Abstract
The series of experiments summarized here were conducted with the objective to evaluate the benefits of using biochar for coating corn seeds. Seeds coated with a slurry containing bio-based ingredients and biochar were tested for germination and vigor, and for their potential to being infected by the fungus Aspergillus flavus, using a novel single seed incubator specifically designed for these purposes. Biochar-treated seeds were also planted for two years in experimental fields in the Mississippi Delta to evaluate their effect on corn yield and aflatoxin contamination of kernels. Experiments were conducted with two types of commercial biochar; one was obtained from hardwood residues and the other from coconut shells. Application of both types of biochar for coating the seeds did not affect seed germination and vigor. However, treated seeds showed increased wettability and a more rapid water uptake. This resulted in a 8.5% shortening of germination time. Microbiological analysis using plate culturing and qPCR methods showed that biochar was not conducive to the growth of A. flavus. This was also confirmed by analyzing soil samples that were collected from experimental fields located in the Mississippi Delta. Most importantly, although aflatoxin contamination was different in the two experimental years, aflatoxin contamination of corn kernels was not affected by biochar-based formulations.
1. Introduction
Rapid, uniform, and successful seed germination and seedling stand establishment are basic requirements for satisfactory crop production. After planting, seeds and seedlings are exposed to a variable and unpredictable number of biotic and abiotic stress factors that can reduce, delay, or even compromise their germination and seedling stand establishment. Seeds of many agricultural crops are thus routinely treated with pest control agents and other substances to reduce these risks and improve plantability (e.g., better flowability, seed singulation, reduced seed skip) (Hitaj et al., Citation2020; McGee, Citation1988). Among the different treatment approaches, seeds with regular shape and adequate size (e.g., corn, soybean, cotton, sunflower, etc.) are film-coated by covering the seeds with a liquid slurry containing plastic-forming polymers, pesticides, and pigments, using rotating drum or pan coating machines. For rapid drying of the treated seeds, fillers are routinely added to the slurry. Common fillers that are used in the seed treatment include clay minerals such as talc and bentonite, calcium carbonate, gypsum, zeolite, peat, etc (Accinelli et al., Citation2021; Taylor et al., Citation1991).
Since the introduction of industrial seed film-coating in the 1980s, agrochemical companies have developed their own proprietary formulations with a variety of synthetic polymers to uniformly cover seed surfaces with adherent plastic-like coats. However, significant but inconsistent amounts of coating fragments can become detached by mechanical abrasion during seed handling and planting operations, even when effective coating formulations are used. These coating fragments then become dispersed in the environment (Accinelli et al., Citation2018, Citation2020; Nuyttens et al., Citation2013). These detached seed coat fragments can retain pesticide residues, and studies have indicated that these fragments can have a detrimental effect on bees, terrestrial birds, and other organisms and can contaminate the soil with persistent microplastic-like particles (Krupke et al., Citation2012). For this reason, there is increasing interest in developing biodegradable and bio-based film coat formulations, especially when they are to be combined with biopesticides. As with all film coat formulations, the germination and vigor of treated seeds should not be adversely affected by the surface-applied substances. In addition, the risk of promoting the growth of fungi capable of infecting the root after seed germination, other plant pathogens, and other harmful microorganisms should be also accurately evaluated. The soil-borne filamentous and aflatoxin-producing fungus Aspergillus flavus is included among these microorganisms.
Aflatoxins are carcinogenic secondary metabolites produced by A. flavus and related species, including A. parasiticus and A. nominus. These fungi can infect many crops, such as corn, peanuts, cotton, and others, resulting in direct and indirect losses in many agricultural areas worldwide (Abbas et al., Citation2006). A. flavus is a ubiquitous fungus, which is readily isolated from soil and crop residues remaining in the upper soil. In the soil, the fungus plays an important role in the decomposition of organic matter and humus formation. Spores that are produced during the saprophytic life phase of this fungus are transported by air, raindrops, insects, and other vectors and can thus infect susceptible crops (Scheidegger & Payne, Citation2003). A. flavus is ubiquitous in the soil microbial community, and its relative level of soil inoculum is correlated with the risk of aflatoxin contamination of susceptible crops in combination with other factors (e.g., air temperature and relative humidity, etc.) (Abbas et al., Citation2009; Fouché et al., Citation2020; Juraschek et al., Citation2022).
In recent years, an increasing number of seed and agrochemical companies have proposed coating solutions containing natural ingredients mixed with biodegradable and renewable polymers instead of conventional oil-based and persistent polymers. Although these solutions are environmentally preferable, in that no release of persistent microplastic fragments into the soil environment occurs, their effects on the soil microbial community have not been clearly elucidated, particularly effects on proliferation of plant pathogenic and/or mycotoxin-producing fungi (i.e., A. flavus).
