Abstract
The benefits that Trichoderma species provide to plants are numerous and have already been extensively studied. However, the information about the intervention of some factors in the association between fungi and plants is limited. Therefore, this study evaluated the impact of ten isolates of Trichoderma longibrachiatum endophytes and epiphytes added to hydrogel substrate and inoculated on banana plants in vitro for acclimatization. The experiment was conducted using a completely randomize design and the effect of the treatments was assessed with an analysis of variance (ANOVA), and a Tukey’s range test. Then, the relationship between the persistence of spores of endophytic fungi in the substrate with hydrogel and endophytism was determined by a Pearson correlation. The results indicated significant differences in endophytism, persistence, root, plant length, and fresh weight (P < 0.05). Treatment O III 3 M 3 with a previous epiphytic lifestyle had (55.5%) endophytism, followed by O I 3.2 and V IV 2.1 with (44.4%) which had a previous endophytic lifestyle, the latter also had higher persistence, however, endophytic lifestyle did not influence persistence (r = 0.5, r2 = 0.2). All hydrogel isolates promoted root length as opposed to applying hydrogel alone, but isolate VIV2.1, which had higher persistence and root length, and a high percentage of endophytism, did not affect plant length, stem diameter, number of leaves, and fresh weight. A combination of hydrogel and T. longibrachiatum, which can grow inside or outside banana plant tissues, can provide positive effects on acclimatization.
1. Introduction
Due to the numerous advantages it offers, micropropagation has become increasingly relevant in modern production systems (Chandran et al., Citation2020; Lee et al., Citation2019; Twaij et al., Citation2020). These benefits include the production of higher-quality plants, the cultivation of disease-free specimens, and the ability to rapidly multiply plants on a large scale within a relatively short timeframe. Additionally, micropropagation contributes to the growth and conservation of plants that are otherwise challenging to propagate (Hussain et al., Citation2012; Lee et al., Citation2019). However, despite the several advantages of this plant production method over traditional ones, it also comes with disadvantages. The primary challenge lies in reducing mortality during the transplanting process to ex vitro conditions, which serves as a limiting factor for successful production (Salgado Pirata et al., Citation2022). According to Galan et al. (Citation2018) and Hazarika (Citation2003), micropropagated banana seedlings have been reported to experience up to 60% mortality. This high mortality rate is attributed to the difficulty plants have in adapting to different light levels, temperature fluctuations, substrate used, and nutrition. These factors can cause plant stress that eventually leads to shock and death. However, endophytic fungi play a crucial role in aiding plant adaptation and nutrition, providing significant benefits. They establish mutualistic relationships within the soil where they develop (Alam et al., Citation2021). Endophytic fungi are defined as a microorganism that spends most or all of their life cycle colonizing host plant tissues without causing damage (Galindo-Solís & Fernández, Citation2022; Schouten, Citation2019). One of the fungi most commonly found endophytic associated with plants is the genus Trichoderma, which is known to provide several benefits to the host plant such as; preventing pathogenic colonization (Sudha et al., Citation2016), stimulating stem growth and promoting root development, disease resistance, as well as secondary metabolites that inhibit a specific pathogen or other endophytic fungi that are usually competitors for space and nutrients, inducing transcriptomic changes (Tseng et al., Citation2020; Tyśkiewicz et al., Citation2022). At the same time, this fungus can solubilize phosphate, improve phosphorus (P) uptake, nitrogen (N) fixation, and hormone production such as auxin, abscisins, ethylene, gibberellins, and indole-acetic acid (IAA), which are important for plant growth regulation, as well as improving resistance to environmental stress and nutrient recycling (Firáková et al., Citation2007).
These attributes are directly associated with the adaptation of banana plants from in vitro to ex vitro conditions, where evapotranspiration occurs, leading to water stress and wilting (Irsyadi, Citation2021). In this regard, it has been documented that Trichoderma viride and Pseudomonas fluorescens establish a positive interaction with the plant in vitro by improving its stress tolerance capacity and protecting it from shock, even in the biotization of plants in vitro, Trichoderma asperellum enhances growth and tolerance to pathogens and plant metabolites, in addition to inducing resistance in ex vitro conditions (Castro-Restrepo et al., Citation2022; Gundrathi & Babu, Citation2019). Although the benefits of Trichoderma have been well-documented, it is crucial to conduct research aimed at optimizing plant acclimatization in regions with limited rainfall and irrigation water resources. According to Report MX2021–0031, published by the United States Department of Agriculture (USDA), water stress in Mexico has increased by 85 percent across the country, affecting ten states that collectively represent 60–70 percent of the Mexican total agricultural output. In this context, the combination of novel strategies to mitigate water stress and provide benefits to both plants and beneficial fungi populations is paramount. This may involve the use of different Trichoderma species in combination with hydrogels as an innovative approach to address this pressing issue. Hydrogels are polymeric materials with a three-dimensional (3D) network structure that possess hydrophilic polymeric chains, as well as an ability to absorb and retain a large volume of water in its interstitial structures (Chai et al., Citation2017; Husain et al., Citation2018). The use of hydrogels as an encapsulation and transport method within pharmaceutical, biological, and agrochemical industries is steadily increasing. Some reports were published by Ortega-Torres et al. (Citation2020), who tested the efficacy of the hydrogel known as potassium polyacrylate on cucumber crops and observed yields comparable to those achieved with conventional irrigation. Potassium polyacrylate, an extremely absorbent polymer for water and other aqueous solutions (Huang et al., Citation2018; Montesano et al., Citation2015), has been observed to enhance tobacco growth. Its usage results in increased plant weight, stem diameter, greater leaf numbers, and expanded leaf area. This improvement is attributed to its ability to enhance water retention properties in substrates and alleviate water stress (Huang et al., Citation2018). Therefore, we hypothesize that hydrogel and endophytic fungal strains can significantly improve vegetative and root development in ex vitro banana plants during the acclimatization process. This stage is crucial for developing sufficient roots that allow better adaptation and survival (Tyśkiewicz et al., Citation2022). In this sense, the aim was to promote root, stem, and leaf growth in banana plants ex vitro by applying an entophytic and epiphytic Trichoderma longibrachiatum, along with potassium polyacrylate hydrogel in the substrate during the acclimatization of banana plants while maintaining the endophytic nature of Trichoderma longibrachiatum and its persistence.
