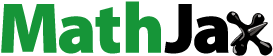
Abstract
Prolonged drought of cowpea at the seedling stage can affect survival and productivity. This study sought to evaluate cowpea genotypes for their resistance to seedling-stage drought stress. Fifteen cowpea genotypes were used in this study. The experiment was a factorial experiment arranged in a Completely Randomized Design with three replications. Drought stress was imposed for 4 weeks after the full expansion of the first trifoliate leaf. Soil Plant Analysis Development (SPAD) chlorophyll meter readings of unifoliate, first, and second trifoliate leaves of each genotype were measured on a weekly basis due to the predictive ability of chlorophyll content on drought tolerance. The data were subjected to ANOVA, and the means were separated at a 5% probability level. Genotypes were ranked according to their tolerance level based on their chlorophyll inflectance index (CII). The results revealed significant genotype by stress interaction effects. Seedling stage screening under water-stressed conditions in combination with measurements of leaf chlorophyll measurements proved to be an effective way for rapid screening of genotypes for drought tolerance. IT14K-1424-12, IT10K-837-1 and IT10K-303-1 had consistently high chlorophyll content under drought stress for unifoliate and trifoliate leaves. These genotypes also fell into one cluster. Based on CII with a threshold of 51% for the fourth week of drought stress of unifoliate and trifoliate leaves, three genotypes (IT14K-1424-12, IT10K-837-1 and IT10K-303-1) were found to have Type I drought-tolerant mechanism. These genotypes could be used in future breeding interventions or released as varieties.
1. Introduction
Cowpea (Vigna unguiculata L. Walp.) is a grain legume crop of global economic importance and it originated from Africa (Carvalho et al., Citation2019). It is grown for its grains and fodder due to its diverse morphology (Gonçalves et al., Citation2016; Ravelombola et al., Citation2020). According to Santos et al. (Citation2020), dry cowpea grain has high protein levels (20.0–39.4%) and low-fat content (3.1–30.4%). Aside from being an excellent source of protein, cowpea contains all the essential amino acids, making it an important food component in several countries including Ghana (Freire Filho et al., Citation2012; Oliveira et al., Citation2016; Vasconcelos et al., Citation2010). Apart from being a good source of nutrients, cowpea contains dietary fibres, antioxidants, polyunsaturated fatty acids (PUFA) and polyphenols (da Silva et al., Citation2018; Nkomo et al., Citation2021). Cowpea also plays an important role in soil fertility improvement, it has the capability of fixing atmospheric nitrogen and suppressing weeds as a cover crop (Kebede & Bekeko, Citation2020).
The global production of cowpea is about 8.9 million metric tons cultivated in an area of around 15 million hectares (FAOSTAT, Citation2020). Cowpea cultivation is widely distributed throughout the tropics and subtropical regions, with the largest production in Africa coming from West Africa (Gnankambary et al., Citation2020).
Cowpea cultivation is challenged by various factors including abiotic stresses such as drought. Cowpea yield remains one of the lowest among all grain legumes in sub-Saharan Africa, due to several constraints, such as drought. According to Ibitoye (Citation2015), drought causes grain and fodder yield reduction by 62% and 56%, respectively. It can occur at any stage of crop development. Early-season drought stress can affect crop establishment. Drought stress during flowering or pod-filling stages may also result in yield losses (Tumwesigye & Musiitwa, Citation2002). It is reported that climate change will intensify the frequency of drought and flood, particularly in several African countries, and it is projected that by 2050, water shortages are expected to affect 67% of the world’s population (Ceccarelli et al., Citation2004).
In Ghana, cowpea is cultivated across all ecological zones. However, most of the cowpea production occurs in the Savanna ecological zone of Ghana where drought is predominant due to erratic rainfall and heat stress accompanied by poor soil fertility (Fahad et al., Citation2017; Yahaya et al., Citation2019). Most farmers rely on rainfall for crop production. The rainfall pattern in northern Ghana is monomodal and spans from June to mid-October. As cowpea is a short-season crop, it is usually planted from mid-July to mid-August so that the harvesting period will coincide with the onset of the dry season. Due to climate change, consistently over the years, drought has been observed to affect plant stands of cowpea (Omomowo & Babalola, Citation2021). This drought takes place for a minimum of 2 weeks in August in most parts of northern Ghana. Efforts have therefore been made to screen cowpea genotypes for resistance to seedling-stage drought (Ajayi et al., Citation2018; Carvalho et al., Citation2019; Ravelombola et al., Citation2020). Only a few studies have exploited the measurement of physiological traits, such as chlorophyll content in assessing drought tolerance of cowpea in Ghana (Alidu et al., Citation2019). According to previous findings, Chlorophyll is responsible for converting light energy into chemical energy and the photosynthetic rate is directly affected by the chlorophyll content (Arjenaki et al., Citation2012). Determining drought tolerance by measuring the chlorophyll content is important because it gives an indication of the health of the plant (Monteoliva et al., Citation2021). Arunyanark et al. (Citation2008) established a relationship among SPAD chlorophyll meter reading (SCMR), chlorophyll density and transpiration efficiency, and demonstrated that the Soil Plant Analysis Development (SPAD) chlorophyll meter could be used for rapid and cost-effective assessment of chlorophyll as proposed by Percival and Sheriffs (Citation2002) in its use to rapidly and accurately detect and quantify drought tolerance.