Biochar, a soil amendment that is obtained from thermal decomposition of organic materials under limited oxygen conditions (Das et al., Citation2021; Lehmann, Citation2007), is a potential candidate to be used as a filler for bio-based coating solutions. Due to its high water retention capability, biochar is expected to facilitate water imbibition by seeds, resulting in improved seedling establishment. However, very little information is available on the use of biochar as a material for the treatment of seeds (Głodowska et al., Citation2016; Zhang et al., Citation2022).
The main objective of the present study was to evaluate the benefits of a formulation containing biodegradable polymers and biochar powder for coating corn (Zea mays) seeds. Since no specific tests for evaluation of seed germinability of coated seeds are currently available, another objective of the present study was to design a novel tool for assessing this important parameter. Finally, the study also investigated the effects of this bio-based formulation on corn yield and kernel size, population and composition of soil A. flavus, and aflatoxin contamination of corn kernels.
2. Methods and materials
2.1. Seed coating
Seeds of the corn hybrid VT Double Pro (Bayer CropScience, St. Louis, MO, USA) were coated with a two-step procedure using a Hege 11 liquid seed treater (Wintersteiger Inc., Salt Lake City, UT, USA). First, approximately 0.9 kg of seeds were placed in the liquid seed treater spinning plate. While spinning the seeds, 40 mL of 7.5% pre-gelatinized starch solution was slowly added using a 50-mL syringe to coat the seeds. Next, 40 g of biochar powder was added to the sticky seeds and mixed until the seeds were completely covered. The coated seeds were air dried for 24 h at room temperature using a box fan. Two types of biochar were selected for this study, a hardwood biochar (HB; Rockwood Sustainable Solutions, Lebanon, Tennessee, USA) and a coconut hull-based biochar (CN; Cool Planet, Greenwood Village, Colorado, USA). For both biochar types, the application rate was 45 g·kg−1 seeds. The chemical composition and additional properties of the two biochar types are summarized in Table .
Table 1. Selected properties of the two biochar types used in the study
3. Seed wettability, water uptake, and seed germination
Wettability of treated seeds was evaluated by the sessile drop method using a Theta Lite optical tensiometer (Biolin Scientific AB, Västra Frölunda, Sweden). The apparent contact angle was measured during a 10 s interval after dropping a 10 µL drop of double distilled water on the seed surface. For each treatment, a total of 10 seeds were examined. Measurements also included seed samples that were coated with the commercial polymer formulation Sepiret (BASF, Ludwigshafen, Germany) and dewaxed seeds. The latter seeds were obtained by the removal of the external waxy layer as described in Accinelli et al. (Citation2021).
Water uptake and germination ability were evaluated using a novel seed germination tube specifically designed for testing treated seeds (Figure ). As shown in Figure , a single seed was placed in the center of a 2-cm diameter cylinder provided with two lateral cotton filters (diameter of 10 mm; length of 18 mm). The disc was then secured at the connection plane between two conical tubes, which were then connected using a screw cylinder. With the exception of the two 50 mL centrifuge tubes, all remaining parts of the test were designed and manufactured using a Form 3 L SLA 3D Printer equipped with a 100 µm resolution clear resin. Printer and resin were purchased from Formlabs Inc. (Boston, MA, USA). The two filters were moistened with ultrapure water to achieve a total volume of 3 mL. Tubes were then incubated in a germination chamber at 25 °C with 12 h of light per day. For water uptake measurements, seeds were removed at selected intervals (5, 10, 20, 30 and 40 h) and weighed before and after drying at 105 °C for 48 h. Germination, expressed as percent of normal seedlings, was recorded daily with mean germination time (MGT) calculated as follows: MGT = Σ(ni hi)/Σni, in which ni is the number of seedlings present on interval i, and hi is the number of hours since the beginning of the test (Ellis & Roberts, Citation1980). Seeds were considered to have germinated after the radicle emerged. For each treatment, seed water uptake and germination data were obtained from 50 and 100 seeds, respectively.
Figure 1. Corn seed germination and seed water uptake studies were carried out using a specifically designed test tube system (left). Seeds (A: control; B: dewaxed; C: commercially treated; D: biochar treated) were secured between two cotton plugs at the junction of two test tubes held together by a threaded sleeve created with a 3D printer.