2. Materials and methods
The experiment was conducted in two locations. One of them is situated in the locality “El Jobo”, Tlapacoyan, Veracruz state, Mexico, at 20°0’38.72” N latitude and 97°9´56.55” W longitude, characterized by a warm semi-humid environment. The other location is the Facultad de Ciencias Agrícolas y Pecuarias, Teziutlán, Puebla state, Mexico, situated at 19°52’34.24” N latitude and 97°21’ 38.77” W longitude, with a warm, humid temperate environment. At both locations, experiments were conducted from December 2021 to March 2022 and from June to September 2022.
2.1. Elements used in the experiment
2.1.1. Trichoderma isolates
3 isolates of endophytic and 7 of non-endophytic origins of T. longibrachiatum (Table ) obtained from the previous studies by Ramírez-Torres et al. (Citation2022) and stored in the fungal collection of the Laboratorio de Biología Molecular of the Facultad de Ciencias Agrícolas y Pecuarias belonging to the Benemérita Universidad Autónoma de Puebla, were used.
Table 1. Description of treatments formed with hydrogel and T. longibrachiatum (1 X 105 spores per mL)
2.1.2. Banana plants
144 in vitro banana plants of the oaxaqueño variety were obtained from the Nature Source Improved Plants (NSPI) laboratory at nine weeks of age, 4.5 to 5.5 centimeters in height, 0.4 centimeters in stem diameter, and three leaves on each plant.
2.1.3. Transplanting substrate
For the substrate preparation, perlite, loam, and sterilized peat moss were mixed at a ratio of 3:1:3 on a volume basis, placed on sterile white plastic strips of 60 × 60 cm with a scoop shovel according to Ramírez-Torres et al. (Citation2022); Rivera et al. (Citation2021) and for each 1.92 kg of the mixture, 18 grams of hydrogel (Silos de agüa S.A. de C.V.) previously hydrated with 100 mL of sterile distilled water was added for each gram of hydrogel. The hydrated hydrogel was added to the substrate and 250 mL of 0.03 % Tween 80 solution was added with a concentration of 1 X 105 spores per mL of each treatment (Table ) and was manually inoculated through a sprayer. Finally, 12 black 500-gauge 8 × 15 cm bags for each treatment were filled with 160 g of the mixture of the substrate, hydrogel, and endophytic fungal spores.
2.2. Experimental conditions
For the experiment, 12 treatments were created. These treatments included 10 with the isolate combined with hydrogel, one with hydrogel alone, and one with sterile distilled water (Table ). Each treatment was replicated four times, with each experimental unit consisting of 3 plants that exhibited similar characteristics. In total, there were 12 plants per treatment. These plants were transplanted from the in vitro culture medium, washed with tap water, and then submerged in a 0.03% Tween 80 solution with 1 × 105 spores per mL for 5 minutes. Subsequently, the plants were planted in black bags previously filled with substrate inoculated with each of the treatments. The distribution of these bags followed a completely randomized design within a microtunnel. The microtunnel had a steel structure measuring 1 × 3 meters in length and an elevation of 0.9 meters. It was covered with white polyethylene plastic, and a shade net was placed over it to maintain a luminosity level of 35%.
During the two months of the experiment, temperature inside and outside the microtunnel was recorded with a datalogger (Tzone, model: TempU 03), and 3 daily irrigations were applied to plants through nebulizers (10:00 am, 01:00 pm, and 04:00 pm) with a time of 1 min each irrigation to avoid hydric stress. Two fertilizations were made, first at 26 days and second at 34 days after transplanting, applying 10 mL of NPK 20-20-20 fertilizer at a rate of 144 g per 1.44 L of water at the plant base.
2.3. Treatment evaluation
After two months the following variables were evaluated:
Root length, plant length, root diameter, plant fresh weight, and leaves number: Length of the main root (LR) from the stem base to the tip, root diameter from the longitudinal middle part (DR), plant length (LP) from the top to the base of the stem, the fresh plant weight (FPW) expressed in grams was obtained with a granatary balance, and the leaves number (LN) was counted.