Photosynthesis plays an important role in plant growth; however, this can be reduced in plants under stressed conditions due to the potential damage to the chloroplast (Munné-Bosch et al., Citation2001). Given the complexity of drought tolerance in cowpeas as a result of the various traits for drought tolerance evaluation (Verbree et al., Citation2015), it will be useful to exploit a rapid and accurate drought screening method such as seedling stage drought screening combined with measuring chlorophyll content of stressed plants. Mai-Kodomi et al. (Citation1999) described two types of drought tolerance (Type I and Type II) of cowpea at the seedling growth stage. In Type I drought tolerance, both unifoliate and trifoliate leaves are fully green under drought conditions, whereas in Type II, genotypes have delayed senescence of their trifoliate leaves. However, there is no information on the drought tolerance mechanism of genotypes used in this study. The purpose of this study was to evaluate the drought-tolerance ability of advanced cowpea breeding lines, specifically, to determine the chlorophyll content of cowpea seedlings under moisture stressed conditions, explore the drought tolerance mechanisms in the cowpea genotypes and identify cowpea genotypes that have high photosynthetic abilities under drought stress.
2. Materials and methods
2.1. Experiment 1
An initial study was carried out between April and May 2021 at the screen house of the Council for Scientific and Industrial Research-Savanna Agricultural Research Institute (CSIR-SARI), Nyankpala, Ghana. Using all the test genotypes (15) and two stress factors (water stressed and non-stressed), the experiment was a factorial experiment laid out in a completely randomized design (CRD) with three replications. The primary data collected were on the trifoliate leaves (TF) across five sampling occasions. The outcome and challenges of experiment 1 provided a basis for the experimental procedure and data collected in experiment 2.
2.2. Experiment 2
2.2.1. Location of study
The experiment was conducted at the screen house of the Council for Scientific and Industrial Research-Savanna Agricultural Research Institute (CSIR-SARI), Nyankpala, Ghana, between April and May of 2021 and 2022. List of genotypes used in this study have been listed in Table . Environmental descriptions of the experimental site are presented in Table .
Table 1. List of selected cowpea genotypes used in this study obtained from CSIR-SARI
Table 2. Environmental description of experimental site during the experimental period (April to May)
2.2.2. Source of experimental materials
A total of 15 cowpea genotypes were used in this study (). These materials have been identified to have high and stable yields from previous multilocational yield trials. Eight of these lines were obtained from the International Institute for Tropical Agriculture (IITA) while the remaining seven were obtained from CSIR-SARI.
2.2.3. Experimental design
The experiment was a factorial experiment laid out in a Completely Randomized Design (CRD). The two factors were the genotypes (15) and stress factor (water stressed and non-water stressed conditions). The treatment was a combination of the levels of the two factors.
2.2.4. Screenhouse evaluation for drought tolerance
The procedure for evaluating drought tolerance was described previously by Ravelombola et al. (Citation2018). Plastic containers were filled up with sandy-loamy soil. Each plastic container was irrigated 2 days before sowing to obtain the required field capacity moisture level. A total of six holes were created in each container, and two seeds were sown per hole. The plants were later thinned to one plant/stand or per hole at emergence. Uniform and healthy plants were kept for evaluation.
A week after emergence, a blanket NPK 15-15-15 fertilizer was applied at a rate of 100 kg/ha, and pots were watered with 150 mL of tap water at 3-day intervals until the first trifoliate leaves expanded. Environmental impact was not minimized, but plants were placed to ensure uniformity of environmental impacts on the plants. At this time, irrigation was withdrawn for stress to be imposed, whereas watering proceeded for the control treatment. In an attempt to minimize environmental impact within the screen house, each drought stress container was placed next to a well-watered container.
2.2.5. Data collection
Data were taken on chlorophyll content of unifoliate, first trifoliate and second trifoliate leaves of water-stressed and non-water-stressed plants using SPAD-502 Plus (Spectrum Technologies, Inc., Plainfield, IL). Measurements were taken every week following the onset of drought. Measurements were made three times for each leaflet of the unifoliate or trifoliate plant and the mean kept for the final chlorophyll value.
The Chlorophyll Inflect Index (Gonzalez, Citation1996) parameters were approximated using the following formulas previously described: Chlorophyll absolute decrease (CD) = CC − CS; Chlorophyll Inflect Index (CII) = 100%* CD/(CC), where CC means the chlorophyll content for control and CS means the chlorophyll content for drought stress.
During the fourth week of drought stress, data was taken on plant greenness score using a previously described scale of 1–5 (1 = completely green plants, 2 = plants beginning to lose greenness, 3 = signs of chlorosis and necrosis become visible, 4 = severe chlorosis and necrosis and 5 = plants were completely dead (Ravelombola et al., Citation2018)). Data on plant greenness and drought stress were taken for each plant in a pot, and the average values were recorded.
The score value was changed into a percentage to reduce the error in the statistical analysis. The “Greenness”’ value was determined as 100% (5-Overall plant greenness score-1)/4. The Greenness Inflect Index (GII) parameter was employed using the following formulas to track changes in Greenness over weeks: Greenness absolute decrease (GD) = GC − GS; Greenness inflect index (GII) = 100%* GD/(GC), where GC stands for control and GS for drought stress.
