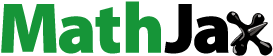
Abstract
According to the World Food Programme (WFP), the projected increase in the human population stands at 2 billion people by 2050. At the same time, world food production has been witnessing a declining trend over recent years, and 690 million (8.9%) of the world’s population are already in severe starvation. Climate variability and climate change impacts on food security are very eminent today. Against this backdrop, this study explored the real effects of climate variability and change on food security in Africa by applying the system Generalized Method of Moments (GMM) and the Panel Corrected Standard Errors (PCSEs) estimation techniques on data from 2001 to 2018 for 38 selected African countries. The findings reveal that higher amounts of precipitation positively influence food security along two dimensions: food availability and utilization. Hotter temperatures negatively impact food availability and utilization. However, it aids food accessibility in Africa. Similarly, carbon dioxide emissions improve food availability and are harmful to food accessibility and utilization in Africa. Consequently, the effects of climate variability and change on food security in Africa are undesirable, thereby putting the continent at risk of food insecurity over the long run. These findings provide practical implications for policy change to address the disastrous effects of climate variability and change on food security in Africa.
1. Introduction
Climate variability, otherwise known as climate change, affects food security worldwide. The advanced countries, which we called “emitters”, tend to be at the benefiting end, while their counterparts, the Less Developed Countries (LDCs) termed in this study as “sufferers”, are at the losing end. The intensity of climate change effects varies across countries, and this majorly depends on each country’s location, technology, and affluence (IPCC, Citation2022). However, regardless of a country’s economic status, location, and or technological level, the effects of climate variability or change spare no one. Global temperatures are increasing due to the persistent emission of greenhouse gasses into the atmosphere from both natural occurrences and anthropogenic factors (Al-Mulali & Che Sab, Citation2018; Bouznit & Pablo-Romero, Citation2016). Rising temperatures and environmental greenhouse gases (GHGs) reduce water and land availability, affecting crop production qualitatively and quantitatively (Watson et al., Citation1995).
Persistent changes in erratic weather patterns have disastrous effects on the developed and developing world regarding their cultivation, processing, industrialization, and economic status. Climate variability or change has become a dangerous phenomenon threatening humanity and wildlife’s existence (IPCC, Citation2014). However, it is important to state clearly that environmental factors such as temperature, precipitation, and wind play an essential role throughout man’s life cycle. Similarly, the survival of all life on earth and their interactions with the troposphere, ocean, and land are closely linked (IPCC, Citation2018). Nevertheless, addressing climate change is necessary to achieve food security (IPCC, Citation2018).
Furthermore, the Food and Agriculture Organization (FAO) opined that in the 21st century, there are now more malnourished people in the world, particularly in developing countries. For instance, in 2021, about 193 million people (21.3%) worldwide, were in a worse situation of food insecurity compared to about 108 million (11.3%) people in the same situation in 2016 (FAO & WFP, Citation2020). Climate variability and change account for about 600–700 million people facing food insecurity worldwide (GNAFC & FSIN, Citation2021; IFAD & WHO, Citation2020; RPCA, Citation2016). In particular, Africa’s food insecurity concerns continue to pose serious problems. For example, between 2000 and 2020, food insecurity in Africa rose from 25% to 37%, albeit a relative decline in the mid-decade. (FAO et al., Citation2020).
In the same vein, many African countries are agrarian, and agriculture alone contributes about 14% to their gross domestic product (GDP). The sector employed about 52.9% of the sub-workforce in 2019 (Painter et al., Citation2022). Nevertheless, agriculture is still a traditional industry in Africa. The sector is very vulnerable to climate variability and change . A significant number of these countries still rely on rain-fed agriculture. Therefore, a dramatic decline in crop and livestock production due to changing rainfall patterns and rising temperatures affects agricultural output and food distribution in general. Against this backdrop, there is a need for further investigations into the relationship between climate variability or change and food security in Africa.
Moreover, the global population is estimated to reach about nine billion by 2050, which will require about an extra 70% increase in global food production to feed the growing population (Kader, Citation2005; Khandelwal et al., Citation2022). The projected increase in global food demand calls for serious concern regarding food security across its three main dimensions, such as food availability, food accessibility, and food utilization in all countries. Lloyd et al. (Citation2011) estimated that by 2050, undernourishment would increase by 55% in Eastern and Sub-Saharan Africa and 62% in Southern Asia. Meanwhile, the United Nations Sustainable Development Goals 2 and 13 aimed at ending Hunger by 2030 and reducing the emission of greenhouse gases into the atmosphere by about 1.5C in 2050, respectively. The current trajectories cast doubts about whether countries can achieve these targets.
Previous studies abound in the climate change and food security literature centered on either one dimension of food security, climate change, or both. See, for example, (Badolo & Somlanare, Citation2013; IFAD & WHO, Citation2020; Kinda et al., Citation2019; Knox et al., Citation2012; Leisner, Citation2020; Loladze, Citation2014; Rowhani et al., Citation2011; Zhu et al., Citation2018). However, the current study follows the example of Affoh et al. (Citation2022) by incorporating three food security dimensions into the analysis. Thus, food availability, food accessibility, and food utilization. The study also uses three variables of climate variability or change (temperature, precipitation, and carbon dioxide emission). The current study goes beyond the existing literature and the most recent study by Affoh et al. (Citation2022) to extend the data to cover 38 countries in Africa. The study further contributes to the food security visa-vis-climate variability or change literature in Africa by checking for the robustness of the results and accounting for cross-sectional dependence among the variables. These targets are achieved through the application of the Pane Corrected Standard Errors (PCSE) and the system Generalized Method of Moments (GMM) estimation techniques.
The rest of the study is structured as follows; the second section discusses the literature review where previous empirical literature on food security-visa-vis-climate variability and change are analyzed; the third section presents the methods, variables, and sources of data; and the fourth section presents the estimation strategy. The fifth section reports the empirical results, the sixth section presents the discussions of the findings, and the seventh section highlights the conclusion and policy directions.
2. Literature review
This section discusses the related empirical studies conducted over the past on the food security-visa-vis-climate variability or change scholarship. The empirical literature reviews are presented along three food security dimensions. Thus, food availability, food accessibility, and food utilization.
2.1. Climate variability or change and food availability
The supply side of food security is known as food availability. Climate uncertainties such as higher temperatures, changes in precipitation patterns, and carbon dioxide emissions have adverse effects on agriculture, mainly crops and livestock production (Wiebe et al., Citation2015). Food availability includes production, commercial imports and exports, food aid, and domestic inventories. Climate change can disrupt food availability, reduce access to food, and affect food quality. Additionally, variations in extreme weather events and reduced water availability can reduce crop yields (Nelson et al., Citation2010).
Moreover, Affoh et al. (Citation2022) examined the relationship between climatic factors such as rainfall amount, temperature, and carbon dioxide (CO2) emission and three food security dimensions in 25 Sub-Saharan Africa between 1985 and 2018. The study adopted the Pool Mean Group (PMG) panel autoregressive distributed lag model. The findings show that higher temperatures negatively affect food accessibility and availability but do not influence food utilization. Also, rainfall and carbon emissions positively influenced food availability in Sub-Saharan Africa. Similarly, Verschuur et al. (Citation2021) conducted a study in Lesotho to investigate the impact of climate change on food security. The study found that drought and climate variability negatively impact food availability in the country and that trade overdependence contributes to a long period of severe food insecurity in the state of volatile agriculture.
