Abstract
Egg consumers are becoming increasingly concerned about animal welfare, so it is crucial to develop a better understanding of whether alternative housing systems lead to higher quality eggs. So, the current study was designed to assess the egg quality characteristics, sensory features, fatty acid profile, and cholesterol levels of eggs produced in enriched caging and barn housing systems. An experiment was conducted on Lohmann Brown Classic line laying hens (aged 28–48 weeks) fed analogous feed and housed in enriched cages and barn housing systems. Eggs’ quality traits, nutritional value, and sensory acceptance were evaluated. Hens kept in enriched cages had higher liveability and lay rates but lower feed consumption and feed conversion ratio (FCR) at almost all trial periods. Barn-laid eggs were heavier and had larger yolks; enriched cage eggs showed higher albumen, Haugh unit (28, 32, 36 weeks of age), stronger and thicker eggshells (32 weeks of age); barn-laid eggs stronger and thicker eggshell (48 weeks of age), higher SFA (start of the trial) and PUFA (at the end) contents. The differences between enriched cage and alternative barn housing systems are negligible, as no clear trend was discovered between them during different trial periods while hens were fed an identical diet.
1. Introduction
The growing interest in healthy and nutritious food production has led to a greater focus on animal welfare among consumers, especially when it comes to animal-derived products (Chand et al., Citation2018). As consumers shift towards healthier lifestyle choices, there is a growing concern about animal welfare in the production of animal-derived products. For instance, eggs are a valuable source of essential nutrients such as essential fats, proteins, vitamins, minerals, and trace elements (Hincke et al., Citation2019). The breeding quality of laying hens has been greatly improved in recent years due to advanced technology solutions (Preisinger, Citation2018; Wilson, Citation2017). Lithuanian egg producers favor the Lohmann Brown Classic line combination for its affordability and ability to produce high-quality eggs. In general, eggs are a good source of essential nutrients for both infants and adults and also contain biologically active components (Abeyrathne & Ahn, Citation2015). The quality of eggs is influenced by factors such as the shell, albumen, yolk, and the health of the laying hens. Additionally, there is also a concern about the correlation between animal welfare and the resulting nutritional value of eggs produced from alternative housing systems for laying hens.
Housing systems can improve animal well-being and provide higher-quality production, and this is a non-genetic feature (Ghanima, Mahmoud, Elsadek, et al., Citation2020). Numerous studies have highlighted that housing systems affect not only animal welfare but also animal performance and production quality (Campbell et al., Citation2019; El-Sabrout, Citation2018; Ghanima, Mahmoud, Elsadek, et al., Citation2020; Wei et al., Citation2019). Poultry farmers are not exempt from this phenomenon. Therefore, it is crucial to understand how different housing systems affect not only the performance of laying hens but also egg nutrition and quality factors. An important question arises as to whether eggs produced by hens housed in cage-free barn systems have a higher quality than those produced by hens in enriched cages.
Egg production in Lithuania, as in other European countries, primarily relies on enriched cage systems. However, there is a growing interest in eggs from non-cage housing systems, particularly barns. Eggs from hens kept in cage-free systems, where they are free to roam across large indoor sheds, are classified as “barn-laid” eggs. The hens are constantly indoors and do not have access to the outdoors during daylight hours, which is the only difference between this system and the free-range system. Numerous scientific studies have demonstrated that the housing system can significantly influence the quality features of eggs, including egg weight, shape, eggshell and colour characteristics, albumen, and yolk traits (Batkowska & Brodacki, Citation2017; Ferrante et al., Citation2009; Jones et al., Citation2015; Konkol et al., Citation2020). In order to ensure the welfare of laying hens and produce high-quality eggs, it is crucial to have a thorough understanding of the benefits and drawbacks of different housing systems. Furthermore, given that selecting a high-quality product is of great importance to consumers, the present study was undertaken to investigate the egg quality characteristics, sensory attributes, fatty acid profile, and cholesterol level of eggs produced by Lohmann Brown Classic laying hens in enriched caging and barn housing systems. The hens were provided with an analogue diet to ensure uniformity of nutrient intake.
2. Materials and methods
2.1. Experimental design
The study was conducted with the laying hen line combination Lohmann Brown Classic by collecting eggs from an industrial poultry farm in Lithuania, which has two types of laying hen housing systems. During the trial, the laying hens were housed in two types of housing systems: 1) in enriched cages (n = 30 hens/cage; n = 15000 laying hens/housing system), with a nest, perch, nipple drinker and feeder; single cage dimensions: 244 cm (length) x 94 cm (width) x 80 cm (height); for 1 hen 761.28 cm2 of cage area; 2) in barn on the deep litter (n = 15000 laying hens/housing system), where at least 250 cm2 of floor for 1 laying hen (no more than nine laying hens kept per 1 m2); single hole laying nest with 10, 9 or 8 nest holes per section side (sections length 244 cm); in addition to more space for feeding, drinking, and laying eggs, each bird can walk freely, spread its wings, and fly around. The birds in both housing systems were fed the same combination of feeds (for 1 bird, about 125 g feed/day), the qualitative characteristics of which are given in Table . Feeding and care conditions were in accordance with the Lohmann Brown Classic line combination guidelines (The Lohman Tierzucht guide, Citation2019).
Table 1. Feed ingredient composition and nutrient content (28–48 weeks of age)
The microclimate of the different poultry houses was recorded during the trial; all data was obtained directly from the poultry farm. Throughout the trial, microclimate parameters were measured at three different sites in the poultry house: the entrance, the middle, and the end wall. The averages of the measurements collected during the trial are presented Figure .
Figure 1. Microclimate parameters of different housing systems. Note: 1 Recommended norm according to the Lohmann Brown Classic Line Combination Guidelines (The Lohman Tierzucht guide, Citation2019).
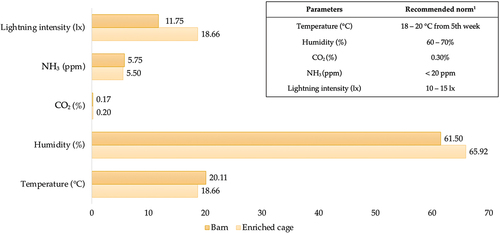
During the trial, all eggs were counted and weighed daily in a poultry farm. The average body weight (g) of the hens was recorded; liveability (%), rate of lay (%), average weight of 1 egg (g), feed consumption for 1 bird (g/bird), and feed conversion ratio (FCR) were measured and calculated for each trial period. All productivity data is presented as averages for each trial period.
2.2. Egg and sample collection
A total of 720 eggs (360 from each housing system) were collected from 28 to 48 weeks of age. A total of 60 eggs from each housing system (n = 60 eggs/housing system; total n = 60 × 2 housing systems = 120 eggs) were collected every four weeks (28, 32, 36, 40, 44 and 48 weeks of age) until the end of trial (at 48 weeks of age). To minimise variation between results, all the sample eggs were taken at random from different housing systems on the same day and stored at the same temperature and humidity levels until further analysis.
Immediately after each egg collection, the following egg quality indicators were determined (n = 60 eggs/housing system): general egg quality, eggshell properties, and yolk colour intensity. The following analyses were performed on egg yolk mixtures mixed from 6 separate yolks (1 mixture/sample = 6 egg yolks; n = 10 yolk mixtures/housing system) three times throughout the test period (total n = 10 × 3 = 30 yolk mixtures/housing system; at the beginning, middle, and end of the trial): yolk chemical composition; determination of the fatty acid profile; and cholesterol levels.
2.3. Egg quality assay
The egg weight, albumen height, Haugh unit, and yolk colour intensity (n = 60 eggs/housing system) were determined using the multifunctional automatic egg characteristics analyser Egg Multi-Tester EMT-5200 (Robotmation Co., Ltd., Chuo, Japan). The weight of the yolk was weighed with a Kern PBS/PBJ scale (Kern & Sohn GmbH, Balingen, Germany).
