Abstract
Of the 12 medicinal ginseng species, three species (Panax ginseng, P. quinquefolium, and P. notoginseng) are significant given their biological activities and hence are widely used around the world. These species have cardiovascular protection, improved immunomodulatory ability, antioxidant, anti-tumor, cholesterol-lowering, anti-inflammatory and other effects. Hence, such ginseng is used in various products (foods, health foods, daily necessities, cosmetics, etc.). However, ginseng cultivation causes replanting problems due to its allelopathic effects. This review provides a comprehensive insight into the allelopathic ginseng plant and analyzes the allelopathic effects on soil diseases and soil microorganisms for ginseng researchers. More in-depth research is needed to reveal the mechanisms of ginseng allelopathy.
1. Introduction
It is known that ginseng is a kind of the most precious medicinal plants in the world. Its main component is ginsenoside, which has important pharmacological and medicinal uses (Ratan et al., Citation2021). Ginseng products benefit qi (qi refers to the vital energy that flows throughout the body, supporting its functions and promoting overall health (Putiri et al., Citation2017), invigorate the spleen to eliminate humidity, and replenish blood to nourish the heart (M. Liu, Pan, et al., Citation2022). Ginseng roots contain saponins, polysaccharides, organic acids, proteins, volatile oils, and trace elements (H. J. Liu et al., Citation2018). Hence, ginseng is used to treat
cardiovascular system and central nervous system diseases, improve myocardial function, improve memory, enhance immunity, and improve sleep, as well as to have antiaging, anti-shock, and anti—myocardial ischemia properties, among others. Historically, most ginseng species were wild and non-domesticated, while its artificial cultivation has developed throughout the past 50–60 years. Ginseng requires specific climate and soil conditions; hence, it is only artificially cultivated in China, South Korea, North Korea, and Russia (Natalie et al., Citation2022). Ginseng root exudates contain many chemicals (phenolic acids, organic acids, esters, alkanes, ginsenoside, etc.) that are detrimental to its continuous cropping (Le et al., Citation2019, Citation2020; Luo et al., Citation2022). In the ginseng rhizosphere, the secreted chemicals play inhibition role for the ginseng growth with the functions of disrupting the balance the microbial flora and cause soil diseases (Chandra et al., Citation2021; G. L. Tian et al., Citation2021). Ginseng is a highly useful medicinal commercial plant; moreover, ginseng products are often sold as high-end gifts (Figures ). Therefore, this review discusses ginseng’s chemical composition, medicinal values, cultivation, and allelopathic effects, which may be helpful in ginseng research and future developments in the ginseng industry.
2. Ginseng cultivation
2.1. Ginseng species of the Panax genus
Twelve medicinal ginseng species are grown in different countries and used in traditional medicines (Table ). Traditional Chinese medicinal plants of the Panax genus include the following: Ginseng (Panax ginseng), American ginseng (P. quinquefolium) and Notoginseng (P. notoginseng). They facilitate various bioactivities, such as cardiovascular protection and immunomodulatory ability, as well as antioxidant, anti-tumor, cholesterol-lowering, and anti-inflammatory properties (Jang et al., Citation2022). Therefore, these functions are beneficial to overall health and have been commercialized and sold worldwide in health foods, cosmetics, etc (Gong et al., Citation2016). Regarding differentiation, ginseng species are difficult to distinguish; hence, some research has been done to categorize them. B. B. Kim et al. (Citation2005) used Amplified Fragment Length Polymorphism (AFLP) to distinguish Korean P. ginseng from Chinese P. ginseng and P. quinquefolius. Due to the presence of phenolic compounds, Wee et al. (Citation2020) detected anticoagulant components in Korean red ginseng. Sun et al. (Citation2010) successfully used real time PCR and single nucleotide polymorphism (SNP) markers to distinguish “Chunpoong” from many ginseng cultivars. In order to help businesses and customers understand what red ginseng is made of, a monograph on the herb was created; The Korea Food and Drug Administration has approved red ginseng as a health food due to its effectiveness (So et al., Citation2018). By examining the mitochondrial nicotinamide adenine dinucleotide (NADH) dehydrogenase subunit 7 (nad7) intron 3 regions, G. Li et al. (Citation2017) created a quick and accurate approach to distinguish Russian wild ginseng from Chinese-produced ginseng. And an amplification refractory mutation system (ARMS) was utilized by J. G. In et al. (Citation2010) to identify Korean mountain ginseng. Researchers have not only differentiated the types of ginsengs from the perspective of morphology-they have also distinguished ginseng types from a molecular perspective (Goodwin & Proctor, Citation2019).
Table 1. List of 12 ginseng spp. distributed in the world
2.2. Metabolic physiology
Numerous research have documented the pharmacological effects of ginseng root on human health, including immune-stimulating, anti-cancer, antiaging, anti-oxidative, and blood pressure regulation properties. The presence of ginsenosides, polysaccharides, polyacetylenic alcohols, and peptides is what causes these pharmacological effects (Attele et al., Citation1999). Recent research on the ginseng metabolome have shown that it changes depending on the plant’s components, origins, and ages of cultivation (J. Liu et al., Citation2017; Kim et al., Citation2018). But limited knowledge exists regarding how ginseng growing seasons affect its metabolism. And with A proton NMR (1H NMR)-based metabolomics technique was used to investigate the metabolic changes of ginseng roots and leaves during their growing seasons (H. J. Lee et al., Citation2019). There needs a dearth of information on ginseng metabolomics, and more study is required.
2.3. Culture methods
Y. J. Kim et al. (Citation2018) investigated the physiochemical properties of cultivated P. ginseng sprouts with nanobubble water, as well as the antioxidative activity of its enzymatic hydrolysates. Nanobubble water promoted the height and thickness of P. ginseng sprouts compared to normal water; the cultivated P. ginseng sprout composition (ash, crude fat, and crude protein) was similar to other cultured groups. The contents of crude saponin, total ginsenoside, total polyphenol, and flavonoid were higher in NB-4 than in NA-4. Among the hydrolysates, the fungamyl hydrolysate had the highest ABT radical scavenging activity while the viscozyme hydrolysate had the highest DPPH radical scavenging activity (D. G. Kim et al., Citation2018). After the rice harvest, and it was evaluated the growth traits and quality of P. ginseng growing in upland and lowland paddy fields (S. W. Lee et al., Citation2004). The upland ginseng had a higher survival rate and shoot growth than that grown in lowland paddy fields. The lowland paddy field ginseng taproot was harder and rustier than the upland ginseng. In comparison with paddy ginseng, the taproot of upland ginseng was lighter when getting dried. In-situ N-containing water production was created by Song et al. (Citation2021) utilizing plasma technology, and the effects of the plasma-treated water on ginseng growth and its bioactive phytochemicals were assessed. And the improvement of far-red and UV-B light have the positive effects on the extending of ginseng growth (J. Choi et al., Citation2022). The findings demonstrated that P. ginseng growth and phytochemical accumulation during sprouting under aeroponic circumstances might be facilitated by the use of plasma-treated water as a liquid N fertilizer. The biomass and active ingredients of ginseng were significantly impacted by various ginseng growth techniques.
2.4. Field management
The ginseng culture soils were comprised of silt loam, loam, and sandy loam in less slope, alluvial, or local valley. The suitable soil pH was in the range of 5.0 to 5.2, and its EC was in the range of 0.93 to 3.78 ds m−1 for ginseng culture. Nitrate nitrogen was between 63 and 490 mg kg−1, accessible P2O5 was between 55 and 163 mg kg−1, and organic matter was between 37 and 35 g kg−1. Compared to the cultivation of Korean ginseng, the land was more fertile (Y. H. Park et al., Citation2016). There are three different sizes of ginseng cultivation scales: small scales of 0.5–3.0 ha, middle scales of 4.0–10.0 ha, and giant scales of 30–700 ha. Direct seeding and ginseng seedling transplantation are the two techniques used to grow ginseng on recently cleared forest land. Ginseng field beds were shaded with 100–130 cm tall arches made of vinyl films of various colors. According to research by K. Kim et al. (Citation2020), locations, where ginseng has been farmed for 13 years as opposed to 7 years, significantly higher soil organic matter (OM), total nitrogen (TN), and cation exchangeable capacity (CEC). There were much more ginsenoside G-Rb1, Rb2, Rc, Rd, Re, Rf, and Rg1 in the former. The OM, TN, and CEC of the farmed soils were favorably connected with ginseng growth, and the root length and ginsenoside content were positively correlated. Different field management also has a significant impact on the accumulation of ginseng biomass and active substances (H. Yu et al., Citation2019; Kawakatsu & Fukuda, Citation2023); hence, traditional field management should be improved.
