390/su13010317
- Bell, R. W., Haque, M. E., Jahiruddin, M., Rahman, M. M., Begum, M., Miah, M. A. M., Islam, M. A., Hossen, M. A., Salahin, N., Zahan, T., Hossain, M. M., Alam, M. K., & Mahmud, M. N. H. (2019). Conservation agriculture for rice-based intensive cropping by smallholders in the Eastern Gangetic Plain. Agriculture, 9(1), 5. https://doi.org/10.3390/agriculture9010005
- Carretta, L., Cardinali, A., Onofri, A., Masin, R., & Zanin, G. (2021). Dynamics of glyphosate and aminomethylphosphonic acid in soil under conventional and conservation tillage. International Journal of Environmental Health Research, 15(6), 1037–23. https://doi.org/10.1007/s41742-021-00369-3
- Chaki, A. K., Gaydon, D. S., Dalal, R. C., Bellotti, W. D., Gathala, M. K., Hossain, A., Siddique, N.-E.-A., & Menzies, N. W. (2021). Puddled and zero-till unpuddled transplanted rice are each best suited to different environments- An example from two diverse locations in the Eastern Gangetic Plains of Bangladesh. Field Crops Research, 262, 108031. https://doi.org/10.1016/j.fcr.2020.108031
- Chatterjee, S., Gangopadyay, P., & Chattopadhyay, C. (2021). Input-based assessment on integrated pest management for transplanted rice (Oryza sativa) in India. Crop Protection (Guildford, Surrey), 141, 105444. https://doi.org/10.1016/j.cropro.2020.105444
- Chauhan, B. S. (2012). Weed ecology and weed management strategies for dry seeded rice in Asia. Weed Technology, 26(1), 1–13. https://doi.org/10.1614/WT-D-11-00105.1
- Chhokar, R. S., Sharma, R. K., Gathala, M. K., & Pundir, A. K. (2014). Effects of crop establishment techniques on weeds and rice yield. Crop Protection (Guildford, Surrey), 64, 7–12. https://doi.org/10.1016/j.cropro.2014.05.016
- Chhokar, R. S., Sharma, R. K., Jat, G. R., Pundir, A. K., & Gathala, M. K. (2007). Effect of tillage and herbicides on weeds and productivity of wheat under rice–wheat growing system. Crop Protection, 26(11), 1689–1696. https://doi.org/10.1016/j.cropro.2007.01.010
- Choudhary, M., Jat, H. S., Datta, A., Yaday, A. K., Sapkota, T. B., Mondal, S., Meena, R. P., Sharma, P. C., & Jat, M. L. (2018). Sustainable intensification influences soil quality, biota, and productivity in cereal-based agroecosystems. Applied Soil Ecology: a Section of Agriculture, Ecosystems & Environment, 126, 189–198. https://doi.org/10.1016/j.apsoil.2018.02.027
- Choudhury, P. P., Singh, R., Ghosh, D., & Sharma, A. R. (2016). Herbicide use in Indian Agriculture. 110. Indian Society of Weeds Science, Jabalpur.
