Abstract
Commercial orchid industry is a lucrative business worldwide. New varieties and hybrids are being developed hastily in a race to keep up with the ever-growing demand for novelty and quality of flowers. The orchid exports contribute a lion’s share of the worldwide cutflower and plant export industry. Cymbidium mosaic virus (CymMV) is a major threat to the orchid industry as it is mechanically transmissible and quarantined in many countries. This study evaluates the effectiveness of the novel root endophyte Piriformospora indica (syn. Serendipita indica) when integrated with commonly used antiviral chemicals and defense inducing compounds to effectively control CymMV in commercially cultivated Dendrobium orchids. The effectiveness of each treatment was assessed by observing the reduction of CymMV titer using DAC-ELISA. Plants treated with Virus Ex @1.0 ml L−1foliar (antiviral formulation) and combination treatment with P. indica root inoculation and Virus Ex −1 ml L−1foliar spray showed significantly lower viral titer than other treatments. This suggests that P. indica can be used as an effective and easy treatment method that can be adopted by farmers and orchid growers in combination with regular antiviral formulations to control of CymMV.
1. Introduction
Growing orchids for flowers and decoration is an ever-growing multimillion business worldwide (Chandra De et al., Citation2015; Lepcha et al., Citation2020). Orchids are often thought as a symbol of beauty, elegance, love and hospitality. The South East Asian countries are a major hub for cultivation and export of orcihds. Among orchids, Dendrobium spp. is the most popular and preferred orchid in the cutflower industry. Other popular orchids include Cattleya, Cymbidium, Dendrobiums, Odontoglossum and Vanda. These are commercially cultivated epiphytic orchids with a large number of varieties and hybrids (Chandra De et al., Citation2015). Two major orchid viruses Cymbidium mosaic virus (CymMV) and Odontoglossum ringspot virus (ORSV) have been causing severe economic losses to the commercial orchid industry. The infected plants are often rejected during quarantine. The viruses had detrimental impacts on the growth and development of orchids (Bratsch et al., Citation2021). Symptomatic orchids are poor developed with smaller sized and necrotic flowers. Leaves show blighted appearance thereby reducing their market value (Seoh et al., Citation1998). Both CymMV and ORSV are transmissible through agricultural tools and implements that have come in contact with infected plant sap (Moles et al., Citation2007). CymMV and ORSV were detected in cultivated varieties of Bulbophyllum,Cattleya, Cymbidium, Cymbidium, Dendrobium, Epidendrum, Oncidium, Phalaenopsis, Paphiopedilum, Rhynchostylis, Vanda and Vanilla in India (Chandra De et al., Citation2015; Pant et al., Citation2010; Sherpa et al., Citation2007). Detection of viral disease in plants during import or export may end up in huge losses to the plant trader. The entire lot of plants may get rejected due to strict phytosanitary regulations. Virus disease with incidence varying from 5% to 77.58% was recorded from major orchid growing regions around the world (Safeer & Umamaheswaran, Citation2021; Sherpa et al., Citation2007; F. X. Liu et al., Citation2013). Even though there are strict regulations and standard quarantine procedures imposed in export of orchids, many diseases and pest infestations occur through these multiple checkpoints. It is not uncommon for orchid viruses to pass through the regular quarantine procedures undetected as asymptomatic plants and can be later detected in many orchid nurseries (Safeer & Umamaheswaran, Citation2021). Piriformospora indica is a root endophytic fungus discovered from the rhizosphere of desert plants in the Thar Desert of India (Verma et al., Citation1998). Researchers are discovering its multiple biological applications such as inducing rooting, hardening of tissue culture plantlets, protection against biotic and abiotic stress (Druege et al., Citation2007; Oelmüller et al., Citation2009; Roylawar et al., Citation2021; Shah et al., Citation2019). P. indica was also found to effectively improve the general root and shoot growth of tissue cultured Cymbidium aliofolium orchid plants (Shah et al., Citation2019). The plants that were root colonized by P. indica showed improved production of plant bioactive compounds such as ascorbic acid, auxins, D L- proline. And those plants were able to swiftly adapt during the hardening procedure (Shah et al., Citation2019). A better management method is the need of the hour for orchid viruses such as CymMV and ORSV. Antiviral chemicals that have been found to apparently combat viral diseases of orchids are available in the market and are widely used by orchid growers, but with conflicting results. This study uses the novel root entophyte P. indica in combination with commonly available antiviral chemicals and salts to get better control of CymMV in commercially cultivated Dendrobium orchids. Moreover, in the study analysis of plant defense related compounds such as peroxidase (PO), polyphenol oxidase (PPO) and phenylalanine ammonia-lyase (PAL) induced due to the treatments is also studied. Generally, in a plant system, the interaction between a pathogen or mycorrhizae causes certain changes in the production of enzymes related to the defense mechanism such as PO, PPO and many enzymes activated within the plant system (Kuvalekar et al., Citation2011; Song & Goodman, Citation2001). Plants in response to infection by viruses show an increase in activity of defence-related enzymes such as PO, PPO and PAL (Helal, Citation2019). Thus the increase in activity of peroxidases and polyphenol oxidases are directly correlated to the development of resistance against the pathogen. In a similar study Clarke et al. (Citation2002), treated leaves Phaseolus vulgaris with plant hormones such as dihydrozeatin, jasmonic acid and salicylic acid and analyzed the activities of plant enzymes such as catalase, glutathione reductase, peroxidase and superoxide dismutase. These enzymes play an important role in scavenging reactive oxygen species in virus infected plants. The result of the study suggests that the treatment with plant hormones increased the production of defence-related enzymes that actively hindered the replication of White clover mosaic virus (WClMV).