2.5. Rhizosphere microbes
Ginseng’s lateral roots were easier for colonization after being inoculated with the Glomus intraradice, those also boosted the concentrations of monomeric and total ginsenosides, as well as the root activity, polyphenol oxidase, and catalase activities (L. Tian et al., Citation2019). The ginseng rhizosphere soil can benefit from the inoculation of G. intraradices, because it enhances some helpful bacterial species, reduces harmful fungus, and benefits ginseng yields. The quality of Panax ginseng is diminished by a number of abiotic factors, for example, high levels of heavy metals in ginseng growth locations. Rhizobium panacihumi DCY116T in ginseng seedlings can increase the biomass, proline, total phenolic content, total soluble sugar content, and related gene expressions while lowering H2O2 levels less than in non-bacterized Al-stressed seedlings. And the Rhizobium panacihumi could be used as growth-promoting rhizobacteria for the future production of ginseng or other crops in those areas exposed to heavy metals (Kang et al., Citation2021).
It is reported that the continuous cropping practices for P. notoginseng and severe root-rot disease notably affected the community structure and the diversity of rhizospheric and root endophytic bacteria (Tan et al., Citation2017). And the oxidation and deposition of rhizosphere Fe, Al, and Mn facilitated by nitrate-dependent Fe (II)-oxidizing bacteria might be key factors contributing to rusty root disease (Q. Wang et al., Citation2019). In ginseng, the use of Paenibacillus yonginensis DCY84T to improve salt stress tolerance; ginseng primed with DCY84T and exposed to 300 mM NaCl showed the same metabolite profile as control ginseng plants, suggesting that DCY84T effectively reduced salt stress (Sukweenadhi et al., Citation2018).
2.6. Soil-borne diseases
The growth, yield, and quality of the ginseng root system are major affected by the two principal soil-borne root diseases of ginseng: rust and mycoplasmosis (S. Xia et al., Citation2000). Furthermore, other relevant pathogens in soil include the following: Fusarium solani, Rhizoctonia solani, Alternaria panax, Pythium debaryanum, and Phytophthora cactorum. In addition, there are about 20 kinds of parasitic nematodes harmful to ginseng growth, with M. changbaiensis and Meloidogyne hapla being two major groups. The mites H. sapronyzarum, Hisriostoma feroniarum, and Rizoglyphus echiphus are also harmful to ginseng growth (S. Xia et al., Citation2000). The pathogenic mechanism of nematodes and mites is to create a wound in the ginseng root’s epidermis, opening it up for pathogens and infection, causing root diseases.
2.7. Biocontrol agents
Ginseng produces bioactive metabolites such as ginsenosides, oleanic acids, and phenolic compounds, which have pharmacological properties, including anticancer, antiplatelet, and antimicrobial activities (Y. H. Park et al., Citation2019). Mountain-cultivated ginseng (MCG) is propagated under natural conditions in the forest and requires a long period of cultivation.
During three or five years of ginseng cultivation in Korea, various diseases reduce the P. ginseng yield up to 30–60%. Bae et al. (Citation2004) have suggested that some rhizobacteria could induce resistance against various plant diseases in ginseng. Botrytis cinerea communities from Asian ginseng of northeastern China have been examined and tested for their traits of resistance to several fungicides. Lu et al. (Citation2015) demonstrated that the efficacy of existing fungicides is decreased by B. cinerea‘s multi-fungicide resistance. Based on the expression patterns against biotic and abiotic factors, O. R. Lee et al. (Citation2011) compiled the potential defense-related genes in ginseng and figured out their functional roles. The biological activities of ginseng protein affect the defense responses dependent on salicylic acid or jasmonic acid. However, some unknown genes suppress specific pathogens, while others restrict their growth. Inoculating the rhizosphere soil of ginseng with beneficial bacteria has been shown to promote the growth of mycorrhiza, which is conducive to the cultivation of ginseng.
Ginseng is susceptible to being attacked by pathogens which severely affect its quality and yield. It is elevated content of L-valine, phenylalanine, tropic acid, SA, and saccharopine in ginseng leaves and the accumulation of α-linolenic acid, arachidonic acid, palmitic acid, arachidic acid, SA, and saccharopine in ginseng stems are considered the DRMs closely associated with P. cactorum infection which can be exploited to be candidates of diagnostic biomarkers (Kan et al., Citation2023). And the Paenibacillus yonginensis DCY84T (KCTC33428, JCM19885) displays plant growth promotion via induced systemic resistance of abiotic stresses from Gram-positive rod-shaped bacterium isolated from humus soil of Yongin Forest in Gyeonggi Province, South Korea (Y. J. Kim et al., Citation2017).
3. Continuous cropping problem
Ginseng cannot be replanted in the same field within 10–20 years, because it causes roots rot, frequent diseases and reduces the yield and quality. The problem of continuous ginseng cropping has become the main limitation for ginseng industry development. The reasons for the continuous cropping problem are very complicated. Till now, the following reasons of such soil sickness have been studied: (i) aggravation of soil-borne pathogens, (ii) autotoxicity (allelopathic toxicity of root exudates), and (iii) imbalance of soil microorganisms.
3.1. Soil-borne disease
In farmlands, the years of continues cultivation of ginseng (P. ginseng) may result the microbial makeup changes in the soil, which in turn would cause the boosts of soil-borne disease. To create an effective technology for P. ginseng cropping, the effects of culture methods and duration on the variety and composition of the P. ginseng rhizosphere microbial community was investigated in many research. Based on the farm and forest field cultivation, there are noticeable differences in the richness, variety, and relative abundance of microbial species. When compared to farm soil, the bacterial communities in forests (where P. ginseng not grown) were more diverse and rich (where P. ginseng was grown). Although the same lands with the long-time cultivation would have been removed for farming and deforestation has drastically the range of microbial communities in the soil. With the rapidly meet of P. ginseng, the ginseng has been planted for centuries. The quantity of soil-borne pathogens (Monographella cucumerina, Ilyonectria mors-panacis, I. robusta, Fusarium solani, and Nectria ramulariae) presents varied with ages (Tong et al., Citation2021). Lu et al. (Citation2020) investigated the taxonomy of the fungal complex that causes red-skin root disease in Chinese ginseng, and it was found that a total of 230 isolates from 209 sickness samples were obtained and classified into 12 species (Dactylonectria spp., D. hordeicola, F. acuminatum, F. avenaceum, F. solani, F. torulosum, I. mors-panacis, I. robusta, and Rhexocercosporidium panacis, as well as three novel species I. changbaiensis, I. communis, and I. qitaiheensis). Additionally, the primary communities constructure of the microbiology were I. communis (36.1%), I. robusta (20.9%), and F. solani (23.9%); those were all harmful to ginseng roots and caused red-skin root disease under specific circumstances. Typically, a prominent pathogenic fungus was found in ginseng cultivation is F. oxysporum; the antagonistic fungi is named as Penicillium sp. YJM-2013, which has been discovered to be more conductive to the accumulation of ginsenosides in P. ginseng adventitious roots than F. oxysporum. The generation of signal molecules is increased, and transcription factors and functional genes are activated in their expression (S. H. Wang et al., Citation2020). The sensitivity to total ginsenoside fraction (T-GF), protopanaxadiol-type ginsenoside fraction (PPD-GF), and protopanaxatriol-type ginsenoside fraction (PPT-GF) in the roots was investigated in the ginseng pathogen Cylindrocarpon destructans and five non-pathogens. At the same time, the root rot pathogen, Cylindrocarpon destructans and Fusarium solani, can assist the ginseng improve resistance to stress (T. Li et al., Citation2020). And the five non-pathogens and the ginseng pathogen Cylindrocarpon destructans were tested for sensitivity to total ginsenoside fraction (T-GF), protopanaxadiol-type ginsenoside fraction (PPD-GF), and protopanaxatriol-type ginsenoside fraction (PPT-GF) in the roots of P. ginseng. The results demonstrated that ginsenosides may serve as host chemical defenses, while the one potential pathogenicity factor for ginseng root infections is their capacity to deglycosylate ginsenosides of the PPD class (Zhao et al., Citation2012).
