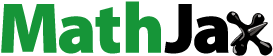
Abstract
Rice (Oryzae sativa L.) is one of the most important cereal crops on which an ever-increasing population is dependent for food globally. However, constraints associated with infectious diseases, notably brown spot transmitted by seed and wind reduced its production and productivity. This study was conducted to characterize Bipolaris oryzae isolates morphologically in Fogera plain, Amhara region of Ethiopia. Twenty-nine B. oryzae isolates were collected from Dera, Fogera, and Libokemkem districts and characterized morphologically at the Ambo Agricultural Research Center plant pathology laboratory. These isolates were grouped into nine categories on their colony morphology and growth pattern. About 34.48% of the isolates showed black with fluffy growth pattern, while 68.96% of the conidia shape were slightly curved. Moreover, 58.62% of 29 isolates was initially hyaline. Fast, moderate and slow mycelial growth habits were also observed in 29 isolates, from 3.07 to 15.49 μm in length and from 1.00 to 4.27 μm in breadth conidia size and from 1.52 × 109 to 1.80 × 1010/ml sporulation rates. Therefore, knowing such characteristics is crucial and it needs to identify the pathogen inherent genetic characteristics of the above isolates for fruitful management recommendations.
Reviewing Editor:
Subjects:
Introduction
Rice (Oryza sativa L.) is an important staple food crop for people on this planet (Martin, Citation2015). Rice is an important food source in Eastern, Central, and Southern Africa. It is the second most common staple food in East Africa after corn (Martin, Citation2015). It is also a vital food security commodity in Ethiopia, and its growth has brought about major changes in farmers’ livelihoods, creating job opportunities for many people across different parts of the country (Dawit & Thompson, Citation2020). In the Fogera Plain of the Amhara region, rice plays an important role in abating the problem of food insecurity in the farming community and is consumed as enjera, tela, and (Tilahun et al., Citation2018). Fogera district is one of the potential areas for rice production in Ethiopia, with an estimated contribution of 42% to the national rice production (Tamirat & Jember, Citation2017).
However, biotic, abiotic (salinity, water stress, temperature, soil nutrient imbalance, etc), technical, and socioeconomic factors, either alone or in combination, restrict the adoption of improved rice production technologies and create hurdles in obtaining higher potential yields (Fahad et al., Citation2019; Laha et al., Citation2017). The presence of diseases, insects, and weed infestation, and the lack of resistant varieties have significantly contributed to decreased rice yield and quality in Ethiopia (Laha et al., Citation2017; Teshome & Alemu, Citation2011). In rice production, diseases caused by fungi such as rice blast, brown spot, and sheath rot, are major drawbacks, resulting in quantitative and qualitative losses of the harvested products.
A brown spot is a soil, seed, and airborne (Laha et al., Citation2017; Martin, Citation2015), and is known to cause both quantitative and qualitative losses (Hosagoudar et al., Citation2019; IRRI (International Rice Research Institute), Citation2013; Laha et al., Citation2017). It is widely distributed across all rice-growing areas worldwide (Aryal et al., Citation2016). Yield reduction caused by brown spot has been reported from 6 to 90% in Asia (Yosep et al., Citation2020), and a loss of 50–90% was recorded in Bengal (India), resulting in the death of two million people in India in 1942 (Aryal et al., Citation2016; Laha et al., Citation2017). Depending on the nature of the disease, stage of plant growth at the time of infection, variety tolerance, management, and weather conditions, the estimated losses can vary from 1 to 100% (Wasihun & Flagote, Citation2016). Based on the survey results, the brown spot is the second most common yield reducer next to blast among rice diseases today in Ethiopia (Tekalign et al., Citation2019; Wasihun & Flagote, Citation2016), and its severity was 14.4% in Fogera plain (Desalegn et al., Citation2019).