2.3.1. Eggshell properties
The strength of the eggshell (n = 60 eggshells/housing system) was determined by the Egg Shell Force Gauge MODEL—II (Robotmation Co., Ltd., Chuo, Japan) device, the weight of the eggshell with and without membrane by the scales Kern PBS/PBJ (Kern & Sohn GmbH, Balingen, Germany) and the thickness of the eggshell (in the middle) by the electronic micrometre Mitutoyo Digimatic Micrometer (Mitutoyo, Sakado, Japan).
2.3.2. Yolk colour by coordinates L*a*b*
Yolk colour by coordinates (n = 60 yolks/housing system) was determined by using the Chroma Meter CR-410 Colour Gauge (Konica Minolta, Inc., Osaka, Japan). Respectively to the coordinates on the CIE-LAB international colorimetric scale, which is based on variations between three primary complementary colour pairs: red-green (a*), yellow-blue (b*), and black-white (L*). The third metric, specific lightness L*, is a function of reflectance, or the ratio between the intensity of reflected light and the intensity of input light, which ranges from 0 (black) to 100 (white). A white calibration was used to standardize the photometer.
2.4. Yolk chemical composition
To determine the following chemical composition parameters after general analysis remained, egg yolks were mixed (6 egg yolks = 1 sample) with 10 replicated yolk mixtures from each housing system were analysed (n = 10 yolk mixtures/housing system). For yolk dry matter determination, it was dried in an oven at 105 °C until it reached a constant weight. The Kjeldahl method was used to determine the protein content (Helrich, Citation1990). The Soxhlet extraction method was used to extract fat using petroleum ether (a boiling range of 40–60 °C). After three hours of incineration in a muffle furnace at 550 °C (Commission Regulation (EC) No. 152/2009), the ash content was estimated.
2.5. Yolk fatty acids profile and cholesterol content analysis
2.5.1. Fatty acids profile samples’ preparation and analysis conditions
Extraction of lipids from egg yolks (n = 10 yolk mixtures/housing system) for fatty acid analysis was performed with chloroform/methanol (2:1 v/v) as described by Folch et al. (Citation1957). The total lipids’ fatty acid methyl esters (FAME) were synthesized using the procedure described by Christopherson and Glass (Citation1969). The fatty acid methyl esters (FAMEs) were analysed using a gas chromatograph, GC–2010 Plus (Shimadzu Corp., Kyoto, Japan), fitted with a flame ionization detector. The separation of FAME was affected on a Restek capillary column Rt-2560 (100 m; 0,25 mm ID; 0,25 μm df) (Restek corporation, Bellefonte, PA, USA) by temperature programming from 160 °C to 230 °C. The temperature of the injector was 240 °C, the detector was 260 °C, and the flow rate of carrier gas (nitrogen) was 0.79 ml/min. The injection volume was 1.0 μl. The FAMEs were identified by comparing their retention times with those of the authentic standard mixtures. The relative content of each fatty acid in the sample was expressed as a relative percentage of the sum of the fatty acids (% of the total acid content); it was determined using a chromatographic data processing program GCsolution (Ver. 2.32; Shimadzu Corp., Kyoto, Japan).
The overall content of saturated (SFA), monounsaturated (MUFA), and polyunsaturated (PUFA) fatty acids, PUFA/SFA, and omega-6/omega-3 (n6/n3) ratios were calculated using the average quantity of each fatty acid.
2.5.2. Cholesterol content samples’ preparation and analysis conditions
Cholesterol in standard mixtures and egg yolk extracts (n = 10 yolk mixtures/housing system) was separated by the method described by Zhang et al. (Citation2004) with some modifications. Saponification of the sample was carried out by adding 2.0 mL of 50% potassium hydroxide solution, then incubated at 50 °C for 60 minutes with constant agitation in a GFL 1083 water bath (GFL GmbH, Burgwedel, Germany). Prior to high-performance liquid chromatography (HPLC) analysis, the sample extract was centrifuged at 4000 rpm for 20 minutes (Boeco C-28A, Hettich Zentrifugen, Hamburg, Germany). The organic phase (0.5 mL) was pipetted into a 1.5 mL HPLC vial and evaporated to dryness under a nitrogen stream. The residue was dissolved in 0.5 mL of mobile phase (acetonitrile: 2-propanol, 55:45). A Shimadzu HPLC system (Shimadzu corp., Kyoto, Japan) was used for the cholesterol analysis, which included a system controller SCL-10A, solvent delivery module LC-10AT, auto injector SIL-10AD, UV-Vis detector SPD-10AV, column oven CTO-10AC, and on-line degasser DGU-14A. HPLC analysis was carried out at ambient temperature on a LiChrospher RP-18 e column (150 × 4.6 mm, 5 µm) (Alltech Associates Inc, Deerfeld, USA) with a guard (LiChrospher RP-18 e, 7.5 × 4.6 mm, 5 µm) (Alltech Associates Inc, Deerfeld, USA). The eluate was monitored at 208 nm. The mobile phase was an isocratic mixture of acetonitrile and 2-propanol (55:45 v/v) and the flow rate was 1.00 mL/min. Sample injection volumes were 10 µL.
The results were calculated by measuring the peak areas of the sample and the standard solution. Data collection and evaluation were performed by using operating system Workstation LC solution (Shimadzu corp., Kyoto, Japan).
2.6. Egg sensory analysis
A sensory profile test was used to assess the sensory features of additionally collected eggs (n = 40 eggs/housing system) at the end of the trial (48 weeks of age). A trained team of 8 evaluators examined pre-selected egg samples and chosen concepts to represent their sensory attributes. Using mathematical statistical approaches, a method of sensory attributes is developed for each product, displaying the intensity of each property (Parpinello et al., Citation2006). The test was conducted by a panel of eight evaluators who had been chosen and trained to work in accordance with ISO 8586. A fully balanced randomised sampling design using 5 replicate eggs (n = 5 eggs/housing system for 1 evaluator; 5 replicate eggs x 8 evaluators = 40 eggs from each housing system) were used to evaluate the sensory profile. The intensity of each albumen and yolk property of the investigated products was evaluated on a 9-step graduated numerical scale: 1 indicates that the property was not felt, 5 indicates that it was moderately expressed, and 9 indicates that it was very strongly expressed.
2.7. Statistical analysis of results
Within all trial periods, statistical tests were performed to compare enriched cage and barn housing systems produced egg marginals between quality traits while feeding the same diet. All obtained data analysis was performed by SPSS for Windows, version 25.0 (IBM Corp., released in 2017, Armonk, NY, USA) with the following replicates per housing system for each analysis:
• each trial period of laying hen productivity (n = 15000 laying hens/enriched cages; n = 15000 laying hens/barn);
• general egg quality parameters, eggshell properties, and yolk colour intensity every four weeks (n = 60 egg/housing system; n = 60 eggs x 6 trial periods = 360 eggs/housing system)
• yolks chemical composition, fatty acids, and cholesterol levels every 6 weeks (n = 10 yolk mixtures (each containing 6 yolks)/housing system; n = 10 yolk mixtures x 3 trial periods = 30 yolk mixtures/housing system for each analysis);
• eggs sensory analysis at the end of the trial (n = 5 eggs/housing system for 1 evaluator; 5 replicate eggs x 8 evaluators = 40 extra eggs collected from each housing system).
Before each data package analysis, the Kolmogorov-Smirnov test was performed for normality determination. Then, for normally distributed data, a parametric independent T-test was conducted on all obtained data within all trial periods to detect differences among the two housing systems. To determine the relationship between the variables, standard error of the means (SEM) was calculated and presented in each table. A calculated P value of less than or equal to 0.05 (p ≤ 0.05) was considered statistically significant.