3.2. Autotoxicity
Panax ginseng, a perennial medicinal plant, which cannot be planted again in the same field in the following 10 to 20 years. Because the issues like root rot and recurrent disease would arise, leading in low harvests. Salicylic acid, gallic acid, benzoic acid, 3-phenyl propionate, and cinnamic acid are the primary allelochemicals secreted by the roots of ginseng. These have allelopathic effects that reduce the diversity and number of soil microbes, making ginseng planting difficult for the next harvest. L. Li et al. (Citation2018) conducted research on auto-toxic compounds, as well as mitigation method to address the continuous cropping problem of ginseng. Metagenomic molecular sequencing has been used to determine the autotoxic phenolic acids produced by ginseng roots, which can change the fungal structure and diversity in ginseng rhizosphere soil. Trichoderma harzianum effectively inhibits the growth of the main disease (C. destructans) related to the continuous cropping of ginseng, reducing the autotoxic phenolic acids present in the soil and colonizing the soil-fertilizer mixture for an extended time; this study proposed a type of disease prevention using a control technology comprised of “physical disinfection + chemical elimination + biological fertilizer recovery. ” This technology can improve the soil’s microorganisms, reduce the incidence of ginseng diseases and improving the yield and quality of ginseng spp. Research on auto-toxic substances and mitigation strategies was raised up by L. Li et al. (Citation2018) to address the issue of ginseng being continuously harvested. The autotoxic phenolic acids produced by ginseng roots, which can alter the variety and structure of the fungi in the ginseng rhizosphere soil, have been identified using metagenomic molecular sequencing. This study proposed a type of disease prevention using a control technology made up of “physical disinfection + chemical elimination + biological fertilizer recovery.” Trichoderma harzianum effectively inhibits the growth of the main disease (C. destructans) related to the continuous cropping of ginseng, reducing the autotoxic phenolic acids present in the soil and colonizing the soil-fertilizer mixture for an extended period of time.
3.3. Soil microorganisms
The effects of successive cropping on the microecology of ginseng rhizosphere soil and the related mechanisms were listed in the present research. The results revealed that the numbers and populations of microorganisms and enzyme activities were remarkably changed in continuously cropped ginseng soil. The presence of Medicago sativa, Fructus perillae, and Trifolium repens increased the populations of microorganisms in the ginseng soil (M. Li, Citation2008). The abundance and diversity of soil microorganisms involved in continuous ginseng cropping have undergone significant changes, thus causing problems specifically related to this type of cropping.
Due to the auto-toxic substances and mitigation strategies raised up by L. Li et al. (Citation2018) ginseng continuously harvested was emphrased that the autotoxic phenolic acids produced by ginseng roots, which can alter the variety and structure of the fungi in the ginseng rhizosphere soil, have been identified using metagenomic molecular sequencing. And L. Li et al. (Citation2018) proposed a type of disease prevention using a control technology made up of “physical disinfection + chemical elimination + biological fertilizer recovery.” Trichoderma harzianum effectively inhibits the growth of the main disease (C. destructans) related to the continuous cropping of ginseng, reducing the autotoxic phenolic acids present in the soil and colonizing the soil-fertilizer mixture for an extended period of time. In addition, the microbial composition of the soil, as well as the quantity, variety, and traits of the soil microflora of P. notoginseng in connection to a rise in the continuous cropping years, those were all investigated by H. J. Liu et al. (Citation2018). The findings revealed that bacteria and fungus dominated in the microbial communities of P. notoginseng. Moreover, the soil microflora changed significantly with the seasons and cropping years, resulting in the failure of replanted P. notoginseng. It was identified that the antifungal properties of six major ginsenosides (Rb1, Rb2, Rc, Rd, Re, and Rg1) as well as the metabolites of ginsenoside Rb1 (Gypenoside XVII [G-XVII] and F2) against the ginseng root pathogen C. destructans (J. Wang et al., Citation2018); and other pathogens such as F. graminearum, and it was determined that C. destructans pathogenicity toward ginseng root was unrelated to its capacity to deglycosylate ginsenosides (L. Tian et al., Citation2019). At the same condition, in fields with continuous maize, monoculture ginseng, and one-, three-, and five-year maize rotations after ginseng, Jiao et al. (Citation2019) analyzed nutrients, phenolic acids, and microbial communities; the results demonstrated that a three-year maize rotation was required to overcome the replant failure of American ginseng mostly. H. Li et al. (Citation2008) examined the impact of P. notoginseng continuous cropping on the characteristics of rhizosphere soil and discovered that pH variations and nutrient imbalances may be the root of P. notoginseng continuous cropping issue. In particular, ginsenosides and their metabolites perform antibacterial activities against pathogens, changing soil nutrients, and changing pH levels, thereby changing the diversity of the soil microorganisms.
4. Medicinal importance
Ginseng is highly valued for its therapeutic applications since it contains a variety of potent compounds that are advantageous to human health. The pharmacological action of it has been investigated globally. Numerous consumer goods have been created as a result of ginseng’s discovery as being very useful for human health.
4.1. Active substances
The key ingredient responsible for ginseng’s medicinal properties is ginsenoside (Table ). And most studies on their structures and bio-activities have been detected and analyzed (H. Liu et al., Citation2020a; L. Li et al., Citation2018; Wu et al., Citation2012). Ginseng contains oligosaccharides, which T. Li et al. (Citation2020) discovered and created a method to identify and test the existance. According to research performed by Fu et al. (Citation2014), it is found that ginseng leaves’ crude polysaccharides of the side effect on the human hepatoma cell SMMC-7721, GLCP enhanced autophagy and death in this cell type. Caveolin-1, a crucial structural molecule of caveolae, may also be linked to the process. Ginseng leaves had a weak inhibitory effect on liver cancer, although the chemical played a decreased trend in tumor.
Table 2. Ginseng chemical compounds useful in medicine, food and health related products
The ginsenoside contents of P. ginseng were detected that Rg1, 1.3 mg g−1; Re, 1.3 mg g−1, Rb1: 6.4 mg g−1, Rc: 2.5 mg g−1, Rb2: 2.3 mg g−1, and Rd: 0.9 mg g−1, according to M. H. Lee et al. (Citation2015) report that the water extraction was fixed at 90 °C for 14–16 h and then the concentration procedures. Traditional detective methods should be well preserved, as the specific ginseng species has its own chemical characteristics.
According to Choi’s report that the ginsenoside and phenolic contents of hydroponically grown ginseng leaves, fruits, and roots were different; in details, the ginseng grown hydroponically had leaves with greater concentrations of ginsenosides (Rg1, Rg2+Rh1, Rd, and Rg3) and p-coumaric acid than ginseng grown in the wild. The leaves’ 2, 2’-azino-di-(3-ethylbenzothiazoline)-6-sulfonic acid radical-scavenging capacities peaked at this period. As a result, ginseng leaves cultivated hydroponically had a natural antioxidant that was good to the environment.
H. Li et al. (Citation2008) developed an effective method for purifying ginsenosides from red, white, and grown wild ginseng roots using microporous resins. Siddiqi et al. (Citation2013) succesfully concentrated on the various molecular chemicals that include the nuclear factor kappa-B receptor activator, nuclear factor kappa-B ligand, matrix metallopeptidase-9, bone morphogenetic protein-2, and Smad-signaling pathways in order to highlight the evidence supporting the therapeutic properties of ginsenosides both in vivo and in vitro. By steaming or sun drying Panax ginseng, the active ingredients can be altered. Six malonyl-ginsenosides (Rg1, Rb1, Rb3, Rc, Rd, and Rb2) and 15 amino acids had less of their concentration after steaming. Ginsenoside-Rh1, 20(S)-Rg2, 20(S, R)-Rg3, and Maillard reaction product, on the other hand, such as AF (arginine-fructose), AFG (arginine-fructose glucose), and maltol concentrations were increased. These findings can be fit for P. ginseng quality assurance and standardization, offering a solid scientific foundation for pharmacological studies on processed ginseng (G. In et al., Citation2017).
A viable liquid chromatography diode array detection (LC-DAD) method was created for the first time to analyze 14 ginsenosides in ginseng gathered from 66 distinct production regions in Northeast China concurrently. The findings showed that, among other things, the growth environment and cultivation method affected the quality of ginseng roots and rhizomes from various sources. Numerous other ginsenosides may be discovered using the newly developed LC-ESI-IT-TOF-MSn technology, and the LC-DAD method could be utilized to evaluate the potency of ginseng and improve the growing conditions for ginsenoside production (H. P. Wang et al., Citation2016). One of the metabolites of ginsenosides found in red ginseng root (c. a. Meyer), Compound K in wild type ginseng, 20-O-(d-glucopyranosyl)-20(S)-protopanaxadiol, has a high bioavailability. The ideal conditions for enzyme treatment to convert ginsenosides from red ginseng extract to control have been developed by Y. J. Park et al. (Citation2021), and the properties of bio-converted red ginseng extract have also been demonstrated.