Studies to determine the status of rice diseases in Ethiopia have revealed that blast, brown spot, sheath rot, sheath blight, rice yellow mottle virus, and sheath brown spot are important in this order. These studies reported that brown spot prevalence, incidence, and severity were 34, 40.25, and 8.57%, respectively (Tekalign et al., Citation2019). In addition, fungicides and varieties have been screened for brown spot disease in rice ecosystems (Tekalign et al., Citation2019; Wasihun & Flagote, Citation2016).
Morphological and genetic characterization of Bipolaris oryzae and the development of brown spot management options for Ethiopian rice production are the top priorities. Since the introduction and expansion of rice were recent, during the Derg regime in 1991 (Alemu et al., Citation2018), the research system did not bring an expected improvement in brown spot management. In rice disease management, identifying natural enemies/biological controls as well as determining the effect of nitrogen levels and other soil nutrients on rice diseases are other research areas for the future. Hence, the primary step is the characterization of the pathogen owing to its race dynamism, followed by the development of resistant genotypes. Rice pathogenic fungi species identification has paramount importance to differentiate their taxonomic relationships which can be used to study the genetic polymorphism at intraspecies level (Kumar et al., Citation2011; Sobanbabu et al., Citation2018).
Despite serious yield losses of rice caused by B. oryzae in the area, the absence of information on the aggressive pattern of B. oryzae isolates, epidemiology, race identification, virulence of different races of the pathogens, and resistance of lowland rice varieties or genotypes against rice brown spot, blast, and sheath rot are the gaps of rice protection to be addressed in Ethiopia (Tekalign et al., Citation2019). Hence, the characterization of B. oryzae for its phenotypic traits has to be addressed, and this information should be documented for further research in Ethiopia. Therefore, this study was conducted to morphologically characterize the isolates of B. oryzae pathogen in rice production hubs.
Materials and methodology
Collection of damaged plant samples
The sample collection was conducted in rice-growing areas of the South Gondar Administrative Zone in three districts, namely Dera, Libo-Kemkem, and Fogera districts, as shown in . Districts were chosen based on their production coverage and disease occurrence. In each district, rice-growing farming fields, including the Fogera National Rice Research and Training Center experimental site, were assessed randomly during 2019–2020. The assessment was performed at Shina-Tsion Kebele in Libo-Kemkem district; Abua-Kokit, Tiwa-Zakena, and Quhar-Michael Kebele (localities), as well as at the Fogera Rice Research and Training Center experiment field in Fogera district; and at Jigna and Zara localities in Dera district ().
Table 1. Description of collected B. oryzae isolates.
In each rice field, four diseased leaf samples were randomly collected during the heading and ripening stages of the rice plant. The sampled locations were 1–3 km apart, depending on the availability of the crop. The isolates used for cultural characterization of B. oryzae are listed in . Each leaf sample was labeled and placed on envelope paper, while the icebox was used to conserve and protect from desiccation during collection and transferred to the refrigerator until it was taken to the laboratory for processing. Twenty-nine brown spot infected samples were collected. Thirteen isolates were collected from Fogera (including two samples from the research station), 12 isolates from Dera, and four isolates from Libo-Kemkem districts. Morphological characterization was conducted at the Ambo Agricultural Research center, Plant Pathology Laboratory.
Experimental procedures
Media preparation, isolation and purification of B. oryzae isolates
Five spoons of PDA powder were added to 500 ml of distilled water and boiled while adding one tablet of CAPH (chloramphenicol 250 g) to avoid bacterial contamination. The boiled medium was autoclaved for 15 min, and then 15–20 ml of boiled medium was poured into washed and autoclaved Petri dishes. The plates with PDA were inverted in a hood after 30 min to avoid the formation of water vapor.
Fresh samples were collected from the Ambo Agricultural Research Center laboratory for B. oryzae isolation and morphological characterization. The infected leaf samples along with the green portion were cut into five small pieces, rinsed in 70% alcohol and 30% sterilized distilled water, and then transferred to a plate that had sterilized distilled water to remove unwanted impurities (Monisha et al., Citation2019) (). The leaf bits were then placed on a blotter paper and air-dried to remove excess water from the surface. The leaf bits were picked with forceps and placed on labeled petri dishes containing PDA. The plates were sealed with Parafilm and transferred to an incubator.