Furthermore, R. K. Singh et al. (Citation2022) assessed the food security status within the eight South Asian Association for Regional Cooperation (SAARC) nations through the four major food security dimensions: food accessibility, availability, utilization, and food stability. The study revealed that only two nations, Bangladesh and Sri Lanka, were food secure. Additionally, Badolo and Somlanare (Citation2013) conducted a study on the impact of low rainfall on food security in 25 countries of Sub-Saharan Africa, including 77 other developing countries. The study used panel econometric analysis on the selected countries’ data from 1960–2008. The study found that rainfall variability worsens food insecurity and supply in developing countries. However, the study shows that the effect of rainfall shock is more paramount in Sub-Saharan Africa than in other regions. Thus, the study concluded that countries prone to food price inflation witness severe effects of climate variability.
In addition to the above, Stuch et al. (Citation2020) conducted a study to investigate the effect of climate variability on food security in four regions of Sub-Saharan Africa between 1971 and 2000. The findings depicted that corn and cereals (sorghum & millet) yields for the subsistence small-medium scale farmers have declined over the years and that the Central African region was the most affected with about 89% decrease in corn and cereals production and harvesting in contrast to 85% in the West African sub-region, 32% in the East African sub-region and 29% in Southern Africa.
Besides, Mekonnen et al. (Citation2021) conducted a study in the northern region of Ethiopia to analyze the extent to which climate change has affected food availability in the region from 1985–2016. The study selected a total of 185 farmers, and the results were analyzed using descriptive and inferential statistics in addition to the logistic regression model. The study reveals that climate change has hurt family food security over the past three decades. The findings also revealed that crop production has fallen due to low rainfall, extreme erosion, and temperature rise. The findings further revealed that about 60.5% of respondents surveyed were food insecure. Therefore, the study concluded that improving the local focus on climate change, facilitating the participation of female-headed households in income-earning activities, and strengthening existing adaptation measures influence food security.
Also, WU et al. (Citation2021) examined the effect of climate variability on cereal corn farming in China from 1979 to 2016. The study adopted the Feasible Generalized Least Square (FGLS) method to analyze the data. The findings reveal that higher temperatures lessen the quantity of corn produced, and moderate rainfall boosts corn production in China’s south and north regions. Similarly, Fuller et al. (Citation2018) observed that a persistent increase in annual temperatures of about 0.8°C had caused a drastic decline in plantain production in Central Africa between 1950 and 2013. The study predicted that by 2080, temperatures in Central Africa would rise by about 2°C degrees Celsius, resulting in a 39% decrease in plantain yield and a 51% decrease in education investment.
Further, Rowhani et al. (Citation2011) analyzed the impact of climate variability on crop production in Tanzania and found that the continued increase in temperature and seasonal changes in precipitation hampered cereals’ yield. The study pointed out that in 2050, the temperature rise will be 2°C; seasonal precipitation variability will amount to 20%, and that will generally cause a severe reduction in crop yield (corn, sorghum, and rice) by 3.6%, 8.9%, and 28.6% respectively. Concordantly, C. Zhao et al. (Citation2017) used four estimation methods, including statistical regressions analytical methods, global grid-based, local point-based, and field-warming experiments, to assess the impacts of temperature changes on crop yields such as corn, wheat, and soybeans. The study found that global temperature increases negatively affect crop production, resulting in a food supply shortage. Evidence from rural Africa and Asia also revealed that climate change reduces crop yields and marine foods (Dasgupta & Dasgupta, Citation2017).
2.2. Climate variability or change and food accessibility
Climate variability or change adversely influences global, regional, and local food accessibility. Food accessibility represents the demand side of food security. In this case, food accessibility refers to the ability of the household to purchase, afford, and tastes food commodities that permit them to effectively and efficiently curtail hunger and poverty in their midst (Dessie & Ademe, Citation2017; FAO, Citation2015). The higher atmospheric concentration of carbon dioxide (CO2), yearly-rising temperatures, changes in rainfall patterns, and variations in severe weather conditions, contributed to a decrease in cereal yields. The reduction in crop production causes food insecurity and destabilizes food delivery and accessibility due to fluctuations in food prices (FAO, Citation2015).
Asare-Nuamah (Citation2021) analyses how smallholder agriculturists understand climate change impacts on subsistence cultivation, food security, and accessibility within the country zone of Adansi North in Ghana. The study employed a survey and semi-structured meeting to collect data from 378 smallholder agriculturists and 41 key informants and used the blended strategy to analyze the data. The results indicated that climate variability affects food accessibility as it reduces the income of smallholder agriculturists by reducing crop yields.
Additionally, the study by Wang (Citation2010) presents an experimental confirmation of the determinants of food security. The study incorporated different variables, alongside provincial residents’ per capita disposable income, food retail cost list, rural catastrophe zone, real estate beneath development, and sparing of urban and regional inhabitants. The study used different estimation strategies, including the Pooled OLS, Fixed Effect, Difference-GMM, and System-GMM, on data from 1985–2007. A sample of 27 area energetic board records in China was employed to assess the relationship between all factors. The study, agreeing with the estimates of the system-GMM, concluded that climate variability adversely influences food accessibility and overall food security in modern-day China.
Similarly, Gebre and Rahut (Citation2021) investigated the relationships between the ubiquity of household food insecurity and climate change vulnerability in East Africa using primary data gathered in Ethiopia, Kenya, and Tanzania between 2018–2019. The findings indicated that 52% of the region’s families considered for the study were food secure, 15% and 26% were just minimally and moderately food safe, and 7% had acute food insecurity. Additionally, the study reported that household demographic factors, social, financial, human capital, and natural assets/capital contribute significantly to lowering household-level food insecurity. However, the study concluded that exposure to climate change and crop losses resulting from climate variability highly influence the extent of family food insecurity across the nations in East Africa.
Furthermore, climate change directly affects the quantity of water in Egypt. It has led to the intrusion of saltwater from the Mediterranean into the groundwater, making agriculture vulnerable (Omar et al., Citation2021). Harris et al. (Citation2022) carried out a study to examine the plausible destructive impacts of climate risks on the food furnishes in the Indian States and Union Territories. The climatological and geological information derived from meteorological stations and satellite imagery was employed to decide the presence of eight climate-related risks such as droughts, wildfires, floods, intense rainfall, landslides, hurricanes, extreme temperatures, and sea level rises over the period 2000–2020. The study found that climatic risks in Indian states have destructive influences on the country-wide food supply, production, inter-state trade, and increasing food prices. Similarly, Alvi et al. (Citation2021) and Wossen et al. (Citation2018) reveal that climate change increases food prices, thereby affecting the purchasing power and the ability of poor households to access sufficient, safe, nutritious, and preferred food.
Moreover, Leisner (Citation2020) contends that in agricultural regions, climate change variables such as high concentrations of atmospheric carbon dioxide (CO2), other greenhouse gases, warmer temperatures, and changes in precipitation patterns affect crop productiveness. It reduces the world’s capability to preserve food manufacturing, lower food prices, and provide nutritious food for the developing and increasingly growing population. The environmental changes due to climate change have adversely impacted the household’s income and wealth and cut household investment in education. Besides, Thorlakson and Neufeldt (Citation2012) conducted a study in the western part of Kenya to survey the effect of climate change on subsistence agriculturists. The study found that lower precipitation and hotter temperature increase food insecurity by reducing crop yields and lowering farmers’ income.
In the same vein, Painter et al. (Citation2022) found that the East African communities in the coastal region experience food insecurity due to climate change. According to the study, many people along the coast rely on the ocean for sustenance, and marine resources are essential to regional food security. Hence, the study concluded that knowledge of the marine environment is urgently needed to better support decision-making efforts to address climate variability.