Widespread fermentation technique is utilized to change the ginseng’s active ingredients. After examining the features and antioxidant activity of ginseng seeds fermented by Bacillus, Lactobacillus, and Pediococcus strains, M. H. Lee et al. (Citation2015) found that fermented ginseng seeds had greater levels of 3-ethylbenzothiazoline-6-sulfonic acid and SOD than unfermented ginseng seeds. To detect the triterpenoids in ginseng, H. P. Wang et al. (Citation2016) created the high-performance liquid chromatography combined with electrospray ionization ion trap time-of-flight multistage mass spectrometry (LC-ESI-IT-TOF-MS/MS) technique. There have been 60 substances (1–60) isolated from Chinese ginseng, including 58 triterpenoids. First-time identifications of substances 1, 2, 7, 15–20, 35, 39, 45–47, 49, 55–57, 59, and 60 were made (Figure ).
Figure 2. The total ion chromatography of Jilin ginseng roots and rhizomes in negative ion detection mode (A) and its corresponding amplified chromatograms (A1, A2, A3) (H. P. Wang et al., Citation2016).
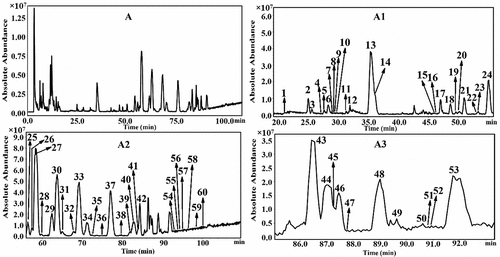
A byproduct of processing ginseng berries, ginseng berry pomace (GBP) includes a variety of bioactive ingredients (ginsenosides and their derivatives). Although GBP has just recently been used as a useful biomaterial, it offers a promising source of bioactive substances. The antioxidant activity of GBP was favorably linked with the phenolics, polar ginsenosides, and fatty acids (O. R. Lee et al., Citation2011). The outcomes showed that while the UHP treatment had larger amounts of fatty acids and sugars, the relative abundance of the main metabolites and few ginsenosides was higher in the control.
A quantitative ginsenoside analysis is crucial for ginseng investigations since each ginsenoside has a distinct medicinal action and metabolic route. Therefore, H. J. Lee et al. (Citation2019) performed a quick, simultaneous, and quantitative analysis of 26 compounds in white and red Panax ginseng using the multiple reaction monitoring (MRM) mode of ultra-high-performance liquid chromatography-tandem mass spectrometry (UHPLC-MS/MS). These compounds included Rb1, Rb2, Rc, Rd, Re, Rf, Rg1, Rg2(R), Rg2(S), Rg3(S).
Through the use of 18 genuine standards of ginsenosides, Yao et al. (Citation2021) performed a study to qualitatively and quantitatively describe the dynamic variations of ginsenosides in ginseng (P. ginseng) pulp steamed at 100 or 120 °C for varied durations (1–6 h). The results of this investigation showed that although levels of the eight polar ginsenosides Rg1, Re, Rb1, Rc, Rb2, Rb3, F1, and Rd decreased, levels of the ten less polar ginsenosides Rf, Rg2, 20(S) Rh1, 20(R)-Rg2, F4, 20(S)-Rg3, 20(R)-Rg3, PPT, Rg5, and 20(R)-Rh2 increased with For the highest total ginsenosides, steaming at 100 °C for one hour was ideal. Rd, a ginsenoside of the protopanaxadiol (PPD) class, is present in relatively small amounts in ginseng. It was shown to be more effective than another protopanaxadiol ginsenoside, Rb1, which is present in ginseng in large quantities. It was possible for Cladosporium fulvum to convert Rb1 to Rd. Purified and described G-I and G-II were recovered from the C. fulvum culture filtrate. Both would be helpful in industrial applications since they could both selectively convert Rb1 to Rd (Gao et al., Citation2010; Zhao, Gao, et al., Citation2009; Zhao, Wang, et al., Citation2009).
4.2. Pharmacological activity
With silico analysis applied by Mathiyalagan et al. (Citation2013), it was found that annotate is the putative sequences followed by the Kyoto Encyclopedia of Genes and Genomes and gene ontology functional; and retrieved 6,226 expressed sequence tags (ESTs) was detected from cDNA libraries of P. ginseng. And those genes are important for growth, pathogenicity, pigments, the ginsenoside pathway, and the formation of ginseng were discovered by the orthologs biochemical and InterProScan protein functional domain analysis.
A potential 346 targets were identified for the predicted 69 conserved miRNAs by searching the ginseng EST database and the predicted target concentrates involved in secondary metabolic processes, responses to biotic and abiotic stress, and transcription regulator activities, as well as a variety of other metabolic processes. By scamming through the ginseng EST database, it was found that a potential 346 targets for the predicted 69 conserved miRNAs. The predicted targets were concentrated in secondary metabolic processes, reactions to biotic and abiotic stress, transcription regulator activities, and a number of other metabolic processes.
A high temperature of 140 °C and a high pressure of 3 kg cm−2 were used to generate high-temperature and pressure-treated red ginseng (HRG). Additionally, the HRG contained more phenolic acids than white ginseng or commercial red ginseng (CRG), such as maltol (62.132 g g−1), p-hydroxybenzoic acid (8.233 g g−1), and syringic acid (69.388 g g−1) (24). Furthermore, by increasing the mRNA expression levels of copper and zinc (Cu/Zn)-SOD and catalyst, the shift in phenolic acids in HRG resulted in the prevention of oxidative stress in C2C12 muscle cells and 3T3-L1 adipocytes (Yoon et al., Citation2012). A mouse model experiment was used to study the effects of HRG on oxidative stress, and the results revealed that levels of glutamic oxaloacetic transaminase, glutamic pyruvic, and transaminase hepatic malondialdehyde were significantly lower in the HRG group than in the exercise groups supplemented with CRG or not supplemented. The HGR group had greater levels of lactate dehydrogenase, glucose-6-phosphate dehydrogenase, and muscle glycogen activity than did the CRG group. Additionally, the HRG treatment group demonstrated increased mRNA expression of muscle regulatory factor 4 and Cu/Zn-SOD. These findings suggested that HRG may enhance exercise capacity and mitigate the oxidative damage brought on by exercise (S. Y. Yu et al., Citation2014).
The bioactivity of one ginsenoside, in particular 20(R)-Rg3, which has a strong anti-cancer impact, has grown dramatically. It was said that Re is the most prevalent polar ginsenoside in fresh ginseng pulp; however, the the effects was significantly reduced. Significantly, following steaming, the number of ginsenoside species rose from 18 to 42, the primary underlying processes for the conversion of polar into less-polar ginsenosides were the demalonylation and acetylation of ginsenosides (Yao et al., Citation2021). At the same time, Yuan et al. (Citation2015) isolated a glycoside hydrolase family 1 enzyme from Cellulosimicrobium cellulans sp. 21; and it was overexpressed and characterized with an eye toward using it to produce minor ginsenosides. They hypothesized that CcBgl1A might be useful for producing the pharmacologically active minor ginsenosides Gyp XVII, CO, Mb, and F2. Chinese traditional medicine uses American ginseng (P. quinquefolium), which is a native of the Northeastern United States. The majority of American ginseng’s pharmacological effects are attributed to its ginsenosides (6). Researchers have looked at how the fungi Fusarium solani and F. oxysporum affect the ginsenosides in American ginseng root’s metabolism. By ingesting them, the harmful Fusarium spp. might lower the concentrations of ginsenoside (Jiao et al., Citation2015).
4.3. Ginseng for human health
Ginseng is thought to inhibit the development and spread of cancer cells by increasing catalase activity, decreasing malondialdehyde levels, and activating glutathione peroxidase and superoxide dismutase to defend against free radical damage. Red ginseng’s non-saponins may enhance memory and learning, while its acidic polysaccharides could boost interferon production (H. Liu et al., Citation2020b; Z. D. Xia et al., Citation2022). D. G. Kim et al. (Citation2018) found that Korean red ginseng extracts increase cell viability by regulating the expression of p53 signaling intermediates, therefore defending cells against peroxynitrite-induced genotoxicity. To boost its effectiveness and preserve it, raw ginseng can be transformed into red or white ginseng. Black ginseng is a new kind of processed ginseng manufactured from white ginseng with nine steaming cycles, which turns the ginseng black in color. Nitric oxide (NO) and reactive oxygen species (ROS) generation, as well as inflammation in both IRE1-dependent and XBP1-independent ways, can all be decreased by black ginseng. Black ginseng has anti-inflammatory and antioxidant properties via the endoplasmic reticulum stress pathway (An et al., Citation2021). In animal models, demonstrated that red ginseng (P. ginseng) extract reduced acute gout inflammation by reducing NLRP3 inflammasomes. Clinical studies have demonstrated that red ginseng pills reduce NLRP3 expression in gout patients. According to several studies, red ginseng may help cure gout in addition to other treatments (J. Lee et al., Citation2020). The anti-tumor activity of ginseng proteins has been studied in the human breast cancer cell line MCF-7, and it was found that these proteins could do so by preventing cell growth and migration, arresting the cell cycle, and inducing apoptosis in MCF-7 cells. These proteins may act through the Bcl-2 and Bax apoptotic pathways (S. Liu et al., Citation2019). To uncover novel anti-UV and whitening agents, 21 fractions of white, red, and black ginseng were isolated, screened, and their antioxidant activities on AAPH- or H2O2-induced damage were examined. F10, an anti-UV filter and whitening cosmetics component, included disaccharide sucrose as its primary active ingredient (Dai et al., Citation2021).