Trend in endophytism of fungi used in the experiment. This variable was measured to know the trend in the lifestyle of the isolates. To determine this, the methodology of dos Reis, Citation2022 was followed for each treatment. In brief, 1 g of the main root was taken and cut into 10 pieces of 1 cm length and washed with 1% sodium hypochlorite for 30 seconds, rinsed in sterile water for 5 minutes, and dried with sterile paper towels. The pieces were placed on Potato Dextrose Agar (PDA, brand: BD Bioxon) culture medium with streptomycin and chloramphenicol at doses of 50 μL L−1 of medium to prevent bacterial growth. Then Petri dishes were incubated at 32 ºC ±2 and after 24 hours the external growth in each piece of tissue was checked after 4 days. For this, fungus pieces were taken and observed with a Leica DM75® composite microscope according to Subhashinia et al. (Citation2016); identity was determined by taxonomic keys described by Barnett and Hunter (Citation1998). Additionally, cuts were made in the root tissue corresponding to the pieces in which Trichoderma growth was detected, and the presence or absence of internal mycelium was observed.
Persistence of fungal spores on the substrate with hydrogel: To evaluate the persistence rate of spores, after two months, the methodology described by (Goettel & Douglas, Citation1996) was followed, consisting of two experimental units randomly chosen in each treatment; further, a sample of 10 g of soil was taken from each unit, and then diluted in 90 ml of 0.05% Tween 80.05 %, the solution obtained was shaken in Vortex a MaxiMix™ I Thermo Scientific™ for 1 min and from it 100 μL were taken and spread with a sterile glass triangle on the surface of Potato Dextrose Agar (PDA, brand: BD Bioxon) with streptomycin and chloramphenicol at a dose of 50 μL L−1 of medium. Each Petri dish was incubated at 32 ºC ±2 for 120 h, and the growth area obtained from each Colony Forming Unit (CFU) was calculated using Image J software for Windows.
2.4. Statistical analysis
For data analysis, assumptions of homogeneity and normality were checked, for values where the assumptions were not obtained, a Box-Cox transformation was performed, and subsequently, the effect of the treatments was determined with a variance analysis (ANOVA), and a comparison of means by Tukey’s test at a significance level of 0.05, using the SAS program version 9.0 for Windows. For the data concerning colonization of endophytic fungal strains in ex vitro banana seedlings treated with hydrogel, in addition to checking the assumptions of homogeneity and normality, a Kruskal-Wallis test was performed using PROC NPAR1WAY WILCOXON (Wilcoxon signed-rank test with a significance level of 0.05). Finally, the relationship between the persistence of spores of endophytic fungi in the substrate with hydrogel and endophytism was determined by a Pearson correlation analysis.
3. Results
3.1. Root length, root diameter, plant length, plant fresh weight, and number of leaves
A significant difference was observed (P ≤ 0.05) and according to the Tukey test, the treatment that promoted better root length was the isolate VIV2.1 with a hydrogel which had endophytic behavior, unlike the control and only hydrogel applied (Table ). The effect of the isolate O I 5 M 3 + hydrogel (4) was observed to promote greater plant length than the other treatments including the control and only hydrogel, however, no synergic effect of Trichoderma plus hydrogel was observed in plant diameter or the effect of the treatment P IV 5 R O + hydrogel (10) and control was statistically different (Table ), The diameter of the plants treated with O I 5 M 3 was shorter than control plants diameter, while plants treated only with hydrogel, suggesting that diameter growth was not affected by the treatment. Likewise, the treatment that promoted greater fresh plant weight was O I 1 R 3 + hydrogel (3), Table . According to the Kruskal-Wallis test, there was no difference between treatments for the variable number of leaves.
Table 2. Tukey test and mean comparisons of isolated results. Letters indicate grouping. All isolates were hydrogel-coated except the control. Length of the main root (LR) from the stem base to the tip, root diameter from the longitudinal middle part (DR), plant length (LP) from the top to the base of the stem, the fresh plant weight (FPW), leaves number (LN)
3.2. Trend in endophytism of fungi used in the experiment
Obtained data on endophytism indicated a significant difference between treatments (X20.0009 = 28.2403, P < 0.05). According to the comparison of least squares means obtained through the Kruskal-Wallis test, it was possible to observe efficacy in root endophytism in treatment 9 (O III 3 M 3) followed by treatments 2 (V IV 2.1) and 5 (O I 3.2), Table .
Table 3. Comparison of least squares means obtained with Kruskal-Wallis test of T. longibrachiatum endophytism on the banana root
Although the recorded temperature exhibited some variation, ranging from 13 ºC to 26 ºC in the season from December 2021 to March 2022 in “El Jobo”, Tlapacoyan, Veracruz state, Mexico (Figure ), and from 13 ºC to 31 ºC in the season from June to September 2022 at the Facultad de Ciencias Agrícolas y Pecuarias, Teziutlán Puebla Mexico (Figure ); places where the experiments were conducted, the results of the variables for both applied treatments were similar.
Figure 1. Recorded temperatures during two different seasons when the experiment was conducted. a) temperatures recorded in the season from December 2021 to March 2022 at El Jobo, Tlapacoyan, Veracruz state, Mexico. b) temperatures recorded in the season from June to September 2022 at the Facultad de Ciencias Agrícolas y Pecuarias, Teziutlán Puebla Mexico.