2.3. Climate variability, change, and food utilization
The continuous rise in carbon dioxide (CO2) concentration in the atmosphere has produced poor-quality rice. The increase in the concentration of CO2 in the atmosphere has impacted human health through the consumption of poor-quality food lacking adequate protein, micronutrients, and vitamins such as zinc, iron, and other nutrients. Zhu et al. (Citation2018) conducted a study investigating the impact of climate variability or change on food utilization. The study employs multi-year, multi-location in situ FACE (free-air CO2 enrichment) experiments for 18 genetically diverse rice lines throughout Asia. The results revealed that the decline in food quality had impacted human health from 138 million to 1.4 billion people worldwide. The reason is that rice is the primary food source for about 2 billion people worldwide. The study depicted further that an increase in carbon dioxide (CO2) has led to a decline in Vitamin B1, B2, B5, and B9. It further reported that the health of 600 million people who depend on rice consumption had been affected due to the poor-quality state of rice.
Moreover, Myers et al. (Citation2017) have pointed out that the global food system faces an unprecedented increase in demand due to a growing population and environmental degradation. The study indicated that the capacity to guarantee food security and diet faced with quickly changing biophysical conditions would have an unusual influence on the global malady burden. Human activities and climate change will impact the amount and quality of food delivered and the capacity to distribute the food equitably. Rising temperatures and erratic rainfall also increase the spread of pests and diseases, heat stress, water and forage challenges in livestock, and the reduction in growth and reproduction. The effect is the low nutritional value of the food supplied (Thornton & Herrero, Citation2015). Studies across the globe have equally reported the loss of animals and the decline in milk and wool production due to climate change (Chakrabarti, Citation2015).
Furthermore, Bourgault et al. (Citation2017) carried out a study to investigate the relationship between the atmospheric concentration of CO2 and grain quality and yield. The study found that greater levels of atmospheric concentration of CO2 improve grain growth only under wet conditions. In contrast, the study revealed further that the quality of grain significantly reduced with elevated levels of atmospheric concentration of CO2. Similarly, Loladze (Citation2014) conducted a study to examine how increasing levels of atmospheric concentration of CO2 affect the ionome of plants. The study found that higher levels of atmospheric carbon dioxide (CO2) emission significantly affect plants’ nutrients by decreasing the amount of protein and nitrogen concentration and increasing the total nonstructural carbohydrates (TNC: mainly sugars and starch).
Last but not least, adaptation and mitigation measures are essential in combating the disastrous impact of climate variability or change. In this light, adhering to renewable energy will help reduce a higher degree of carbon dioxide emission into the atmosphere and, simultaneously, have a lossless impact on food quality, utilization, and food security. Ajayi et al. (Citation2022) conducted a study investigating the role of renewable energy consumption in mitigating climate change effects in Nigeria. The study recognizes the inherent doable of renewable power resources to mitigate the incidents of climate variability and global warming. The study, therefore, concluded that for Nigeria to sustain financial growth, especially in agriculture and food security, renewable power for electricity generation ought to be adept in the nation’s rural improvement plan. That renewable energy strength represents a way to mitigate a nation’s contributions to anthropogenic climate change.
3. Methods, variables, and data sources
This study adopts the Panel Corrected Standard Errors (PCSEs) estimator and the system Generalized Methods of Moments (sys-GMM) estimator on data from 2001–2018 for 38 selected countries in Africa to model the short-long-run impact of climate variability or change on food security in the context of Africa. The 38 countries were carefully selected based on data available on the key variables of interest in this study across the sample. The dependent variable considered for this study is food security, which is measured using three disaggregated components such as food availability, food accessibility, and food utilization. The primary independent variable in this study is climate change, which is also measured based on three disaggregated data such as the mean annual temperature, the mean annual precipitation, and the amount of carbon emission (metric per tons per capita).
The variables such as Cereal yield (CY); Agriculture, forestry, and fishing (value added) as a percentage of GDP (GDPA); and Cereal Dietary Energy Supply (CDES) are proxy measures of food availability, food accessibility, and food utilization, respectively. The main reason for using these variables as proxy measures of the food security dimensions is that they comprehensively measure the three-food security indicators. A recent study by Affoh et al. (Citation2022) also employed these variables as food security indicators in Sub-Saharan Africa. Furthermore, the impact of climate variability and change on food security is more evident through its direct effect on food availability by either decreasing or increasing agriculture yields and indirectly affecting the gross domestic food supply in the case of Africa.
Besides, Agriculture, forestry, and fishing, value added as a percentage of GDP (GDPA), constitutes agriculture’s contribution to economic growth. It is essential to state that agriculture employs about 60% of the economically active population in Africa. Therefore, one cannot overlook the contribution of Agriculture to economic activities and the overall well-being of the African population. Agriculture enables farmers to access food commodities in the market. Hence, the GDPA variable fits a proxy measure of food accessibility. Finally, due to difficulty in accessing data on what constitutes the nutritional value of food in Africa, the current study, just as Affoh et al. (Citation2022), uses the Cereal Dietary Energy Supply (CDES) as a proxy measure of food utilization. Cereal Dietary Energy Supply (CDES) measures the total calories from cereal consumption daily. Also, the study employed the CDES as a proxy measure of food utilization because cereal consumption constitutes a chunk of the diet of the African population.
Moreover, the control variables considered for this study are those assumed to influence food security significantly in Africa. Therefore, we introduce these variables in the estimations to take care of their confounding effects on African food security. The control variables include; Population Growth, Inflation, Real Gross Domestic Product (Real GDP), and Cereal Crop Production. The exclusion of these variables may result in what we call “variables bias or omitted variable problem”, which may undermine the efficiency of the results. Table summarizes all the variables and their data sources.
Table 1. Variables description and data sources
4. Estimation strategies
The main objectives of this study are to estimate the impact of climate variability (short-run effects) and climate change (long-run effects) on food security in Africa. Consequently, the current study carefully selected the estimation strategies based on their significance and peculiar characteristics. The two-step system GMM is employed to enable us to ascertain both the effects of climate variability and change on food security in Africa. The GMM estimation technique was developed first by Holtz-Eakin et al. (Citation1988) and further elaborated by Arellano and Bond (Citation1991), Areliano and Boverb (Citation1995), and Blundell and Bond (Citation1998). The significance of the two-step system GMM over the difference GMM estimation technique is that it accounts for the endogeneity problem that may arise because of poor instruments. In solving the endogeneity problem, the two-step system GMM develops additional moment conditions that make it possible to use the first-difference forms of the lagged dependent variables as valid instruments for the level form of equations 2, 3, and 4, respectively, and use the level form of the lagged dependent variables as valid instruments in place of the endogenous variables in the first difference form of equations 2, 3, and 4 (Blundell & Bond, Citation1998).
Furthermore, the two-step system GMM controls for the problems of autocorrelation and heteroskedasticity that are most likely to exist in panel data. The Arellano-Bond test for second-order autocorrelation (AR2) in first-difference order assists in checking for autocorrelation in this study while using the “robust” routine in STATA to account for heteroskedasticity. Also, using the Hansen J-test for over-identifying restrictions, we can test for the validity of the instruments in the model(s). Roodman (Citation2009) posited that instrument proliferation could compromise the strength of the Hansen J statistics in the system-GMM model. As such, this study used the “Collapse” option in STATA as proposed by Roodman (Citation2009) to collapse all the internally generated instruments to take care of the instrument proliferation trap. Hence, the two-step system GMM estimation technique produces consistent heteroskedasticity and autocorrelation results.
Equation 1 represents the generic form of the GMM econometric model.
Where is the dependent variable which is assumed to be persistent;
is the lag value of the dependent variable;
is a
vector of the explanatory variable;
is a
vector of coefficients of the explanatory variables;
is the coefficient of the lag value of the dependent variable;
is the idiosyncratic error term, and
is the country-specific effects.
Based on equation 1, the operational form of the econometric model(s) set out for the estimations are specified in equations 2, 3, and 4, respectively.
Equations 2, 3, and 4 show the effects of climate variability on food availability, food accessibility, and food utilization, respectively.