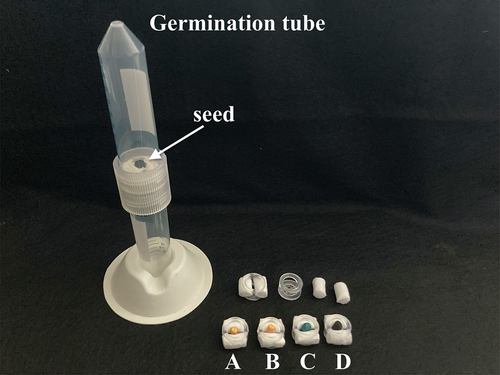
4. Field experiments
Biochar-coated seeds were also evaluated in a field experiment conducted at the Mississippi State University Delta Research and Extension Center (Stoneville, MS, USA). Experiments were carried out in 2020 and 2021 in two 2-ha corn fields (33.4240° N, 90.9151° W) that were managed according to conventional no-till practices of the region. Each plot (15 m long and 10 m wide) was separated by a 1 m wide buffer area. Corn was planted on May 6th and April 6th in 2020 and 2021, respectively, using a MaxEmerge 5 Planter (John Deere Co, Moline, IL, USA) at the rate of 84,000 seeds per ha. The soil had a textural composition of 331, 418, and 252 g·kg−1 of sand, silt and clay, respectively, a pH of 6.2 and total carbon and nitrogen of 13.1 and 1.4 g·kg−1, respectively. Corn was harvested on October 11th and September 1st in 2020 and 2021, respectively, using a Kincade 8-XP plot combine (Kincaid Equipment Manufacturing, Haven, KS, USA) equipped with H2 grain gauge and Mirus software (Harvest Master, Logan, UT, USA). Corn samples were collected in unsealed bags and allowed to dry at 45 °C in a ventilated oven for two to three days to ensure the moisture content was below 14% to prevent clumping during grinding for analyses.
5. Aflatoxin analysis
Corn samples were prepared for extraction by grinding approximately 500 g to a cornmeal consistency. Fifty grams of the ground sample was subsequently combined with 70% methanol at a 1:5 ratio (w/v). The resulting mixture was shaken for 30 minutes on a reciprocal shaker at the lowest setting. After resting, the solution was strained through Whatman #1 filter paper to remove large particulates. Twenty milliliters of the filtered solution were collected in scintillation vials, which were stored at − 20 °C until analyzed.
For aflatoxin analysis by high-performance liquid chromatography (HPLC), 500 µL of sample was diluted with 500 µL 100% acetonitrile in 2 mL microcentrifuge tubes. A volume of 800 µL of the mixture was further purified through solid phase extraction using a homemade clean-up column (3 ml syringe) packed with alumina basic powder (200 g) and a glass wool filter under high-pressure vacuum. Purified samples were transferred to 700 µL HPLC vials (Waters Corp., Milford, MA, USA). Five standards were created by serial dilution of a working stock solution and injection solution. The working stock solution was derived from Aflatoxin Mix 4 solution (Sigma-Aldrich, St Louis, MO, USA). Blanks were loaded before and after the standards and after every 10 samples. Waters’ Empower software directed the Waters 717 Autosampler to extract 20 µL of sample and flow through a Waters Nova-Pak® C18 4 µm 3.9 × 150 mm column at 30 °C by a Waters HPLC 515 pump at 0.9 mL⋅min−1 and approximately 3600 psi. Post-column derivatization was performed by a Photochemical Reactor for Enhanced Detection (Aura Inc., San Diego, CA, USA), and aflatoxin was detected with a Waters 2475 Fluorescence Detector set at 365 nm (excitation) and 440 nm (emission). Mobile phase was a solution of water, methanol, and 1-butanol (700:360:12.5) while blanks and injection solutions were composed of methanol, water, and glacial acetic acid (600:380:1). Data were expressed as the total of aflatoxins B1, B2, G1 and G2.
6. Fungal isolations
Samples from the seed germination study were also analyzed for A. flavus infection during the process. Microbial DNA that was trapped by the pair of cotton plugs was isolated using the DNeasy UltraClean Microbial Kit (Qiagen Corp, Hilden, Germany) following the manufacturer’s instructions. DNA was quantified using a BioDrop spectrophotometer (BioDrop Ltd, Cambridge, UK) and then amplified with an Open qPCR (ChaiBio, Santa Clara, CA, USA) using the following conditions: 2 min at 50 °C, 10 min at 95 °C, 40 cycles of 15 s at 95 °C and 1 min at 60 °C. Each 25 μL of reaction mixture contained 12.5 μL of 2× TaqMan Universal PCR Master Mix (Applied Biosystems, Foster City, CA, USA), 0.2 μM of each primer (Accinelli et al., Citation2022), and 40 ng of DNA. Samples were amplified on a standard curve (r2 = 0.92; efficiency = 94%; slope = − 0.21) generated by plotting cycle threshold values (Ct) against known spore concentration values.