2. Materials and methods
CymMV was detected in Dendrobium by observing the symptoms expressed and its presence was confirmed by DAC-ELISA (using CymMV-specific antibodies obtained from Agdia Pvt Ltd. CAB89500/100) and transmission electron microscopy (Plant Virology unit, Indian Agricultural Research Institute, New Delhi; Figure ). In the present experiment, symptomatic Dendrobium orchid plants with a natural incidence of CymMV were used. The presence of the virus was confirmed by DAC-ELISA using a specific antibody for CymMV. Treatments were carried on the selected plants of uniform age, from a single lot. The initial and final titer value of CymMV in the leaf samples was recorded using DAC-ELISA by using CymMV-specific antibodies. The observed variation between initial and final optical density (OD) was considered to be the main criteria for finding the effectiveness of the treatments in controlling the disease. The field trial was carried out within a protected polyhouse of an orchid grower and the observations were recorded periodically for 180 days. The fungus P. indica was root inoculated in individual treatment as well in combination with antiviral formulation and defence inducing compounds.
3. Polyhouse experiment
The treatment included T1- Virus Ex @ 1 mL−1 foliar spray which is a commonly used antiviral formulation of monoterpic alcohols derived from botanical source, T2- and T3 were defence inducing compounds such as Acibenzolar-S- methyl @100 ppm and Salicylic acid @150 ppm applied as foliar spray. T4- P. indica root inoculated plants, T5, T6 and T7 were P. indica root inoculated plants with Virus Ex @ 1 mL−1, Salicylic acid @150 ppm and Acibenzolar-S- methyl @100 ppm, respectively, applied as foliar sprays (Table ). The present experiment was planned with the observations from a preliminary experiment carried out on Chenopodium amaranticolor plants using local lesion assay technique. The best treatments and combinations from the local lesion assay were included in the present study. The chemical formulations and root endophyte were found to be compatible at the given concentration without any phytotoxicity. The change in virus titer was analyzed at the end of 30 days after treatment (DAT) by drawing leaf samples from each treatment and analyzing them with DAC-ELISA CymMV-specific antibodies. The treatments were repeated at 30 day interval for 3 months and the change in virus titer at the end of field experiment was also analyzed. The field experiment was limited to three treatments as the post entry quarantine period for imported Dendrobium plants were 60 days and after this period, the plants would generally be shifted to other facilities to be sold off to orchid growers.
Table 1. Treatment applied on Dendrobium orchid plants to control Cymbidium mosaic virus
3.1. Root inoculation with P. indica
Root inoculation of Dendrobium by P. indica by using root dip method was standardized during the present study. A spore suspension of P. indica of 10−6cfu was prepared by macerating a 10-day-old culture of P. indica in Kaefer medium and adjusting it with sterile distilled water. Orchid plantlets with fresh root growth were selected for root inoculation. The orchid plants were carefully lifted from perforated pots, roots washed under running tap water and dried under shade for 24 hours without further addition of water. The Dendrobium plantlets were then dipped in spore suspension of P. indica with only the roots immersed in the suspension for 5 h. The plants were then carefully lifted and placed in plastic pots containing moistened sterile absorbent cotton at the base and along the sides. The root inoculated plants were placed in this condition for 48 h for P. indica to properly colonize the roots. The plants were then transferred to pots with a potting mixture containing a mixture of charcoal, broken pieces of brick/tiles, coconut husk (in 1:1:1 ratio) cut into suitable size/wood chips. The roots were excised, gently crushed and placed over a microscopic glass slide. The tissue was then stained using lactophenol cotton blue stain and observed under a light microscope after 5 days to confirm the colonization of P. indica in roots. The roots were observed in this method for a month to confirm the colonization of P. indica. The treatment plan was prepared based on the results of a preliminary assay that had a disease of CymMV. The plants were maintained inside insect-proof polyhouse conditions at 50% shade condition with regular watering for the entire observational period of 180 days.
3.2. Analysis of effect of treatments on CymMV infected Dendrobium plants
A sample of 1 gram of leaf tissue from each treated plants was collected and DAC-ELISA was carried out to determine the possible change in virus titer. The estimation procedure described by Huguenot et al. (Citation1993) was followed with minor modifications. The sample was homogenized in 5 ml coating buffer (carbonate buffer, 100 mM pH 9.6) containing 2 per cent (w/v) polyvinylpyrrolidone (PVP) maintained on cold conditions. Healthy leaf tissues were included as negative control, which were collected from healthy plants maintained under greenhouse were also homogenized and used as the negative control. The homogenate was filtered and centrifuged at 5000 rpm (2655 G) for 10 min at 4°C and this crude extract was used for analysis. Specific antibody for CymMV available at Advanced Research Center for Plant Disease Diagnosis, Department of Plant Pathology, College of Agriculture, (Thiruvananthapuram, Kerala) was used as primary antibody and anti-rabbit IgG-Alkaline phosphatase antibody produced in goat (Sigma-Aldrich, A3687) was used as a secondary antibody. The absorbance of samples was read at 405 nm in an ELISA reader (Microplate reader 680, BioRad). The final titer for each treatment was estimated by using the average of four replications. The significance between results from the OD value was analyzed by Analysis of Variance of Completely Randomized Design and pairwise comparison has been done by Tukey test by using the GRAPES software of Kerala Agricultural University (Gopinath et al., Citation2021). Graph no. 1 was plotted based on the results.
4. Estimation of defense related enzyme production
The estimation of defense-related enzymes such as PO, PPO and PAL were carried out to observe whether the induced resistance (IR) pathways were activated in the plant system as a result of the treatments.