3. Results
3.1. Laying hen productivity
The productivity parameters of the laying hens in both housing systems were recorded daily, and the average productivity results for each trial period are given in Table . In both housing systems, the trial began with the same number of hens (15000 laying hens). At 28 weeks of age, hens kept in barn housing system had a higher average body weight (1873.00 g) compared to hens from enriched cages (1819.33 g; p < 0.05). On the other hand, the hens in the enriched cage housing system had a higher significant average body weight (1894.00 g), weighing 22.33 g more than the barn hens (1871.67 g) at 36 weeks of age (p < 0.05). The liveability rate was higher in the enriched housing system in all trial periods. To be more precise, it was 0.42, 0.73, 1.11, 1.72, 2.41, and 3.17% higher compared with the barn at 28, 32, 36, 40, 44, and 48 weeks of age (p < 0.05). Almost every trial period, the rate of lay was found to be higher for hens housed in the enriched cage housing system, continuing the trend observed with higher bird liveability values. When laying hens were 32, 36, 40, and 44 weeks old, the enriched cage housing system had a higher rate of lay (94.83, 94.48, 94.47, and 93.83%, respectively) compared to the barn (90.78, 93.41, 92.59, and 91.53%, respectively) (p < 0.05). Except at the end of the trial (48 weeks of age), a 10.98% higher rate of lay was observed in the barn housing system compared to enriched cages (p < 0.05). The differences in the average weight of 1 egg between housing systems were negligible throughout the trial periods. Except for 40 weeks of age, when barn hens produced 0.70 g heavier eggs, and 48 weeks of age, when they produced 1.41 g heavier eggs in the enriched cage system (p < 0.05). Except for the first trial period, average feed consumption for one hen varied significantly across all trial periods. At 32, 36, 40, 44, and 48 weeks of age, feed consumption in the barn housing system was 6.88, 16.37, 16.25, 13.65, and 20.01 g/bird higher than in the enriched cage (p < 0.05). Feed conversion ratio (FCR) data was unevenly distributed throughout the trial periods: higher FCR was discovered at 28 and 36 weeks at the enriched housing system compared to the barn, but lower in the remaining trial periods (32, 40, 44, and 48 weeks of age; p < 0.05).
Table 2. Average laying hen productivity traits while kept in enriched cages and barn housing systems at 28, 32, 36, 40, 44, and 48 weeks of age
3.2. Egg quality
General egg quality traits were examined six times (at 28, 32, 36, 40, 44, and 48 weeks of age) every four weeks (Table ). At 28, 32, and 36 weeks of age, barn-laid eggs were 2.33, 5.59, and 2.62 g heavier compared to eggs from the enriched cage system, respectively (p < 0.05). The same tendency continued until the trial’s end, but the results obtained at 40, 44, and 48 weeks of age were considered not significant (p > 0.05). The weight of the egg yolk was apportioned in the same way that the total weight of the egg was. Compared to the enriched cage, the barn system hens produced heavier egg yolks at 28, 32, and 36 weeks of age (p < 0.05). Eggs from the enriched cage housing system showed higher albumen height at 28 and 32 weeks of age, respectively, by 0.85 and 0.94 mm, compared to barn-laid eggs (p < 0.05). As Haugh unit is directly correlated to albumen height, the same trend was observed after the study’s findings: the Haugh unit at 28 and 32 weeks of age was found to be slightly higher in barn-laid eggs than in enriched cage eggs (p < 0.05).
Table 3. Quality features of egg from enriched cages and barn housing systems at 28, 32, 36, 40, 44, and 48 weeks of age (n = 60 eggs/housing system at each trial period)
3.2.1. Egg shell properties
The differences between eggshells from different laying hen housing systems are given in Table . It was revealed by the results of the study that at 32 weeks of age, eggs from enriched cages had stronger eggshells (p < 0.05). The shell strength was 49.61 N, while in the barn-laid eggs it was 45.67 N (p < 0.05). Furthermore, in the last trial period (48 weeks of age), barn-laid eggs had a stronger eggshell (46.27 N), while eggs from enriched cages had a weaker shell (40.95 N) (p < 0.05). Significant differences were obtained at 48 weeks of age after weighing the eggshells with and without the membrane: barn-laid eggshells, respectively, with and without membranes, were heavier by 0.53 and 0.29 g compared to enriched cage eggs (p < 0.05). However, no significant differences between housing systems when comparing eggshell thickness were identified throughout all trial periods (p > 0.05).
3.2.2. Yolk colour
An egg multi-tester was used to measure the colour intensity of egg yolks, and a colorimeter was used to establish the colour coordinates (Table ). Eggs from an enriched cage system at 36 and 44 weeks of age showed a more intense yolk colour compared to barn-laid yolks (p < 0.05). The colour intensity of barn egg yolks was found to be greater than that of enriched cages at only 40 weeks of age (p < 0.05). According to the coordinate L* (lightness), eggs from barn housing systems were found to be 0.88, 1.78, 0.82, and 1.76 units lighter than those from enriched cages at 32, 36, 44, and 48 weeks of age, respectively; except at 40 weeks of age, when egg yolks were found to be 2.34 units lighter in enriched cages compared to barn-laid (p < 0.05). Egg yolks from the enriched cages were 4.15 and 6.14 units redder (according to the a* coordinate) than those from the barn at 36 and 48 weeks of age, respectively (p < 0.05). At 40 weeks of age, a very significant difference was detected between the groups when the hens from the barn produced 10.04 more reddish egg yolks compared to the hens in enriched cages, according to the a* coordinate (p < 0.05). Egg yolks from the barn housing system were more yellowish at coordinate b* by 10.72 and 2.95 units compared to enriched cage egg yolks at 40 and 44 weeks of age, respectively (p < 0.05).
3.3. Chemical composition
The most important indicators reflecting the chemical composition of egg yolk were identified (Table ). Nevertheless, the findings suggest that the laying hen housing system has no impact on the egg yolk’s dry matter, protein, fat, or ash content, as no significant differences were detected (p > 0.05).
Table 4. The chemical composition of egg yolks from enriched cage and barn laying hen housing systems at the trial’s start, middle, and end (n = 10 yolk mixtures/housing system at each trial period)
3.4. Fatty acid profile and cholesterol content
The fatty acid profiles of egg yolks from enriched caging and barn housing systems at the start (28 weeks of age), middle (38 weeks of age), and end (48 weeks of age) are presented in Table . Among the 16 fatty acids determined, palmitic (C16:0), elaidic (C18:1 n-9), and linoleic (C18:2 n-6) acids were most prevalent in the egg yolk profile throughout the trial period. At the beginning of the trial, egg yolks from hens reared in an enriched cage housing system were 0.04%, 0.25%, and 0.13% higher in heptadecanoic (C17:0), arachidonic (C20:4 n-6), and eicosapentaenoic (C20:5 n-3) acids than barn-laid eggs, respectively (p < 0.05). However, egg yolks from the barn system had a nearly 2-fold higher concentration of nervonic acid (C24:1) than yolks from the enriched cage system (p < 0.05).
Table 5. Fatty acid profiles of egg yolks from enriched cage and barn laying hen housing systems at the trial’s start, middle, and end (n = 10 yolk mixtures/housing system at each trial period)
In terms of total saturated fatty acids (SFA) in egg yolks, it was greater than 35% of the total fatty acid content in all housing systems during all trial periods. Only at the start of the trial was a statistically significant difference observed when 0.88% more SFA was found in barn-laid egg yolks (p < 0.05). The findings regarding monounsaturated fatty acids (MUFA) were not significant across all time periods (p > 0.05). Significant differences in PUFA values were obtained at the end of the trial: 1.38% greater PUFA content in barn-laid egg yolks compared to caged birds (p < 0.05).
The calculation of the PUFA/SFA ratio revealed no trend throughout the trial periods. For example, at the beginning of the trial, this ratio was higher in yolk samples from the enriched cage housing system (0.84%) compared to barn-laid eggs (0.83%) (p < 0.05). At the end of the trial, the results were shifted in the opposite direction: a higher PUFA/SFA ratio was achieved in barn-laid yolk samples (0.90%) and a slightly lower ratio in the enriched cage (0.84%) (p < 0.05).
3.4.1. N-3, n-6, and cholesterol content
During the trial, the content of n-3 and n-6 fatty acids and their ratio in egg yolks were assessed (Table ). The results demonstrated that laying hen housing systems seemed to have no effect on egg yolk n-3 or n-6 in any of the trial periods (p > 0.05). Nevertheless, a few exceptions were obtained. Firstly, higher levels of n-3 (4.24%) were detected in the yolks of caged hen eggs at the start of the trial (p < 0.05). However, in the middle of the trial, a higher n-6/n-3 ratio, compared to enriched cage egg yolks, was found in the barn-laid yolk samples, which reached 7.50% (p < 0.05). After cholesterol levels analysis in egg yolks, no significant differences across housing systems were discovered (Table ; p > 0.05).