Aspergillus populations within individual plots were assessed during the season for changes over time by culturing soil samples. Soil samples were collected prior to planting and post-harvest. From the center of each plot, three subsamples were taken from the top 4–6 cm of soil with a sterile trowel at random intervals. The subsamples were combined in a 1-quart Ziploc® bag to achieve approximately 1 kg samples. Soil isolations were performed as described by Abbas et al. (Citation2004). In brief, 10 g soil was added to a screw cap bottle containing 90 mL of potassium phosphate buffer and shaken on a reciprocal shaker for 30 minutes at high speed. Using a wide bore pipette tip, 200 μL of the mixture was transferred onto modified Dichloran Rose Bengal (mDRB) agar and spread across the surface using a sterile cell spreader. Four replicates were made for each sample. Plates were then incubated upside down for five days at 37 °C. Propagule density of A. flavus was determined on selective media as described by Horn and Dorner (Citation1998). Ten colonies from each plate were transferred to potato dextrose agar plates amended with β-cyclodextrin and evaluated for percent aflatoxigenicity according to Abbas et al. (Citation2004).
7. Statistical analysis
Data from field experiments were subjected to the analysis of variance ANOVA. Experimental plots consisted of four 15-m rows, which were arranged in a randomized complete block design with six replicates. Mean values from field and laboratory studies were separated by Fisher’s least significant difference (LSD), and P values < 0.05 were considered significant. All the data were processed using the software SPSS ver. 27.0.1.0 (SPSS Ltd., Chicago, IL, USA).
8. Results and discussion
8.1. Effect of biochar on seed wettability, water uptake and germination
Seed surface wettability, as measured by the apparent contact angle between seed surface and sessile water drops, is shown in Figure . As indicated by these measurements, the surface of untreated seeds showed low affinity to water. More precisely, with an apparent contact angle higher than 90°, the external layer of seed corn pericarp can be categorized as hydrophobic. Removal of this waxy layer (e.g., dewaxed seeds) increased its wettability. Also, seeds coated with a commercial polymer mixture were more wettable than untreated seeds (Table ). The main role of the outer surface of the seed pericarp, the cuticle, is to protect seeds from pathogens and desiccation (Jeffree, Citation2006). Maintaining an adequate level of moisture inside the seed is directly connected to embryo longevity and vitality (Cordova-Tellez & Burris, Citation2002; Zhang et al., Citation2010). Application of the biochar-based formulation to the surface of corn seeds resulted in a significant increase in their wettability. With average wettability values of 24.3° to 25.5°, the external layer of these treated seeds turned hydrophilic, with no significant differences between the two biochar types. Increased surface area and occurrence of hydrophilic functional groups are some of the noted benefits from different agricultural applications of biochar (Hill et al., Citation2019). As a soil amendment, biochar has been used for reducing the phytotoxic effect of residual herbicides and other xenobiotics in soil (Marris, Citation2006; Rogovska et al., Citation2012; Wang et al., Citation2022). More recently, there has been increasing interest in achieving similar effects from the application of biochar by seed treatment (Ajeng et al., Citation2020). To date, most applications of biochar as a seed treatment have used it for pelleting seeds of native species to facilitate planting in vegetation restoration operations. This application is especially important for plant species that have small and irregularly shaped seeds (Ma et al., Citation2022). In these cases, biochar is combined with other organic or mineral materials (i.e., peat and clay minerals) using pelleting or extruder machines, to obtain seeds with uniform, spherical shapes and increased mass. With regular shapes and increased size, the plantability of biochar-pelleted seeds is consequently improved (Law et al., Citation2023). In the present experiment, the effects of biochar-coated seed on water uptake and germination were evaluated using a single seed test tube specifically designed for assessing these properties. Consistent with observations in soil amendment studies, water uptake was found to be more rapid in biochar-coated than in untreated control seeds. As indicated in Figure , after 10 h from the beginning of the water uptake study, the water contents of biochar-coated seed and control seeds were 27% and 38%, respectively. At the end of the 40-h experiment, moisture of both seed types was approximately 45%, with no significant differences among treated and untreated seeds. However, this plateau was reached significantly earlier in the treated than in the untreated seeds. Imbibition capability of biochar-coated seeds was compared with that of commercially coated and dewaxed seeds. Seed moisture measurements showed that water uptake was more rapid in the biochar-treated seeds than in others tested (Figure ). Application of the two different biochar powders did not affect seed germination (Table ). As expected, the more rapid seed water uptake also resulted in a shortening of the mean germination time of biochar-treated seeds (Table ). The wettability assay used in this study differed from other available approaches (i.e., rolled towel and sand germination test) in that samples remained undisturbed over the entire experimental period and physically separated from other seeds, thus avoiding potential interactions. In addition, this study showed that seed water uptake was similar in seeds that were coated with two different types of biochar. This is consistent with the wettability of this material, as a results of its chemical nature and high specific surface.
Figure 2. Examples of measurement of the apparent contact angles of sessile water drops on the surface of untreated corn seeds (A) and seeds that were coated with the hardwood biochar (B). Measurements were also conducted on samples of corn seed that were coated with a commercial polymer (C) and seed which were subjected to the removal of the external waxy layer (D, dewaxed seeds).