4.1. Peroxidase (PO)
Samples for analysis of PO were collected from fully grown younger leaves at intervals, before the next set of treatments. Peroxidase activity in leaves was determined as per the procedure described by Srivastava (Citation1987) using 1 ml 0.05 M pyrogallol and 50 µl of plant enzyme extract using a spectrophotometer. The change in absorbance was measured at 30 second intervals. The activity of peroxidase was expressed in µg g−1min−1. The average value of four replications was taken and significance between treatments was analyzed by Analysis of Variance of Completely Randomized Design and pairwise comparison done with Tukey Honest test by using the GRAPES software of Kerala Agricultural University (Gopinath et al., Citation2021). Graph no. 2 was plotted based on the results from Table .
Table 2. Pairwise comparison of change in virus titer of Dendrobium plants as a result of various treatments detected by CymMV-specific antibody in DAC-ELISA
Table 3. Pairwise comparison of Peroxidase activity (µg g−1min−1) in Dendrobium plants as a result of treatment with resistance inducing compounds, antiviral formulation and Piriformospora indica
4.2. Confirmation of peroxidase isozymes by electrophoretic analysis
The overall presence of peroxidase isozymes in plants was assessed at 30 days after treatments (DAT) during the period of experiment. Isoforms of peroxidase enzymes from orchid leaves were analysed by using anionic PAGE, with the procedure described by Wagih and Coutts (Citation1982). Leaf sample each weighing 1 gram was taken from all treatments, macerated with 1:1 w/v, 50 mM tris-HCl (pH 7.6) in cold conditions to prevent dissociation of isozymes. The homogenate was then filtered with cheesecloth and then centrifuged at 15,000 rpm (22,640 G) for 10 min at 4°C. This supernatant was loaded into wells of 7.5% polyacrylamide gel prepared beforehand. The gel was prepared by a similar procedure as in PAGE except for the addition of 2% Triton X-100 in place of SDS. The electrophoresis was performed at 60 V in the initial phase, later increased to 100 V. After the protein has run for 3/4th of the gel block, the electrophoresis unit was disconnected and the gel incubated at room temperature for 20–30 min in 0.6 M sodium acetate buffer (pH 5.4) containing 0.5% O- dianisidine HCl. The gel block was then transferred to 0.1 M hydrogen peroxide for the development of visible bands of isozyme. The relative mobility (Rm) of visible bands was then calculated and photographed under win a Gel documentation system (Gel DOC TM XR+) available at ARCPDD, Department of Plant Pathology, College of Agriculture, Vellayani (Figure ).
4.3. Polyphenol oxidase (PPO)
Polyphenol oxidase activity was estimated according to the procedure described by Mayer et al. (Citation1965) using similar procedures of estimation of peroxidase. The PPO activity of leaf samples was analyzed at specific intervals after application of treatments. The enzymatic activity was expressed as the change in the absorbance of the reaction mixture per minute per gram on fresh weight basis. The average value of four replications was taken and significance between treatments was analyzed by Analysis of Variance of Completely Randomized Design and pairwise comparison done using Tukey Honest test by using the GRAPES software of Kerala Agricultural University (Gopinath et al., Citation2021). Graph no. 3 was plotted based on the results from Table .
Table 4. Pairwise comparison of Polyphenol oxidase activity (µg g−1min−1) in Dendrobium plants as a result of treatment with resistance inducing compounds, antiviral formulation and Piriformospora indica
4.4. Phenylalanine ammonialyase (PAL)
PAL activity was analysed based on the procedure described by Dickerson et al. (Citation1984) using 0.1 M sodium borate buffer (pH 8.8), 0.2 ml enzyme extract and 0.1 ml of 12 mM l-phenylalanine prepared in the same buffer. The reaction mixture and blank was incubated at 40°C for 30 min and the reaction was stopped by adding 0.2 ml of 3 N HCl. The absorbance was read at 290 nm in a spectrophotometer. PAL activity was expressed as micrograms of cinnamic acid produced per min per gram on a fresh weight basis. The average value of four replications was taken and significance between treatments was analyzed by Analysis of Variance of Completely Randomized Design and pairwise comparison done using Tukey Honest test by using the GRAPES software of Kerala Agricultural University (Gopinath et al., Citation2021). Graph no. 4 was plotted based on the results from Table .
Table 5. Pairwise comparison of Phenylalanine ammonia-lyase activity (µg g−1min−1) in Dendrobium plants as a result of treatment with resistance inducing compounds, antiviral formulation and Piriformospora indica
5. Results
5.1. Detection of virus
CymMV infected Dendrobium plants showed typical mosaic patterns on leaves, whereas no visible symptoms were observed on stems, roots or flowers (Figure ). The young leaves showed streaks with well-defined margins which, during later stage, turns chlorotic and covers the entire leaf surface. Severely infected plants had blighted appearance and overall stunting with less or almost no flowers. The presence of CymMV in Dendrobium plants was detected with the help of DAC- ELISA. The transmission electron microscopy analysis showed viral particles with single, non-enveloped flexuous rod shaped with 400–1000 nm in length (Figure ). The electron micrograph confirmed the morphological characteristics of CymMV as described by Jensen and Gold (Citation1955).
5.2. Root colonization of P. indica
Intercellular mycelium of P. indica was observed in 5 days after inoculation. Superficial mycelial growth was found to appear on roots and at 20 days post-inoculation, indicating successful root colonization. Sporulation was observed in cortical cells at 30 days post-inoculation, when observed under light microscope with Lactophenol cotton blue stain. The fungus colonized the epidermal and cortical region of Dendrobium roots in greenhouse conditions with ample moisture and 50% sunlight (Figure ).