4.4. Ginseng products
Ginseng is a worldwide product and has been sold over 35 different nations. The main places of production are generally China, South Korea, Canada, and the US. Ginseng has been sold in a variety of forms across the world, including fresh, dried, boiling, red ginseng, and etc. In addition to being utilized in nutritional supplements and medicinal supplies, ginseng is consumed as food (Table ). The projected value of the global ginseng market, which includes ginseng root and different processed products, is $2,084 million (Baeg & So, Citation2013). Consumer markets for processed ginseng products are anticipated to keep growing as awareness of complementary medicine and healthful foods spreads throughout the globe.
Table 3. Medicinal ginseng species commonly used world wide
The demand for ginseng products to prevent and treat sickness is rising along with the interest in health concerns, and ginseng’s pharmacological effectiveness and function are still being thoroughly researched. Ginseng is frequently found in a variety of meals (such as beverages, rice cakes, pastries, jellies, salad dressing, etc). (Table ). S. Y. Choi et al. (Citation2012) discovered that various types of coffee beans may be processed with a micro-coating solution comprising ginseng extract generated from a range of ginseng varieties mixed with hydroxypropyl methyl cellulose to create high-quality ginseng coffee (Table ).
Table 4. Concentrations of ginseng coating extracts for coffee
5. Abiotic environmental stress
Heavy metal exposure is a type of abiotic stress that can induce oxidative stress and decrease the quality of the ginseng crop. Siderophore-producing rhizobacteria have the capable to bioremediating HM contamination (Huo et al., Citation2021). When under salt stress, Nuclear Transcription Factor Y (NF-Y) gene family is involved in the regulation of the response to salt stresses in ginseng (M. Liu, Pan, et al., Citation2022). The exogenous putrescine significantly reduced H2O2 production and MDA content by maintaining the balance of osmolytes and antioxidant enzymes, which triggered the protection against cellular damage of ginseng sprouts under salty stress (Islam et al., Citation2021). And in addition, two GPX cDNAs were characterized from the Panax ginseng were elevated strongly by salt stress and chilling stress in ginseng (Y. J. Kim et al., Citation2014).
6. Future outlines
To date, much research has been done on ginseng, but ginseng’s allelopathic mechanisms remain unclear. For example, (i) the role of specific individual allelochemicals is not clear. More in-depth studies, therefore, are required to uncover the mechanisms underlying the role of individual allelochemicals. (ii) The allelopathic molecular mechanism of ginseng may change crop traits through genetic control. Some emerging technologies, such as SWATH-MS, have been used to analyze the upregulation and downregulation of stress proteins in ginseng to identify ginseng’s functional membrane or cell wall proteins and complex signaling pathways, as well as study the molecular mechanisms of ginseng’s allelopathic effects.
Acknowledgments
This work was financially supported by Open Research Fund of Zhejiang Tiantong Forest Ecosystem National Observation and Research Station (TTK201905); Hangzhou West Lake Scenic Area Science and Technology Development Project (2019-008). Kaimei Zhang thanks the China Scholarship Council (CSC) for the support to study abroad.
Disclosure statement
No potential conflict of interest was reported by the authors.
Additional information
Notes on contributors
Kaimei Zhang
Kaimei Zhang, Associate Professor at the College of Biology and the Environment, Nanjing Forestry University, research interests are Botany Science and Allelopathic Effects.
Shengai Zhang
Shengai Zhang, Experimentalist at the School of Health of Binzhou Vocational College, mainly engages in research on health and nutrition.
Atsushi Ebihara
Atsushi Ebihara, Researcher at Department of Botany, National Museum of Nature and Science, research covers Phylogeny of pteridophytes (Hymenophyllaceae), Studies on native gametophytes of pteridophytes, and Biodiversity analyses of the Japanese Archipelago.
Xiaoqi Zhou
Xiaoqi Zhou, Professor at the School of Ecological and Environmental Sciences, East China Normal University, current research focuses on soil carbon and nitrogen cycling and greenhouse gas efflux in terrestrial ecosystems and underlying microbial processes under global change.
Likun Fan
Likun Fan, official at the Qianjiang Management Office of Westlake, research on the biodiversity and plant culture; Pengfei Li, Assistant at the School of Health of Binzhou Vocational College, mainly engages in research on health and nutrition.
Zhuqi Zhang
Zhuqi Zhang, Professor at the College of Bioengineering of Binzhou Vocational College, mainly engaged in research on ginseng plants.
Yuyan Wang
Yuyan Wang, Professor at Agricultural College of Qingdao Xingxing University, mainly engaged in research on medicinal plants.
Yu Shen
Yu Shen, Professor at the College of Biology and the Environment, Nanjing Forestry University, research interests cover Environmental Biology and the application of nanoparticles in agriculture and the environment.
References
- An, M. Y., Lee, S. R., Hwang, H. J., Yoon, J. G., Lee, H. J., & Cho, J. A. (2021). Antioxidant and anti-inflammatory effects of Korean Black ginseng extract through er stress pathway. Antioxidants, 10(1), 62. https://doi.org/10.3390/antiox10010062
- Attele, A. S., Wu, J. A., & Yuan, C. S. (1999). Ginseng pharmacology: Multiple constituents and multiple actions. Biochemical Pharmacology, 58(11), 1685–17. https://doi.org/10.1016/S0006-2952(99)00212-9
- Baeg, I. H., & So, S. H. (2013). The world ginseng market and the ginseng (Korea). Journal of Ginseng Research, 37(1), 1–7. https://doi.org/10.5142/jgr.2013.37.1
- Bae, Y. S., Park, K., & Kim, C. H. (2004). Bacillus spp. as biocontrol agents of root rot and phytophthora blight on ginseng. Plant Pathology Journal, 20(1), 63–66. https://doi.org/10.5423/PPJ.2004.20.1.063
- Chandra, S. P., Vianney, Y. M., Christie, T. L., Wongso, M., Widjaja, M., Yang, D. C., Kang, S. C., Atoum, M. F. M., & Sukweenadhi, J. (2021). Mass Production of Panax ginseng CA Mey. Root Cultures in Indonesia Sarhad Journal of Agriculture, 37(1), 98–109. https://doi.org/10.17582/journal.sja/2021/37.s1.98.109
- Choi, S. Y., Cho, C. W., Lee, Y., Kim, S. S., Lee, S. H., & Kim, K. T. (2012). Comparison of ginsenoside and phenolic ingredient contents in hydroponically-cultivated ginseng leaves, fruits, and roots. Journal of Ginseng Research, 36(4), 425–429. https://doi.org/10.5142/jgr.2012.36.4.425
- Choi, S. Y., Hong, H. D., Bae, H. M., Choi, C., & Kim, K. T. (2011). Phytochemical characteristics of coffee bean treated by coating of ginseng extract. Journal of Ginseng Research, 35(4), 436–441. https://doi.org/10.5142/jgr.2011.35.4.436
- Choi, J., Kim, J., Yoon, H. I., & Son, J. E. (2022). Effect of far-red and UV-B light on the growth and ginsenoside content of ginseng (Panax ginseng CA Meyer) sprouts aeroponically grown in plant factories. Horticulture, Environment, and Biotechnology, 63(1), 77–87. https://doi.org/10.1007/s13580-021-00380-9
- Dai, Y. L., Yang, D., Song, L. H., Yang, H. M., & Yu, J. B. (2021). Low molecular weight oligosaccharide from Panax ginseng C.A. Meyer against UV-mediated apoptosis and inhibits tyrosinase activity in-vitro and in-vivo. Evidence-Based Complementary and Alternative Medicine, 2021, 1–13. https://doi.org/10.1155/2021/8879836
- Fu, X., Shi, D., Qu, C., Zhong, G., Zou, W., & Liu, J. (2014). Anticancer effects of ginseng leaves crude polysaccharides on human hepatoma cell SMMC-7721. Chinese Medicine, 05(2), 87–93. https://doi.org/10.4236/cm.2014.52010
- Gao, J., Zhao, X., Liu, H., Fan, Y., & Cheng, H. (2010). A highly selective ginsenoside Rb1-hydrolyzing β-d-glucosidase from Cladosporium fulvum. Process Biochemistry, 45(6), 897–903. https://doi.org/10.1016/j.procbio.2010.02.016
- Gong, J. T., Li, K. M., Sun, M., Liu, H. J., & Zhang, Z. L. (2016). Allelopathy and soil sickness in continuous cropping of Panax medicinal plants. Allelopathy Journal, 39, 1–17.