The inoculated Petri Plates were incubated at 28 °C for four days. The actively growing mycelium was sub-cultured aseptically by taking a six mm2 mycelia disk using a cork borer and inverted to the other Petri Plate (Soni et al., Citation2015). The plates were then sealed, transferred to an incubator with a temperature adjusted at 28 °C, and incubated for 14 days.
Cultural characterization and identification of the pathogen
Bipolaris oryzae isolates were cultured on PDA medium to produce conidia. To confirm the identity of B. oryzae, the morphology of mycelia, conidia, presence of septation, and colour were observed under a compound microscope (Sobanbabu et al., Citation2018). All isolates were grouped based on their colony growth pattern and evaluated using various characters on 9 cm diameter PDA plates. The colony diameters of the isolates were measured with a caliper at three and seven-day intervals of incubation at 28 °C to characterize the pathogens growth pattern (Monisha et al., Citation2019; Valarmathi & Ladhalakshmi, Citation2018). Colony morphology, mycelial colour, sporulation, conidium size, colony shape, luster, and dry mycelial weight were recorded (Monisha et al., Citation2019).
The colony morphology (growth pattern) was identified based on the colony growth characters and the colours observed on PDA (Kumar et al., Citation2011; Meghana & Hiremath, Citation2019; Valarmathi & Ladhalakshmi, Citation2018). The plates and their colonies were then photographed using a smartphone camera (24 megapixels) after five days of growth (Valarmathi & Ladhalakshmi, Citation2018). Nine mm2 mycelial discs from 7 days old cultures was taken, the spore suspension was prepared using sterile distilled water () and examined under a compound microscope using a hemocytometer, and the number of conidia for each isolate was recorded. On the other hand, the ten days old culture mycelia were scraped and glass slides were prepared under aseptic conditions for spore size and shape measurement. This was done with the help of cellsens entry software and a camera-mounted Olympus compound microscope (Soni et al., Citation2015).
Spore size (μm)
The spore size of each isolate was measured using a camera-mounted Olympus compound microscope. The slides were thoroughly washed and autoclaved for 15 minutes. A 6 mm2 mycelial disc was obtained using a cork borer, and the mycelia were scraped with a needle to prepare a spore suspension (Kumar et al., Citation2011). The spore suspension was then dropped on the slides and covered with coverslips in a safety hood. The prepared slides were examined under compound microscope at the mycology laboratory of the Ambo Agricultural Research Center (AmARC). To determine the width and length, spores were visualized at 400× magnification under an Olympus compound microscope connected to a desktop computer, and measurements were made using the CellSens entry software. Five spores from each isolate were measured (Mahboubeh et al., Citation2017).
Sporulation conidia/ml
The number of spores was measured using a hemocytometer. A 9 mm2 mycelial disc was obtained using a cork borer, and the mycelia were scraped with a needle. The mycelia were gently mixed in 1000 µL of sterile distilled water, and the suspensions were then poured into small beakers and vortexed for 5 seconds. One microliter of the spore suspension was dropped on the counting chamber, filled slowly, spread without any overfill or underfill, and covered with a slip. Spores in each of the 0.1 mm3 corner squares were counted (Mahboubeh et al., Citation2017). The number of spores/µL was calculated using the following equation.
where n is the average cell count per square of the four corner squares.
Radial growth (mm)
The first measurement of colony radial growth was done after three days after inoculation, and later, the growth of the seventh-day-old culture was measured using a caliper. The radial growth of isolates on PDA was measured by the average rate of the X- and Y axis of each isolate from the first day after inoculation until maximum growth on the petri dishes (Mahboubeh et al., Citation2017).
Dry mycelial weight (mg)
Nine millimeter mycelia were taken from 14-day old culture and separated from the PDA. The mycelia of the fungus were harvested by draining the medium through a filter paper. The filter paper with the fungal mycelial mat was dried under fluorescent light for three days. After two days, the dry mycelial weight of B. oryzae was measured twice at 36 h intervals using a sensitive balance (Channakeshava & Pankaja, Citation2018).