5.3. Effect of treatments on CymMV infected Dendrobium plants
The Dendrobium plants at 30 DAT showed significant change in virus titer compared to untreated control plants. At 30 DAT; T1- Virus Ex (1.0 ml L−1) foliar spray, T4- P. indica root inoculation, T5- P. indica root inoculation + Virus Ex (1 ml L−1) foliar spray and T7- P. indica root inoculation + Acibenzolar-S-methyl (100 ppm) foliar spray showed significantly lowest virus titer (Table no. 2). By the end of the field experiment T1- Virus Ex (1.0 ml L−1) foliar spray, T4- P. indica root inoculation, T5- P. indica root inoculation + Virus Ex (1 ml L−1) foliar spray and showed significantly lowest virus titer (Table no. 2). The treatments T2-Acibenzolar-S-methyl (100 ppm) foliar spray, T3- Salicylic acid (150 ppm) foliar spray and T6- P. indica (10−6cfu) root inoculation + Salicylic acid (150 ppm) foliar spray did not show a significant reduction in virus titer at 30 DAT and at the time of completion of field experiment (Table ; Graph 1). No visible change in symptoms was observed in Dendrobium plants at 30 DAT. Symptom remission was observed in all plants that were root inoculated with P. indica (T4, T5, T6 and T7) during the second application of treatments. A general increase in root and shoot growth was observed in P. indica inoculated plants by the end of field experiment.
5.4. Estimation of defense related enzyme production
5.4.1. Peroxidase activity
Peroxidase activity varied widely during the period of treatment and was generally highest during first 10 days of application of treatments. The overall activity came down at 30 days after treatment. The highest level of PO activity was observed on 2 DAT and 5 DAT, in the treatments T1-Virus Ex (1 mlL−1foliar spray), T6- P. indica root inoculation + Salicylic acid (150 ppm) spray, T7-P. indica root inoculation + Acibenzolar-S-methyl (100 ppm) spray and T5—P. indica root inoculation + Virus Ex (1 mlL−1) foliar spray. At 2 DAT; T1- Virus Ex (1 ml L−1foliar spray) showed activity of 24.76 µg g−1min−1 which was significantly higher than all other treatments. Whereas at 5 DAT, T6- P. indica root inoculation + Salicylic acid (150 ppm) spray showed highest significant activity of 26.09 µg g−1min−1 (Table ; Graph 2)
Graph 2. Peroxidase activity (µg g−1min−1) in Dendrobium plants as a result of treatment with resistance inducing compounds, antiviral formulation and Piriformospora indica.
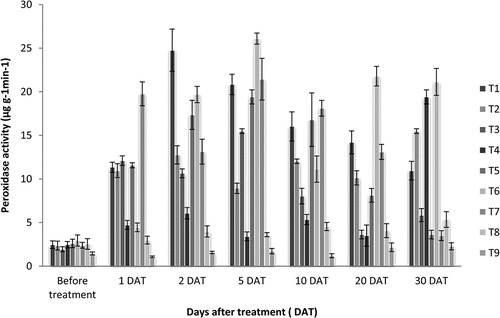
The enzymatic activity of PO was found higher at 30 DAT when compared to the values at 20 DAT for T2- Acibenzolar-S-methyl (100 ppm) foliar spray and T4- P. indica (106 cfu) root inoculation. The plant samples from treatments T2 and T4 and T6 showed sudden increase in PO activity at 30 DAT which could not be detected by anionic PAGE analysis for peroxidase isoforms. The relative mobility (Rm) of peroxidase isoforms at 30 days after treatment did not show much variation to the value of untreated plant. The reason for this sudden increase in PO activity in T2 and T4 and T6 could be attributed to the influence by any unknown biotic or abiotic factors. Polyphenol oxidase activity
The overall PPO activity in plants reached its peaked 2 DAT and gradually reduced by 20 DAT. At 2 DAT, the enzyme activity in plants treated with T2 -Acibenzolar-S-methyl-100 ppm (4.014 µg g−1min−1) and T3- Salicylic acid—150ppm (3.956 µg g−1min−1) were significantly higher than all treatments. On 5 DAT, plants treated with T2 -Acibenzolar-S-methyl-100 ppm (3.433 µg g−1min−1) showed significantly higher activity compared to other treatments. On 10 DAT also, treatments with ASM-100 ppm (2.550 µg g−1min−1) and Salicylic acid (150ppm spray) (2.995 µg g−1min−1) recorded the highest activity and were significantly highest (Graph 3). At 30 DAT, the plants with T4 -P. indica (106cfu) root inoculation (2.040 µg g−1min−1) and T7- P. indica root inoculation + ASM (100 ppm spray) (2.015 µg g−1min−1) showed significantly higher activity than other treatments (Table ; Graph 3).
5.4.2. Phenylalanine ammonia-lyase activity
The overall PAL activity in treatments increased at 1 DAT and gradually reduced by the 10 DAT. At 1 DAT and 2 DAT the plants treated with; T1- Virus Ex (1 ml L−1foliar spray), T5- P. indica root inoculation + Virus Ex (1 ml L−1) foliar spray and T7- P. indica root inoculation + Acibenzolar-S-methyl (100 ppm) foliar spray showed highest activity. At 30 DAT, the activity of PAL was lower in all samples and similar to that of the condition before treatment application (Table ; Graph 4).