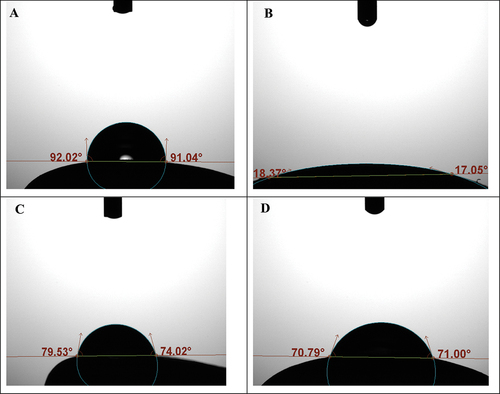
Figure 3. Water uptake by untreated corn seeds (control) and by seeds that were coated with hardwood (HW) or coconut (CN) biochar. Measurements are also included of samples of seed that were coated with a commercial polymer and seeds that were subjected to the removal of the external waxy layer (dewaxed seeds). Each point represents mean ± STD (n = 3).
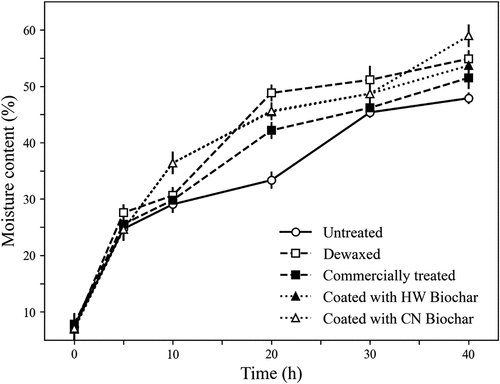
Table 2. Wettability of corn seeds was measured as reduced apparent contact angle of sessile water drops on the surface of seeds that were either untreated (control) or subjected to treatments with two types of biochar (hardwood, HW, and coconut, CN, biochar). Measurements included samples of seeds that were coated with a commercial polymer and seeds that were subjected to the removal of the external waxy layer (dewaxed seeds). Data are presented as mean ± STD. Values followed by the same letter are not significantly different (P < 0.05; LSD)
Table 3. Germination and mean germination time of untreated seeds (control) compared with seeds that were coated with hardwood (HW), coconut (CN) biochar, a commercial polymer or seeds which were subjected to removal of the external waxy layer (dewaxed seeds). Data are presented as mean ± STD. Values followed by the same letter in the same column are not significantly different (P < 0.05; LSD)
9. Microbiological aspects and aflatoxin contamination of corn kernels
In addition to evaluating seed germination, the test tube-based system described in this study, was also designed to allow for the rapid and effective recovery of microbial DNA from germinating seeds. As shown in Figure , the test tube was provided with two cotton plugs, which were placed on the opposite sides of each germinating seed. Cotton swabs are routinely used for recovering microbial DNA from various sources (Gray et al., Citation2023). In this study they were also used as a germination substrate. Preliminary investigations confirmed that using cotton instead of a polyester plug did not affect the amount of A. flavus and other microbial DNA recovered. Cotton plugs were also more effective in providing water to germinating seeds than those manufactured with polyester (data not shown). The cotton plugs were analyzed for DNA content using qPCR and summarized in Table .
Table 4. Aspergillus flavus propagules recovered during the germination process from untreated (control) seeds, and from seeds coated with hardwood (HW) biochar, coconut (CN) biochar, commercial polymer or subjected to the removal of the external waxy layer (dewaxed seeds). Data are presented as mean ± STD. Values followed by a letter are not significantly (P < 0.05) different from other values followed by the same letter (ANOVA)
In many corn growing areas, aflatoxin contamination of kernels is a serious threat. In addition to the application of specific crop management practices (i.e., avoiding crop water stress, effective insect control, etc.) and control strategies (i.e., field application of non-toxigenic A. flavus isolates), planting healthy seeds coated with materials not conducive to the growth of the fungus A. flavus is a basic prerequisite for reducing the risk of aflatoxin contamination of corn (Accinelli et al., Citation2019). Considering the increasing interest in using bio-based and biodegradable materials in seed coating, this aspect should be evaluated in the process of developing novel seed treatment formulations. As expected, the number of A. flavus propagules recovered from untreated seeds increased during the germination process. This was also observed with biochar-treated seeds and those coated with a commercial polymer or dewaxed. However, the initial level of A. flavus was slightly reduced by seed coating with biochar. Because A. flavus is a saprophyte and opportunistic plant pathogen, the highly oxidated and slowly degradable organic materials, such as biochar, do not likely promote fungal growth. Studies have shown that starchy and oil-rich substrates (i.e., corn endosperm, peanut cotyledons, etc.) are very conducive to the growth of A. flavus. When applied as a soil amendment, biochar is expected to improve soil aeration and water retention, creating more suitable conditions in the soil for the growth of soil microorganisms (Hammerschmiedt et al., Citation2021; Wei et al., Citation2021). However, data from this study indicated that application of the two types of biochar to seeds did not increase the growth of the fungus A. flavus, thus confirming the high resistance to microbial degradation of this material.