The overall plant healthiness was determined in the fifth week of drought stress using a 1-to-5-point scale (1 = fully green, 2 = new trifoliate totally green with chlorotic unifoliate and trifoliate, 3 = chlorotic new trifoliate, 4 = severe necrosis on all leaves with a green developing tip, and 5 = dead plants). The score value was changed into a percentage to reduce the error in the statistical analysis. The “Healthiness”’ value was determined as 100% (5-Overall plant healthiness score-1)/4. The Healthiness Inflect Index (HII) parameter was employed using the following formulas to track changes in healthiness over weeks: Healthiness absolute decrease (HD) = HC − HS; healthiness inflect index (HII) = 100%* HD/(HC), where HC stands for control and HS for drought stress.
After data acquisition, plants were re-watered for 1 to 2 weeks and percentage of plants that recovered after the period of imposed drought was recorded as the recovery rate.
2.2.6. Data analysis
Percentage data were square root transformed before analysis.
The data collected were subjected to a one-way ANOVA using the Statistical Tool for Agricultural Science, version 2.0.1 (STAR, Citation2014). Means were separated using the Tukey at 5% probability level. Principal component analysis (PCA) and Cluster analysis was done using R version 4.0.3 (RStudio Team, 2021) to group and identify most tolerant genotypes
Pearson’s correlations test was also done to determine relations between the drought tolerance indices.
2.2.7. Canonical discriminant analysis and drought tolerance index
Drought tolerance indicators were standardized before final evaluation in order to fully assess drought tolerance. In this study, we used two methods to assess the drought tolerance of each genotype: 1) Principal component analysis (PCA) and Cluster analysis was done for the 15 genotypes based on context parameters; and 2) all breeding lines were scored by their orders in five drought-tolerance parameters one by one, according to the tolerance from small to large (the most tolerance line shall be scored as 1 and most susceptible as 15). We then added five scores for each line, respectively, to comprehensively evaluate drought tolerance.
The broad-sense heritability (H) of the measured traits was estimated using the formula:
where is the total genetic variance,
is the residual variance, and
is the number of replications. The estimates for
and
were
and Var (Residual), respectively. EMS (G) and Var (Residual) were obtained from the ANOVA table.
3. Results
3.1. Experiment 1
Water stressed and non-water stressed plants of the different genotypes differed significantly in their chlorophyll content for trifoliate leaves for all the different weeks (p < 0.001) (Table ). Except for week 1, genotypic effect on chlorophyll content was not significant (p < 0.001).
Table 3. ANOVA table for chlorophyll content in trifoliate leaves for week 1 to week 5 in experiment 1
3.2. Experiment 2
Except for the second trifoliate (non-stressed) chlorophyll content which had a medium heritability score of 60.44%, all the other traits had a high broad-sense heritability score that ranged from 73.23% to 95.56%. The highest broad-sense heritability score was obtained from water-stressed plants for the second trifoliate chlorophyll content trait (Table ).
Table 4. Broad-sense heritability of chlorophyll content, plant greenness and plant healthiness
There was a significant genotype by stress effect in unifoliate chlorophyll content during the second, third and fourth weeks of drought stress (Table ). IT14K-1424-12 had the highest chlorophyll content of 36.93, which was not significantly different from IT10K-837-1, IT10K-303-1, IT10K-817-3, IT86D-610, Kirkhouse Benga and Wangkae during the third week of drought stress in unifoliate leaves. In week 4, however, the highest chlorophyll content was observed in IT14K-1424-12 (26.1), which was not significantly different from IT10K-837-1 and IT10K-303-1 (Table ).
Table 5. ANOVA table of unifoliate leaf chlorophyll contents from week 1 to week 4
Table 6. Chlorophyll content of unifoliate leaves under water-stressed and non-water stressed condition
Note: Lowercase letters represent mean separation. Values followed by the same letters in each column imply they are not significantly different.
Significant differences (p < 0.05) were observed in the first trifoliate and second trifoliate leaf chlorophyll contents of stressed and non-stressed cowpea genotypes (Tables ). Genotypes were, however, more discriminated during the fourth week of drought stress. For the first trifoliates, during the fourth week of drought stress, IT10K-837-1 had the highest chlorophyll content of 30.43 which was not significantly different from IT14K-1424-12, IT10K-303-1, IT86D-610, SARI-3-11-100, IT10K-817-3 and IT13K-1070-2. For the second trifoliates, IT14K-1424-12 had a significant (p < 0.05) high chlorophyll content of 39.6 which is not significantly different from IT10K-303-1, IT10K-837-1, IT13K-1070-2, IT11K-61-82, IT86D-610 and Wangkae during the fourth week of drought stress (Table ).
Table 7. ANOVA table for first trifoliate leaf chlorophyll contents from week 1 to week 4
Table 8. ANOVA table for second trifoliate leaf chlorophyll contents from week 1 to week 4
Table 9. Chlorophyll content of cowpea trifoliate leaves of water-stressed and non-water-stressed condition
Chlorophyll inflect index (CII) measures the chlorophyll content change of stressed plants compared to the same genotype of non-stressed plants. A higher value indicates a higher chlorophyll content loss.