Preservation of B. oryzae isolates
The isolated fungi were purified by subculturing the B. oryzae isolates on PDA plates. The seven days old pure cultures were placed in a laminar flow hood and the active growing mycelia were cut into smaller pieces using a laboratory needle. Preservation tubes with a diameter of 15 mm were used, one-third of which were filled with glycerol, and then the cut pieces of mycelia were inserted into the tubes in a slant position. The tubes were labeled, covered with Parafilm, placed together in a beaker, and transferred to a −23 °C freezer for a long period.
Data collection and analysis
The fungal colony morphology (growth pattern and colour), mycelial growth, colony margin/edge, colony growth habit, radial growth on the third and seventh days of incubation, luster character of the mycelia, conidia colour, shape, size, number of septations per conidium, sporulation of conidia per ml and dry mycelial weight were recorded. The isolates were clustered based on colony diameter (mm), conidia length (µm), conidia width (µm), and dry mycelial weight (mg) as well as the clustering image was created using SAS 9.4 version.
Results and discussion
Colony characterization of B. oryzae isolates
The morphology of the assessed B. oryzae isolates collected from the three districts varied, and different growth patterns were observed. Based on colony morphology, the 29 isolates of B. oryzae were grouped into nine categories. Isolates such as MCBO 4, MCBO 6B, MCBO 15 C, and MCBO 41B were grey coloured with suppressed colony growth; isolates MCBO 6 A, MCBO 14 A, MCBO 23B, and MCBO 39 A had grey-coloured colonies with suppressed and white spotted mycelial growth, whereas isolates MCBO 8, MCBO 9 A, MCBO 10B, MCBO14B, and MCBO 40B developed grey colour with fluffy cottony growth (). Similarly, MCBO 10 A, MCBO 10 C, MCBO 13 A, MCBO 15 A, MCBO 23 A, MCBO 32, MCBO 33, MCBO 38, MCBO 41 A, and MCBO 48 had black-coloured colonies with fluffy growth, whereas MCBO 6 C, MCBO 13B, and MCBO 39B developed black colonies with suppressed mycelial growth ().
Table 2. Groups of B. oryzae isolates by colony growth pattern and colour.
Approximately 34.48% of B. oryzae isolates were black in colony colour and fluffy in growth pattern, while 17.24% of tested isolates showed greyish fluffy with white cottony growth, whereas greyish suppressed and grey with fluffy white cottony mycelial growth were observed in 13.79% of isolates (). However, the percentage of yellowish-grey with suppressed and white cottony growth groups in B. oryzae isolates was 3.45. All isolates collected from the Libokemkem district showed moderate mycelial growth habits in colony lustre compared to isolates from other districts. Furthermore, 75% of the isolates from the studied locations were flat in mycelial growth and regular in colony margins, whereas 25% of them showed cottony growth as well as irregular in colony edge.
Most of these variable morphological patterns and colours of B. oryzae were similarly confirmed and reported by Valarmathi & Ladhalakshmi (Citation2018) (17 isolates grouped as: black with fluffy growth, grey with fluffy growth and white spots, grey with fluffy growth, and grey with suppressed growth). The three groups of growth patterns (greyish with cottony mycelium, a mixture of grey and white mycelium with fluffy growth, and greyish cottony mycelium with white spots) were divided by Monisha et al. (Citation2019), which confirmed the above categories. Kumar et al. (Citation2016) also grouped 116 isolates into black with suppressed growth, black with cottony growth, black with fluffy growth, grey with suppressed growth, grey with cottony growth, grey with fluffy growth, greyish white with cottony growth, and white with cottony growth morphology. However, two different colony colours and morphologies, yellowish coloured with suppressed growth and white coloured with cottony mycelial growth, were observed for the first time in this study, on isolates MCBO 15B and MCBO 9B, respectively ().