- Goodwin, P. H., & Proctor, E. (2019). Molecular techniques to assess genetic variation within and between Panax ginseng and Panax quinquefolius. Fitoterapia, 138, 104343. https://doi.org/10.1016/j.fitote.2019.104343
- Huo, Y., Kang, J. P., Ahn, J. C., Kim, Y. J., Piao, C. H., Yang, D. U., & Yang, D. C. (2021). Siderophore-producing rhizobacteria reduce heavy metal-induced oxidative stress in Panax ginseng Meyer. Journal of Ginseng Research, 45(2), 218–227. https://doi.org/10.1016/j.jgr.2019.12.008
- In, G., Ahn, N. G., Bae, B. S., Lee, M. W., Park, H. W., Jang, K. H., & Kwak, Y. S. (2017). In situ analysis of chemical components induced by steaming between fresh ginseng, steamed ginseng, and red ginseng. Journal of Ginseng Research, 41(3), 361–369. https://doi.org/10.1016/j.jgr.2016.07.004
- In, J. G., Kim, M. K., Lee, O. R., Kim, Y. J., Lee, B. S., Kim, S. Y., & Yang, D. C. (2010). Molecular identification of Korean mountain ginseng using an amplification refractory mutation system (ARMS). Journal of Ginseng Research, 34(1), 41–46. https://doi.org/10.5142/JGR.2010.34.1.041
- Islam, M. J., Ryu, B. R., Azad, M. O. K., Rahman, M. H., Rana, M. S., Lim, J. D., & Lim, Y. S. (2021). Exogenous putrescine enhances salt tolerance and ginsenosides content in Korean ginseng (Panax ginseng Meyer) sprouts. Plants, 10(7), 10. https://doi.org/10.3390/plants10071313
- Jang, W., Jang, Y., Cho, W., Lee, S. H., Shim, H., Park, J. Y., Xu, J., Shen, X., Liao, B., Jo, I. H., & Kim, Y. C. (2022). High-throughput digital genotyping tools for Panax ginseng based on diversity among 44 complete plastid genomes. Plant Breeding and Biotechnology, 10(3), 174–185. https://doi.org/10.9787/PBB.2022.10.3.174
- Jiao, X. L., Lu, X. H., Chen, A. J., Luo, Y., Hao, J. J., & Gao, W. W. (2015). Effects of Fusarium solani and F. oxysporum infection on the metabolism of ginsenosides in American ginseng roots. Molecules, 20(6), 10535–10552. https://doi.org/10.3390/molecules200610535
- Jiao, X. L., Zhang, X. S., Lu, X. H., Qin, R., Bi, Y. M., & Gao, W. W. (2019). Effects of maize rotation on the physicochemical properties and microbial communities of American ginseng cultivated soil. Scientific Reports, 9(1), 1–12. https://doi.org/10.1038/s41598-019-44530-7
- Kang, J. P., Huo, Y., Yang, D. U., & Yang, D. C. (2021). Influence of the plant growth promoting Rhizobium panacihumi on aluminum resistance in Panax ginseng. Journal of Ginseng Research, 45(3), 442–449. https://doi.org/10.1016/j.jgr.2020.01.001
- Kan, H., Qu, S., Dong, K., Wang, S., Xu, C., Wang, Y., & Hua, S. (2023). Integrated metabolome and transcriptome analysis unveils the underlying molecular response of Panax ginseng plants to the phytophthora cactorum infection. Agriculture, 13(2), 509. https://doi.org/10.3390/agriculture13020509
- Kawakatsu, T., & Fukuda, N. (2023). Dense planting and environmental control (temperature, light intensity, and concentration of nutrient solution) can increase the yield of ginseng (Panax ginseng CA Meyer) seedlings in indoor cultivation with artificial light. Horticulture, Environment, and Biotechnology, 1–12. https://doi.org/10.1007/s13580-022-00506-7
- Kim, N. Y., & Han, M. J. (2005). Development of ginseng yogurt fermented by Bifidobacterium spp. Korean Journal of Food Cookery Science, 221, 575–584.
- Kim, K., Huh, J. H., Um, Y., Jeon, K. S., & Kim, H. J. (2020). The comparative of growth characteristics and ginsenoside contents in wild-simulated ginseng (Panax ginseng) on different years by soil properties of cultivation regions. Korean Journal of Plant Reources, 33, 651–658.
- Kim, Y. J., Jang, M. G., Noh, H. Y., Lee, H. J., Sukweenadhi, J., Kim, J. H., Kim, S. Y., Kwon, W. S., & Yang, D. C. (2014). Molecular characterization of two glutathione peroxidase genes of Panax ginseng and their expression analysis against environmental stresses. Gene, 535(1), 33–41. https://doi.org/10.1016/j.gene.2013.10.071
- Kim, B. B., Jeong, J. H., Jung, S. J., Yun, D. W., Yoon, E. S., & Choi, Y. E. (2005). Authentication of Korean Panax ginseng from Chinese Panax ginseng and Panax quinquefolius by AFLP analysis. Journal of Plant Biotechnology, 7(2), 81–86.
- Kim, Y. J., Joo, S. C., Shi, J., Hu, C., Quan, S., Hu, J., & Zhang, D. (2018). Metabolic dynamics and physiological adaptation of Panax ginseng during development. Plant Cell Reports, 37(3), 393–410. https://doi.org/10.1007/s00299-017-2236-7
- Kim, D. G., Shin, J. H., & Kang, M. J. (2018). Physiochemical Characteristics of Panax ginseng C. A Meyer sprout cultivated with nanobubble water and antioxidant activities of enzymatic hydrolysates. Journal of Agriculture & Life Science, 52(4), 109–120. https://doi.org/10.14397/jals.2018.52.4.109
- Kim, Y. J., Sukweenadhi, J., Seok, J. W., Kang, C. H., Choi, E. S., Subramaniyam, S., & Yang, D. C. (2017). Complete genome sequence of Paenibacillus yonginensis DCY84 T, a novel plant symbiont that promotes growth via induced systemic resistance. Standards in Genomic Sciences, 12(1), 1–7. https://doi.org/10.1186/s40793-017-0277-8
- Lee, J., Hong, S. M., Park, N., Lee, J., Jang, S. G., Cho, M. L., & Park, S. H. (2020). Red ginseng extracts as an adjunctive therapeutic for gout: Preclinical and clinical evidence. Food and Agricultural Immunology, 32(1), 1–14. https://doi.org/10.1080/09540105.2020.1854189
- Lee, H. J., Jeong, J., Alves, A. C., Han, S. T., In, G., Kim, E. H., & Hong, Y. S. (2019). Metabolomic understanding of intrinsic physiology in Panax ginseng during whole growing seasons. Journal of Ginseng Research, 43(4), 654–665. https://doi.org/10.1016/j.jgr.2019.04.004
- Lee, M. H., Lee, Y. C., Kim, S. S., Hong, H. D., & Kim, K. T. (2015). Quality and antioxidant activity of ginseng seed processed by fermentation strains. Journal of Ginseng Research, 39(2), 178–182. https://doi.org/10.1016/j.jgr.2014.10.007
- Lee, O. R., Sathiyaraj, G., Kim, Y. J., In, J. G., Kwon, W. S., Kim, J. H., & Yang, D. C. (2011). Defense genes induced by pathogens and abiotic stresses in Panax ginseng C.A. Meyer. Journal of Ginseng Research, 35(1), 1–11. https://doi.org/10.5142/jgr.2011.35.1.001
- Lee, S. W., Seong, N., Kang, S., Hyun, D., Chang, K. Y., Cha, S., & Hyun, G. S. (2004). Comparison of growth characteristics and quality of ginseng (Panax ginseng) grown under upland and paddy field. Korean Journal of Crop Science, 49, 389–393.