To estimate the effects of climate change on the various dimensions of food security (food availability, food accessibility, and food utilization), the study generated the long-run coefficients of only significant variables under equations 2, 3, and 4, respectively. Therefore, the long-run coefficients of the variables and their asymptotic standard errors are generated based on the delta method of Papke et al. (Citation2005). The formula for generating the long run coefficients is ; where
each long run coefficient,
the coefficient of the Kth independent variable, and
the coefficient of the lagged dependent variable used as a regressor.
Furthermore, it is essential to state that the two-step system GMM estimator does not account for cross-sectional dependence. Therefore, for purposes of robustness and after checking for cross-section dependence, the current study further adopted the Panel Corrected Standard Errors (PCSEs) to take effect of the cross-sectional (and temporal) dependence among the variables (Hoechle, Citation2007). Also, results based on the PCSEs are robust and consistent in most especially long panels. Besides, results based on the PCSEs are also robust and consistent in most especially long panels. Thus, panels with large cross-sectional units and a short period per cluster (N > T), see, for White (Citation1980, Citation1984) and Liang and Zeger (Citation1986). However, results based on the PCSEs are only for short-run analysis. The reason for its adoption in addition to the above reasons is to enable us to compare the results with the two-step system GMM short-run estimates for robustness check.
Equation 5 presents the generic form of the econometric model of the PCSEs estimation:
Where is the dependent variable;
is a
vector of the explanatory variable;
is a
vector of coefficients of the explanatory variables;
is the intercept term;
is a
vector of the unobserved explanatory variables, which is assumed to be contemporaneously exogenous to the conditional error, and
is the idiosyncratic error term.
Operationally, we can rewrite the PCSEs econometrics specification in equation 5 as in equations 6, 7, and 8 showing the linkages between climate variability and the various food security dimensions such as food availability, food accessibility, and food utilization, respectively:
5. Empirical results
5.1. Descriptive statistics
When analyzing the statistical properties of the food security indicators in Africa, the data from Table shows that the average value of food utilization (CDES) is 60.392 with a standard deviation of 8.536; food availability (CY) has an average value of 1585.169 with a standard deviation of 1386.786, and food accessibility (AGDP) has an average value of 21.197 with a standard deviation of 14.439. The lower standard deviations of the food security indicators from their means imply that African countries are not so much heterogeneous regarding food security issues. However, food availability has a maximum value of 9453.7 and a minimum value of 34.3, while food accessibility has a maximum value of 79.049 and a minimum value of 1.798. The above findings indicate that some African countries are better off regarding food availability and accessibility, while others are already facing food insecurity issues within the study period. Relatively, food utilization in Africa is much more evenly distributed, with a maximum value of 81 and a minimum value of 19.
Table 2. Descriptive statistics
Also, when analyzing the descriptive statistics of the climate change indicators in Africa, the results from Table indicate that the average value of the mean annual temperature (MAT) is 24.293 with a standard deviation of 3.834; mean annual precipitation (MAP) has an average value of 1010.905 with a standard deviation of 696.491; while the carbon dioxide emission (CO2) has an average value of 0.997 with a standard deviation of 1.495. The results show a relative disparity in the mean annual temperatures across Africa. However, the amount of atmospheric carbon dioxide emissions vary significantly across the countries as shown by the maximum rate of CO2 emission in Africa being 8.573 within the specified period (achieved by South Africa in 2008), and the minimum rate of CO2 emissions was 0.027 (achieved by Central African Republic in 2013). Likewise, the standard deviation of CO2 is 1.495 far above the mean which is 0.997 implying relative disparities among the countries in terms of CO2 emissions. Concordantly, the mean annual precipitation is relatively less dispersed though some countries are already facing a deficit (drought) in terms of the amount of rainfall received, as the maximum value is 3061.66 and the minimum value is 10.88.
Furthermore, renewable energy consumption (REC) has a mean value of 59.611 with a standard deviation of 28.385, and cereal production (CP) has a mean value of 3,289,702.2 with a standard deviation of 5,534,476.8. Also, population growth (POP) has a mean value of 2.328 with a standard deviation of 0.905; inflation (INFL) has a mean value of 6.474 with a standard deviation of 9.642; whereas annual GDP growth (GDPg) has a mean value of 4.258 with a standard deviation of 4.385. The general implication is that African countries are highly heterogeneous regarding cereal production, inflation rates, and the levels of economic growth and development.
5.2. Trend of analysis of mean annual precipitation in selected African Countries
Figure shows the trend of rainfall or mean annual precipitation across the 38 selected African countries over the period 2001 to 2018. The figure shows that the state of rainfall in Africa has been fluctuating over the period under consideration, though it shows a rising trend in recent times. The mean annual precipitation falls slightly between 2001 and 2002, it then rose in 2003 and declined thereof until 2005. The rainfall amount witnesses an astronomical surge in 2006 when it almost reaches its peak. It then declined sharply in 2007 and rose back again reaching its maximum in 2010. From 2010 to 2015, the mean annual precipitation declined considerably reaching its lowest in 2015. From 2015 thereafter, the selected African countries experienced a relative increase in the amount of rainfall received over the years. The relative instabilities in terms of the quantity of rainfall received, call for serious concerns regarding measures to address food insecurity issues in Africa as agriculture is largely rain-fed in the continent.
5.3. Test for cross-sectional dependence
The study runs the Pesaran test for cross-sectional dependence on models 1, 2, and 3 to examine whether cross-sectional dependence exists among the variables. Table reveals that the null hypothesis of the Pesaran test for cross-sectional independence is rejected for models 1 and 2, respectively, at the 1% significance level. However, we failed to reject the null hypothesis of the Pesaran test for cross-sectional independence even at the 10% significance level for model 3 since the p-value (0.3297) is greater than the 0.1 level of significance. The conclusion is that models 1 and 2 are cross-sectionally dependent, while model 3 is cross-sectionally independent. Therefore, this necessitates the application of the PCSEs estimation method to the models for robustness checks.
Table 3. Pesaran’s test for cross-sectional dependence
5.4. Impact of climate variability and change on food availability
Table shows the results of the sys-GMM and the PCSEs estimations. The results for both estimations show that an increase in mean annual precipitation (MAP) and carbon dioxide emission (CO2) exerts a positive and statistically significant impact on food availability in the short run, all things being equal. However, a short-run increase in mean annual temperature appears to worsen food availability under both estimations, albeit the effects are statistically insignificant. Moreover, holding other factors constant, a short-run growth in cereal crop production (lnCP) has a positive and statistically significant impact on food availability under both estimation techniques. Interestingly, renewable energy consumption (REC), population growth (POP), and inflation (INFL) appear to worsen food availability under both estimations. Though the effect of REC and POP are generally not statistically significant under the sys–GMM. The effect of INFL appears to be positive but statistically insignificant in the short run under the PCSEs estimations. Annual GDP growth, however, is positively related to food availability in the short run, despite not being statistically significant under both methods of estimation.
Table 4. Sys–GMM and PCSEs estimates of food availability determinants
The long-run dynamics reveal that other things being equal, an increase in MAP, CO2 and lnCP positively and significantly impacts food availability, whereas higher INFL negatively impacts food availability in the long run.
5.5. Impact of climate variability and change on food accessibility
Table reports the results of the sys-GMM and the PCSEs estimations. The results show that under both estimation techniques, an increase in mean annual temperature (MAT) and mean annual precipitation (MAP) impact food accessibility positively in the short run, among other things. However, MAP is not statistically significant, only under the sys-GMM estimation. In contrast, the results suggest further that ceteris paribus, an increase in carbon dioxide emission (CO2), impacts negatively and statistically significantly on food accessibility in the short run under both estimation techniques. Again, holding other factors constant, a short-run increase in cereal crop production (lnCP) has a positive and statistically significant impact on food accessibility under both estimation methods. Nevertheless, renewable energy consumption (REC) positively impacts food accessibility in the short run under both estimations, although not statistically significant under the sys-GMM. However, population growth (POP), inflation (INFL), and annual GDP growth (GDPg) appear to show mixed outcomes under both estimations and are generally not statistically significant except for INFL, which is significant only under the PCSEs estimation.