Table 6. The total content of essential fatty acids n-3 and n-6, their ratio and cholesterol levels in egg yolks from enriched cage and barn laying hen housing systems at the trial’s start, middle, and end (n = 10 yolk mixtures/housing system at each trial period)
3.5. Egg sensory profile
The sensory properties of the albumen and yolk of the different egg types were nearly identical (Figure ). There was no difference in the intensity of albumen or yolk odour based on the method of housing, and no extraneous odour or taste was observed in any of the groups evaluated (P > 0.05; Figure ). The total odour intensity of the yolk is the only feature that shows a tendency for eggs to differ. The total odour intensity of the yolk is the only feature that differs between eggs, being slightly higher in enriched cage eggs than in barn-laid eggs (p < 0.05; Figure . (B)). All eggs’ albumen colour was homogeneous, and the yolk colour was of moderate intensity. However, the laying hens’ housing conditions had no impact on the overall sensory features of the eggs.
4. Discussion
In the current study, two different laying hen housing systems on the same poultry farm were investigated while feeding hens analogues of standard compound feed. This study is highly relevant for egg consumers, who seek to put not only superior-quality animal products on their tables but also vital input by choosing production with the highest animal welfare standards. Although scientists’ worldwide research-based results do not always imply significant differences between the various housing systems of laying hens (Ghanima, Mahmoud, Alagawany, et al., Citation2020; Ketta et al., Citation2020), there are several researchers’ reports to the contrary, indicating quality differences between eggs from cage and alternative systems (Lewko & Gornowicz, Citation2011; Philippe et al., Citation2020; Pistekova et al., Citation2006; Popova et al., Citation2020; Samiullah et al., Citation2017; Sokołowicz et al., Citation2018b).
4.1. Laying hens productivity
Regular monitoring of hen housing systems and egg production methods is essential to ensuring the welfare of the birds. Various factors such as diseases, musculoskeletal and foot health, pest and parasite burden, behaviour, stress, psychological conditions, diet, and genetics can all affect the welfare of laying hens (Lay et al., Citation2011). Productivity indicators are essential for poultry farmers who keep laying hens in any housing system, and body weight is one of the critical factors affecting egg production efficiency as it is positively correlated with heavier egg development. In the recent study, it was observed that the average body weight of laying hens did not exhibit a consistent trend across different trial periods. Notably, significant differences were found only at 28 weeks of age in the higher-weight hens from the barn and at 36 weeks of age in the higher-weight hens from the enriched cage housing system. Another crucial factor to consider in terms of productivity is the liveability of hens. Throughout all trial periods, the liveability rate was found to be significantly higher in the enriched cage housing system compared to the barn. High liveability rates are of utmost importance to the poultry industry, as they indicate the ability of hens to survive and produce eggs. Mortality rates can have a significant impact on egg production as well as on the economic viability of the farming operation. Hence, the liveability of hens is a critical productivity measure that needs to be closely monitored and optimised to ensure the sustainability of the poultry farming industry. Recent results are aligned with Weeks et al. (Citation2016) research, in which they investigated the relevance of welfare, productivity, sustainability, and mortality in laying hens kept in different housing systems and discovered that hens reared in alternative housing systems (barn, free range, and free-range aviary) had a higher mortality rate compared to cage systems. As with the liveability rate, a higher rate of lay was found in hens that were kept in enriched cages, except for the last trial period, when the hen rate of lay decreased by about 10% in the mentioned housing system. Due to their higher egg production, highly productive laying hens have been shown to possess a higher energy and nutrient demand than moderately productive layers (Lieboldt et al., Citation2015). Consequently, according to the recent research, while a greater rate of lay was observed in the laying hens in the enriched cage housing system, their feed intake (g/bird) was significantly lower than that of hens kept in a barn, which had a lower rate of lay but higher feed consumption. The same trend as laying hens’ feed consumption—a higher feed conversion ratio (FCR)—was also found in the barn housing system. Studies that were previously summarized and published between 1980 and 2003 found that hens kept in aviaries or other alternative housing systems had lower productivity (feed consumption and FCR) than chickens kept in cages, with no correlation between the production method and mortality or cannibalism (Aerni et al., Citation2005). Furthermore, the microclimate data provided by the poultry farm showed that the enriched cage housing system had an average light intensity of 18.66 lx during the entire trial period, which was higher than the recommended norm of 10–15 lx. This could be linked to the lower feed consumption observed in this housing system, as laying hens are known to be sensitive to intense lighting conditions.
4.2. General egg quality features
Scientific research into the effect of hen housing systems on egg weight has produced a variety of outcomes. In similar housing systems to the recent experiment, Samiullah et al. (Citation2017), Ghanima, Mahmoud, Alagawany, et al. (Citation2020), and Ketta et al. (Citation2020) discovered no impact of the housing method on egg weight. Other researchers discovered significant differences in housing systems: eggs from hens raised in enriched cages had lower egg weight results than those raised on the floor (Araujo Netto et al., Citation2017), egg weight was significantly heavier when produced in an enriched cage system compared to litter (Englmaierová et al., Citation2014), and eggs from organic systems were heavier than those from litter systems (Dalle Zotte et al., Citation2013). A recent study revealed that hens raised in barn systems produced heavier eggs than those raised in enriched cages at 28, 32, and 36 weeks of age. This trend persisted even after considering the proportion of egg yolk weight relative to the total weight of the egg, which indicated that the barn-laid egg yolk weight was increased. However, there is a lack of consistent patterns or evident trends among the findings of many scientists on this topic. Furthermore, variables such as laying hen line combinations, feeding, and other factors that affect the growing environment can influence egg weight and other quality indicators.
The Haugh unit has become the most widely used measurement of albumen, or internal egg quality, and it is widely regarded as the fundamental standard for determining interior egg quality. The Haugh unit is defined as the relationship between egg weight and the height of the albumen (Jones, Citation2012). The Haugh unit is directly related to albumen height and can be used to assess the freshness of eggs; higher Haugh unit values indicate superior albumen quality and are associated with better egg freshness (Champati et al., Citation2020). In a recent study, eggs produced by hens in the enriched cage system during the trial had higher albumen height, which resulted in a significantly increased Haugh unit in comparison to barn-laid eggs at 28 and 32 weeks of age. Recent findings support those of Englmaierová et al. (Citation2014), who demonstrated that eggs from an enriched cage have a higher Haugh unit than those from a litter system, which is comparable to a barn.
The eggshell is a multilayer bioceramic composite structure consisting of a crystalline mineral phase. The building blocks of this structure are primarily composed of calcium carbonate, with calcite being the most common form. Within and upon this framework, proteins such as glycoproteins, proteoglycans, collagen, keratins, and dermatans are synthesised in the form of fibres (Hincke et al., Citation2019). Along with its crystalline structure, eggshell is a brittle element that can develop microcracks when subjected to damage against solid things such as a cage floor (Bain et al., Citation2006). The use of cage-free barn systems for hen rearing may aid in preventing eggshell breakage due to the softer surface (deep litter). However, a recent study found no significant difference in eggshell strength between hens raised in enriched cages versus those in barn housing systems. Stronger eggshells were detected in the enriched cage system at 32 weeks of age (49.61 ± 0.97 N), while eggshells in the barn were stronger at 48 weeks of age (46.27 ± 1.30 N). The strength of the eggs (rate of compression) typically ranges between 30 and 50 N (Hamilton & Bryden, Citation2021). Therefore, both housing systems’ eggshells met the strength norms, according to the obtained data. There are a few publications that report on the interior and shell quality outcomes (Englmaierová et al., Citation2014; Sekeroglu et al., Citation2010; Yenice et al., Citation2017). In general, studies have shown that hens raised in cages tend to have higher shell strength values compared to those in barns or other alternative systems. However, differences in breeding and management practises can also impact these results. In the recent study, it was found that barn-laid eggs had stronger eggshells with and without membranes, resulting in heavier eggshells compared to caged hen eggs at 48 weeks of age. Despite conflicting with some previous studies, the findings indicated that barn-laid eggs had better shell quality traits than those from enriched cages at the end of the 48-week trial. It is worth noting that the thickness of eggshells was not affected by different housing methods.