Padi-tuya, SARI-2-50-80 and SARVX-09-004 had CII higher than 51% for unifoliate leaves during the third and fourth weeks of drought stress and during the fourth week of stress in the first and second trifoliate leaves. This means these genotypes lost their chlorophyll content more quickly under drought stress and are therefore susceptible to seedling stage drought stress (Table ).
Table 10. Chlorophyll inflect index (CII) of unifoliate, first trifoliate and second trifoliate at third and fourth weeks of drought stress
IT14K-1424-12, IT10K-837-1 and IT10K-303-1 had consistently low CII of unifoliate leaves during the third and fourth weeks (less than 30% and 60% respectively) of drought stress and during the fourth week of stress in the first and second trifoliate leaves. Only these two genotypes (IT14K-1424-12 and IT10K-837-1) had CII less than 51% during the fourth week of drought stress in unifoliate leaves while the remaining genotypes had a chlorophyll inflect index higher than 51%. Based on this observation, these two genotypes can be described to have Type I drought tolerance. IT10K-817-3, IT11K-61-82, IT10K-1070-2, IT86D-610, Wangkae and Kirkhouse Benga had CII of trifoliate less than 51% but have CII_W4U be greater than 51%. These genotypes can be considered to have Type II drought tolerance mechanism (Table ).
Table 11. Tolerance rating based on chlorophyll inflect index of unifoliate and trifoliate leaves
A dendrogram was used to generate two major clusters of accessions under drought stress at 50% similarity index (Figure ). Cluster 1 consisted of IT10K-837-1, IT14K-1424-12 and IT10K-303-1. These genotypes have been identified to have high chlorophyll content under drought stress and have been identified to be tolerant to both Type I and II drought stress. Cluster 2 is made of two sub-clusters: 2a and 2b.
Cluster 2a could be considered to have genotypes that were moderately tolerant to seedling stage drought stress, while cluster 2b could be considered to have genotypes that were susceptible to seedling-stage drought stress. Out of the four significant sampling parameters (third and fourth weeks of drought stress for unifoliate leaves and during the fourth week of stress in the first and second trifoliate leaves), the eight genotypes under cluster 2a had chlorophyll inflectance index greater than 50% in one to three of these parameters. These genotypes could be classified as being moderately tolerant to drought stress.
Cluster 2b consisted of four genotypes: Padi-tuya, SARI-3-11-100, SARI-2-50-80 and IT07K-299-6. These genotypes were characterized by a high (>50%) percentage chlorophyll inflectance index during all the significant sampling periods (third and fourth weeks of drought stress and during the fourth week of stress for the first and second trifoliate leaves).
IT14K-1424-12, IT10K-837-1, Kirkhouse Benga and IT10K-303-1 had a greenness index score of less than 35, indicating tolerance to drought. While SARI-6-2-6, IT07K-299-6, SARVX-09-004 and SARI-2-50-80 had greenness index greater than 60%, indicating susceptibility (Table ).
Table 12. Plant greenness and plant healthiness of water-stressed and non-water-stressed plants
Chlorophyll content of unifoliate leaves (W4U_s) on the one hand is positively and strongly correlated with chlorophyll content of first trifoliate leaves at 4 weeks of drought stress (W4FT_s) (p < 0.05) and chlorophyll content of second trifoliate leaves at 4 weeks of drought stress (W4ST_s) on the other. As the chlorophyll content of unifoliate leaves at 4 weeks of drought stress (W4U_s) increases, chlorophyll content of the first trifoliate (W4FT_s) and chlorophyll content of the second trifoliate leaves at 4 weeks of drought stress (W4ST_s) also increases. Plant healthiness inflect index (HII) on the one hand was highly and strongly correlated with chlorophyll inflect index of unifoliate leaves (CII_W4U), first trifoliate leaves (CIIW4FT) and second trifoliate leaves (CII_W4ST) on the other (p < 0.05). The plant healthiness inflect index had an inverse relationship with chlorophyll content (p < 0.05) (Table ).
Table 13. Correlation of analysis of drought-related traits
This indicates that any of these traits could be used in classifying genotypes as either tolerant or susceptible as ranking of correlated traits will be consistent with each other. By using these multiple traits, a dendrogram was constructed and this helped to group genotypes according to their tolerance level.
Genotype ranking across traits was used to identify the genotypes that were drought-tolerant and drought-susceptible based on multiple traits. Genotypes were ranked for all traits (Table ) and genotypes that overlapped between highly correlated traits were chosen.
Table 14. Tolerance index of the cowpea genotypes
4. Discussion
Drought stress has been reported to cause significant yield losses of crops globally (Cairns et al., Citation2013; Ravelombola et al., Citation2020). Cowpea production can be seriously impacted by seedling-stage drought (Ravelombola et al., Citation2020; Verbree et al., Citation2015), in addition drought at the reproductive and seed filling stages is yield limiting (Nunes et al., Citation2022). The use of drought-tolerant crop varieties is the most sustainable way of mitigating the impact of drought stress. Drought is a complex trait, and evaluating several genotypes for drought tolerance can be laborious and time-consuming given the number of indicators of drought resistance (Xiong et al., 2020). Research has established that genotypes which exhibit seedling drought tolerance are more resistant to drought under field conditions than those genotypes which exhibit susceptibility to seedling-stage drought stress (Isma’ila et al., Citation2016; Ravelombola et al., Citation2018; Singh & Matsui, Citation2002; Tomar et al., Citation2016). Seedling stage screening of cowpea genotypes and measuring the chlorophyll content using SPAD chlorophyll meter will help to rapidly screen several genotypes and detect and quantify their drought tolerance.