Table 5. Sys—GMM and PCSEs estimates of food accessibility determinants
In terms of the long-run dynamics, the results indicate that holding other factors constant, an increase in MAT and lnCP impacts positively and statistically significantly on food accessibility. In contrast, a unit increase in CO2 in the atmosphere adversely affects food accessibility in the long run.
5.6. Impact of Climate Variability and Change on Food Utilization
Table presents the results of the sys-GMM and the PCSEs estimations. The estimates for both estimation techniques show that an increase in mean annual precipitation (MAP) in the short run impacts food utilization positively and statistically significantly, ceteris paribus. In contrast, a unit increase in carbon dioxide emission (CO2) and an increase in mean annual temperature (MAP) negatively and statistically significantly influence food utilization in the short run, holding other factors constant. Furthermore, holding other factors constant, an increase in cereal crop production (lnCP) in the short run has a positive and statistically significant impact on food utilization under both estimation techniques. Likewise, renewable energy consumption (REC) appears to positively influence food utilization in the short run, though it is insignificant only under the sys-GMM estimation. Population growth (POP) exerts a negative and statistically significant impact on food utilization in the short run under the sys-GMM, though it appears to be positive but statistically insignificant under the PCSEs.
Table 6. Sys—GMM and PCSEs estimates of food utilization determinants
Similarly, analyzing the results from the long-run perspective, the results suggest that an increase in MAT, MAP, and lnCP positively and statistically significantly impacts food utilization, whereas population growth (POP) has a deleterious effect on food utilization in the long run.
6. Discussions of findings
There is a growing concern in recent times regarding food insecurity in the world by 2050, with most people pointing fingers at climate variability and change. Nevertheless, other factors also contribute to the food insecurity issues around the world today. Therefore, to gain more insight into the impact of climate variability and change on food security in Africa, this study assesses the impact of three climatic factors such as temperature, precipitation, and carbon dioxide emission, on three leading food security indicators such as food availability, food accessibility, and food utilization. Following are detailed discussions of the effects of climate variability/change on each of the various dimensions of food security in Africa.
6.1. Impact of climate variability/change on food availability in Africa
The findings convincingly show that an increase in annual precipitation and carbon dioxide emissions positively impacts food availability in the short and long run. In contrast, higher temperatures negatively impact food availability in the short run, though the effect is generally not significant under both estimations employed in this study. The positive and statistically significant short and long-run impact of high annual precipitation corroborates with the findings of studies by (Affoh et al., Citation2022; Verschuur et al., Citation2021; WU et al., Citation2021). This empirical result underlies the importance of precipitation in food availability in Africa. Increased precipitation enhances soil aeration through burrowing activities by micro-organisms, aid plant growth, improves plant nutrients, and consequently, higher crop yields. Precipitation is significant to food availability in Africa, where most farmers rely on rain-fed agriculture due to poor irrigation schemes (OECD/FAO, Citation2016).
Moreover, the positive impact of carbon dioxide emissions on food availability as reported in this study concords with the results of previous studies (Affoh et al., Citation2022; Lobell & Field, Citation2008; Loladze, Citation2014; Zhu et al., Citation2018). The implication is that an increasing amount of CO2 in the atmosphere is necessary for plant growth and the overall crop yield. Higher levels of atmospheric CO2 are associated with improved photosynthesis by plants and their overall growth and development, thereby translating into better cereals crop yield, which is increasingly becoming the world’s most important food source (Lobell & Field, Citation2008). This result arguably is consistent with expectations, given that plants required carbon dioxide for photosynthesis. However, it is unclear in the literature regarding which quantity of CO2 in the atmosphere is detrimental to plants’ growth and development. Hence, measures should be taken to curb CO2 emissions at reasonable rates around the globe as higher levels of CO2 in the atmosphere also have severe environmental consequences such as global warming and extreme weather patterns which could be detrimental to plants and wildlife. On the contrary, higher temperatures negatively influence food availability in the short run, though the effect is generally insignificant. The negative impact of temperature increases on cereal crop yields and the overall food availability in Africa, as reported in this study, attests to the findings of previous studies (Affoh et al., Citation2022; Fuller et al., Citation2018; Rowhani et al., Citation2011; WU et al., Citation2021). World temperature increase hampers food availability by decreasing cereal crop yield, particularly in Africa, where the climate is tropical and seasonally dry.
6.2. Impact of climate variability/change on food accessibility in Africa
Furthermore, the results indicated that temperature increases and increases in annual precipitation impact food accessibility positively, albeit only the effect of temperature is statistically significant. The positive effect of temperature on food accessibility appears somewhat to be counterintuitive. Perhaps, it means that higher temperatures do not so much affect households’ food income in Africa since the climate is already tropical and seasonally dry. Notwithstanding, (Affoh et al., Citation2022) have found that higher temperature affects food accessibility in Sub-Saharan Africa. The differences in the outcomes may result from the different estimation techniques employed and the variations in the sample size used for both studies.
In contrast, carbon dioxide emissions negatively and statistically significantly affect food accessibility in Africa. The reason is that a higher concentration of CO2 emission due to natural and anthropogenic factors causes global warming and adverse climate and weather patterns, thereby threatening human survival. The effect is low economic activities coupled with low productivity, low incomes, rising poverty, and hence a decrease in food accessibility, particularly for low- and middle-income earners.
6.3. Impact of climate variability/change on food utilization in Africa
Moreover, the findings of this study suggest that higher levels of mean annual precipitation positively influence food utilization in Africa both in the short- and long run. However, rising temperatures and higher atmospheric carbon dioxide emissions negatively impacted food utilization in Africa, albeit the effect of only temperature increase is significant. The positive relationship between increasing precipitation and food utilization indicates that the number of calories intakes from cereal crops or the quality of dietary energy supply from cereal crops that constitute a chunk of the diet of the African population needs a higher amount of precipitation. Drought and water stress adversely affect the nutritional value of plants, as it makes crops experience stunted growth, poor aeration of the soil, low burrowing activities by living or micro-organisms, and consequently, reducing the nutritional value of cereal crops. Previous empirical studies by (Affoh et al., Citation2022; Fischer et al., Citation2019; S. S. Singh et al., Citation2012; C. X. C. X. Zhao et al., Citation2009) support the findings of this study.
Nevertheless, rising temperatures harm food utilization in Africa in the short and long run. The findings confirm our initial finding that higher temperatures negatively affect crop yields and, thus, food availability in Africa, though the result was generally not significant. Higher temperatures affect food utilization by causing a decline in the nutritional value of crops through stunted growth, poor health of plants, and loss of essential nutrients. This finding agrees with studies by (Chakrabarti, Citation2015; Thornton & Herrero, Citation2015). The study also found that increasing the amount of carbon dioxide CO2 emission in the atmosphere harms food utilization in Africa, particularly in the short run. This finding corroborates the results of past empirical studies conducted by (Bourgault et al., Citation2017; Loladze, Citation2014; Myers et al., Citation2014; Zhu et al., Citation2018). Similarly, we observed that the continuous rise in the concentration of carbon dioxide (CO2) in the atmosphere has resulted in the production of poor-quality of cereal crops, which has impacted human health through the consumption of poor-quality food lacking adequate protein, micronutrients, and vitamins as zinc, iron and other nutrients. However, Affoh et al. (Citation2022) found no link between temperature changes, carbon dioxide (CO2), and food utilization in Sub-Saharan Africa.