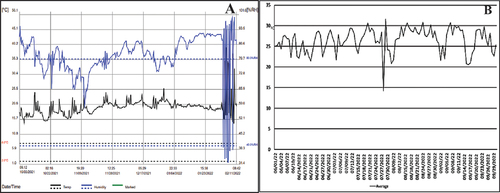
3.3. Persistence of endophytic and epiphytic fungal spores on hydrogel substrate
The ANOVA showed significant statistical differences F(9,5) = 3.34, (P ≤ 0.05) between treatments, and according to Tukey’s test (P ≤ 0.05), the treatment with the highest conidia persistence was the inoculum of isolate V IV 2.1, followed by V IV 3.2 and OI 3.2, all of the endophytic provenance (Figure ). The results of Pearson’s correlation between persistence and endophytism had a value of r = 0.5, in which a direct relationship was observed; however, the coefficient of determination was r2 = 0.2 indicating a weak relationship among variables.
4. Discussion
4.1. Root length, root diameter, plant length, fresh plant weight, and leaves number
Higher plant root length promoted by Trichoderma with hydrogel suggests that some Trichoderma isolates can stimulate root growth and provide several benefits for banana plant biotization (Castro-Restrepo et al., Citation2022). For instance, it has been observed that species such as T. asperellum, T. harzianum, and T. longibrachiatum increase root length when applied to tomato plants (Ruiz et al., Citation2018). Similarly, in passion fruit, an increase in root length was observed compared to the control when Trichoderma harzianum was applied (Cubillos et al., Citation2009). This growth is attributed to the ability of the isolates to solubilize phosphates, micronutrients, and mineral cations essential for plant metabolism (Tucci et al., Citation2011). However, the response in stimulating plant growth and development depends on the specific isolate used, its interaction with plant tissues, as well as factors such as soil concentration, application method, humidity, and temperature, among others (Grabka et al., Citation2022; Sridhar, Citation2012). Likewise, the combined effect of Trichoderma with hydrogel suggests a synergistic effect. All isolates, regardless of their origin, promoted root length to varying degrees compared to applying hydrogel alone. In studies conducted by Singh et al. (Citation2022), it was observed that in soil treated with 2 g m−2 of hydrogel, and rice seeds treated with 10 g kg−1 of Trichoderma, there was a significant improvement in crop growth by 6.45%, yield by 12.32%, the number of grains per panicle by 8.26%, nutrient absorption, and water use efficiency by 24.15% compared to the control.
On the other hand, the effects of the treatment on plant length were similar to the results observed by Sen et al. (Citation2019) in an experiment conducted with pots, in which seeds coated with hydrogel + Trichoderma were sown, and hydrogel was applied to the soil, resulting in a high rate of crop growth and high rate of relative growth. However, they did not measure stem diameter, which could be compared with the present results of treatments showing no effect on plant diameter. The increase in plant thickness when applying Trichoderma indicates hormonal stimulation of cells that make up the meristematic tissue responsible for both plant length and diameter growth. This is supported by the observation that Trichoderma spp. can solubilize phosphates and micronutrients essential for plant metabolism. Additionally, they produce phytohormones such as auxins, cytokinins, gibberellins, and especially indoleacetic acid (IAA) which stimulate plant growth (Domínguez et al., Citation2016; Tucci et al., Citation2011).
Likewise, the treatment O I 1 R 3 + Hydrogel (3) promoted a greater fresh weight, aligning with the results obtained by Singh et al. (Citation2022). This underscores the notion that even though the isolates belong to the same species, there are discernible differences in behavior among them. Furthermore, their effects vary when applied alongside hydrogel. These isolates exhibit specificity in influencing various characteristics such as stem and root length, which subsequently affect plant weight and even the number of leaves. In contrast to the present results, where no statistically significant differences were observed among treatments regarding the number of leaves, the study conducted by Dujeshwer and Singh (Citation2018) demonstrated that sowing seeds treated with 10 g kg−1 of Trichoderma in furrows with 5 kg ha−1 of hydrogel significantly improved plant height, biomass, and the number of branches.
4.2. The trend in endophytism of fungi used in the experiment
The results of endophytism agree with findings reported by Ruano et al. (Citation2016) and Samolski et al. (Citation2012), who noted that most Trichoderma species either colonize the root surface or live as endophytes within plant tissues. Notably, endophytism does not appear to be directly influenced by environmental conditions prior to inoculation, as suggested by Redkar et al. (Citation2022). However, their evolution from ancestors with diverse lifestyles equips them with a plethora of cell wall-modifying enzymes and proteins, ultimately determining their lifestyle and level of specialization (Lahrmann et al., Citation2015; Mesny et al., Citation2021). In this specific case, isolates VIV2.1 and OI3.2, originating from an endophytic lifestyle, exhibited a notable percentage of endophytism. This observation may imply that the pre-existing lifestyle of these isolates had an influence on their behavior in this experiment. Conversely, isolate OIII3M3, which had an epiphytic lifestyle prior to this experiment, demonstrated a higher capacity for endophytic colonization compared to VIV2.1, OI3.2.1, and OI3.2. This suggests the possibility that lifestyle influences the immediate subsequent behavior.