The yolk colour is a crucial indicator of egg quality that may influence consumer preferences (Lordelo et al., Citation2017; Samiullah et al., Citation2017; Sokołowicz et al., Citation2018b). The yolk colour of an egg is influenced by several factors, including the type of feed, supplements, and access to natural soil, where hens can forage for additional feed. The concentration of carotenoid pigments in the hen’s diet is the primary determinant of yolk colour intensity, which can vary depending on consumer preferences in different countries (Dalle Zotte et al., Citation2021; Philippe et al., Citation2020). Based on the data obtained from egg multi-tester analyses, the enriched cage system produced yolks with the deepest colour at 36 and 44 weeks of age. On the other hand, the barn-laid egg yolks exhibited a higher colour intensity only when the laying hens were 40 weeks old. According to colour by coordinates, barn-laid egg yolks were lighter (L*) during most of the trial periods, except when laying hens were 40 weeks of age; at 36 and 48 weeks of age, yolks from the enriched cages were redder (a*), but at 40 weeks, they were less red compared to barn-laid. According to coordinate b*, more yellow yolk was obtained in barn-laid eggs at 40 and 44 weeks of age. It is important to note that the same feed, without any added colour feed additives, was used across all the different housing systems analysed in recent research. In a study by Philippe et al. (Citation2020), three egg production systems—battery cages, enriched cages, and an aviary system (similar to a barn)—were compared using the same feed in all groups. However, eggs produced in the aviary system had a more intense yolk colour compared to both cage systems, which can be attributed to hens pecking and potentially consuming litter substrate, resulting in a deeper yolk colour. Additionally, Singh et al. (Citation2009) proposed that the intensity of yolk colour may be influenced by the level of egg production, with paler yolks indicating higher productivity. Nevertheless, it was shown by the recent study that the outcomes of yolk colour assessment differed depending on the trial period, with no clear trend identified throughout the entire trial. Therefore, despite the same feed being used for all experimental treatments, diverse yolk colour data distribution was observed during the trial.
4.3. Egg yolk chemical composition
The chemical composition of eggs can be influenced by a variety of factors, including the genetic origins of the laying birds, bird age, and housing and rearing strategies, with particular emphasis on feeding and egg storage conditions (Lordelo et al., Citation2017; Quan & Benjakul, Citation2019; Rakonjac et al., Citation2018; Sokołowicz et al., Citation2018b). In the recent investigation, it was found that the chemical composition of egg yolks was not significantly influenced by different housing systems. Specifically, the levels of dry matter, protein, fat, and ash in the yolks were not affected by the housing and rearing strategy employed. Another scientist Dalle Zotte et al. (Citation2021) performed an experiment to compare purchased organic eggs with laying hens’ eggs from barn and cage system farms. The experiment’s findings showed that organic eggs purchased from the market had lower levels of protein, fat, and ash when compared to barn and caged eggs. Nevertheless, hens reared in barns and cages typically consume nutritionally balanced diets tailored to meet their specific dietary needs, which may account for the absence of significant chemical composition differences between the two types of eggs in the recent study.
Numerous scientific investigations have been conducted to validate this statement, and the outcomes consistently demonstrate that the fatty acid composition of eggs, irrespective of the avian species, is often influenced by the fatty acid composition of the feed (Dalle Zotte et al., Citation2021; Hammershøj & Finn Johansen, Citation2016). In the recent study, it is important to note that the hens in the various housing systems were given an identical diet, which is a critical factor to consider when evaluating the results. When comparing the different housing systems and analysing individual fatty acids, significant differences were observed only at the beginning of the experiment, which implies that feeding practises and the type of feed given to the laying hens may be more influential in altering the fatty acid composition of eggs than the housing system itself. Higher amounts of arachidonic (C20:4 n6) and eicosapentaenoic (C20:5 n3) acids were found in egg yolks from an enriched cage system. Additionally, during the same testing period, barn-laid egg yolks were shown to have nearly twice the quantity of nervonic acid (C24:1) than eggs from cages. The recent study’s results were not significantly different as laying hens from different housing systems were fed the same diet, and the lipid profile is known to be influenced by the feed, which is a reasonable explanation. However, the total amount of saturated fatty acids (SFAs) at the start of the trial was slightly higher in barn-laid eggs after estimating the total content of the individual fatty acid groups. Furthermore, no influence of different housing systems was observed when comparing total monounsaturated fatty acid (MUFA) content. Typically, eggs in dietary recommendations are considered phospholipid-enriched and low-cost foods, as the polyunsaturated fatty acids (PUFAs) sourced in egg yolk are more beneficial to maximise their efficacy (Xiao et al., Citation2020). At the end of the study, the barn-laid housing system had a notable impact on the presence of PUFAs, resulting in a higher concentration in the yolks of eggs. A similar trend was observed in the research conducted by Popova et al. (Citation2020), which also found a significant increase in PUFA content in eggs from alternative housing systems compared to conventional.
To evaluate the nutritional value of lipids, the PUFA/SFA and n-6/n-3 ratios are often used, with ratios of PUFA/SFA greater than 0.45 and n-6/n-3 less than 4 considered sufficient to prevent the development of ischemic heart disease (Simopoulos, Citation2002; Tomaszewska et al., Citation2021). In the case of the study, the PUFA/SFA ratio in egg yolks was found to be greater than 0.45 in all trial periods and housing systems. When assessing the differences between egg PUFA and SFA ratios from different housing systems, the results were unevenly distributed, with barn-laid housing systems increasing the ratio at the start of the trial and enriched cage systems increasing it at the end. The increased PUFA/SFA ratio in barn-laid eggs at the start of the trial may have been due to higher concentrations of polyunsaturated arachidonic (C20:4 n6) and eicosapentaenoic (C20:5 n3) acids. Significant differences between housing methods were only noticed in the middle of the trial, when the n6/n3 ratio increased to 6.98 in eggs from enriched cage systems and 7.50 in eggs from the barn. Therefore, regardless of the housing system, the obtained n6/n3 ratio is relatively low compared to the results reported by other researchers. For example, in the study by Dalle Zotte et al. (Citation2021), the indicated ratio for cage system eggs produced in Italy was as high as 19.2 and 17.6 for barn eggs.
In general, due to the fatty acid profile, according to Sokołowicz et al. (Citation2018a), the housing system can impact the percentage of MUFA and PUFA, as well as n6/n3 ratio in egg yolks. No impact of the housing system on egg yolk cholesterol content was found by the previously mentioned author, which is consistent with recent findings. Overall, eggs have received unfavourable attention due to their cholesterol levels for many years. Because the human body synthesises cholesterol found in egg yolks, consumers have been advised to limit their dietary cholesterol intake to prevent chronic diseases like coronary heart disease (Tomaszewska et al., Citation2021). However, it was discovered that exogenous cholesterol merely contains a trace quantity of hematic cholesterol (Brugiapaglia et al., Citation2014). Meanwhile, research on egg consumption shows that consuming eggs daily has no impact on cholesterol levels in humans and can boost post-meal metabolic reactions (Fuller et al., Citation2015; Katz et al., Citation2014; Njike et al., Citation2016). As evidenced by the results obtained during the research, cholesterol and the remaining lipids were found to be relatively constant, and the cholesterol levels in the egg yolks remained nearly comparable throughout all trial periods, despite the hen housing system. In contrast, Pistekova et al. (Citation2006) found that the cholesterol levels of egg yolks from hens kept on litter (similar to a barn) were found to be higher than in caged housing. In any case, the diet has a significant effect on changes in lipid profiles, and in the experiment, the diet in both hen housing systems was identical.