6.4. Impact of the control variables on the different food security dimensions
Apart from the climatic factors, cereal production is identified in this study as the most significant factor influencing food security in Africa, as it positively and significantly impacted food security across the three dimensions: availability, accessibility, and utilization. It implies that higher volumes of cereal production contribute to Africa’s food security. Affoh et al. (Citation2022) observed a similar trend in their submission, highlighting the significant role of cereal production in addressing the food insecurity issues in Africa. Besides, inflation has short and long-term detrimental impacts on food availability in Africa. Inflation distorts the price system and makes it difficult for farmers to access farming materials and tools, adversely affecting crop yields and the general food availability in Africa. However, the effect of inflation on the other food security dimensions is insignificant. Population growth negatively and statistically significantly influences food utilization in Africa both in the short- and long-run. Higher population growth increases the chances of malnourishment due to inadequate food supply and the consumption of foods lacking the essential nutrients for healthy growth and human development. Finally, renewable energy consumption appears to positively influence food security in Africa in the short run along two dimensions: food accessibility and food utilization. Renewable energy usage is highly encouraged because it has the potential to reduce the atmospheric component of carbon dioxide emission, which is one of the main drivers of global warming, high heating, and unfavorable weather patterns. Ajayi et al. (Citation2022) opined that adhering to renewable energy will help reduce a higher degree of carbon dioxide emission into the atmosphere and, at the same time, lossless impact on food quality, utilization, and food security in general.
7. Conclusion and policy directions
The impact of climate variability and change on food security has created a heated debate among policymakers, governments, development practitioners, agriculturists, analysts, and researchers around the globe for decades now. This study contributes to the existing literature by employing the two-step system GMM and the PCSEs estimation techniques to re-investigate the impact of climate variability and change on food security, focusing on Africa, a continent tip to be the most vulnerable to climate change effects and most of its population is involved in agriculture. Overall, our findings reveal that a higher amount of rainfall or mean annual precipitation positively influences two food security dimensions (availability and utilization) in the short- and long run. Higher temperatures adversely affect food availability and utilization; however, it aids food accessibility in Africa. Moreover, carbon dioxide emissions improve food availability and are harmful to food accessibility and utilization in Africa. Consequently, the effects of climate variability and change on food security in Africa are not welcoming, and this has put the continent at risk of food insecurity issues over the next decades if efforts are not put in place now. Apart from the effects of the climate variables on the different food security dimensions, three of our control variables have both short- and long-run impacts on food security in Africa. Thus, cereal production positively and significantly influences all three dimensions of food security employed in this study; inflation only negatively and statistically significantly affects food availability in Africa; whiles population growth worsens food insecurity by negatively impacting food utilization. Renewable energy usage, however, was found to have only short-run positive effects on food accessibility and utilization in Africa and a short-run negative effect on food availability under the PCSEs estimations. Given these findings, the study suggests the following recommendations for policy change toward addressing the climate change and food security concerns in Africa and the world at large:
First, African governments need to pull their resources together and collaboratively fight against, if not all, most anthropogenic activities such as the burning of fossil fuel, bush burning, deforestation, sand winning, illegal mining, and improper disposal of industrial waste, among others that engender the environment and poses severe climatic consequences thereby threatening human survival and habitation.
Likewise, due to the poor irrigation system in Africa, governments and development practitioners need to provide more funds, roll out adequate irrigation schemes, and dig more dams to support irrigation farming in Africa. This initiative will go a long way to enhancing high crop production, food quality, and accessibility, thereby helping to reduce the food insecurity concerns in the continent where agriculture is highly rain-fed and subjected to climatic uncertainties.
Moreover, governments should also subsidize farming materials and tools for farmers and support farmers with lower interest-bearing capital or loans to enable them to expand their scale of production. This policy perspective will help increase the quantity and quality of food supply, as well as help in making farmers’ income sustainable in Africa. This is particularly important, especially in rural Africa, where most of the African population resides and whose primary occupation is agriculture.
In addition, farmers must be supported with high-yielding crops and drought-resistant varieties and educated on rainwater harvesting methods. Similarly, good agricultural practices, such as crop rotation, drip irrigation, terracing, and proper planting and harvesting techniques, to mention but a few, should complement the above policy measure to achieve the most effect. This initiative is imperative because it has the adaptive qualities of allowing farmers to overcome the losses from extreme weather patterns and mitigative features that reduce greenhouse gas emissions into the atmosphere, which are responsible for global warming.
Besides, the development and establishment of more agriculture-related R&D institutes can be a better way to go. Research and development are paramount in the fight against climate change’s impact on African food security, as this will help uncover and adopt new varieties less prone to higher temperatures and drought. This policy should be backed by expanding the weather station capacity with remote sensing tools to help make proper agricultural pest and disease forecasting and control.
Last but not least, taming inflation, controlling population growth, and encouraging the usage of renewable energy supplies are all essential elements in enhancing food security in Africa. African governments and development practitioners need to pay enough attention to these different policy perspectives when rolling out measures toward addressing the food insecurity issues in Africa and across the globe.
Disclosure statement
No potential conflict of interest was reported by the author(s).
Data availability statement
The data used for this study can be found online at: https://data.worldbank.org/indicator (accessed on 9 August 2022); http://www.fao.org/faostat/en/#data/FBSH (accessed on 9 August 2022); https://climateknowledgeportal.worldbank.org/download-Data (accessed on 9 August 2022)
Additional information
Funding
References
- Affoh, R., Zheng, H., Dangui, K., & Dissani, B. M. (2022). The impact of climate variability and change on food security in Sub-Saharan Africa: Perspective from panel data analysis. Sustainability (Switzerland), 14, 2. https://doi.org/10.3390/su14020759
- Ajayi, O. O., Mokryani, G., & Edun, B. M. (2022). Sustainable energy for national climate change, food security, and employment opportunities: Implications for Nigeria. Fuel Communications, 10, 100045. https://doi.org/10.1016/j.jfueco.2021.100045
- Al-Mulali, U., & Che Sab, C. N. B. (2018). Electricity consumption, CO2 emission, and economic growth in the Middle East. Energy Sources, Part B: Economics, Planning, and Policy, 13(5), 257–21. https://doi.org/10.1080/15567249.2012.658958
- Alvi, S., Roson, R., Sartori, M., & Jamil, F. (2021). An integrated assessment model for food security under climate change for South Asia. Heliyon, 7(4), e06707. https://doi.org/10.1016/j.heliyon.2021.e06707
- Areliano, M., & Boverb, O. (1995). Another look at the instrumental variable estimation of error-components models. 68(August 1990), 29–51. https://doi.org/10.1016/0304-4076(94)01642-D
- Arellano, M., & Bond, S. (1991). Some tests of specification for panel data: monte carlo evidence and an application to employment equations. Review of Economic Studies, 58(2), 277–297. https://doi.org/10.2307/2297968
- Asare-Nuamah, P. (2021). Climate variability, subsistence agriculture and household food security in rural Ghana. Heliyon, 7(4), e06928. https://doi.org/10.1016/J.HELIYON.2021.E06928
- Badolo, F., & Somlanare, K. R. (2013). Rainfall shocks, food prices vulnerability and food security: Evidence for Sub- Saharan African countries (pp. 1–20). https://www.afdb.org/fileadmin/uploads/afdb/Documents/Knowledge/AEC%202012%20-%20Rainfall%20Shocks%20Food%20Prices%20Vulnerability%20and%20Food%20Security-Evidence%20for%20Sub-Saharan%20African%20Countries.pdf
- Blundell, R., & Bond, S. (1998). Initial conditions and moment restrictions in dynamic panel data models (pp. 87). https://doi.org/10.1016/S0304-4076(98)00009-8
- Bourgault, M., Brand, J., Tausz-Posch, S., Armstrong, R. D., O’Leary, G. L., Fitzgerald, G. J., & Tausz, M. (2017). Yield, growth, and grain nitrogen response to elevated CO2 in six lentils (Lens culinaris) cultivars grown under Free Air CO2 Enrichment (FACE) in a semi-arid environment. European Journal of Agronomy, 87, 50–58. https://doi.org/10.1016/j.eja.2017.05.003
- Bouznit, M., & Pablo-Romero, M. D. P. (2016). CO2 emission and economic growth in Algeria. Energy Policy, 96, 93–104. https://doi.org/10.1016/j.enpol.2016.05.036
- Chakrabarti, K. D. (2015 September). In: Challenges in designing counterinsurgency policy: An institutionalist perspective. 50.