On the other hand, authors such as (Grabka et al., Citation2022) point out that the diversity and colonization rate of endophytic fungi is not static. Environmental factors such as rainfall, wind, and temperature associated with the season (Collado et al., Citation1999; Sridhar, Citation2012), as well as the location and plant age, may have an impact on the density of endophytic fungi (Arnold & Herre, Citation2003), These factors might provide a better explanation for the observed effects than the previous lifestyle theory. However, even though temperature data at the experiment sites fluctuated by 5 ºC, the obtained data were similar in both experiments. According to studies by Eastburn and Butler (Citation1991), T. harzianum exhibits higher colonization activity in soils with matric potentials of 0.5 to 1 bar, indicating that controlled humidity can enhance its soil activity. Therefore, the addition of hydrogel to the soil or substrate where plants and endophytic fungi grow can help maintain and improve moisture levels (Yang et al., Citation2020). Thus, the correct dosage of applied hydrogel can also influence fungal endophytism. However, in the present study, such an influence was not observed, possibly due to the absence of treatments without hydrogel that could have helped explain this effect. In this case, the applied dosage for both experiments was 3 g L−1 of hydrated hydrogel, which was similar to studies conducted by Pontes et al. (Citation2018) who mentioned that the best dosage for Enterolobium contortisiliquum was 2 to 3 g L−1, and that a high dosage of hydrogel could potentially lead to plant mortality. However, the impact of dosage on endophytism and fungal mortality remains to be fully understood. Therefore, further studies incorporating additional variables are recommended to gain a better understanding of its effect on fungi
4.3. Persistence of endophytic and epiphytic fungal spores on hydrogel substrate
For the higher persistence rate observed by fungi of endophytic origin, authors like Eastburn and Butler (Citation1991); Knudsen and Bin (Citation1990) reported that Trichoderma persistence in soil is related to several factors like the humidity among others, as its availability improves the conditions of the microbial communities present in the soil. Likewise, when the hydrogel is applied to a substrate, the moisture provided improves soil conditions, since the hydrogel retains 90% of the supplied water, i.e., 2/3 of this water is absorbed by the plant, while the remaining water remains available in the soil and can be used by microorganisms (Chang et al., Citation2021). For example, Krasnopeeva et al. (Citation2022); Chang et al. (Citation2021) found that the hydrated technical copolymer of acrylamide and potassium acrylate which contains 5.28% unpolymerized monomers, can be colonized by soil bacteria such as Rhizobium radiobacter 28SG and Bacillus aryabhattai 31SG. Likewise, Dehkordi (Citation2018) tested the use of superabsorbent polymers on the persistence of microorganisms and did not observe an antagonistic influence on soil microbial colonies, enhancing soil microbial movement.
However, not all types of hydrogel can preserve bacterial colonies in the soil, and the reactions of various microorganisms to this composition and its dosage may differ (Dehkordi, Citation2018). In this case, conidia from epiphytic fungal isolates exhibited lower persistence, as illustrated in Figure . These observations suggest that endophytic fungi have a greater ability to survive in a substrate than epiphytes. However, while the correlation results between persistence and endophytism imply a direct relationship, the determination coefficient indicates a weak connection between these variables. This suggests that endophytic or epiphytic behavior does not significantly influence the persistence of spores in the substrate that interacts with the plant root.
In addition, although the present study was directed to the number of spores recovered and not to plant survival, it is important to study this aspect since authors such as Sen et al. (Citation2019) reported that in plants subjected to drought, higher persistence in plants treated with hydrogel and Trichoderma was observed, in contrast to untreated plants, and suggests using the combination of hydrogel plus Trichoderma.
5. Conclusion
In conclusion, our hypothesis regarding the previous endophytic behavior of fungi as a determinant of their tendency to become endophytic in the roots of other plants is not always true. For example, isolate 9 (OIII3M3) of non-endophytic origin exhibited the highest endophytic persistence. Neither lifestyle appears to significantly influence the persistence of spores in the substrate interacting with the root. Therefore, the treatment with the V IV 2.1 endophytic isolate exhibited the highest conidia persistence but may grow either inside or outside plant tissues. Additionally, the combined effect of isolates O I 5 M 3, VIV2.1, and OI1R3 of T. longibrachiatum with hydrogel showed a synergistic effect, promoting increased stem and root length, fresh plant weight, and providing benefits for banana acclimatization.
Acknowledgments
The authors extend their appreciation to Consejo Nacional de Ciencia y Tecnología (CONACyT), Mexico for the scholarship provided to the main author for his training and development of this research work.
Disclosure statement
No potential conflict of interest was reported by the author(s).
Data availability statement
Data are accessible through the corresponding author Carmela Hernández Domínguez, [email protected]
Additional information
Funding
References
- Alam, B., Lǐ, J., Gě, Q., Khan, M. A., Gōng, J., Mehmood, S., Yuán, Y., & Gǒng, W. (2021). Endophytic fungi: From symbiosis to secondary metabolite communications or vice versa? Frontiers Plant Science, 12, 791033. https://doi.org/10.3389/fpls.2021.791033
- Arnold, A. E., & Herre, E. A. (2003). Canopy cover and leaf age affect colonization by tropical fungal endophytes: Ecological pattern and process in Theobroma cacao (Malvaceae). Mycology, 95(3), 388–11. PMID: 21156627. https://doi.org/10.1080/15572536.2004.11833083
- Barnett, H. L., & Hunter, B. B. (1998). Illustrated genera of imperfect fungi (4th ed.) (p. 218). APS Press, St Paul.