6. Discussion
The use of antiviral principles is widely adopted among farmers in controlling many viral diseases in agricultural and horticultural crops (Sangeetha et al., Citation2020; Elbeshehy, Citation2017). This study demonstrated the use of P. indica as a promising biocontrol technique to manage CymMV in Dendrobium orchids. When applied as root inoculation, along with antiviral compounds, P. indica was found to effectively reduce CymMV in Dendrobium orchids. The effects of P. indica have been well defined by researchers as it improves the physical and physiological conditions in epiphytic orchids like Cymbidium sp. (Shah et al., Citation2019). In the present study, P. indica was found to successfully colonize the fleshy tender roots of Dendrobium orchids in just 5 days and develop sporulation in 30 days after inoculation. Root colonization of the fungus in Oncidium sp. was achieved in just three days after inoculation by H. Liu et al. (Citation2019). Both Dendrobium sp. and Oncidium sp. are epiphytic and are grown in similar conditions in greenhouses. As observed in our present study as well as in the previous studies mentioned above, it can be concluded that the use of P. indica for root inoculation is a viable treatment option for both epiphytic as well as terrestrial orchids. In our study, Dendrobium plants that were root inoculated with P. indica, foliar treatment with the antiviral formulation Virus Ex (1 ml L−1) and the combination of P. indica (106 cfu) root inoculation + Virus Ex (1 ml L−1) foliar spray were found to be effective in reducing the CymMV titer in Dendrobium plants. The effectiveness of using formulated plant antiviral products rather than crude plant extracts have been emphasized in many previous studies and many attempts have been carried out to purify and identify these compounds (Mukhtar et al., Citation2008; Ong, Citation2004). The extracts of Azadirachta indica and Bougainvillea spectabilis applied as pre-inoculation treatment followed by foliar spray with extracts of Thuja orientalis extract and Nigella sativa was found to reduce Zucchini yellow mosaic virus (ZYMV) in watermelon. The experiment showed an overall reduction of viral load and symptom expression of ZYMV in watermelon plants. The reduction in concentration of ZYMV in leaves of watermelon plants after application of antiviral plant extracts was determined by DAS-ELISA at 21 days post inoculation (dpi; Elbeshehy, Citation2017). The effect of the formulated antiviral principle Virus Ex in inducing defence related enzymes was observed to be of short duration except for PO. The PPO and PAL activity in Dendrobium plants treated with Virus Ex (1 ml L−1) was reduced on 10 DAT. Whereas the plants showed better control of CymMV and recovery from disease. Plants that were root inoculated with P. indica also showed similar trend. In the combination treatment of P. indica root inoculation + Virus Ex (1 ml L−1) foliar spray, a higher overall activity of PO, PPO and PAL was observed in comparison to plants treated with P. indica alone. This combination treatment of formulated antiviral compound and bioagent also showed better disease control in Dendrobium plants. Oxidative pathways are often triggered in plants, during viral infection or when treated with certain chemicals. These pathways may have certain deleterious effect on overall growth of plants (Qamer et al., Citation2021; Seyfferth & Tsuda, Citation2014). In the present study the plant growth promoting bioagent P. indica, effectively helped the Dendrobium plants to overcome stress induced due to virus infection. In our observation at 150 days post first treatment, the plants that were root inoculated with P. indica showed lower virus titer as well as better growth in comparison with those plants treated with IR inducing compounds. The use of bioagents in management of plant viral diseases have given consistent results in many studies as early as 1990s. Sofy et al. (Citation2019), in their work, quotes many previous studies that uses mostly bacterial agents that are very effective in managing plant viral diseases. G.s et al. (Citation1996) observed that tomato plants can obtain resistance by means of ISR when they are treated with plant growth promoting rhizobacteria (PGPR). The overall fruit yield was higher, even though a high disease carrying vector population was observed in the field. In a similar study, Murphy et al. (Citation2003), demonstrated that a combination of PGPRs can lower disease severity of Cucumber mosaic virus (CMV) in tomato seedlings and improve the overall growth of the plants. Similarly, Kandan et al. (Citation2005) used different strains of Pseudomonas fluorescens as the bio-agent to effectively reduce the incidence of Tomato spotted wilt virus (TSWV) in tomato plants. Our study demonstrates that combination of a bio-agent with antiviral formulation gave promising results in controlling virus disease of Dendrobium orchid. Mishra et al. (Citation2014a), successfully combines a bioagent Pseudomonas sp. and an elicitor chitosan for better management of Tomato leaf curl virus in open field conditions. The supplementation of chitosan with Pseudomonas sp. induced a higher PO, PPO and PAL in tomato plants comCitationpared to the diseased control. The induction of PO, PPO and PAL enzymes in plant system is often associated to increase in resistance through ISR (Mishra, Jagadeesh, Krishnaraj, Prem et al., Citation2014b). Peroxidases are produced as part of complex oxidative bursts in plants due to biotic and abiotic stress (Jaiswal et al., Citation2013; Jouili et al., Citation2011; Miao et al., Citation2006; Passardi et al., Citation2004). Montalbini et al. (Citation1995), observed that the activity defence-related enzyme PO increases in plants during virus infections. Similarly, high activity of ascorbate peroxidase (APX) and guaiacol peroxidase (GPX) was observed in pumpkin plants infected by Pumkin yellow vein mosaic virus, when compared to uninfected plants (Jaiswal et al., Citation2013). In the present experiment, all the virus infected plants showed slightly higher enzymatic when compared to the healthy plants. The Dendrobium plants treated with antiviral formulation and defence inducing compounds showed much higher increase in the activity of PO, PPO and PAL than untreated diseased control plants. But the significant increase in activity of all the three enzymes came down to near normal condition at 30 DAT. Nurcahyani et al. (Citation2016), in their study, found an increasing peroxidase activity in ground orchid with increasing concentration of fusaric acid. Therefore, a general reduction of PO activity in plants that have once been infected by a pathogen can be related to a reduction in the activity of the pathogen and activation of defence mechanism. In the present study, the change in activity of defence-related enzymes was comparatively stable in plants that were root inoculated with P. indica. Whereas many strains of PGPRs induce the accumulation of PO, PPO, PAL and other defence-related compounds in plants in order to enhance plant defence against pathogens (Bukhat et al., Citation2020; Ramamoorthy et al., Citation2001) . This trend suggests that the disease control strategy due to association of P. indica in Dendrobium orchid may differ from that of other PGPRs. Further molecular studies are required to properly understand the mode of action of P. indica in Dendrobium orchids and its role in managing CymMV. The present study reveals that P. indica can be used as an effective biological control agent in Dendrobium orchids. The ease of application and prolonged effect on managing virus disease, compatibility with other chemical agents and maintaining plant health can make P. ind one of the best choices for orchid growers all over the world.