- Gathala, M. K., Jat, M. L., Saharawat, Y. S., Sharma, S. K., Singh, Y., & Ladha, J. K. (2017). Physical and chemical properties of a sandy loam soil under irrigated rice-wheat sequence in the indo-gangetic plains of South Asia. Journal of Ecosystem and Ecography, 7. https://doi.org/10.4172/2157-7625.S7-002
- Gathala, M. K., Kumar, V., Sharma, P. C., Saharawat, Y. S., Jat, H. S., Singh, M., Kumar, A., Jat, M. L., Humphreys, E., Sharma, D. K., Sharma, S., & Ladha, J. K. (2013). Optimizing intensive cereal-based cropping systems addressing current and future drivers of agricultural change in the northwestern Indo-Gangetic Plains of India. Agriculture, Ecosystems & Environment, 177, 85–97. https://doi.org/10.1016/j.agee.2013.06.002
- Gathala, M. K., Ladha, J. K., Sharma, P. K., Saharawat, Y. S., Sharma, P. K., Pathak, H., Sharma, S., & Pathak, H. (2011). Tillage and crop establishment affects sustainability of South Asian rice–wheat system. Agronomy Journal, 103, 961–971. https://doi.org/10.2134/agronj2010.0394
- Gathala, M. K., Laing, A. M., Tiwari, T. P., Timsina, J., Islam, S., Bhattacharya, P. M., Dhar, T., Ghosh, A., Sinha, A. K., Hossain, S., Hossain, I., Molla, S., Rashid, M., Kumar, S., Kumar, R., Chaudhary, B., Jha, S. K., Ghimire, P., Bastola, B., … Gerard, B. (2020a). Energy-efficient, sustainable crop production practices benefit smallholder farmers and the environment of the Eastern Gangetic Plains, South Asia. Journal of Cleaner Production, 246, 118982. https://doi.org/10.1016/j.jclepro.2019.118982
- Gathala, M. K., Laing, A. M., Tiwari, T. P., Timsina, J., Islam, M. S., Chowdhury, A. K., Chattopadhyay, C., Singh, A. K., Bhatt, B. P., Shrestha, R., Barma, N. C. D., Rana, D. S. J., Gérard, B, T. M., & Gerard, B. (2020b). Enabling smallholder farmers to sustainably improve their food, energy and water nexus while achieving environmental and economic benefits. Renewable and Sustainable Energy Reviews, 120, 1096. https://doi.org/10.1016/j.rser.2019.109645
- Gathala, M., Yadav, S., Mazid, M. A., Humphreys, E., Sharif, A., Krupnik, T., Rashid, M. H., Chauhan, B. S., Kumar, V., Russell, T., Saleque, M. A., Kamboj, B. R., Jat, M. L., Malik, R. K., Tiwari, T. P., Mondal, M., Rahman, M., Saha, A., Khaled, S., and McDonald, A. J. (2014). Guidelines for dry seeded aman rice (DSR) in Bangladesh IFAD and CSISA Joint Publication. International Rice Research Institute (IRRI) and the International Maize and Wheat Improvement Center (CIMMYT), 32 www.knowledgebank.irri.org.
- Gill, G., Bhullar, M. S., Yadav, A., & Yadav, D. B. (2013). Technology for successful production of direct seeded rice. A training manual based on the outputs of ACIAR (Australian Centre for International Agricultural Research) funded project CSE/ 2004/033. A Joint Publication of University of Adelaide. 32. Punjab Agricultural University Ludhiana, Punjab; CCS Haryana Agricultural University, Hisar, Haryana.
- Green, J. M., & Owen, M. D. K. (2011). Herbicides resistant crops: Utilities and limitations for herbicide resistant weed management. Journal of Agricultural and Food Chemistry, 59(11), 5819–5829. https://doi.org/10.1021/jf101286h
- Gupta, R. K., Naresh, R. K., Hobbs, P. R., Jiaguo, Z., & Ladha, J. K. (2003). Sustainability of post green revolution agriculture: The rice-wheat cropping systems of Indo- Gangetic plains and China. In J. K. Ladha, J. E. Hill, J. M. Duxbury, R. K. Gupta, & R. J. Buresh (Eds.), Improving the productivity and sustainability of rice-wheat systems: Issues and impacts (Vol. 65, pp. 1–25. ASA Special Publication.
- Haque, M. E., & Bell, R. W. (2019). Partially mechanized non-puddled rice establishment: On-farm performance and farmers’ perceptions. Plant Production Science, 22(1), 23–45. https://doi.org/10.1080/1343943X.2018.1564335
- Hossain, K., Timsina, J., Johnson, D., Gathala, M. K., & Krupnik, T. J. (2020). Multi-year weed community dynamics and rice yields as influenced by tillage, crop establishment, and weed control: Implications for rice-maize rotations in the eastern Gangetic plains. Crop Protection, 138, 105334. https://doi.org/10.1016/j.cropro.2020.105334
- Islam, S., Gathala, M. K., Tiwari, T. P., Timsina, J., Laing, A. M., Maharjan, S., Chowdhury, A. K., Bhattacharya, P. M., Dhar, T., Mitra, B., Kumar, S., Srivastwa, P. K., Dutta, S. K., Shrestha, R., Manandhar, S., Sherestha, S. R., Paneru, P., Siddquie, N., Hossain, A., Gerard, B. (2019). Conservation agriculture based sustainable intensification: Increasing yields and water productivity for smallholders of the Eastern Gangetic Plains. Field Crops Research, 238, 1–17. https://doi.org/10.1016/j.fcr.2019.04.005
- Jat, H. S., Kumar, V., Kakraliya, S. K., Abdallah, A. M., Datta, A., Choudhary, M., Gathala, M. K., McDonald, A. J., Jat, M. L., & Sharma, P. C. (2021). Climate-smart agriculture practices influence weed density and diversity in cereal-based agri-food systems of western Indo-Gangetic plains. Scientific Reports, 11(1), 15901. https://doi.org/10.1038/s41598-021-95445-1
- Kaur, S., & Singh, S. (2015). Bio-efficacy of different herbicides for weed control in direct-seeded rice. Indian Journal of Weed Science, 47, 106–109.