- Dasgupta, A., & Dasgupta, P. (2017). Socially embedded preferences, environmental externalities, and reproductive rights. Population and Development Review, 43(3), 405–441. https://doi.org/10.1111/padr.12090
- Dessie, W., & Ademe, A. S. (2017). Training for creativity and innovation in small enterprises in Ethiopia wondifraw mihret dessie and. International Journal of Training and Development. https://doi.org/10.1111/ijtd.12107
- FAO. (2015). Climate change and food security: Risks and responses (pp. 110). FAO. 978-92-5-108998-9. https://www.fao.org/3/a-i5188e.pdf
- FAO.,IFAD., UNICEF., & WHO. (2020). The State of Food Security and Nutrition in The World: Transforming Food Systems For Affordable Healthy Diets. Rome FAO. IEEE journal of selected topics in applied earth observations and remote sensing (pp. 320). 978-92-5-132901-6.
- FAO & WFP. (2020). Impacts of COVID-19 on food security and nutrition: Developing effective policy responses to address the hunger and malnutrition pandemic. In HLPE issues paper (Issue September).
- Fischer, S., Hilger, T., Piepho, H. P., Jordan, I., & Cadisch, G. (2019). Do we need more drought for better nutrition? The effect of precipitation on nutrient concentration in East African food crops. The Science of the Total Environment, 658, 405–415. https://doi.org/10.1016/J.SCITOTENV.2018.12.181
- Fuller, T. L., Sesink Clee, P. R., Njabo, K. Y., Tróchez, A., Morgan, K., Meñe, D. B., Anthony, N. M., Gonder, M. K., Allen, W. R., Hanna, R., & Smith, T. B. (2018). Climate warming causes declines in crop yields and lowers school attendance rates in Central Africa. Science of the Total Environment, 610–611, 503–510. https://doi.org/10.1016/j.scitotenv.2017.08.041
- Gebre, G. G., & Rahut, D. B. (2021). Prevalence of household food insecurity in East Africa: Linking food access with climate vulnerability. Climate Risk Management, 33, 100333. https://doi.org/10.1016/J.CRM.2021.100333
- GNAFC, & FSIN. (2021). Global Report on Food Crises: Joint Analysis for Better Decisions.
- Harris, F., Amarnath, G., Joy, E. J., Dangour, A. D., & Green, R. F. (2022). Climate-related hazards and Indian food supply: Assessing the risk using recent historical data. Global Food Security, 33, 100625. https://doi.org/10.1016/j.gfs.2022.100625
- Hoechle, D. (2007). Robust standard errors for panel regressions with cross-sectional dependence. Stata Journal, 7(3), 281–312. https://doi.org/10.1177/1536867x0700700301
- Holtz-Eakin, D., Newey, W., & Rosen, H. S. (1988). Estimating vector autoregressions with panel data. Econometrica, 56(6), 1371–1395. https://doi.org/10.2307/1913103
- IFAD, F. A. O., & WHO, U. N. I. C. E. F. (2020). The State of Food Security and Nutrition in the World 2020. Transforming Food Systems for Affordable Healthy Diets https://doi.org/10.4060/ca9692en
- IPCC. (1995). Climate Change 1995: The IPCC second assessment report. In Robert, T. Watson, M.C., Zinyowera, & Richard, H. Moss (Eds.), Scientific-Technical Analysis of Impacts, Adaptations, and Mitigation of Climate Change (pp. 399–426). IPCC.
- IPCC. (2014). Summary for Policymakers. In Climate Change 2014: Impacts, Adaptation, and Vulnerability. Part A: Global and Sectoral Aspects. Contribution of Working Group II to the Fifth Assessment Report of the Intergovernmental Panel on Climate Change [Field, C.B (pp. 1–32). Cambridge University Press. https://doi.org/10.1017/cbo9780511976988.002
- IPCC. (2018). Global warming? Nature. https://doi.org/10.1038/291285a0
- IPCC. (2022). The evidence is clear: The time for action is now. We can halve emissions by 2030. IPCC Working Group III. Vol. 151
- Kader, A. A. (2005). Increasing food availability by reducing postharvest losses of fresh produce. Acta Horticulturae, 682(682), 2169–2176. https://doi.org/10.17660/ActaHortic.2005.682.296
- Khandelwal, S., Parwez, S., & Mehra, M. (2022). Climate change: Effects on health and nutrition. Reference Module in Food Science. https://doi.org/10.1016/B978-0-12-821848-8.00131-1
- Kinda, S. R., Badolo, F., & Tajani, F. (2019). Does rainfall variability matter for food security in developing countries? Cogent Economics and Finance, 7(1), 1. https://doi.org/10.1080/23322039.2019.1640098
- Knox, J., Hess, T., Daccache, A., & Wheeler, T. (2012). Climate change impacts crop productivity in Africa and South Asia. Environmental Research Letters, 7(3), 034032. https://doi.org/10.1088/1748-9326/7/3/034032
- Leisner, C. P. (2020). Review: Climate change impacts on food security- focus on perennial cropping systems and nutritional value. Plant Science, 293, 110412. https://doi.org/10.1016/J.PLANTSCI.2020.110412
- Liang, K. Y., & Zeger, S. L. (1986). Longitudinal data analysis using generalized linear models. Biometrika, 73(1), 13–22. https://doi.org/10.1093/BIOMET/73.1.13
- Lloyd, S. J., Sari Kovats, R., & Chalabi, Z. (2011). Climate change, crop yields, and undernutrition: Development of a model to quantify the impact of climate scenarios on child undernutrition. Environmental Health Perspectives, 119(12), 1817–1823. https://doi.org/10.1289/ehp.1003311
- Lobell, D. B., & Field, C. B. (2008). Estimation of the carbon dioxide (CO2) fertilization effect using growth rate anomalies of CO2 and crop yields since 1961. Global Change Biology, 14(1), 39–45. https://doi.org/10.1111/J.1365-2486.2007.01476.X
- Loladze, I. (2014). Hidden shift of the ionome of plants exposed to elevated CO2 depletes minerals at the base of human nutrition. ELife, (2014, 3. https://doi.org/10.7554/ELIFE.02245
- Mekonnen, A., Tessema, A., Ganewo, Z., & Haile, A. (2021). Climate change impacts household food security and farmers’ adaptation strategies. Journal of Agriculture and Food Research, 6, 100197. https://doi.org/10.1016/J.JAFR.2021.100197
- Myers, S. S., Smith, M. R., Guth, S., Golden, C. D., Vaitla, B., Mueller, N. D., Dangour, A. D., & Huybers, P. (2017). Climate change and global food systems: potential impacts on food security and undernutrition. Annual Review of Public Health, 38(1), 259–277. https://doi.org/10.1146/annurev-publhealth-031816-044356
- Myers, S. S., Zanobetti, A., Kloog, I., Huybers, P., Leakey, A. D. B., Bloom, A. J., Carlisle, E., Dietterich, L. H., Fitzgerald, G., Hasegawa, T., Holbrook, N. M., Nelson, R. L., Ottman, M. J., Raboy, V., Sakai, H., Sartor, K. A., Schwartz, J., Seneweera, S., Tausz, M., & Usui, Y. (2014). Increasing CO2 threatens human nutrition. Nature, 510(7503), 139–142. https://doi.org/10.1038/nature13179