Acknowledgements
The authors are grateful towards orchid growers and importers of Kerala for their wholehearted support and providing infrastructure for the study. The research grant was provided by Kerala Agricultural University and laboratory facilities by ARCPDD, Department of Plant Pathology, College of Agriculture, Vellayani
Disclosure statement
No potential conflict of interest was reported by the authors.
Additional information
Funding
Notes on contributors
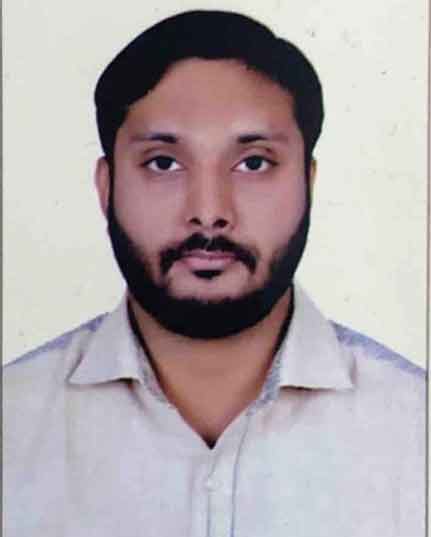
Safeer M M
Safeer M M working as Assistant Professor in Instructional Farm, College of Agriculture, Vellayani, Thiruvananthapuram dist., Kerala, India. He completed master’s degree in Plant Pathology in Kerala Agricultural University. He has a working experience of two years as Agricultural officer in the Department of Agriculture, Govt. of Kerala. The author is engaged in research, extension and teaching activity since the year 2021 in Kerala Agricultural University. The work on Cymbidium mosaic virus was done as a part of his Ph. D. research in Plant pathology, College of Agriculture Vellayani, Kerala Agricultural University.
Susha, S. Thara
Susha, S. Thara working as Assistant Professor (Plant Pathology) in Kerala Agricultural University is the recipient of Young Scientist Award from Kerala State Council for Science, Technology and Environment. Engaged in extension and research work in Agriculture since the year 2000. Guided many Post graduate and Ph. D Scholars and published about 25 research papers in Plant Pathology.
References
- Bratsch, S. A., Olszewski, N., & Lockhart, B. (2021). Incidence of Cymbidium mosaic, Odontoglossum ringspot, and orchid fleck virus in orchids in Minnesota and production of antibodies for use in ELISA to detect orchid fleck virus. European Journal of Plant Pathology, 159(3), 543–17. https://doi.org/10.1007/s10658-020-02185-5
- Bukhat, S., Imran, A., Javaid, S., Shahid, M., Majeed, A., & Naqqash, T. (2020). Communication of plants with microbial world: Exploring the regulatory networks for PGPR mediated defense signaling. Microbiological Research, 238, 126486. https://doi.org/10.1016/j.micres.2020.126486
- Chandra De, L., Promila, P., Rao, A. N., & Rajeevan, P. K. (2015). “2 global orchid industry”. Commercial Orchids. De Gruyter Open Polandhttps://doi.org/10.2478/9783110426403.2
- Clarke, S. F., Guy, P. L., Burritt, D. J., & Jameson, P. E. (2002). Changes in the activities of antioxidant enzymes in response to virus infection and hormone treatment. Physiologia Plantarum, 114(2), 157–164. https://doi.org/10.1034/j.1399-3054.2002.1140201.x
- Dickerson, D. P., Pascholati, S. F., Hagerman, A. K., Butler, L. G., & Nicholson, R. L. (1984). Phenylalanine ammonia lyase hydroxyl cinnmate: COA maydis or ligase in maize mesocotyls inoculated with Helminthosporium maydis or Helminthosporium carbonum . Physiological. Plant Pathology, 25(2), 111–123. https://doi.org/10.1016/0048-4059(84)90050-X
- Druege, U., Baltruschat, H., & Franken, P. (2007). Piriformospora indica promotes adventitious root formation in cuttings. Scientia Horticulturae, 112(4), 422–426. https://doi.org/10.1016/j.scienta.2007.01.018
- Elbeshehy, E. K. F. (2017). Inhibitor activity of different medicinal plants extracts from Thuja orientalis, Nigella sativa L., Azadirachta indica and Bougainvillea spectabilis against Zucchini yellow mosaic virus (ZYMV) infecting Citrullus lanatus. Biotechnology & Biotechnological Equipment, 31(2), 270–279. https://doi.org/10.1080/13102818.2017.1279572
- Gopinath, P. P., Prasad, R., Joseph, B., & Adarsh, V. S. (2021). Collection of shiny apps for data analysis in agriculture. Journal of Open Source Software, 6(63), 3437. grapesAgri1: https://doi.org/10.21105/joss.03437
- G.s, R., Liu, L., Murphy, J. F., Tuzun, S., & Kloepper, J. W. (1996). Induced systemic resistance of cucumber and tomato against cucumber mosaic cucumovirus using plant growth-promoting rhizobacteria (PGPR). Plant Disease, 80(8), 891–894. https://doi.org/10.1094/PD-80-0891
- Helal, I. M. (2019). Use of biocides for controlling viral diseases that attack common bean and cucumber plants. Folia Hort, 31(1), 159–170. https://doi.org/10.2478/fhort-2019-0011
- Huguenot, C., Furneaux, M. T., Thottappilly, G., Rossel, H. W., & Hamilton, R. I. (1993). Evidence that cowpea aphid-borne mosaic and Blackeye cowpea mosaic viruses are two different potyviruses. Journal of General Virology, 74(3), 335–340. https://doi.org/10.1099/0022-1317-74-3-335
- Jaiswal, N., Singh, M., Dubbey, R. S., Venkataramanappa, V., & Datta, D. (2013). Phytochemicals and antioxidative enzymes defense mechanism on occurrence of yellow vein mosaic disease of pumpkin (Cucurbita moschata). Biotechnology, 3(4), 287–295. https://doi.org/10.1007/s13205-012-0100-6
- Jensen, D. D., & Gold, A. H. (1955). Hosts, transmission, and electron microscopy of Cymbidium mosaic virus with special reference to Cattleya leaf necrosis. Phytopathol, 45(6), 327–334.