4.4. Sensory profile
Alternative egg production systems are often perceived as more desirable by consumers due to their reported benefits for animal welfare and egg quality. However, when it comes to the purchase of eggs, sensory characteristics are the primary consideration. Therefore, it is crucial for egg producers to inform consumers about the various production housing systems and their impact on sensory attributes (Rondoni et al., Citation2020). Since sensory properties are one of the primary determinants of egg purchase, the sensory profile of eggs raised in various housing systems has received limited attention yet (Hammershøj & Finn Johansen, Citation2016). For example, the method of evaluating the stability of the sensory profile of eggs marketed at multiple timings has never been validated (Dalle Zotte et al., Citation2021). Consumers’ purchasing habits for eggs have undoubtedly altered in the last decade as they have increasingly required more environmentally and animal-friendly production systems, selecting an expanding number of eggs from cage-free and other alternative housing systems (Berkhoff et al., Citation2020). It was indicated by the study that the flavour or odour intensity of eggs is not affected by the housing environment of laying hens, and fresh egg samples do not exhibit any distinguishable odour or taste. Despite the option for customers to select barn-laid eggs based on animal welfare, the findings suggest that this choice should not be made with the expectation of improved sensory characteristics.
5. Conclusions
Depending on the lighting conditions in different housing systems, the decreased feed intake of Lohmann Brown Classic laying hens kept in an enriched cage system could be caused by increased light intensity. This factor might have an impact on the quality of final egg production because enriched cage eggs are lighter compared to barn-laid eggs. In general, the enriched cage housing system demonstrated consistently higher rates of both liveability and egg production when compared to the barn-laid system.
Since no clear trend was discovered in recent research, the laying hen housing methods had no discernible impact on the qualitative, chemical, and sensory qualities of the eggs produced on the same poultry farm but in different housing systems over all trial periods, except for the barn-laid egg yolks’ greater contents of SFA at the beginning and PUFA at the end of the trial.
Given that the laying hens were fed the same compound feed throughout all of the trial periods, the slight variations in the eggs from different housing systems are negligible. However, acknowledging the housing system may only encourage consumers to choose a more hen-friendly rearing method according to animal welfare standards.
Disclosure statement
No potential conflict of interest was reported by the authors
Additional information
Funding
Notes on contributors
Asta Racevičiūtė-Stupelienė
Asta Racevičiūtė-Stupelienė is a professor of animal science at the Institute of Animal Rearing Technologies, Veterinary Academy, Lithuanian University of Health Sciences, located in Kaunas, Lithuania. Her research area focuses on poultry and pigs, their housing systems, animal welfare, and feed alternatives.
Vilma Vilienė
Vilma Vilienė is a professor of animal science at the Institute of Animal Rearing Technologies, Veterinary Academy, Lithuanian University of Health Sciences, located in Kaunas, Lithuania. Her research area is mainly focused on rabbits; however, a wide range of research is carried out with poultry, with attention paid to natural alternatives to feed raw materials and chemical additives.
Saulius Bliznikas
Saulius Bliznikas is a senior researcher at the Institute of Animal Sciences, Lithuanian University of Health Sciences, located in Baisogala, Lithuania. His work in the Laboratory of Chemistry is supported by analytical methods, chromatography, and other innovative methods focused on research objects of both plant and animal origin.
Vilma Šašytė
Vilma Šašytė is a senior technician at Dr. L. Kriaučeliūnas Small Animal Clinic, Veterinary Academy, Lithuanian University of Health Sciences, located in Kaunas, Lithuania. Her field of work includes laboratory diagnostics, installation, assimilation, and application of new laboratory equipment and methodologies.
Monika Nutautaitė
Monika Nutautaitė is a PhD student of animal science and assistant at the Institute of Animal Rearing Technologies, Veterinary Academy, Lithuanian University of Health Sciences, located in Kaunas, Lithuania. Her research focuses mainly on the search for alternative feed materials using natural resources. Most of the research involves rabbits and poultry.
References
- Abeyrathne, E. D. N. S., & Ahn, D. U. (2015). Isolation of value-added components from egg white and their potential uses in food, nutraceutical and pharmaceutical industries. In R. R. Watson, & F. De Meester (Eds.), Handbook of Eggs in Human Function (pp. 35–21). Human Health Handbooks.
- Aerni, V., Brinkhof, M. W. G., Wechsler, B., Oester, H., & Fröhlich, E. (2005). Productivity and mortality of laying hens in aviaries: A systematic review (Vol. 61). Cambridge University Press on behalf of World’s Poultry Science Association.
- Araujo Netto, D., Lima, H. J. D., Alves, J. R., de Morais, B. C., Rosa, M. S., & Bittencourt, T. M. (2017). Production of Laying Hens in Different Rearing Systems Under Hot Weather. Acta Scientiarum Animal Sciences, 40(1). 37677–e37677. https://doi.org/10.4025/actascianimsci.v40i0.37677 https://search.proquest.com/docview/2068407945
- Bain, M. M., MacLeod, N., Thomson, R., & Hancock, J. W. (2006). Microcracks in Eggs. Poultry Science, 85(11), 2001–2008. a. https://doi.org/10.1093/ps/85.11.2001
- Batkowska, J., & Brodacki, A. (2017). Selected Quality Traits of Eggs and the Productivity of Newly Created Laying Hen Hybrids Dedicated to an Extensive Rearing System. Archiv Für Tierzucht, 60(2), 87–93. https://doi.org/10.5194/aab-60-87-2017
- Berkhoff, J., Alvarado-Gilis, C., Pablo Keim, J., Antonio Alcalde, J., Vargas-Bello-Pérez, E., & Gandarillas, M. (2020). Consumer Preferences and Sensory Characteristics of Eggs from Family Farms. Poultry Science, 99(11), 6239–6246. https://dx.doi.org/10.1016/j.psj.2020.06.064. https://doi.org/10.1016/j.psj.2020.06.064.
- Brugiapaglia, A., Lussiana, C., & Destefanis, G. (2014). Fatty Acid Profile and Cholesterol Content of Beef at Retail of Piemontese, Limousin and Friesian Breeds. Meat Science, 96(1), 568–573. https://dx.doi.org/10.1016/j.meatsci.2013.08.012. https://doi.org/10.1016/j.meatsci.2013.08.012
- Campbell, D. L. M., de Haas, E. N., & Lee, C. (2019). A Review of Environmental Enrichment for Laying Hens during Rearing in Relation to their Behavioral and Physiological Development. Poultry Science, 98(1), 9–28. doi:10.3382/ps/pey319 https://www.narcis.nl/publication/RecordID/oai:library.wur.nl:wurpubs%2F546212
- Champati, A., Samal, L., Charana Behura, N., Kumar Pati, P., Muduli, S., & Kashinathrao Popalghat, H. (2020). Carcass Traits and Meat Composition of Hansli × CSML Bird Under Intensive and Semi-Intensive Rearing Systems. Journal of Animal Research, 10(6), 1051–1055. Dec, 01. https://search.proquest.com/docview/2513323093. https://doi.org/10.30954/2277-940X.06.2020.26
- Chand, N., Naz, S., Irfan, M., Ullah Khan, R., & Ur Rehman, Z. (2018). Effect of Sea Buckthorn (Hippophae Rhamnoides L.) Seed Supplementation on Egg Quality and Cholesterol of Rhode Island Red×Fayoumi Laying Hens. Korean Journal for Food Science of Animal Resources, 38(3), 468–475. https://www.ncbi.nlm.nih.gov/pubmed/30018491. https://doi.org/10.5851/kosfa.2018.38.3.468.