Based on mycelial growth morphology, the isolates were grouped into flat and cottony. Twenty-three isolates were cottony, seven of which had flat mycelial growth (). In contrast, three groups were observed for all isolates, based on their colony growth habits. Fourteen isolates showed fast growth habits, and three of them were covered with a 90 mm plates diameter after seven days of inoculation; 12 isolates had moderate growth habits, and the other three were slow in colony growth habits. The colony margin, or edge was also grouped into two groups: 20 isolates had regular shapes, and the remaining nine showed irregular margins.
Table 3. Morphological variability of B. oryzae isolates on artificial growth media.
Similarly, 58.33, 33.33, and 8.33% of isolates from the Fogera district were fast, moderate, and slow in mycelial growth habits, respectively (). In contrast to isolates from Libokemkem, 91.6% of the mycelial growth of isolates from Fogera localities were cottony except for one isolate which had flat growth. On the other hand, of the 13 isolates collected from the Dera district, seven, four, and two were fast, moderate, and slow mycelial growth habits, respectively. 84.62% of these isolates had also cottony growth, and 15.38% of them were flat. Thus, isolates of B. oryzae were collected from Fogera and Dera localities showed the fastest mycelial growth.
This result further supports a similar finding reported by Meghana and Hiremath (Citation2019), where out of 10 isolates were characterized, seven isolates were regular and three of them were irregular in the colony margin, while five were flat and the remaining were cottony in mycelial growth. Moreover, the lustre character of the isolate colonies was of two types: the colony of six isolates was shining, and 23 of them showed a dully white character. This was also stated in Kumar et al. (Citation2016). Kumar et al. (Citation2016) also observed fast, moderate, and slow-growing isolates ().
Conidial characterization of B. oryzae isolates
The mycelia of most isolates of B. oryzae initially appeared white and black in colour and septated, which later turned to grey and dark colour. The conidiophore arises singly or in a group, multi-septate, and is brown in colour-bearing conidia on the tips (). Approximately 58.62% of the 29 isolates initially had hyaline conidia colour, which upon maturity turned to brown and the remaining was light brown, whereas the conidia shape was curved or slightly curved, fusiform or obclavate, occasionally almost cylindrical, with one to five septa with a hilum at the base. The features observed in this study are in agreement with those reported in Meghana and Hiremath (Citation2019), Monisha et al. (Citation2019), and Valarmathi & Ladhalakshmi (Citation2018).
The conidia size varied from 3.07 to 15.49 μm in length and from 1.00 to 4.27 μm in breadth (). The maximum length of conidia was recorded for isolate MCBO 9B (15.49 μm) followed by MCBO 40 A (14. 36 μm) and MCBO 38 (14.26 μm), whereas the minimum was measured for MCBO 9 A (5.74 μm), MCBO 10B (4.96 μm) and MCBO 14B (3.07 μm). Based on conidia width, isolates MCBO 14B (1.00 μm), MCBO 10 C (1.18 μm), MCBO 15 A (1.27 μm), and MCBO 8 (1.88 μm) had the minimum measurement, while the highest breadth (4.21, 4.25 and 4.27 μm) were recorded for isolates MCBO 38, MCBO 4 and MCBO 40 A, respectively. The number of septations per conidium varied, and ranging from one to five (). The maximum number of septa per conidium was recorded for MCBO 6 C (4–5 septa), MCBO 10 C (4 septa), and MCBO 40 A (3–5 septa), whereas the minimum number of septa per conidium was recorded for isolate MCBO 14B (one-three septa).
Table 4. Characteristics of B. oryzae conidia and dry mycelial weight.
The number of spores for B. oryzae isolates varied from 1.52 × 109 to 1.80 × 1010/ml. The minimum number of spores (1.52 × 109 and 1.72 × 109/ml) were recorded from the Libokemkem district (Shina Tsion locality), while the maximum sporulation of conidia per ml was observed from the isolates collected from Jigna (Dera district) and Abuakokit (Fogera district) localities which were 1.80 × 1010 and 1.068 × 1010, respectively. Isolates of B. oryzae with grey colour, suppressed mycelial growth, and grey colour and suppressed mycelia with white spots were observed to have the maximum number of spores and had a light brown coloured and slightly curved conidia shape. The minimum spore number was also observed in isolates with grey-coloured mycelia and suppressed colony growth morphology. In the results of Meghana and Hiremath (Citation2019), the sporulation rate was between 3.75 × 109 and 6.25 × 109, which was similar to the findings of this study. This finding, however, was different from the results reported by Kumar et al. (Citation2016) and Soni et al. (Citation2015), which reported that sporulation ranged from 6.6 × 106 to 7.6 × 206.