- Castro-Restrepo, D., Dominguez, M. I., Gaviria-Gutiérrez, B., Osorio, E., & Sierra, K. (2022). Biotization of endophytes Trichoderma asperellum and Bacillus subtilis in Mentha spicata microplants to promote growth, pathogen tolerance and specialized plant metabolites. Plants, 11(11), 2–15. https://doi.org/10.3390/plants11111474
- Chai, Q., Jiao, Y., & Yu, X. (2017). Hydrogels for biomedical applications: Their characteristics and the mechanisms behind them. Gels, 3(1), 1–6. https://doi.org/10.3390/gels3010006
- Chandran, H., Meena, M., Barupal, T., & Sharma, K. (2020). Plant tissue culture as a perpetual source for production of industrially important bioactive compounds. Biotechnology Reports, 20, e00450. https://doi.org/10.1016/j.btre.2020.e00450
- Chang, L., Xu, L., Liu, Y., & Qiu, D. (2021). Superabsorbent polymers used for agricultural water retention. Polymer Testing, 94, 107021. https://doi.org/10.1016/j.polymertesting.2020.107021
- Collado, J., Platas, G., González, I., & Peláez, F. (1999). Geographical and seasonal influences on the distribution of fungal endophytes in Quercus ilex. New Phytology, 144(3), 525–532. https://doi.org/10.1046/j.1469-8137.1999.00533.x.MID:33862861
- Cubillos, J., Valero, N., & Mejía, L. (2009). Trichoderma harzianum as a promoter of passion fruit plant growth (Passiflora edulis varFlavicarpa Degener). Colombian Agronomy, 27(1), 81–86.
- Dehkordi, D. K. (2018). Effects of hydrophilic polymers on soil water, wheat plant and microorganisms. Applied Ecology and Environmental Research, 16(2), 1711–1724. https://doi.org/10.15666/AEER/1602_17111724
- Domínguez, S., Rubio, M. B., Cardoza, R. E., Gutiérrez, S., Nicolás, C., Bettiol, W., Hermosa, R., & Monte, E. (2016). Nitrogen metabolism and growth enhancement in tomato plants challenged with trichoderma harzianum expressing the Aspergillus nidulans Acetamidase amdS gene. Frontiers in Microbiology, 7, 1182. https://doi.org/10.3389/fmicb.2016.01182
- dos Reis, J. B. A., Lorenzi, A. S., & Do Vale, H. M. M. (2022). Methods used for the study of endophytic fungi: A review on methodologies and challenges, and associated tips. Archives of Microbiology, 204(675), 2–30. https://doi.org/10.1007/s00203-022-03283-0
- Dujeshwer, R., & Singh, K. (2018). Effect of hydrogel and Trichoderma on growth and quality of linseed varieties under rainfed condition. International Journal of Chemical Studies, 6(3), 1896–1899. https://doi.org/10.13140/RG.2.2.14830.05447
- Eastburn, D. M., & Butler, E. (1991). Effects of soil moisture and temperature on the saprophytic ability of Trichoderma harzianum. Mycology, 83(3), 257–263. https://doi.org/10.1080/00275514.1991.12026009
- Firáková, S., Šturdíková, M., & Múčková, M. (2007). Bioactive secondary metabolites produced by microorganisms associated with plants. Biology, 62(3), 251–257. https://doi.org/10.2478/s11756-007-0044-1
- Galan, V., Rangel, A., Lopez, J., Hernandez, J. B. P., Sandoval, J., & Rocha, H. S. (2018). Propagación del banano: Técnicas tradicionales, nuevas tecnologías e innovaciones. Revista Brasileira de Fruticultura, 40(4), 1–21. https://doi.org/10.1590/0100-29452018574
- Galindo-Solís, J. M., & Fernández, F. J. (2022). Endophytic Fungal Terpenoids: Natural Role and Bioactivities. Microorganisms [Internet], 10(2), 2–22. ( PMID: 35208794; PMCID: PMC8875210). https://doi.org/10.3390/microorganisms10020339
- Goettel, M. S., & Douglas, G. I. (1996). Fungi: Hyphomycetes. In L. A. Lacey Ed., Manual of techniques in patology (pp. 221–223). https://doi.org/10.1016/B978-012432555-5/50013-0
- Grabka, R., d’Entremont, T. W., Adams, S. J., Walker, A. K., Tanney, J. B., Abbasi, P. A., & Ali, S. (2022). Fungal endophytes and their role in Agricultural plant protection against pests and pathogens. Plants, 11(3), 2–29. https://doi.org/10.3390/plants11030384
- Gundrathi, I., & Babu, P. S. (2019). Enhancing acclimatization of tissue cultured plants of Albizia amara by Biotization. International Journal of Scientific Research in Biological Sciences, 6(4), 43–50. https://doi.org/10.26438/ijsrbs/v6i4.4350
- Hazarika, B. N. (2003). Acclimatization of tissue-cultured plants. Current Science, 85(12), 1704–1712. https://www.jstor.org/stable/24109975