- Le, K. C., Ho, T. T., Lee, J. D., Paek, K. Y., & Park, S. Y. (2020). Colchicine mutagenesis from long-term cultured adventitious roots increases biomass and ginsenoside production in wild ginseng (Panax ginseng Mayer). Agronomy, 10(6), 785. https://doi.org/10.3390/agronomy10060785
- Le, K. C., Jeong, C. S., Lee, H., Paek, K. Y., & Park, S. Y. (2019). Ginsenoside accumulation profiles in long-and short-term cell suspension and adventitious root cultures in Panax ginseng. Horticulture, Environment, and Biotechnology, 60(1), 125–134. https://doi.org/10.1007/s13580-018-0108-x
- Li, M. (2008). Effect of ginseng root exudates on rhizospheric soil microecology. Department of Environmental Engineering, Master degree, Jilin Agricultural University.
- Li, Z. (2018). Allelopathy of autotoxic compounds and mitigation method for ginseng continuous cropping obstacle. Department of Plant Pathology, Doctor of Philosophy,Shenyang Agricultural University (Chinese).
- Li, G., Cui, Y., Wang, H., Kwon, W. S., & Yang, D. C. (2017). Molecular differentiation of Russian wild ginseng using mitochondrial nad7 intron 3 regions. Journal of Ginseng Research, 41(3), 326–329. https://doi.org/10.1016/j.jgr.2016.06.003
- Li, T., Kim, J. H., Jung, B., Ji, S., Seo, M. W., Han, Y. K., Lee, S. W., Bae, Y. S., Choi, H. G., Lee, S. H., & Lee, J. (2020). Transcriptome analyses of the ginseng root rot pathogens cylindrocarpon destructans and Fusarium solani to identify radicicol resistance mechanisms. Journal of Ginseng Research, 44(1), 161–167. https://doi.org/10.1016/j.jgr.2018.11.005
- Li, H., Lee, J. H., & Ha, J. M. (2008). Effective purification of ginsenosides from cultured wild ginseng roots, red ginseng, and white ginseng with macroporous resins. Journal of MicroBiologyogy and Biotechnology, 18(11), 1789–1791. https://doi.org/10.4014/jmb.0800.192
- Liu, M., Cai, Y., Li, F., Wu, Y., Zhang, M., Wang, Y., Fan, M., Li, B., Huang, X., Zheng, F., & Yue, H. (2022). Metabolomics and network pharmacological analysis to explore the mechanism of ginseng treatment for spleen‐qi deficiency. Journal of Separation Science, 45(24), 4427–4438. https://doi.org/10.1002/jssc.202200457
- Liu, S., Chen, C., Wan, X., Jin, X., Ren, Y., Xiu, Y., & Li, Y. (2019). Antitumor effects and mechanism of protein from Panax ginseng on human breast cancer cell line MCF-7. Pharmacognosy Magazine, 15(65), 715. https://doi.org/10.4103/pm.pm_151_19
- Liu, J., Liu, Y., Wang, Y., Abozeid, A., Zu, Y. G., Zhang, X. N., & Tang, Z. H. (2017). GC-MS metabolomic analysis to reveal the metabolites and biological pathways involved in the developmental stages and tissue response of Panax ginseng. Molecule, 22(3), 496. https://doi.org/10.3390/molecules22030496
- Liu, H., Lu, X., Hu, Y., & Fan, X. (2020a). Chemical constituents of Panax ginseng and Panax notoginseng explain why they differ in therapeutic efficacy. Pharmacological Research, 161, 105263. https://doi.org/10.1016/j.phrs.2020.105263
- Liu, H., Lu, X., Hu, Y., & Fan, X. (2020b). Chemical constituents of Panax ginseng and Panax notoginseng explain why they differ in therapeutic efficacy. Pharmacological Research, 161, 105263. https://doi.org/10.1016/j.phrs.2020.105263
- Liu, M., Pan, Z., Yu, J., Zhu, L., Zhao, M., Wang, Y., Chen, P., Liu, C., Hu, J., Liu, T., Wang, K., Wang, Y., & Zhang, M. (2022). Transcriptome-wide characterization, evolutionary analysis, and expression pattern analysis of the NF-Y transcription factor gene family and salt stress response in Panax ginseng. BMC Plant Biology, 22(1), 1–11. https://doi.org/10.1186/s12870-022-03687-6
- Liu, H. J., Yang, X. Y., Miao, Z. Q., Li, S. D., Chen, Y. H., Liu, G., & Zhang, Z. L. (2018). Characteristics of soil microflora of Panax notoginseng in different continuous cropping years. Allelopathy Journal, 44(2), 145–158. https://doi.org/10.26651/allelo.j./2018-44-2-1160
- Li, L., Wang, Y., Xiu, Y., & Liu, S. (2018). Chemical differentiation and quantitative analysis of different types of panax genus stem-leaf based on a UPLC-Q-exactive orbitrap/MS combined with multivariate statistical analysis approach. Journal of Analytical Methods in Chemistry, 2018, 16. https://doi.org/10.1155/2018/9598672
- Li, Q., Zhang, L., Guan, T., Xu, Y., & Chen, C. (2020). Allelopathic effects of ginsenoside Rgl on seed germination and seedling growth of Panax ginseng. Allelopathy Journal, 49(2), 229–242. https://doi.org/10.26651/allelo.j/2020-49-2-1267
- Lu, X. H., Jiao, X. L., Hao, J. J., Chen, A. J., & Gao, W. W. (2015). Characterization of resistance to multiple fungicides in Botrytis cinerea populations from Asian ginseng in northeastern China. European Journal of Plant Pathology, 144(3), 467–476. https://doi.org/10.1007/s10658-015-0786-5
- Luo, L., Zhang, J., Ye, C., Li, S., Duan, S., Wang, Z., Huang, H., Liu, Y., Deng, W., Mei, X., He, X., Yang, M., & Zhu, S. (2022). Foliar pathogen infection manipulates soil health through root exudate-modified rhizosphere microbiome. Microbiology Spectrum, 10(6), e02418–22. https://doi.org/10.1128/spectrum.02418-22
- Lu, X. H., Zhang, X. M., Jiao, X. L., Hao, J. J., Zhang, X. S., Luo, Y., & Gao, W. W. (2020). Taxonomy of fungal complex causing red-skin root of Panax ginseng in China. Journal of Ginseng Research, 44(3), 506–518. https://doi.org/10.1016/j.jgr.2019.01.006
- Mathiyalagan, R., Subramaniyam, S., Natarajan, S., Kim, Y. J., Sun, M. S., Kim, S. Y., & Yang, D. C. (2013). Insilico profiling of microRnas in Korean ginseng (Panax ginseng). Journal of Ginseng Research, 37(2), 227. https://doi.org/10.5142/jgr.2013.37.227
- Natalie, K., Chandra, S. P., Christanti, P., Hak, K. J., Yang, D. C., & Sukweenadhi, J. Influence of volume medium on growth and ginsenoside level in adventitious root culture of Panax ginseng CA Meyer. (2022). IOP Conference Series: Earth and Environmental Science, 1083(1), 012090. IOP Publishing. https://doi.org/10.1088/1755-1315/1083/1/012090
- Park, Y. J., Hwang, U., Park, S., Sim, S., Jeong, S., Park, M., & Suh, H. J. (2021). Optimal bioconversion for compound K production from red ginseng root by sequential enzymatic hydrolysis and its characteristics. Applied Biological Chemistry, 64(1), 1–11. https://doi.org/10.1186/s13765-020-00587-x
- Park, Y. H., Kim, J. U., Kim, D. H., Son, Y. K., Yun, J. H., Moon, H. P., & Cho, S. Y. (2016). Current status of ginseng cultivation and soil characteristics of northeastern three provinces in China. Korean Journal of Soil Science and Fertilizer, 49(6), 795–806. https://doi.org/10.7745/KJSSF.2016.49.6.795
- Park, Y. H., Mishra, R. C., Yoon, S., Kim, H., Park, C., Seo, S. T., & Bae, H. (2019). Endophytic trichoderma citrinoviride isolated from mountain-cultivated ginseng (Panax ginseng) has great potential as a biocontrol agent against ginseng pathogens. Journal of Ginseng Research, 43(3), 408–420. https://doi.org/10.1016/j.jgr.2018.03.002
- Putiri, A. L., Close, J. R., Lilly, H. R., Guillaume, N., & Sun, G. C. (2017). Qigong exercises for the management of type 2 diabetes mellitus. Medicines, 4(3), 59. https://doi.org/10.3390/medicines4030059