- Khaliq, A., & Matloob, A. (2012). Germination and growth response of rice and weeds to herbicides under aerobic conditions. International Journal of Agriculture and Biology, 14, 775–780.
- Khaliq, A., Matloob, A., Ahmad, N., Rasul, F., & Awan, I. U. (2012). Post emergence chemical weed control in direct seeded fine rice. Journal of Animal and Plant Sciences, 22, 1101–1106.
- Kim, K. U. (1996). Ecological forces influencing weed competition and herbicide resistance. In R. In) Naylor (Ed.), Herbicides in Asian rice:Transitions in weed management (pp. 129–142). Stamford University.
- Kumar, V., & Ladha, J. K. (2011). Direct-seeding of rice: Recent developments and future research needs. Advances in Agronomy, 111, 297–413. https://doi.org/10.1016/B978-0-12-387689-8.00001-1
- Mahajan, G., & Chauhan, B. S. (2013). Herbicide options for weed control in dry-seeded aromatic rice in India. Weed Technology, 27(4), 682–689. https://doi.org/10.1614/WT-D-13-00016.1
- Mahajan, G., & Chauhan, B. S. (2015). Weed control in dry direct-seeded rice using tank mixtures of herbicides in South Asia. Crop Protection, 72, 90–96. https://doi.org/10.1016/j.cropro.2015.03.002
- Mahajan, G., Chauhan, B. S., & Johnson, D. E. (2009). Weed management in aerobic rice in Northwestern Indo-Gangetic Plains. Journal of Crop Improvement, 23(4), 366–382. https://doi.org/10.1080/15427520902970458
- Mahajan, G., Ramesha, M. S., & Chauhan, B. S. (2014). Response of rice genotypes to weed competition in dry direct-seeded rice in India. Scientific World Journal, 641589, 8. https://doi.org/10.1155/2014/641589
- Maity, S. K., & Mukherjee, P. K. (2009). Integrated weed management practices in dry direct seeded summer rice (Oryza sativa). Indian Journal of Agricultural Sciences, 79, 976–979.
- Mitra, B., Patra, K., Bhattacharya, P. M., & Chowdhury, A. K. (2018). Unpuddled transplanting: A productive, profitable and energy efficient establishment technique in rice under Eastern sub-Himalayan plains. ORYZA- an International Journal on Rice, 55(3), 459–466. https://doi.org/10.5958/2249-5266.2018.00055.3
- Mukherjee, P. K., Sarkar, A., & Maity, S. K. (2008). Critical period of crop-weed competition in transplanted and wet-seeded Kharif rice (Oryza sativa L.) under Terai conditions. Indian Journal of Weed Science, 40(3 & 4), 147–152.
- Pal, S., Ghosh, R. K., Banerjee, S., Kundu, R., & Alipatra, A. (2012). Effect of pyrazosulfuron-ethyl on yield of transplanted rice. Indian Journal of Weed Science, 44, 210–213.