- Huang, W. X., Wei, Z. Z., Niu, G. Y., Zhang, Y. J., & Shao, H. F. (2018). Effects of sodium polyacrylate and potassium polymer on growth and physiological characteristics of different flue-cured tobaccos. Bulgarian Chemical Communications, 50(2), 315–323. http://bcc.bas.bg/BCC_Volumes/Volume_50_Number_2_2018/BCC-50-2-2018-APCET-5-Huang-315-323.pdf
- Husain, M. S. B., Gupta, A., Alashwal, B. Y., & Sharma, S. (2018). Synthesis of PVA/PVP based hydrogel for biomedical applications: A review. Energy Sources, Part A: Recovery, Utilization, and Environmental Effects, 40(20), 2388–2393. https://doi.org/10.1080/15567036.2018.1495786
- Hussain, A., Ahmed, I., Nazir, H., & Ullah, I. (2012). Plant tissue culture: Current status and opportunities. In A. Leva (Ed.), Recent advances in plant in vitro culture plant. In technology (pp. 2–28). https://doi.org/10.5772/50568
- Irsyadi, M. B. (2021). Factors that effect of the optimal plantlet growth from tissue culture on the acclimatization stage. Proceeding International Conference on Science and Engineering, 4(3), 100–104. http://ejournal.uin-suka.ac.id/saintek/icse/article/view/2805/2203
- Knudsen, G. R., & Bin, L. (1990). Effects of temperature, soil moisture, and wheat bran on growth of Trichoderma harzianum from alginate pellets. Phytopathology, 80(8), 724–727. https://doi.org/10.1094/Phyto-80-724
- Krasnopeeva, E. L., Panova, G. G., & Yakimansky, A. V. (2022). Agricultural Applications of Superabsorbent Polymer Hydrogels. International Journal of Molecular Science, 23(23), 15134–15136. https://doi.org/10.3390/ijms232315134
- Lahrmann, U., Strehmel, N., Langen, G., Frerigmann, H., Leson, L., Ding, Y., Scheel, D., Herklotz, S., Hilbert, M., & Zuccaro, A. (2015). Mutualistic root endophytism is not associated with the reduction of saprotrophic traits and requires a noncom promised plant innate immunity. New Phytology, 207(3), 841–857. PMID: 25919406. https://doi.org/10.1111/nph.13411
- Lee, T. J., Zobayed, S. M. A., Firmani, F., & Park, E. J. (2019). A novel automated transplanting system for plant tissue culture. Biosystems Engineering, 181(1), 63–72. https://doi.org/10.1016/j.biosystemseng.2019.02.012
- Mesny, F., Miyauchi, S., Thiergart, T., Pickel, B., Atanasova, L., Karlsson, M., Hüttel, B., Barry, K. W., Haridas, S., Chen, C., Bauer, D., Andreopoulos, W., Pangilinan, J., LaButti, K., Riley, R., Lipzen, A., Clum, A., Drula, E. … Hacquard, S. (2021). Genetic determinants of endophytism in the Arabidopsis root mycobiome. Nature Communication, 12(1), 7227. https://doi.org/10.1038/s41467-021-27479-y
- Montesano, F. F., Parente, A., Santamaria, P., Sannino, A., & Serio, F. (2015). Biodegradable Superabsorbent hydrogel Increases water retention properties of growing media and plant growth. Agriculture and Agricultural Science Procedia, 4(11), 451–458. https://doi.org/10.1016/j.aaspro.2015.03.052
- Ortega-Torres, A. E., Flores-Tejeida, L. B., Guevara-González, R. G., Rico-García, E., & Soto-Zarazúa, G. M. (2020). «El Hidrogel Acrilato De Potasio Como Sustrato En Cultivo De Pepino Y Jitomate». Revista mexicana de ciencias agrícolas, 11(6), 1447–1455. https://doi.org/10.29312/remexca.v11i6.2222
- Pontes, R. A., Gondim, F. A., & Costa, M. C. G. (2018). Seedling growth of tree species under doses of hydrogel and two levels of luminosity. Árvore Journal, 42(1), 2–9. https://doi.org/10.1590/1806-90882018000100012
- Ramírez-Torres, D. C., Reyes-López, D., Domínguez-Perales, L. A., Orduño-Cruz, N., Grifaldo-Alcántara, P. F., & Hernández-Domínguez, C. (2022). Endophytism of Trichoderma species and root growth in two varieties of banana in vitro. Acta Agrícola y Pecuaria, 8(1), 1–8. https://doi.org/10.30973/aap/2022.8.0081013
- Redkar, A., Sabale, M., Zuccaro, A., & DiPietro, A. (2022). Determinants of endophytic and pathogenic lifestyle in root colonizing fungi. Current Opinion in Plant Biology, 67, 102226. https://doi.org/10.1016/j.pbi.2022.102226
- Rivera, R. D., Muekay, C. M., Saltos, J. R. M., & Intriago, D. A. M. (2021). Volumen de humedecimiento por la aplicación de hidrogel en suelos de diferentes texturas. La Granja, 33(1), 67–75. https://doi.org/10.17163/lgr.n33.2021.06
- Ruano, D., Prieto, P., Rincón, A. M., Gómez-Rodríguez, M. V., Valderrama, R., Barroso, J. B., & Mercado-Blanco, J. (2016). Fate of Trichoderma harzianum in the olive rhizosphere: Time course of the root colonization process and interaction with the fungal pathogen verticillium dahliae. BioControl, 61(3), 269–282. https://doi.org/10.1007/s10526-015-9706-z