- Ratan, Z. A., Haidere, M. F., Hong, Y. H., Park, S. H., Lee, J. O., Lee, J., & Cho, J. Y. (2021). Pharmacological potential of ginseng and its major component ginsenosides. Journal of Ginseng Research, 45(2), 199–210. https://doi.org/10.1016/j.jgr.2020.02.004
- Siddiqi, M. H., Siddiqi, M. Z., Ahn, S., Kang, S., Kim, Y. J., Sathishkumar, N., & Yang, D. C. (2013). Ginseng saponins and the treatment of osteoporosis: Mini literature review. Journal of Ginseng Research, 37(3), 261. https://doi.org/10.5142/jgr.2013.37.261
- So, S. H., Lee, J. W., Kim, Y. S., Hyun, S. H., & Han, C. K. (2018). Red ginseng monograph. Journal of Ginseng Research, 42(4), 549–561. https://doi.org/10.1016/j.jgr.2018.05.002
- Song, J. S., Jung, S., Jee, S., Yoon, J. W., Byeon, Y. S., Park, S., & Kim, S. B. (2021). Growth and bioactive phytochemicals of Panax ginseng sprouts grown in an aeroponic system using plasma-treated water as the nitrogen source. Scientific Reports, 11(1), 1–10. https://doi.org/10.1038/s41598-021-82487-8
- Sukweenadhi, J., Balusamy, S. R., Kim, Y. J., Lee, C. H., Kim, Y. J., Koh, S. C., & Yang, D. C. (2018). A growth-promoting bacteria, paenibacillus yonginensis DCY84T enhanced salt stress tolerance by activating defense-related systems in Panax ginseng. Frontiers in Plant Science, 9, 813. https://doi.org/10.3389/fpls.2018.00813
- Sun, H., Lee, O. R., Kim, Y. J., Jeong, S. K., In, J. G., Kwon, W. S., & Yang, D. C. (2010). Identification of ’Chunpoong’ among Panax ginseng cultivars using real time PCR and SNP marker. Journal of Ginseng Research, 34(1), 47–50. https://doi.org/10.5142/JGR.2010.34.1.047
- Tan, Y., Cui, Y., Li, H., Kuang, A., Li, X., Wei, Y., & Ji, X. (2017). Diversity and composition of rhizospheric soil and root endogenous bacteria in Panax notoginseng during continuous cropping practices. Journal of Basic Microbiology, 57(4), 337–344. https://doi.org/10.1002/jobm.201600464
- Tian, G. L., Bi, Y. M., Jiao, X. L., Zhang, X. M., Li, J. F., Niu, F. B., & Gao, W. W. (2021). Application of vermicompost and biochar suppresses fusarium root rot of replanted American ginseng. Applied Microbiology and Biotechnology, 105(18), 6977–6991. https://doi.org/10.1007/s00253-021-11464-y
- Tian, L., Shi, S., Ma, L., Zhou, X., Luo, S., Zhang, J., & Tian, C. (2019). The effect of glomus intraradices on the physiological properties of Panax ginseng and on rhizospheric microbial diversity. Journal of Ginseng Research, 43(1), 77–85. https://doi.org/10.1016/j.jgr.2017.08.005
- Tong, A. Z., Liu, W., Liu, Q., Xia, G. Q., & Zhu, J. Y. (2021). Diversity and composition of the Panax ginseng rhizosphere microbiome in various cultivation modesand ages. BMC microBiologyogy, 21(1), 1–13. https://doi.org/10.1186/s12866-020-02081-2
- Wang, J., Chen, H., Gao, J., Guo, J., Zhao, X., & Zhou, Y. (2018). Ginsenosides and ginsenosidases in the pathoBiologyogy of ginseng-Cylindrocarpon destructans. Plant Physiology & Biochemistry, 123, 406–413. https://doi.org/10.1016/j.plaphy.2017.12.038
- Wang, S. H., Liang, W. X., Lu, J., Yao, L., Wang, J., & Gao, W. Y. (2020). Penicillium sp. YJM-2013 induces ginsenosides biosynthesis in Panax ginseng adventitious roots by inducing plant resistance responses. Chinese Herbal Medicines, 12(3), 257–264. https://doi.org/10.1016/j.chmed.2020.02.003
- Wang, Q., Sun, H., Xu, C., Ma, L., Li, M., Shao, C., Guan, Y., Liu, N., Liu, Z., Zhang, S., & Zhang, L. (2019). Analysis of rhizosphere bacterial and fungal communities associated with rusty root disease of Panax ginseng. Applied Soil Ecology, 138, 245–252. https://doi.org/10.1016/j.apsoil.2019.03.012
- Wang, H. P., Zhang, Y. B., Yang, X. W., Yang, X. B., Xu, W., Xu, F., & Zhang, L. X. (2016). High-performance liquid chromatography with diode array detector and electrospray ionization ion trap time-of-flight tandem mass spectrometry to evaluate ginseng roots and rhizomes from different regions. Molecules, 21(5), 603. https://doi.org/10.3390/molecules21050603
- Wee, J. J., Kim, Y. S., Kyung, J. S., Song, Y. B., KDC, D. J. H., & Lee, S. D. (2010). Identification of anticoagulant components in Korean red ginseng. Journal of Ginseng Research, 34(4), 355–362. https://doi.org/10.5142/jgr.2010.34.4.355
- Wu, W., Song, F., Guo, D., Mi, J., Qin, Q., Yu, Q., & Liu, S. (2012). Mass spectrometry-based approach in ginseng research: A promising way to metabolomics. Current Analytical Chemistry, 8(1), 43–66. https://doi.org/10.2174/157341112798472189
- Xia, Z. D., Sun, B., Wen, J. F., Ma, R. X., Wang, F. Y., Wang, Y. Q., Li, Z. H., Jia, P., & Zheng, X. H. (2022). Research progress on metabolomics in the quality evaluation and clinical study of Panax ginseng. Biomedical Chromatography, e5546. https://doi.org/10.1002/bmc.5546
- Xia, S., Yan, H., Li, R., & Gao, Y. (2000). Occurrence types and control suggestions of ginseng root diseases. Special Wild Economic Animal & Plant Research, 2, 60–62.
- Yao, F., Li, X., Sun, J., Cao, X., Liu, M., Li, Y., & Liu, Y. (2021). Thermal transformation of polar into less-polar ginsenosides through demalonylation and deglycosylation in extracts from ginseng pulp. Scientific Reports, 11(1), 1–11. https://doi.org/10.1038/s41598-021-81079-w
- Yoon, B. R., Lee, Y. J., Hong, H. D., Lee, Y. C., & Lee, O. H. (2012). Inhibitory effects of Panax ginseng treated with high temperature and high pressure on oxidative stress. The Korean Journal of Food and Nutrition, 25(4), 800–806. https://doi.org/10.9799/ksfan.2012.25.4.800
- Yuan, Y., Hu, Y. B., Hu, C. X., Leng, J. Y., Chen, H. L., Zhao, X. S., & Zhou, Y. F. (2015). Overexpression and characterization of a glycoside hydrolase family 1 enzyme from Cellulosimicrobium cellulans sp 21 and its application for minor ginsenosides production. Journal of Molecular Catalysis B-Enzymatic, 120, 60–67. https://doi.org/10.1016/j.molcatb.2015.06.015
- Yu, S. Y., Yoon, B. R., Lee, Y. J., Lee, J. S., Hong, H. D., Lee, Y. C., & Lee, O. H. (2014). Inhibitory effect of high temperature- and high pressure-treated red ginseng on exercise-induced oxidative stress in ICR mouse. Nutrients, 6(3), 1003–1015. https://doi.org/10.3390/nu6031003
- Yu, H., Zhao, J., You, J., Li, J., Ma, H., & Chen, X. (2019). Factors influencing cultivated ginseng (Panax ginseng CA Meyer) bioactive compounds. PLos One, 14(10), e0223763. https://doi.org/10.1371/journal.pone.0223763
- Zhao, X., Gao, J., Song, C., Fang, Q., Wang, N., Zhao, T., & Zhou, Y. (2012). Fungal sensitivity to and enzymatic deglycosylation of ginsenosides. Phytochemistry, 78, 65–71. https://doi.org/10.1016/j.phytochem.2012.02.027
- Zhao, X., Gao, L., Wang, J., Bi, H., Gao, J., Du, X., & Tai, G. (2009). A novel ginsenoside Rb-1-hydrolyzing beta-D-glucosidase from cladosporium fulvum. Process Biochemistry, 44(6), 612–618. https://doi.org/10.1016/j.procbio.2009.01.016
- Zhao, X., Wang, J., Li, J., Fu, L., Gao, J., Du, X., & Tai, G. (2009). Highly selective biotransformation of ginsenoside Rb1 to Rd by the phytopathogenic fungus cladosporium fulvum (syn. Fulvia fulva). Journal of Industrial Microbiology & Biotechnology, 36(5), 721–726. https://doi.org/10.1007/s10295-009-0542-y