The size of A. flavus populations in field soil planted corn seed coated with biochar followed similar trends during the two years studied in Mississippi (Table ). In both years, the number of soil A. flavus propagules increased during the growing season, with a percentage of aflatoxin-producing isolates ranging from 36% to 44%. Comparable results were observed in soil planted with biochar treated seeds. These data are consistent with those from the in vitro experiment. This was further supported by chemical analysis of aflatoxin levels in harvested corn kernels (Table ). Aflatoxin contamination of harvested corn kernels depends on a variety of factors, including day and night air temperatures, soil moisture, insect damage to ears, etc (Abbas et al., Citation2009; Fouché et al., Citation2020). Although aflatoxin contamination varied among experimental plots, the effect of the biochar seed treatment was not significant. Aflatoxin contamination was higher in 2021 than in 2020. During the kernel filling period in these two years, average high temperatures were 36.70 °C in 2020 and 36.70 °C in 2021 and average low temperatures were 35.60 °C in 2020 and 36.70 °C in 2021 (Figure S1). Total rainfall was similar in the two years, with values of 714 mm and 753 mm, in 2020 and 2021, respectively (Figure S1). Similar environmental conditions resulted in similar grain yields, with average values of 117 q⋅ha−1 and no significant differences among treated and untreated seeds (Table ). Several studies have shown that aflatoxin contamination of corn is highly variable between years and difficult to predict (Abbas et al., Citation2017; Accinelli et al., Citation2019). The present experiment reinforced this concept, but more importantly, it demonstrated that coating corn seeds with biochar did not affect aflatoxin contamination of harvested corn kernels.
Table 5. Size of the soil Aspergillus flavus population and percent aflatoxigenicity of isolates recovered from plots planted with untreated corn seeds and corn seeds coated with one of two types of biochar (hardwood, HW, and coconut, CN). In both years, samples were collected at the beginning (Apr.) and at the end (Sept.) at the end of the corn growing season. Aflatoxin contamination of harvested corn kernels and yield are reported. Data are presented as mean ± STD. For each year, values followed by a letter are not significantly (P < 0.05) different from other values followed by the same letter (ANOVA)
10. Conclusions
The increasing interest in removing synthetic substances from seed treatment formulations has prompted research into novel bio-based and renewable materials to use in their place. Biochar possesses interesting properties that are useful for these purposes, including its high-water retention capability and low cost. However, the use of this material should not interfere with seed quality parameters, such as seed germination and vigor, or promotes the growth of the fungus A. flavus, which would increase the risk of aflatoxin contamination of harvested corn kernels, a serious threat to corn production. Laboratory and field experiments conducted for two years in the Mississippi Delta confirmed that biochar-treated seeds did not affect the size of the soil A. flavus population and the level of aflatoxin contamination of corn kernels. These results indicate that this renewable material can be used for coating corn seeds without increased concern regarding aflatoxin contamination.
Acknowledgement
We would like express our gratitude to Prof. Maria Letizia Focarete and Dr Monica Torsello of the Dept of Chemistry “Giacomo Giamician (University of Bologna) for their scientific support in measuring seed wettability.
Disclosure statement
No potential conflict of interest was reported by the author(s).