- Ruiz, M. F., Ornelas-Paz, J. D. J., Olivas-Orozco, G. I., Acosta-Muñiz, C. H., Sepúlveda-Ahumada, D. R., Pérez-Corral, D. A., Rios-Velasco, C., Salas-Marina, M. Á., Fernández-Pavía, S. P., Ruiz-Cisneros, M. F., de Ornelas-Paz, J., Olivas-Orozco, G. I., Acosta-Muñiz, C. H., Sepúlveda-Ahumada, D. R., Pérez-Corral, D. A., Rios-Velasco, C., Salas-Marina, M. Á., & Fernández-Pavía, S. P. (2018). Effect of Trichoderma spp. And phytopathogenic fungi on plant growth and quality of tomato fruit. Mexican Journal of Phytopathology, 36(3), 444–456. https://doi.org/10.18781/r.mex.fit.1804-5
- Salgado Pirata, M., Correia, S., & Canhoto, J. (2022). Ex Vitro Simultaneous Acclimatization and Rooting of Tamarillo Plants (Solanum betaceum Cav.) Propagated In Vitro: Effect of Substrate and Mineral Nutrition. Agronomy, 12(5), 2–13. https://doi.org/10.3390/agronomy12051082
- Samolski, I., Rincón, A. M., Pinzón, L. M., Viterbo, A., & Monte, E. (2012). The qid74 gene from Trichoderma harzianum has a role in root architecture and plant biofertilization. Microbiology (Reading, England), 158(1), 129–138. https://doi.org/10.1099/mic.0.053140-0
- Schouten, A. (2019). Endophytic fungi: Definitions, diversity, distribution and their significance in plant life. Centre for Agriculture and Biosciences International. https://doi.org/10.1079/9781786399427.0006
- Sen, A., Singh, R. K., Yadaw, D., Kumari, P., Srivastava, V. K., Upadhyay, P. K., Sankar, A., Mishra, J., Das, A., Zaidi, N. W., & Dar, M. H. (2019). Effect of Trichoderma and hydrogel on growth, yield, and yield attributes of direct seeded rice (Oryza sativa) under rainfed condition. Indian Journal of Agricultural Sciences, 89(2), 333–338. https://doi.org/10.56093/ijas.v89i2.87094
- Singh, R. K., Jatav, H. S., Lakpale, R., Khan, M., Rajput, V. D., & Minkina, T. (2022). Hydrogel-based Trichoderma formulation effects on different varieties of rice under rainfed condition of Indo-Gangetic Plains. Environment Development Sustainability, 24(1), 7035–7056. https://doi.org/10.1007/s10668-021-01738-w
- Sridhar, K. (2012). Aspect, and prospect of endophytic fungi. In S. C. Sati & M. Belwal (Eds.), Microbes: Diversity and biotechnology. (pp. 43–62). Daya Publishing House: ISBN 9788170357940. https://doi.org/10.13140/RG.2.1.4207.5766.
- Subhashinia, D. V., Anuradhab, M., Damodar, R. D., & Vasanthi, J. (2016). Development of bioconsortia for optimizing nutrient supplementation through microbes for sustainable tobacco production. International Journal of Plant Production, 10(4), 479–490. https://ijpp.gau.ac.ir/article_3044_7fe5184e591e78e073525210d119da7e.pdf
- Sudha, V., Govindaraj, R., Baskar, K., Al-Dhabi, N., & Duraipandiyan, V. (2016). Biological properties of endophytic fungi. Brazilian Archives of Biology and Technology, 59(1), 1–7. https://doi.org/10.1590/1678-4324-2016150436
- Tseng, Y.-H., Rouina, H., Groten, K., Rajani, P., Furch, A. C. U., Reichelt, M., Baldwin, I. T., Nataraja, K. N., Uma Shaanker, R., & Oelmüller, R. (2020). An endophytic Trichoderma strain promotes growth of its hosts and defends against pathogen attack. Frontiers in Plants Science, 11, 573670. https://doi.org/10.3389/fpls.2020.573670
- Tucci, M., Ruocco, M., De Masi, L., De Palma, M., & Lorito, M. (2011). The beneficial effect of trichoderma spp. On tomato is modulated by the plant genotype. Molecular Plant Pathology, 12(4), 341–354. https://doi.org/10.1111/j.1364-3703.2010.00674.x
- Twaij, B. M., Jazar, Z. H., & Hasan, M. N. (2020). Trends in the use of tissue culture, applications and future aspects. International Journal of Plant Biology, 11(1), 8385. https://doi.org/10.4081/pb.2020.8385
- Tyśkiewicz, R., Nowak, A., Ozimek, E., & Jaroszuk-Ściseł, J. (2022). Trichoderma: The current state of its application in agriculture for the biological control of fungal phytopathogens and the stimulation of plant growth. International Journal of Molecular Science, 23(4), 2329. https://doi.org/10.3390/ijms23042329
- Yang, C. R., Feng, W., Liu, J., Qu, Z., & Miao, Q. (2020). Effects of super-absorbent polymer on soil remediation and crop growth in Arid and semi-Arid areas. Sustainability, 12(18), 2–135. https://doi.org/10.3390/su12187825