- Patra, K., Singha, P., & Mitra, B. (2018). Performance of different rice and wheat varieties under alternate crop establishment techniques in rice-wheat rotation. Journal of Crop and Weed, 14(3), 31–40. http://cwssbckv.org/Journal/default.aspx
- Pattanayak, S., Jena, S., Das, P., Maitra, S., Shankar, T., Praharaj, S., Mishra, P., Mohanty, S., Pradhan, M., Swain, D. K., Pramanick, B., Gaber, A., & Hossain, A. (2022). Weed management and crop establishment methods in rice (Oryza sativa L.) influence the soil microbial and enzymatic activity in sub-tropical environment. Plants (Basel), 11(8), 1071. https://doi.org/10.3390/plants11081071
- Rahman, M. (2016). Sustainable weed management for unpuddled transplanted rice. Advances in Plants & Agriculture Research, 5(2), 00175. https://doi.org/10.15406/apar.2016.05.00175
- Rajiv, N., Vikram, S., Shankar, S. S., Virender, K., Krishna, H. K., Prasad, N. C., Pal, P. S., Malik, R. K., Kumar, S. S., & Kumar, S. P. (2018). Comparative assessment of different tillage-cum-crop establishment practices and crop-residue management on crop and water productivity and profitability of rice (Oryza sativa)-wheat (Triticum aestivum) cropping system. Indian Journal of Agricultural Sciences, 63(1).
- Ramesha, Y. M., Umesh, M. R., Anand, S. R., Bhanuvally, M., & Gaddi, A. K. (2017). Herbicide sequence for weed management in direct seeded rice. Journal of Applied and Natural Science, 9(3), 1866–1870. https://doi.org/10.31018/jans.v9i3.1454
- Rao, A. N., Johnson, D. E., Sivaprasad, B., Ladha, J. K., & Mortimer, A. M. (2007). Weed management in direct-seeded rice. Advances in Agronomy, 93, 153–255. https://doi.org/10.1016/S0065-2113(06)93004-1
- Saha, S., Munda, S., Singh, S., Kumar, V., Jangde, H. K., Mahapatra, A., & Chauhan, B. S. (2021). Crop establishment and weed control options for sustaining dry direct seeded rice production in eastern India. Agron, 11, 389. https://doi.org/10.3390/agronomy1102038
- Sahu, R., Mandal, S. K., Sharda, K., Kumar, D., Sahu, J., Prasad, M., Sohane, R. K., & Kumar, R. (2019). Crop residues management with different crop establishment methods in rice (Oryza sativa L.)-wheat (Triticum aestivumL.) cropping system. Current Applied Science and Technology, 37(6), 1–8. https://doi.org/10.9734/CJAST/2019/v37i630322
- Singh, M., Bhullar, M. S., & Chauhan, B. S. (2015). Influence of tillage, cover cropping, and herbicides on weeds and productivity of dry direct-seeded rice. Soil and Tillage Research, 147, 39–49. https://doi.org/10.1016/j.still.2014.11.007
- Singh, V., Jat, M. L., Ganie, Z. A., Chauhan, B. S., & Gupta, R. K. (2016). Herbicide options for effective weed management in dry direct-seeded rice under scented rice-wheat rotation of western Indo-Gangetic Plains. Crop Protection (Guildford, Surrey), 81, 168–176. https://doi.org/10.1016/j.cropro.2015.12.021
- Singh, R. G., Singh, S., Singh, V., & Gupta, R. K. (2010). Efficacy of azimsulfuron applied alone and tank mixed with metsulfuronþchlorimuron (almix) in dry direct seeded rice. Indian Journal of Weed Science, 42.168–172.
- Sinha, A. K., Ghosh, A., Dhar, T., Bhattacharya, P. M., Mitra, B., Rakesh, S., Paneru, P., Shrestha, S. R., Manandhar, S., Beura, K., Dutta, S., Pradhan, A. K., Rao, K. K., Hossain, A., Siddiquie, N., Molla, M. S. H., Chaki, A. K., Gathala, M. K., Islam, M. S., … Menzies, N. W. (2019). Trends in key soil parameters under conservation agricultural-based sustainable intensification farming practices in the Eastern Ganga alluvial plains. Soil Research. https://doi.org/10.1071/SR19162
- Walia, U. S., Walia, S. S., Sidhu, A. S., & Nelly, S. (2012). Bioefficacy of pre-and post-emergence herbicides in direct seeded rice in central Punjab. Indian Journal of Weed Science, 44, 33.
- Zahan, T., Hashem, A., Rahman, M., Bell, R. W., & Begum, M. (2018). Efficacy of herbicides in non-puddled transplanted rice under conservation agriculture systems and their effect on the establishment of the succeeding crops. Acta Scientifica Malaysia (ASM), 2(1), 17–25. http://doi.org/10.26480/asm.01.2018.17.25