The findings from this study indicate that there was a differential response of cowpea genotypes to drought stress. This indicates that genetic variability of the traits exists for the genotypes. Cowpea leaf chlorophyll content reduced as the duration of drought stress increased. This corroborates the findings of other studies in which chlorophyll content decreased as the time of drought stress increased (Alidu et al., Citation2019; Khan et al., Citation2015; Pirzad et al., Citation2011). The variable response of cowpea leaf types to imposed drought suggests different mechanisms for drought tolerance in the leaf types. This is probably due to the genetic differences that exist in the photosynthetic apparatus of each leaf type and leaf types that had high chlorophyll content under drought stress have more robust photosynthetic apparatus, although other physiological and morphological characteristics control productivity of crops beyond chlorophyll content.
Based on the differences in chlorophyll contents of unifoliate and trifoliate leaves of the cowpea genotypes, genotypes were classified as either having Type I or Type II drought resistance. According to Mai-Kodomi et al. (Citation1999) Type I drought-tolerant genotypes will have both unifoliate and trifoliate leaves fully green under drought stress, while Type II genotypes are able to delay senescence at the trifoliate leaf level. In this study, genotypes which had highest chlorophyll contents and lowest chlorophyll inflect index at both unifoliate and trifoliate leaves were considered to exhibit Type I drought tolerance, while genotypes that had high chlorophyll content and lowest chlorophyll inflect index only for trifoliate leaves were considered to exhibit Type II drought tolerance. IT14K-1424-12, IT10K-837-1 and IT10K-303-1 were the three genotypes showing Type I drought tolerance indicating they are good materials for cowpea improvement. These same set of genotypes were grouped together under the Unweighted Pair Group Method with Arithmetic Mean (UPGMA) and were the top ranking based on the drought tolerance rating. Five genotypes, namely, IT10K-817-3, IT11K-61-82, IT10K-1070-2, IT86D-610, Wangkae and Kirkhouse Benga, exhibited type II resistance mechanisms. These genotypes under the UPGMA clustering fell in between the two extremes and appeared to be in the same group based on their drought tolerance index. The number of genotypes identified to have Type II drought tolerance was higher than the number of genotypes exhibiting Type I drought tolerance, a similar observation was made by (Singh & Matsui, Citation2002).
Some genotypes could not be classified either as Type I or Type II. Among these were SARI-2-50-80 and Padi-tuya, which belonged to same cluster under UPGMA, SARVX-09-004 and SARI-6-2-6 also belonged to same cluster under UPGMA. These genotypes were found to lose a significant amount of both unifoliate and trifoliate leaf chlorophyll content and were also ranked among the lowest according to the tolerance index. High chlorophyll loss in these genotypes signifies that the chlorophyll-containing apparatus in their leaves may have been destroyed leading to a reduction in photosynthesis. This group of genotypes could be highly susceptible to the seedling-stage drought. Therefore, using a combination of UPGMA clustering, tolerance index and chlorophyll inflectance index of unifoliate and trifoliate leaves can help to distinguish between drought tolerant and drought-susceptible genotypes.
IT07K-299-6 and SARI-3-11-100 had a moderately low trifoliate chlorophyll content loss but high unifoliate chlorophyll content loss. These genotypes fall in the upper quartile of below average tolerance index values and under cluster 2 of the UPGMA tree. These genotypes could be deemed to be moderately tolerant to drought stress.
The chlorophyll content values obtained in this study for tolerant genotypes are a little higher than some of the values reported by Xiong et al. (2020). The unifoliate values observed were generally lower compared to values obtained for trifoliates. This same pattern was observed in a study by Xiong et al. (2020). This variation in unifoliate and trifoliate chlorophyll content could be attributed to the fact that trifoliate leaves emerged later than unifoliate leaves and therefore do not have much of their photosynthetic apparatus damaged.
Chlorophyll contents of leaf unifoliate and trifoliate leaves and their chlorophyll inflectance indices were found to have a strong association with Plant greenness/Greenness inflectance index. A plant greenness score of 5 means plants are completely dead. This indicates that reduced chlorophyll content is associated with reduced greenness of the leaf tissues. This is in concordance with studies that reported that chlorophyll fluorescence parameters are strongly correlated with whole-plant mortality under environmental stresses (Araus & Hogan, Citation1994; Baker & Rosenqvist, Citation2004; Hakam et al., Citation2000; Percival & Sheriffs, Citation2002; Valladares et al., Citation2005) and were reliable indicators of stresses (Baker & Rosenqvist, Citation2004). Genotypes that can maintain a high chlorophyll content under drought stress will be more tolerant to drought stress, and hence maintain their greenness.
Correlation between the following pairs of traits demonstrates that these traits could help in distinguishing drought-tolerant genotypes: unifoliate and trifoliate chlorophyll content, healthiness inflect index and chlorophyll inflect index, and chlorophyll inflect index and greenness index.
This study reveals that drought stress is involved in chlorophyll content loss. Drought stressed plants had necrotic wilted leaves (Figure ), indicating a reduction in photosynthetic activity, and this will later translate into low yields.