- Jouili, H., Bouazizi, H., & El Ferjani, E. (2011). Plant peroxidases: Biomarkers of metallic stress. Acta Physiologiae Plantarum, 33(6), 2075–2082. https://doi.org/10.1007/s11738-011-0780-2
- Kandan, A, Ramiah, Vasanthi, V. J., Radjacommare, R, Nandakumar, R, Ramanathan, A, Samiyappan, R, 2005 Use of Pseudomonas fluorescens-based formulations for management of tomato spotted wilt virus (TSWV) and enhanced yield in tomato. Biocontrol Science and Technology,15(6), 553–569. https://doi.org/10.1080/09583150500088546
- Kuvalekar, A., Redkar, A., Gandhe, K., & Harsulkar, A. (2011). Peroxidase and polyphenol oxidase activities in compatible host–pathogen interaction in Jasminum officinale and Uromyces hobsoni: Insights into susceptibility of host. New Zealand Journal of Botany, 49(3), 351–359. https://doi.org/10.1080/0028825X.2011.569727
- Lepcha, A., Khade, S. D., & Roy, T. (2020). Economics of flower cultivation with special reference to orchid in hilly areas of West Bengal. Economic Affairs, 65(3), 395–400. https://doi.org/10.46852/0424-2513.3.2020.11
- Liu, F. X., Han, Y. C., Li, W. D., Shi, X. Q., Xu, W., & Lin, M. G. (2013). Incidence of Cymbidium mosaic virus and Odontoglossum ringspot virus affecting Oncidium orchids in Hainan Island, China. Crop Protection (Guildford, Surrey), 54, 176–180. https://doi.org/10.1016/j.cropro.2013.08.013
- Liu, H., Senthilkumar, R., Ma, G., Zou, Q., Zhu, K., Shen, X., Tian, D., Hua, M. S., Oelmüller, R., & Yeh, K. W. (2019). Piriformospora indica-induced phytohormone changes and root colonization strategies are highly host-specific. Plant Signaling and Behaviour, 14(9), 1632688. https://doi.org/10.1080/15592324.2019.1632688
- Mayer, A. M., Harel, E., & Shaul, R. B. (1965). Assay of catechol oxidase, a critical comparison of methods. Phytochem, 5(4), 783–789. https://doi.org/10.1016/S0031-9422(00)83660-2
- Miao, Y., Lv, D., Wang, P., Wang, X. C., Chen, J., Miao, C., & song, C. P. (2006). An Arabidopsis glutathione peroxidase functions as both a redox transducer and a scavenger in abscisic acid and drought stress responses. The Plant Cell, 18(10), 2749–2766. https://doi.org/10.1105/tpc.106.044230
- Mishra, S., Jagadeesh, K. S., Krishnaraj, P. U., & Arasu, M. V. (2014a). Histological and Histochemical changes induced by amendment of chitosan and inoculation of Pseduomonas sp. against Tomato leaf curl virus (ToLCV) in tomato (Lycopersicon esculentum Mill. African Journal of Microbiology Research, 8(40), 3540–3550. https://doi.org/10.5897/AJMR2014.6847
- Mishra, S., Jagadeesh, K. S., Krishnaraj, P. U., & Prem, S. (2014b). Biocontrol of tomato leaf curl virus (ToLCV) in tomato with chitosan supplemented formulations of Pseudomonas sp. under field conditions. Australian Journal of Crop Science, 8(3), 347–355. https://www.cropj.com/mishra_8_3_2014_347_355.pdf
- Moles, M., Delatte, H, H., Farreyro, K., & Grisoni, M, M. (2007). Evidence that Cymbidium mosaic virus (CymMV) isolates divide into two subgroups based on nucleotide diversity of coat protein and replicase genes. Archives of Virology, 152(4), 705–715. https://doi.org/10.1007/s00705-006-0897-6
- Montalbini, P., Buonaurio, R., & Kumar, N. U. (1995). Peroxidase activity and isoperoxidase pattern in tobacco leaves infected with tobacco necrosis virus and other viruses inducing necrotic and non‐necrotic alterations. Journal of Phytopathology, 143(5), 295–301. https://doi.org/10.1111/j.1439-0434.1995.tb00263.x
- Mukhtar, M., Arshad, M., Ahmad, M., Pomerantz, R. J., Wigdahl, B., & Parveen, Z. (2008). Antiviral potentials of medicinal plants. Virus Research, 131(2), 111–120. https://doi.org/10.1016/j.virusres.2007.09.008
- Murphy, J. F., Reddy, M. S., Ryu, C. M., Kloepper, J. W., & Li, R. (2003). Rhizobacteria-Mediated growth promotion of tomato leads to protection against Cucumber mosaic virus. Phytopathol, 93(10), 1301–1307. https://doi.org/10.1094/PHYTO.2003.93.10.1301
- Nurcahyani, E., Agustrina, R., Suroso, E., & Andari, G. (2016). Analysis of peroxidase activity and total phenol from Spathoglottis plicata. Bl. Plantlet Towards Fusarium Oxysporum International Journal of Agricultural and Applied Sciences, 2(6), 79–82. https://doi.org/10.11648/j.ijaas.20160206.11
- Oelmüller, R., Sherameti, I., Tripathi, S., & Varma, A. (2009). Piriformospora indica, a cultivable root endophyte with multiple biotechnological applications. Symbiosis, 49(1), 1–17. https://doi.org/10.1007/s13199-009-0009-y
- Ong, E. S. (2004). Extraction methods and chemical standardization of botanicals and herbal preparations. Journal of Chromatography B: Analytical Technologies in the Biomedical and Life Sciences, 812(1–2), 23–33. https://doi.org/10.1016/S1570-0232(04)00647-6
- Pant, R. P., Das, M., Pun, K. B., Ramachandran, P., & Medhi, R. P. (2010). Occurrence of Cymbidium mosaic and odontoglossum ringspot viruses in orchid germplasm of Sikkim and Darjeeling hills, their identification and diagnosis. Indian Phytopath, 63(3), 326–332.