- Christopherson, S. W., & Glass, R. L. (1969). Preparation of Milk Fat Methylesters by Alcoholysis in an Essentially Nonalcoholic Solution. Journal of Dairy Science, 52(8), 1289–1290. https://doi.org/10.3168/jds.S0022-0302(69)86739-1
- Dale, N. (1994). National Research Council Nutrient Requirements of Poultry - Ninth Revised Edition (1994). The Journal of Applied Poultry Research, 3(1), 101. https://doi.org/10.1093/japr/3.1.101
- Dalle Zotte, A., Cullere, M., Pellattiero, E., Sartori, A., Marangon, A., & Bondesan, V. (2021). Is the Farming Method (Cage, Barn, Organic) a Relevant Factor for Marketed Egg Quality Traits? Livestock Science, 246, 104453. https://dx.doi.org/10.1016/j.livsci.2021.104453. https://doi.org/10.1016/j.livsci.2021.104453
- Dalle Zotte, A., Sartori, A., & Bordesan, V. Physical Egg Quality from Organic Versus Conventional Laying Hens. 15–19 September. 2013, 2013.
- El-Sabrout, K. (2018). Effect of Rearing System and Season on Behaviour, Productive Performance and Carcass Quality of Rabbit: A Review. Journal of Animal Behaviour and Biometeorology, 6(4), 102–108. https://doi.org/10.31893/2318-1265jabb.v6n4p102-108
- Englmaierová, M., Tůmová, E., Charvátová, V., & Skřivan, M. (2014). Effects of Laying Hens Housing System on Laying Performance, Egg Quality Characteristics, and Egg Microbial Contamination. Czech Journal of Animal Science, 59(8), 345–352. https://doi.org/10.17221/7585-CJAS
- Ferrante, V., Lolli, S., Vezzoli, G., & Guidobono Cavalchini, L. (2009). Effects of Two Different Rearing Systems (Organic and Barn) on Production Performance, Animal Welfare Traits and Egg Quality Characteristics in Laying Hens. Italian Journal of Animal Science, 8(2), 165–174. https://www.tandfonline.com/doi/abs/10.4081/ijas.2009.165. https://doi.org/10.4081/ijas.2009.165.
- Folch, J., Lees, M., & Stanley, G. H. S. (1957). A Simple Method for the Isolation and Purification of Total Lipides from Animal Tissues. The Journal of Biological Chemistry, 226(1), 497–509. https://doi.org/10.1016/S0021-9258(18)64849-5
- Fuller, N. R., Caterson, I. D., Sainsbury, A., Denyer, G., Fong, M., Gerofi, J., Baqleh, K., Williams, K. H., Lau, N. S., & Markovic, T. P. (2015). The Effect of a High-Egg Diet on Cardiovascular Risk Factors in People with Type 2 Diabetes: The Diabetes and Egg (DIABEGG) Study—a 3-Mo Randomized Controlled Trial. The American Journal of Clinical Nutrition, 101(4), 705–713. https://www.ncbi.nlm.nih.gov/pubmed/25833969. https://doi.org/10.3945/ajcn.114.096925.
- Ghanima, A., Mahmoud, M., Alagawany, M., Abd El-Hack, M. E., Taha, A., Elnesr, S. S., Ajarem, J., Allam, A. A., & Mahmoud, A. M. (2020). Consequences of various Housing Systems and Dietary Supplementation of Thymol, Carvacrol, and Euganol on Performance, Egg Quality, Blood Chemistry, and Antioxidant Parameters. Poultry Science, 99(9), 4384–4397. https://doi.org/10.1016/j.psj.2020.05.028
- Ghanima, A., Mahmoud, M., Elsadek, M. F., Taha, A. E., Abd El-Hack, M. E., Alagawany, M., Ahmed, B. M., Elshafie, M. M., & El-Sabrout, K. (2020). Effect of Housing System and Rosemary and Cinnamon Essential Oils on Layers Performance, Egg Quality, Haematological Traits, Blood Chemistry, Immunity, and Antioxidant. Animals (Basel), 10(2), 245. https://doi.org/10.3390/ani10020245
- Hamilton, R. M. G., & Bryden, W. L. (2021). Relationship between Egg Shell Breakage and Laying Hen Housing Systems – an Overview. World’s Poultry Science Journal, 77(2), 249–266. https://doi.org/10.1080/00439339.2021.1878480
- Hammershøj, M., & Finn Johansen, N. (2016). Review: The Effect of Grass and Herbs in Organic Egg Production on Egg Fatty Acid Composition, Egg Yolk Colour and Sensory Properties. Livestock Science, 194, 37–43. https://doi.org/10.1016/j.livsci.2016.11.001
- Helrich, K. (Ed.) (1990). Official Methods of Analysis of the Association of Official Analytical Chemists. (15th ed., Vol. 1). Association of Official Analytical Chemists, Inc.
- Hincke, M. T., Da Silva, M., Guyot, N., Gautron, J., McKee, M. D., Guabiraba-Brito, R., & Réhault-Godbert, S. (2019). Dynamics of Structural Barriers and Innate Immune Components during Incubation of the Avian Egg: Critical Interplay between Autonomous Embryonic Development and Maternal Anticipation. Journal of Innate Immunity, 11(2), 111–124. https://www.karger.com/doi/10.1159/000493719. https://doi.org/10.1159/000493719
- Jones, D. R. (2012). “Haugh Unit: Gold Standard of Egg Quality. National Egg Quality.
- Jones, D. R., Cox, N. A., Guard, J., Fedorka-Cray, P. J., Buhr, R. J., Gast, R. K., Abdo, Z., Rigsby, L. L., Plumblee, J. R., Karcher, D. M., Robison, C. I., Blatchford, R. A., & Makagon, M. M. (2015). Microbiological Impact of Three Commercial Laying Hen Housing Systems. Poultry Science, 94(3), 544–551. https://www.ncbi.nlm.nih.gov/pubmed/25480737. https://doi.org/10.3382/ps/peu010
- Katz, D. L., Gnanaraj, J., Treu, J. A., Ma, Y., Kavak, Y., & Njike, V. Y. (2014). Effects of Egg Ingestion on Endothelial Function in Adults with Coronary Artery Disease: A Randomized, Controlled, Crossover Trial. American Heart Journal, 169(1), 162–169. https://www.clinicalkey.es/playcontent/1-s2.0-S0002870314006048. https://doi.org/10.1016/j.ahj.2014.10.001.
- Ketta, M., Tumova, E., Englmaierova, M., & Chodova, D. (2020). Combined Effect of Genotype, Housing System, and Calcium on Performance and Eggshell Quality of Laying Hens. Animals (Basel), 10(11), 2120. https://search.proquest.com/docview/2462546030. https://doi.org/10.3390/ani10112120
- Konkol, D., Popiela, E., & Korczyński, M. (2020). The Effect of an Enriched Laying Environment on Welfare, Performance, and Egg Quality Parameters of Laying Hens Kept in a Cage System. Poultry Science, 99(8), 3771–3776. https://doi.org/10.1016/j.psj.2020.04.017.10.1016/j.psj.2020.04.017
- Lay, D. C., Jr., Fulton, R. M., Hester, P. Y., Karcher, D. M., Kjaer, J. B., Mench, J. A., Mullens, B. A., Newberry, R. C., Nicol, C. J., O’Sullivan, N. P., & Porter, R. E. (2011). Hen Welfare in Different Housing Systems. Poultry Science, 90(1), 278–294. https://www.ncbi.nlm.nih.gov/pubmed/21177469. https://doi.org/10.3382/ps.2010-00962
- Lewko, L., & Gornowicz, E. (2011). Effect of Housing System on Egg Quality in Laying Hens. Annals of Animal Science, 11(4), 607–611. https://search.proquest.com/docview/1321132175. https://doi.org/10.2478/v10220-011-0012-0
- Lieboldt, M.A., Halle, I., Frahm, J., Schrader, L., Weigend, S., Preisinger, R., & Dänicke, S. (2015). Effects of Long-Term Graded L-Arginine Supply on Growth Development, Egg Laying and Egg Quality in Four Genetically Diverse Purebred Layer Lines. The Journal of Poultry Science, 53(1), 8–21. https://www.jstage.jst.go.jp/article/jpsa/53/1/53_0150067/_article/-char/en. https://doi.org/10.2141/jpsa.0150067.
- The Lohman Tierzucht guide. (2019). Management Guide of Lohman Brown-Classic Layers. Lohmann Breeders GmbH.