5. Conclusion
Seedling stage screening under water-stressed conditions in combination with measurement of leaf chlorophyll measurement using SPAD Chlorophyll meter reading has proven to be an effective way to rapidly screen genotypes for tolerance to drought. Through a combination of UPGMA clustering, tolerance index and chlorophyll inflectance index of unifoliate and trifoliate leaves, tolerance genotypes have been identified. IT14K-1424-12, IT10K-837-1 and IT10K-303-1 were identified to be the most drought-tolerant cowpea genotypes and exhibited Type I drought tolerance mechanism. IT10K-817-3, IT11K-61-82, IT10K-1070-2, IT86D-610, Wangkae and Kirkhouse Benga exhibited Type II resistance mechanisms. SARI-2-50-80, Padi-tuya, SARI-6-2-6, IT07K-299-6 and SARVX-09-004 were susceptible to drought. The resistant genotypes can either be used as donors of drought-tolerant genes, while susceptible ones could be improved prior to their releases.
Authors’ contributions
Theophilus Kwabla Tengey: Conceptualization; Investigation; Supervision; Methodology; Data curation; Formal analysis; Writing – original draft. Raphael Adu Gyamfi and Edward Kofi Sallah: Supervision; Writing – review & editing. Issahaku Memunatu, Mariam Seidu and Abbas Francis Senyabor: Methodology. Dominic Ngagmayan Ndela, Emmanuel Israel Affram and Charles Naapoal: Analysis; Writing – review & editing. Ophelia Asirifi Amoako: Writing – review & editing. All authors contributed to manuscript revision, read, and approved the submitted version.
Acknowledgments
This work was supported, in part, by the Bill & Melinda Gates Foundation [OPP1198373] through IITA and ICRISAT on the project “Accelerated Varietal Improvement and Seed Delivery of Legumes and Cereals in Africa (AVISA).” The CSIR-Savanna Agricultural Research Institute (SARI) and the Department of Crop Science of the University for Development Studies are duly acknowledged for the partnership to ensure successful implementation of this work.
Disclosure statement
No potential conflict of interest was reported by the authors.
Additional information
Funding
Notes on contributors
Theophilus Kwabla Tengey
Theophilus Tengey is a Research Scientist (Plant Breeder) at the CSIR-Savanna Agricultural Research Institute (SARI) and leads the cowpea Improvement Programme of the Institute.
Raphael Adu Gyamfi
Dr. Raphael Adu-Gyamfi is an Associate Professor of Plant Science at the University for Development Studies (UDS), Nyankpala.
Edward Kofi Sallah
Mr. Edward Kofi Sallah is a lecturer (Plant Pathology) at the UDS.
Memunatu Issahaku
Issahaku Memunatu is a Technical Officer at the CSIR-SARI.
Dominic Ngagmayan Ndela
Dominic Ngagmayan Ndela holds an MPHIL degree in Crop Science from UDS.
Mariam Seidu
Mariam Seidu is a Technical Officer at the CSIR-SARI.
Abbas Francis Senyabor
Abbas Senyabor is a Technical officer with the CSIR-SARI.
Emmanuel Israel Affram
Emmanuel Israel Affram is Graduate Research Associate with the CSIR–SARI.
Ophelia Asirifi Amoako
Ophelia Asirifi Amoako is a Research Scientist at the CSIR-SARI with specialty in cropping systems.
Charles Naapoal
Charles Naapoal is a Research Assistant at the CSIR-SARI.
References
- Ajayi, A. T., Gbadamosi, A. E., & Olumekun, V. O. (2018). Screening for drought tolerance in cowpea (Vigna unguiculata L. Walp) at seedling stage under screen house condition. International Journal of BioSciences and Technology, 11(1), 1–20.
- Alidu, S. M., Asante, I. K., Tongoona, P., Ofori, K., Danquah, A., & Padi, F. K. (2019). Development and screening of cowpea recombinant inbred lines for seedling drought tolerance. Journal of Plant Breeding and Crop Science, 11(1), 1–10. https://doi.org/10.5897/JPBCS2018.0768
- Araus, J. L., & Hogan, K. P. (1994). Comparative leaf structure and patterns of photoinhibition of the neotropical palms. Scheelea zonensis and Socratea durissima growing in clearing and forest understory during the dry season in Panama. American Journal of Botany, 81(6), 726–738. https://doi.org/10.1002/j.1537-2197.1994.tb15507.x
- Arjenaki, F. G., Jabbari, R., & Morshedi, A. (2012). Evaluation of drought stress on relative water content, chlorophyll content and mineral elements of wheat (Triticum aestivum L.) varieties. International Journal of Agriculture and Crop Sciences, 4(11), 726–729.