- Passardi, F., Penel, C., & Dunand, C. (2004). Performing the paradoxical: How plant peroxidases modify the cell wall. Trends in Plant Science, 9(11), 534–540. https://doi.org/10.1016/j.tplants.2004.09.002
- Qamer, Z., Chaudary, M. T., Du, X., Hinze, L., & Azhar, M. T. (2021). Review of oxidative stress and antioxidative defense mechanisms in Gossypium hirsutum L. in response to extreme abiotic conditions. Journal of Cotton Research, 4(1), 1–9. https://doi.org/10.1186/s42397-021-00086-4
- Ramamoorthy, V., Viswanathan, R., Raguchander, T., Prakasam, V., & Samiyappan, R. (2001). Induction of systemic resistance by plant growth promoting rhizobacteria in crop plants against pests and diseases. Crop Protection, 20(1), 1–11. https://doi.org/10.1016/S0261–2194(00)00056-9
- Roylawar, P., Khandagale, K., Randive, P., Shinde, B., Murumkar, C., Ade, A., Singh, M., Gawande, S., & Morelli, M. (2021). Piriformospora indica primes onion response against stemphylium leaf blight disease. Pathogens, 10(9), 1085. https://doi.org/10.3390/pathogens10091085
- Safeer, M. M., & Umamaheswaran. (2021). Occurrence of Cymbidium mosaic virus in dendrobium orchids in Kerala and its management through meristem culture. Indian Journal of Pure Applied Bioscience, 9(1), 267–275. https://doi.org/10.18782/2582-2845.7723
- Sangeetha, B, Krishnamoorthy, A. S., Renukadevi, P, Malathi, V. G., Sharmila, D. J. S, Amirtham, D 2020 Antiviral activity of basidiomycetous fungi against Groundnut bud necrosis virus in tomato Pesticide Biochemistry and Physiology 166 104570 https://doi.org/10.1016/j.pestbp.2020.104570 https://www.sciencedirect.com/science/article/pii/S0048357520300651
- Seoh, M. L., Wong, S. M., & Zhang, L. (1998). Simultaneous TD/RT-PCR detection of Cymbidium mosaic potexvirus and Odontoglosum ringspot Tobamovirus with a single pair of primers. Journal of Virological Methods, 72(2), 197–204. https://doi.org/10.1016/S0166-0934(98)00018-4
- Seyfferth, C., & Tsuda, K. (2014). Salicylic acid signal transduction: The initiation of biosynthesis, perception and transcriptional reprogramming. Frontiers in Plant Science, 5, 697. https://doi.org/10.3389/fpls.2014.00697
- Shah, S., Thapa, B. B., Chand, K., Pradhan, S., Singh, A., Varma, A., Thakuri, L. S., Joshi, P., & Pant, B. (2019). Piriformospora indica promotes the growth of the in-vitro- raised Cymbidium aloifolium plantlet and their acclimatization. Plant Signaling and Behaviour, 14(6), 1596716. https://doi.org/10.1080/15592324.2019.1596716
- Sherpa, A. R., Bag, T. K., Hallan, V., & Zaidi, A. A. (2007). Incidence of Cymbidium mosaic virus (CymMV) in Sikkim. Indian Phytopath, 60(1), 133–136.
- Sofy, A., Sofy, M., Hmed, A., & Meghawry, N. (2019). Potential effect of plant growth- promoting rhizobacteria (PGPR) on enhancing protection against viral diseases. Book: Field Crops: Sustainable Management by PGPR, 411–445. https://doi.org/10.1007/978-3-030-30926-8_15.
- Song, F., & Goodman, R. M. (2001). Activity of nitric oxide is dependent on, but is partially required for function of, salicylic acid in the signaling pathway in tobacco systemic acquired resistance. Molecular Plant.-Microbe Interactions, 14(12), 1458–1462. https://doi.org/10.1094/MPMI.2001.14.12.1458
- Srivastava, S. K. (1987). Peroxidase and polyphenol oxidase in Brassica juncea infected with Macrophomina phaseolina (Tassi) Goid. and their implications in disease resistance. Journal of Phytopathol, 120(3), 249–254. https://doi.org/10.1111/j.1439-0434.1987.tb04439.x
- Verma, S., Varma, A., Rexer, K. H., Hassel, A., Kost, G., Sarbhoy, A., Bisen, P., Butehorn, B., & Franken, P. (1998). Piriformospora indica, gen. et sp. nov., a new root-colonizing fungus. Mycologia, 90(5), 896–903. https://doi.org/10.1080/00275514.1998.12026983
- Wagih, E. E., & Coutts, R. H. A. (1982). Peroxidase, polyphenoloxidase and ribonuclease in tobacco necrosis virus infected with the W strain of Cucumber mosaic virus. Physiological. Plant Pathology, 1, 133.