References
- Abbas, H. K., Accinelli, C., & Shier, W. T. (2017). Biological control of aflatoxin contamination in U.S. crops and the use of bioplastic formulations of Aspergillus flavus biocontrol strains to optimize application strategies. Journal of Agricultural and Food Chemistry, 65(33), 7081–11. https://doi.org/10.1021/acs.jafc.7b01452
- Abbas, H. K., Wilkinson, J. R., Zablotowicz, R. M., Accinelli, C., Abel, C. A., Bruns, H. A., & Weaver, M. A. (2009). Ecology of aspergillus flavus, regulation of aflatoxin production, and management strategies to reduce aflatoxin contamination of corn. Toxin Reviews, 28(2–3), 142–153. https://doi.org/10.1080/15569540903081590
- Abbas, H. K., Zablotowicz, R. M., Bruns, H. A., & Abel, C. A. (2006). Biocontrol of aflatoxin in corn by inoculation with non-aflatoxigenic Aspergillus flavus isolates. Biocontrol Science and Technology, 16(5), 437–449. https://doi.org/10.1080/09583150500532477
- Abbas, H. K., Zablotowicz, R. M., Weaver, M. A., Horn, B. W., Xie, W., & Shier, W. T. (2004). Comparison of cultural and analytical methods for determination of aflatoxin production by Mississippi Delta Aspergillus isolates. Canadian Journal of Microbiology, 50(3), 193–199. https://doi.org/10.1139/w04-006
- Accinelli, C., Abbas, H. K., Bruno, V., Khambhati, V. H., Little, N. S., Bellaloui, N., & Shier, W. T. (2022). Field studies on the deterioration of microplastic films from ultra-thin compostable bags in soil. Journal of Environmental Management, 305, 114407. https://doi.org/10.1016/j.jenvman.2021.114407
- Accinelli, C., Abbas, H. K., Bruno, V., Nissen, L., Vicari, A., Bellaloui, N., Little, N. S., & Shier, W. T. (2020). Persistence in soil of microplastic films from ultra-thin compostable plastic bags and implications on soil Aspergillus flavus population. Waste Management, 113, 312–318. https://doi.org/10.1016/j.wasman.2020.06.011
- Accinelli, C., Abbas, H. K., Bruno, V., Vicari, A., Little, N. S., Ebelhar, M. W., & Shier, W. T. (2021). Minimizing abrasion losses from film-coated corn seeds. Journal of Crop Improvement, 35(5), 666–678. https://doi.org/10.1080/15427528.2020.1861156
- Accinelli, C., Abbas, H. K., & Shier, W. T. (2018). A bioplastic-based seed coating improves seedling growth and reduces production of coated seed dust. Journal of Crop Improvement, 32(3), 318–330. https://doi.org/10.1080/15427528.2018.1425792
- Accinelli, C., Abbas, H. K., Shier, W. T., Vicari, A., Little, N. S., Aloise, M. R., & Giacomini, S. (2019). Degradation of microplastic seed film-coating fragments in soil. Chemosphere, 226, 645–650. https://doi.org/10.1016/j.chemosphere.2019.03.161
- Ajeng, A. A., Abdullah, R., Ling, T. C., Ismail, S., Lau, B. F., Ong, H. C., Chew, K. W., Show, P. L., & Chang, J. S. (2020). Bioformulation of biochar as a potential inoculant carrier for sustainable agriculture. Environmental Technology & Innovation, 20, 101168. https://doi.org/10.1016/j.eti.2020.101168
- Cordova-Tellez, L., & Burris, J. S. (2002). Embryo drying rates during the acquisition of desiccation tolerance in maize seed. Crop Science, 42(6), 1989–1995. https://doi.org/10.2135/cropsci2002.1989
- Das, S. K., Ghosh, G. K., & Avasthe, R. (2021). Applications of biomass derived biochar in modern science and technology. Environmental Technology & Innovation, 21, 101306. https://doi.org/10.1016/j.eti.2020.101306
- Ellis, R. H., & Roberts, E. H. (1980). Improved equations for the prediction of seed longevity. Annals of Botany, 45(1), 13–30. https://doi.org/10.1093/oxfordjournals.aob.a085797
- Fouché, T., Claassens, S., & Maboeta, M. (2020). Aflatoxins in the soil ecosystem: An overview of its occurrence, fate, effects and future perspectives. Mycotoxin Research, 36(3), 303–309. https://doi.org/10.1007/s12550-020-00393-w
- Głodowska, M., Husk, B., Schwinghamer, T., & Smith, D. (2016). Biochar is a growth-promoting alternative to peat moss for the inoculation of corn with a pseudomonad. Agronomy for Sustainable Development, 36(1), 21. https://doi.org/10.1007/s13593-016-0356-z
- Gray, A., Kuffel, A., & Daeid, N. N. (2023). An improved rapid method for DNA recovery from cotton swabs. Forensic Science International Genetics, 64, 102848. https://doi.org/10.1016/j.fsigen.2023.102848
- Hammerschmiedt, T., Holatko, J., Pecina, V., Huska, D., Latal, O., Kintl, A., Radziemska, M., Muhammad, S., Gusiatin, Z. M., Kolackova, M., Nasir, M., Baltazar, T., Ahmed, N., & Brtnicky, M. (2021). Assessing the potential of biochar aged by humic substances to enhance plant growth and soil biological activity. Chemical and Biological Technologies in Agriculture, 8(1), 46. https://doi.org/10.1186/s40538-021-00242-7