- Arunyanark, A., Jogloy, S., Akkasaeng, C., Vorasoot, N., Kesmala, T., Nageswara Rao, R. C., Wright, G. C., & Patanothai, A. (2008). Chlorophyll stability is an indicator of drought tolerance in peanut. Journal of Agronomy and Crop Science, 194(2), 113–125. https://doi.org/10.1111/j.1439-037X.2008.00299.x
- Baker, N. R., & Rosenqvist, E. (2004). Applications of chlorophyll fluorescence can improve crop production strategies: An examination of future possibilities. Journal of Experimental Botany, 55(403), 1607–1621. https://doi.org/10.1093/jxb/erh196
- Cairns, J. E., Hellin, J., Sonder, K., Araus, J. L., Macrobert, J. F., Thierfelder, C., & Prasanna, B. M. (2013). Adapting maize production to climate change in sub-Saharan Africa. Food Security, 5(3), 345–360. https://doi.org/10.1007/s12571-013-0256-x
- Carvalho, M., Matos, M., Castro, I., Monteiro, E., Rosa, E., Lino-Neto, T., & Carnide, V. (2019). Screening of worldwide cowpea collection to drought tolerant at a germination stage. Scientia horticulturae, 247, 107–115. https://doi.org/10.1016/j.scienta.2018.11.082
- Ceccarelli, S., Grando, S., Baum, M., & Udupa, S. M. (2004). Breeding for drought resistance in a changing climate. In Rao, S. C., Ryan, J. (Eds.), Challenges and Strategies for Dry/and Agriculture. 32pp. 167–190. Crop Science Society of America and American Society of Agronomy.
- da Silva, C. A., da Costa, S. D., Junior Teixeira, D. L., da Silva, P. B., dos Santos, R. C., & Siviero, A. (2018). Cowpea : A strategic legume species for food security and and health. In Legume Seed Nutraceutical Research (pp. 47–65). IntechOpen. https://doi.org/10.5772/intechopen.79006
- Fahad, S., Bajwa, A. A., Nazir, U., Anjum, S. A., Farooq, A., Zohaib, A., Sadia, S., Nasim, W., Adkins, S., Saud, S., Ihsan, M. Z., Alharby, H., Wu, C., Wang, D., & Huang, J. (2017). Crop production under drought and heat stress: Plant responses and management options. Frontiers in Plant Science, 8(1147), 1–16. https://doi.org/10.3389/fpls.2017.01147
- FAOSTAT. (2020). Food and Agriculture Organization of the United Nations. www.fao.org/faostat/en/#data
- Freire Filho, F. R., Ribeiro, V. Q., Rocha, M., de, M., E Silva, K. J. D., Nogueira, M., Do S da, R., & Rodrigues, E. V. (2012). Production, breeding and potential of cowpea crop in Brazil. Plant Physiology and Biochemistry: PPB, 51, 145–152. https://doi.org/10.1016/j.plaphy.2011.10.008
- Gnankambary, K., Sawadogo, N., Di´eni, Z., Batieno, T. B. J., Tignegr´e, J. B. D. S., Sawadogo, M., & Ou´edraogo, T. J. (2020). Assessment of cowpea (Vigna unguiculata (L.) Walp.) mutant lines for drought tolerance. International Journal of Agronomy, 2020, 1–9. https://doi.org/10.1155/2020/8823498
- Gonçalves, A., Goufo, P., Barros, A., Domínguez-Perles, R., Trindade, H., Rosa, E. A. S., Ferreira, L., & Rodrigues, M. (2016). Cowpea (Vigna unguiculata L. Walp), a renewed multipurpose crop for a more sustainable agri-food system: Nutritional advantages and constraints. Journal of the Science of Food and Agriculture, 96(9), 2941–2951. https://doi.org/10.1002/jsfa.7644
- Gonzalez, L. M. (1996). Use of radioinduction of mutations in obtaining salinity-tolerant rice genotypes.
- Hakam, N., Khanizadeh, S., Deell, J. R., & Richer, C. (2000). Assessing chilling tolerance in roses using chlorophyll fluorescence. HortScience, 35(2), 184–186. https://doi.org/10.21273/HORTSCI.35.2.184
- Ibitoye, D. O. (2015). Genetic analysis of drought tolerance in cowpea [Vigna Unguiculata (L.) Walp] Issue December. University of Ghana.
- Isma’ila, M., Ramlatu, M. A., & Zakari, B. G. (2016). Screening of selected varieties of cowpea seedlings [Vigna unguiculata (L.) Walp.] for drought tolerance. Journal of Biology and Nature, 5(1), 31–38.
- Kebede, E., & Bekeko, Z. (2020). Expounding the production and importance of cowpea (Vigna unguiculata (L.) Walp.) in Ethiopia. Cogent Food & Agriculture, 6(1), 1–21. https://doi.org/10.1080/23311932.2020.1769805
- Khan, M. I. R., Nazir, F., Asgher, M., Per, T. S., & Khan, N. A. (2015). Selenium and sulfur influence ethylene formation and alleviate cadmium-induced oxidative stress by improving proline and glutathione production in wheat. Journal of Plant Physiology, 173, 9–18. https://doi.org/10.1016/j.jplph.2014.09.011
- Mai-Kodomi, Y., Singh, B., Myers, O. E., Yopp, J. H., Gibson, P., & Terao, T. (1999). Two mechanisms of drought tolerance in cowpea. Indian Journal of Genetics and Plant Breeding, 59(3), 309–316.
- Monteoliva, M. I., Guzzo, M. C., & Posada, G. A. (2021). Breeding for drought tolerance by monitoring chlorophyll content. Gene Technology, 10(3), 1–11.