The maximum mean dried mycelial weights of 81, 59.5, 56, 55, and 54.5 mg/9 mm mycelia were recorded from isolates MCBO 6 C, MCBO 8, MCBO 9B, MCBO 14B, and MCBO 23B, respectively. On the other hand, the minimum mean dry mycelial weight of 8.5, 12.5, 15, and 19 mg/9 mm mycelia were recorded, respectively, on isolates MCBO 32, MCBO 13 A, MCBO 39B, and MCBO 9 A (). This finding was also inconsistent with the report in Channakeshava and Pankaja (Citation2018), perhaps because the method used to measure the colony diameter varies.
The cultural morphology varied significantly among the isolates. Morphological analysis of B. oryzae isolates from the study areas in Ethiopia revealed variation in parameters such as colony growth habit, conidia and mycelia characteristics. Such variability among the collected isolates corresponds with the findings reported from similar work done elsewhere (Kumar et al., Citation2016; Meghana & Hiremath, Citation2019; Monisha et al., Citation2019; Valarmathi & Ladhalakshmi, Citation2018), although some isolates analyzed in the present study showed disparity and unique features that do not correspond with characteristics measured in works done elsewhere and cited in this manuscript ().
This variability could be due to the genetic diversity of rice varieties. It is also imperative to conduct further analyses using state-of-the-art technologies such as PCR, sequencing, electrophoresis, and advanced genetic diversity studies to substantiate the results of morpho-cultural observations. Furthermore, it seems logical to have a good insight into whether such variation may be associated with disease parameters such as severity, and hence, disease epidemic development.
Cluster analysis of B. oryzae isolates
Based on cluster analysis, the 29 isolates of B. oryzae were clustered into four groups (). The maximum number of clusters was recorded in cluster two (14) followed by cluster three (seven isolates). In contrast, the lowest number of isolates was obtained in a cluster of four (3 isolates) and one (5 isolates) (). Thus, 48.28% of B. oryzae isolates were clustered in cluster two. Moreover, cluster means revealed that the highest colony diameter was measured in cluster two and the lowest was recorded in cluster one ().
Table 5. Clusters of B. oryzae isolates collected from three districts based on morphological characters.
Isolate clusters also varied in conidial length and width. The maximum length of conidia was measured in clusters of four (11.60 µm) and one (11.44 µm), while the lowest measurement of conidia length was recorded in cluster two (8.68 µm). Similarly, the highest conidia breadth was recorded in cluster one (3.57 µm), and the narrowest width was observed in cluster four (2.41 µm). In addition, the isolates of B. oryzae were clustered into four groups based on their dry mycelial weight. Of 29 pure isolates, 10.34% (cluster 4) of them showed the maximum dry mycelial weight (63.83 mg), and 48.28% of isolates had 45.14 mg (cluster two) per 9 mm2 mycelia. Cluster 3 measured the lowest dry mycelia which were 15.14 mg per 9 mm2 of mycelia ().
Table 6. Cluster means of some morphological parameters of B. oryzae.
Finally, pure cultures of B. oryzae isolates were preserved in slants containing glycerol (). The mycelia of each isolate grown on PDA were cut into pieces, and transferred to two glycerol slants, and maintained at −23 °C for a long period of storage for later use, following the procedure and method of long-term preservation of plant pathogens.