- Lordelo, M., Fernandes, E., Bessa, R. J. B., & Alves, S. P. (2017). Quality of Eggs from Different Laying Hen Production Systems, from Indigenous Breeds and Specialty Eggs. Poultry Science, 96(5), 1485–1491. https://www.ncbi.nlm.nih.gov/pubmed/27811323. https://doi.org/10.3382/ps/pew409
- Njike, V. Y., Ayettey, R. G., Rajebi, H., Treu, J. A., & Katz, D. L. (2016). Egg Ingestion in Adults with Type 2 Diabetes: Effects on Glycemic Control, Anthropometry, and Diet Quality—a Randomized, Controlled, Crossover Trial. BMJ Open Diabetes Research & Care, 4(1), e000281. https://doi.org/10.1136/bmjdrc-2016-000281
- Parpinello, G. P., Meluzzi, A., Sirri, F., Tallarico, N., & Versari, A. (2006). Sensory Evaluation of Egg Products and Eggs Laid from Hens Fed Diets with Different Fatty Acid Composition and Supplemented with Antioxidants. Food Research International, 39(1), 47–52. https://doi.org/10.1016/j.foodres.2005.05.010
- Philippe, F. X., Mahmoudi, Y., Cinq-Mars, D., Lefrançois, M., Moula, N., Palacios, J., Pelletier, F., & Godbout, S. (2020). Comparison of Egg Production, Quality and Composition in Three Production Systems for Laying Hens. Livestock Science, 232, 103917. https://doi.org/10.1016/j.livsci.2020.103917
- Pistekova, V., Hovorka, M., Vecerek, V., Strakova, E., & Suchy, P. (2006). The Quality Comparison of Eggs Laid by Laying Hens Kept in Battery Cages and in a Deep Litter System. Czech Journal of Animal Science, 51(7), 318–325. Jul, https://agris.fao.org/agris-search/search.do?recordID=CZ2006000691 https://doi.org/10.17221/3945-CJAS
- Popova, T., Petkov, E., Ayasan, T., & Ignatova, M. (2020). Quality of Eggs from Layers Reared Under Alternative and Conventional System. Brazilian Journal of Poultry Science, 22(1). https://doi.org/10.1590/1806-9061-2019-1172
- Preisinger, R. (2018). Innovative Layer Genetics to Handle Global Challenges in Egg Production. British Poultry Science, 59(1), 1–6. https://doi.org/10.1080/00071668.2018.1401828
- Quan, T. H., & Benjakul, S. (2019). Duck Egg Albumen: Physicochemical and Functional Properties as Affected by Storage and Processing. Journal of Food Science and Technology, 56(3), 1104–1115. https://doi.org/10.1007/s13197-019-03669-x.
- Rakonjac, S., Bogosavljevic-Boskovic, S., Skrbic, Z., Lukic, M., Doskovic, V., Petricevic, V., & Petrovic, M. (2018). Quality and Chemical Composition of Eggs Affected by Rearing System and Hen’s Age. Biotechnology in Animal Husbandry, 34(3), 335–344. https://doi.org/10.2298/BAH1803335R
- Rondoni, A., Asioli, D., & Millan, E. (2020). Consumer Behaviour, Perceptions, and Preferences Towards Eggs: A Review of the Literature and Discussion of Industry Implications. Trends in Food Science & Technology, 106, 391–401. https://doi.org/10.1016/j.tifs.2020.10.038
- Samiullah, S., Saleh Omar, A., Roberts, J., & Chousalkar, K. (2017). Effect of Production System and Flock Age on Eggshell and Egg Internal Quality Measurements. Poultry Science, 96(1), 246–258. https://doi.org/10.3382/ps/pew289
- Sekeroglu, A., Sarica, M., Demir, E., Ulutas, Z., Tilki, M., Saatci, M., & Omed, H. (2010). Effects of Different Housing Systems on some Performance Traits and Egg Qualities of Laying Hens. Journal of Animal and Veterinary Advances: JAVA, 9(12), 1739–1744. https://doi.org/10.3923/javaa.2010.1739.1744
- Simopoulos, A. P. (2002). The Importance of the Ratio of Omega-6/Omega-3 Essential Fatty Acids. Biomedicine & Pharmacotherapy, 56(8), 365–379. https://dx.doi.org/10.1016/S0753-3322(02)00253-6. https://doi.org/10.1016/S0753-3322(02)00253-6
- Singh, R., Cheng, K. M., & Silversides, F. G. (2009). Production Performance and Egg Quality of Four Strains of Laying Hens Kept in Conventional Cages and Floor Pens. Poultry Science, 88(2), 256–264. https://doi.org/10.3382/ps.2008-00237
- Sokołowicz, Z., Krawczyk, J., & Dykiel, M. (2018a). Effect of Alternative Housing System and Hen Genotype on Egg Quality Characteristics. Emirates Journal of Food and Agriculture, 30(8), 695–703. https://search.proquest.com/docview/2126485826. https://doi.org/10.9755/ejfa.2018.v30.i8.1753
- Sokołowicz, Z., Krawczyk, J., & Dykiel, M. (2018b). The Effect of the Type of Alternative Housing System, Genotype and Age of Laying Hens on Egg Quality. Annals of Animal Science, 18(2), 541–556. http://www.degruyter.com/doi/10.2478/aoas-2018-0004. https://doi.org/10.2478/aoas-2018-0004
- Tomaszewska, E., Muszyński, S., Arczewska-Włosek, A., Domaradzki, P., Pyz-Łukasik, R., Donaldson, J., & Świątkiewicz, S. (2021). Cholesterol Content, Fatty Acid Profile and Health Lipid Indices in the Egg Yolk of Eggs from Hens at the End of the Laying Cycle, Following Alpha-Ketoglutarate Supplementation. Foods, 10(3), 596. https://www.ncbi.nlm.nih.gov/pubmed/33799887. https://doi.org/10.3390/foods10030596
- Weeks, C. A., Lambton, S. L., Williams, A. G., & Pritchett-Corning, K. R. (2016). Implications for Welfare, Productivity and Sustainability of the Variation in Reported Levels of Mortality for Laying Hen Flocks Kept in Different Housing Systems: A Meta-Analysis of Ten Studies. PloS One, 11(1), e0146394. https://www.ncbi.nlm.nih.gov/pubmed/26734933. https://doi.org/10.1371/journal.pone.0146394
- Wei, S., Guo, Y., & Yan, P. (2019). Comparison of Two Housing Systems on Behaviour and Performance of Fattening Pigs. Journal of Applied Animal Research, 47(1), 41–45. https://www.tandfonline.com/doi/abs/10.1080/09712119.2018.1561372. https://doi.org/10.1080/09712119.2018.1561372
- Wilson, P. B. (2017). Recent Advances in Avian Egg Science: A Review. Poultry Science, 96(10), 3747–3754. https://www.ncbi.nlm.nih.gov/pubmed/28938769. https://doi.org/10.3382/ps/pex187.
- Xiao, N., Zhao, Y., Yao, Y., Wu, N., Mingsheng, X., Huaying, D., & Yonggang, T. (2020). Biological Activities of Egg Yolk Lipids: A Review. Journal of Agricultural and Food Chemistry, 68(7), 1948–1957. http://dx.doi.org/10.1021/acs.jafc.9b06616. https://doi.org/10.1021/acs.jafc.9b06616.
- Yenice, G., Kaynar, O., Ileriturk, M., Hira, F., & Hayirli, A. (2017). Quality of Eggs in Different Production Systems. Czech Journal of Food Sciences, 34(No. 4), 370–376. https://search.proquest.com/docview/2507342508. https://doi.org/10.17221/33/2016-CJFS.
- Zhang, R., Long, L., Jian‐cai, L., Liu, S., Chen, R., Shi, B., Gao, W., Chen, G., Zheng, Y., & Rao, P. (2004). An Improved Method of Cholesterol Determination in Egg Yolk by HPLC. In J. Whitaker, N. Haard, C. Shoemaker, & P. Singh (Eds.), Food for Health in the Pacific Rim (pp. 198–205). Food & Nutrition Press, Inc. 9584.