- Munné-Bosch, S., Jubany-Marí, T., & Alegre, L. (2001). Drought-induced senescence is characterized by a loss of antioxidant defences in chloroplasts. Plant, Cell & Environment, 24(12), 1319–1327. https://doi.org/10.1046/j.1365-3040.2001.00794.x
- Nkomo, G. V., Sedibe, M. M., & Mofokeng, M. A. (2021). Production constraints and improvement strategies of Cowpea (Vigna unguiculata L. Walp.) genotypes for drought tolerance. International Journal of Agronomy, 2021, 1–9. https://doi.org/10.1155/2021/5536417
- Nunes, C., Moreira, R., Pais, I., Semedo, J., Simões, F., Veloso, M. M., & Scotti-Campos, P. (2022). Cowpea physiological responses to terminal drought—Comparison between four landraces and a commercial variety. Plants, 11(5), 593. https://doi.org/10.3390/plants11050593
- Oliveira, R. M., de, M., Filho, F. R. F., Ribeiro, V. Q., Lopes, Â. C., de, A., Bernardo, K. A., da, S., & Cruzio, A. S. (2016). Diallel analysis in cowpea aiming at selection for extra-earliness. Crop Breeding and Applied Biotechnology, 16(3), 167–173. https://doi.org/10.1590/1984-70332016v16n3a26
- Omomowo, O. I., & Babalola, O. O. (2021). Constraints and prospects of improving cowpea productivity to ensure food, nutritional security and environmental sustainability. Frontiers in Plant Science, 12, 1–25. https://doi.org/10.3389/fpls.2021.751731
- Percival, G. C., & Sheriffs, C. N. (2002). Identification of drought-tolerant woody perennials using chlorophyll. Arboriculture & Urban Forestry, 28(5), 215–223. https://doi.org/10.48044/jauf.2002.032
- Pirzad, A., Shakiba, M. R., Zehtab-Salmasi, S., Mohammadi, S. A., Darvishzadeh, R., & Samadi, A. (2011). Effect of water stress on leaf relative water content, chlorophyll, proline and soluble carbohydrates in Matricaria chamomilla L. Journal of Medicinal Plants Research, 5(12), 2483–2488.
- Ravelombola, W., Shi, A., Chen, S., Xiong, H., Yang, Y., Cui, Q., Olaoye, D., & Mou, B. (2020). Evaluation of cowpea for drought tolerance at seedling stage. Euphytica, 216(8), 1–19. https://doi.org/10.1007/s10681-020-02660-4
- Ravelombola, W., Shi, A., Qin, J., Weng, Y., Bhattarai, G., Zia, B., Zhou, W., & Mou, B. (2018). Investigation on various aboveground traits to identify drought tolerance in cowpea seedlings. HortScience, 53(12), 1757–1765. https://doi.org/10.21273/HORTSCI13278-18
- Santos, R., Carvalho, M., Rosa, E., Carnide, V., & Castro, I. (2020, October). Root and agro-morphological traits performance in cowpea under drought stress. Agronomy, 10(10), 1604. https://doi.org/10.3390/agronomy10101604
- Singh, B. B., Matsui, T. (2002). Cowpea varieties for drought tolerance. In Fatokun, C. A., Tarawali, S. A., Singh, B. B., Kormawa, P. M. and Tamo, M., Cowpea varieties for drought tolerance. Proceedings of the World Cowpea Conference III, Ibadan, Nigeria (pp. 287–300).
- STAR, version 2.0.1 (2014). Biometrics and Breeding Informatics. PBGB Division, International Rice Research Institute, Los Baños, Laguna.
- Tomar, R. S. S., Tiwari, S., Vinod Naik, B. K., Chand, S., Deshmukh, R., Mallick, N., Singh, S., Singh, N. K., Tomar, S. M. S., & Tomar, S. M. S. (2016). Molecular and morpho-agronomical characterization of root architecture at seedling and reproductive stages for drought tolerance in wheat. PLos One, 11(6), 1–21. https://doi.org/10.1371/journal.pone.0156528
- Tumwesigye, E., & Musiitwa, F. (2002). Characterizing drought patterns for appropriate development and transfer of drought resistance maize cultivar in Uganda. 7th Eastern and Southern Africa Regional Maize Conference and Symposium on Low-Nitrogen and Drought Tolerance in Maize, Nairobi, Kenya (pp. 260–262).
- Valladares, F., Dobarro, I., Sa´nchez-Go´mez, D., & Pearcy, R. W. (2005). Photoinhibition and drought in Mediterranean woody saplings: Scaling effects and interactions in sun and shade phenotypes. Journal of Experimental Botany, 56(411), 483–494. https://doi.org/10.1093/jxb/eri037
- Vasconcelos, M. I., Maia, M. F. M., Farias, D. F., Campello, C. C., Carvalho, A. F. U., Moreira, R., de, A., & Oliveira, J. T. A. (2010). Protein fractions, amino acid composition and antinutritional constituents of high-yielding cowpea cultivars. Journal of Food Composition & Analysis, 23(1), 54–60. https://doi.org/10.1016/j.jfca.2009.05.008
- Verbree, D. A., Singh, B. B., & Payne, W. A. (2015). Genetics and heritability of shoot drought tolerance in cowpea seedlings. Crop Science, 55(1), 146–153. https://doi.org/10.2135/cropsci2014.02.0137
- Yahaya, D., Denwar, N., & Blair, M. W. (2019). Effects of moisture deficit on the yield of cowpea genotypes in the Guinea Savannah of northern Ghana. Agricultural Sciences, 10(04), 577–595. https://doi.org/10.4236/as.2019.104046