- Hill, R. A., Hunt, J., Sanders, E., Tran, M., Burk, G. A., Mlsna, T. E., & Fitzkee, N. C. (2019). Effect of biochar on microbial growth: A Metabolomics and BactEriological InvEstigation in E. coli. Environmental Science & Technology, 53(5), 2635–2646. https://doi.org/10.1021/acs.est.8b05024
- Hitaj, C., Smith, D. J., Code, A., Wechsler, S., Esker, P. D., & Douglas, M. R. (2020). Sowing uncertainty: What we do and don’t know about the planting of pesticide-treated seed. BioScience, 70(5), 390–403. https://doi.org/10.1093/biosci/biaa019
- Horn, B. W., & Dorner, J. W. (1998). Soil populations of Aspergillus species from section flavi along a transect through peanut-growing regions of the United States. Mycologia, 90(5), 767–776. https://doi.org/10.1080/00275514.1998.12026969
- Jeffree, C. E. (2006). The fine structure of the plant cuticle. In M. Riederer & C. Müller (Eds.), Biol plant cuticle (pp. 11–125). Blackwell Publishing Ltd. https://doi.org/10.1002/9780470988718.ch2
- Juraschek, L. M., Kappenberg, A., & Amelung, W. (2022). Mycotoxins in soil and environment. The Science of the Total Environment, 814, 152425. https://doi.org/10.1016/j.scitotenv.2021.152425
- Krupke, C. H., Hunt, G. J., Eitzer, B. D., Andino, G., Given, K., & Smagghe, G. (2012). Multiple routes of pesticide exposure for honey bees living near agricultural fields. PLoS ONE, 7(1), e29268. https://doi.org/10.1371/journal.pone.0029268
- Law, Y. K., Lee, C. K. F., Pang, C. C., Hau, B. C. H., & Wu, J. (2023). Vegetation regeneration on natural terrain landslides in Hong Kong: Direct seeding of native species as a restoration tool. Land Degradation & Development, 34(3), 751–762. https://doi.org/10.1002/ldr.4492
- Lehmann, J. (2007). A handful of carbon. Nature, 447(7141), 143–144. https://doi.org/10.1038/447143a
- Marris, E. (2006). Black is the new green. Nature, 442(7103), 624–626. https://doi.org/10.1038/442624a
- Ma, H., Shurigin, V., Jabborova, D., Dela Cruz, J. A., Dela Cruz, T. E., Wirth, S., Bellingrath-Kimura, S. D., & Egamberdieva, D. (2022). The integrated effect of microbial inoculants and biochar types on soil biological properties, and plant growth of lettuce (Lactuca sativa L.). Plants, 11(3), 423. https://doi.org/10.3390/plants11030423
- McGee, D. C. (1988). Maize diseases: A reference source for seed technologists. APS Press.
- Nuyttens, D., Devarrewaere, W., Verboven, P., & Foqué, D. (2013). Pesticide-laden dust emission and drift from treated seeds during seed drilling: A review. Pest Management Science, 69(5), 564–575. https://doi.org/10.1002/ps.3485
- Rogovska, N., Laird, D., Cruse, R. M., Trabue, S., & Heaton, E. (2012). Germination tests for assessing biochar quality. Journal of Environmental Quality, 41(4), 1014–1022. https://doi.org/10.2134/jeq2011.0103
- Scheidegger, K. A., & Payne, G. A. (2003). Unlocking the secrets behind secondary metabolism: A review of Aspergillus flavus from pathogenicity to functional genomics. Journal of Toxicology Toxin Reviews, 22(2–3), 423–459. https://doi.org/10.1081/TXR-120024100
- Taylor, A. G., Min, T.-G., Harman, G. E., & Jin, X. (1991). Liquid coating formulation for the application of biological seed treatments of trichoderma harzianum. Biological Control, 1(1), 16–22. https://doi.org/10.1016/1049-9644(91)90096-I
- Wang, P., Shen, F., Xu, Y., Wang, X., Huang, H., Li, R., Liu, T., Guo, D., Du, J., Guo, Z., & Zhang, Z. (2022). Sustainable biochar effects on the remediation of contaminated soil: A 2-crop season site practice near a lead-zinc smelter in Feng County, China. Environmental Pollution, 302, 119095. https://doi.org/10.1016/j.envpol.2022.119095
- Wei, Z., Wang, J. J., Fultz, L. M., White, P., & Jeong, C. (2021). Application of biochar in estrogen hormone-contaminated and manure-affected soils: Impact on soil respiration, microbial community and enzyme activity. Chemosphere, 270, 128625. https://doi.org/10.1016/j.chemosphere.2020.128625
- Zhang, K., Khan, Z., Liu, J., Luo, T., Zhu, K., Hu, L., Bi, J., & Luo, L. (2022). Germination and growth performance of water-saving and drought-resistant rice enhanced by seed treatment with wood vinegar and biochar under dry direct-seeded system. Agronomy, 12(5), 1223. https://doi.org/10.3390/agronomy12051223
- Zhang, M., Zhuo, J. J., Wang, X., Wu, S., & Wang, X. (2010). Optimizing seed water content: Relevance to storage stability and molecular mobility. Journal of Integrative Plant Biology, 52(3), 324–331. https://doi.org/10.1111/j.1744-7909.2010.00916.x