- Nelson, R., Kokic, P., Crimp, S., Martin, P., Meinke, H., Howden, S. M., de Voil, P., & Nidumolu, U. (2010). The vulnerability of Australian rural communities to climate variability and change: Part II-Integrating impacts with adaptive capacity. Environmental Science and Policy, 13(1), 18–27. https://doi.org/10.1016/j.envsci.2009.09.007
- OECD/FAO. (2016). OECD-FAO Agricultural Outlook 2016‑2025. OECD Publishing. https://doi.org/10.1787/agr_outlook-2016-en
- Omar, M. E. D. M., Moussa, A. M. A., & Hinkelmann, R. (2021). Impacts of climate change on water quantity, water salinity, food security, and socioeconomy in Egypt. Water Science and Engineering, 14(1), 17–27. https://doi.org/10.1016/j.wse.2020.08.001
- Painter, S. C., Popova, E., & Roberts, M. J. (2022). An introduction to East African coastal current ecosystems: At the frontier of climate change and food security. Ocean and Coastal Management, 216(November2021), 105977. https://doi.org/10.1016/j.ocecoaman.2021.105977
- Papke, L., Wooldridge, J., Papke, L., & Wooldridge, J. (2005). A computational trick for delta-method standard errors. Economics Letters, 86(3), 413–417. https://econpapers.repec.org/RePEc:eee:ecolet:v:86:y:2005:i:3:p:413-417 https://doi.org/10.1016/j.econlet.2004.07.022
- Roodman, D. (2009). How to Do xtabond2: An Introduction to Difference and System GMM in Stata, 1, 86–136. https://doi.org/10.1177/1536867X0900900106
- Rowhani, P., Lobell, D. B., Linderman, M., & Ramankutty, N. (2011). Climate variability and crop production in Tanzania. Agricultural and Forest Meteorology, 151(4), 449–460. https://doi.org/10.1016/J.AGRFORMET.2010.12.002
- Singh, S., Gupta, A. K., & Kaur, N. (2012). Influence of drought and sowing time on protein composition, antinutrients, and mineral contents of wheat. The Scientific World Journal, 2012, 1–9. https://doi.org/10.1100/2012/485751
- Singh, R. K., Joshi, P. K., Sinha, V. S. P., & Kumar, M. (2022). Indicator-based assessment of food security in SAARC nations under the influence of climate change scenarios. Future Foods, 5(October), 2021. https://doi.org/10.1016/j.fufo.2022.100122
- Stuch, B., Alcamo, J., & Schaldach, R. (2020). Projected climate change impacts on mean and year-to-year variability of the yield of key smallholder crops in Sub-Saharan Africa. Climate and Development, 1–15. https://doi.org/10.1080/17565529.2020.1760771
- SWAC, OECD Secretariat, RPCA. (2016). Food Issues: DeMOGraPHiC, UrBaN, MiGraTiON, and Security Challenges (Vol. 2, pp. 33). RPCA. https://www.google.com/url?sa=t&rct=j&q=&esrc=s&source=web&cd=&cad=rja&uact=8&ved=2ahUKEwiYh_OE7-X8AhUIgv0HHY_bBg8QFnoECAwQAQ&url=https%3A%2F%2Fwww.food-security.net%2Fen%2Fdocument%2Fmaps-facts-food-issues%2F&usg=AOvVaw0lumfWlrhYoDmrZkKakrrn
- Thorlakson, T., & Neufeldt, H. (2012). Reducing subsistence farmers’ vulnerability to climate change: Evaluating the potential contributions of agroforestry in western Kenya. Agriculture and Food Security, 1(1). https://doi.org/10.1186/2048-7010-1-15
- Thornton, P. K., & Herrero, M. (2015). Adapting to climate change in the mixed crop and livestock farming systems in sub-Saharan Africa. Nature Climate Change, 5(9), 830–836. https://doi.org/10.1038/nclimate2754
- Verschuur, J., Li, S., Wolski, P., & Otto, F. E. L. (2021). Climate change as a driver of food insecurity in the 2007 Lesotho-South Africa drought. Scientific Reports, 11(1), 1–9. https://doi.org/10.1038/s41598-021-83375-x
- Wang, J. (2010). Food security, food prices and climate change in China: A dynamic panel data analysis. Agriculture and Agricultural Science Procedia, 1, 321–324. https://doi.org/10.1016/j.aaspro.2010.09.040
- White, H. (1980). A heteroskedasticity-consistent covariance matrix estimator and a direct test for heteroskedasticity. Econometrica, 48(4), 817. https://doi.org/10.2307/1912934
- White, Halbert. (1984). Asymptotic Theory for Econometricians: Revised Edition (Economic Theory, Econometrics, and Mathematical Economics) (Revised ed.). Emerald Publishing Limited. 978-0127466521. http://www.academicpress.com
- Wiebe, K., Lotze-Campen, H., Sands, R., Tabeau, A., Van Der Mensbrugghe, D., Biewald, A., Bodirsky, B., Islam, S., Kavallari, A., Mason-D’Croz, D., Müller, C., Popp, A., Robertson, R., Robinson, S., Van Meijl, H., & Willenbockel, D. (2015). Climate change impacts agriculture in 2050 under a range of plausible socioeconomic and emissions scenarios. Environmental Research Letters, 10(8), 85010. https://doi.org/10.1088/1748-9326/10/8/085010
- Wossen, T., Alene, A., Abdoulaye, T., Feleke, S., Rabbi, I. Y., & Manyong, V. (2018). Poverty reduction effects of agricultural technology adoption: The case of improved cassava varieties in nigeria. Journal of Agricultural Economics. https://doi.org/10.1111/1477-9552.12296
- WU, J. Z., Zhang, J., GE, Z. M., Xing, L. W., HAN, S. Q., Shen, C., & Kong, F. T. (2021). Impact of climate change on maize yield in China from 1979 to 2016. Journal of Integrative Agriculture, 20(1), 289–299. https://doi.org/10.1016/S2095-3119(20)63244-0
- Zhao, C. X., He, M. R., Wang, Z. L., Wang, Y. F., & Lin, Q. (2009). Effects of different water availability at the post-anthesis stage on grain nutrition and quality in strong-gluten winter wheat. Comptes Rendus Biologies, 332(8), 759–764. https://doi.org/10.1016/J.CRVI.2009.03.003
- Zhao, C., Liu, B., Piao, S., Wang, X., Lobell, D. B., Huang, Y., Huang, M., Yao, Y., Bassu, S., Ciais, P., Durand, J. L., Elliott, J., Ewert, F., Janssens, I. A., Li, T., Lin, E., Liu, Q., Martre, P., Müller, C., & Asseng, S. (2017). Temperature increase reduces global yields of major crops in four independent estimates. Proceedings of the National Academy of Sciences of the United States of America, 114(35), 9326–9331. https://doi.org/10.1073/pnas.1701762114
- Zhu, C., Kobayashi, K., Loladze, I., Zhu, J., Jiang, Q., Xu, X., Liu, G., Seneweera, S., Ebi, K. L., Drewnowski, A., Fukagawa, N. K., & Ziska, L. H. (2018). Carbon dioxide (CO2) levels this century will alter the protein, micronutrients, and vitamin content of rice grains with potential health consequences for the poorest rice-dependent countries. Science Advances, 4(5), 1–9. https://doi.org/10.1126/sciadv.aaq1012