Conclusion
In conclusion, brown spot management was truly achieved through the detailed characterization of B. oryzae and the design of the best control strategy. Nine categories of growth characteristics were observed in the 29 B. oryzae isolates. Approximately 34.48% of these isolates were black with fluffy growth, and 68.96% of the conidia shape were slightly curved. Furthermore, the conidia colour of approximately 58.62% of 29 isolates was initially hyaline and later on maturity turned to brown, and the remaining was light brown. Isolates also varied from 1.52 × 109 to 1.80 × 1010/ml in the production of spores. The variation between the 8.5 mg/9 mm mycelial disk and the 81 mg/9 mm mycelial disk of dry mycelial weight was observed. The preserved isolates of B. oryzae are highly important for further studies on molecular characterization of pathogens in the Fogera National Rice Research and Training Centre (FNRRTC).
Hence, these findings about the morphological characteristics of B. oryzae were not known before, and this widens the knowledge of Ethiopian pathologists to design an appropriate management strategy based on pathogen-specific characteristics. The development of resistant rice varieties against brown spot will also enable the management of the pathogen in an anaerobic ecosystem of rice production and is used as a source of host resistance in further breeding research.
Therefore, the pathogen characteristics of rice diseases should be similarly investigated because disease management is achieved through the primary characterization of a specific pathogen. However, the only morphological characterization of B. oryzae and other pathogens is not able to fully understand the pathogen, and it also needs to investigate its genetic diversity and population structure. Finally, for the complete characterization of B. oryzae, molecular technology should be employed to support the conventional diagnostic methods used and reliably reach the development of a reliable and positive identity of the pathogen.
Ethical approval
This study was evaluated by Bahir Dar University, which was approved by the university board examiners. The plant materials were collected based on the institutional and national ethical guidelines, and the isolates are preserved at Ambo Plant Pathology laboratory for further research works. This study has not been published in any other journal.
Author contributions
The authors contributed to the conception and design this study, analysis, and interpretation of the data. The draft and review of the manuscript was made by Muluadam Berhan Ejigu, Merkuz Abera, and Birhanu Bekele. The authors have also made substantive intellectual contributions to this manuscript and other papers. All authors have approved the final manuscript to be published and are also responsible for the accuracy, integrity, and originality of the study.
Acknowledgements
The authors would like to extend sincere gratitude to the Ethiopian Institute of Agricultural Research (EIAR), FNRRTC and Ambo Agricultural Research Centre (AmARC), respectively, for providing financial, logistics and laboratory support to conduct this work. We are also grateful to the staff members of the Plant Protection Department (Mr. Desalegn Yalew and Mr. Eniyew Nigatu) and the rice breeding program at FNRRTC for the material and technical support provided. Authors also acknowledge the Cogent Food and Agriculture (ORG) editorial office for the free language editing service. No additional information is available for this article. The authors declare that no funds, grants, or other support was received during the preparation of this manuscript.
Disclosure statement
No potential conflict of interest was reported by the author(s).
Data availability statement
The authors confirm that the data supporting the findings of this study are available within the article.
Additional information
Notes on contributors
Muluadam Berhan Ejigu
Muluadam Berhan Ejigu is an associate researcher in plant pathology with MSc degree in plant protection. I am interested on plant mycology research areas.
Merkuz Abera Adimasu
Merkuz Abera Adimasu (Proffesor in Plant Pathology) is advising PhD students in Bahir Dar University, and he is interested in biological sciences research.
Birhanu Bekele Feyissa
Birhanu Bekele Feyissa is a senior plant pathologist and has a PhD degree in plant pathology. He has a research interest on plant virology.
References
- Alemu, D., Tesfaye, A., Abebaw, A., Degu, A., Tilahun, T., & Thompson, J. (2018). A Historical Analysis of Rice Commercialization in Ethiopia: The Case of the Fogera Plain APRA Working Paper 18, Future Agricultures Consortium.
- Aryal, L., Bhattarai, G., Subedi, A., Subedi, M., Subedi, B., & Sah, G. K. (2016). Response of rice varieties to brown spot disease of rice at Paklihawa, Rupandehi. Global Journal of Biology, Agriculture and. Health Sciences, l.5(2):1–15.
- Channakeshava C. & Pankaja, N. S. (2018). Effect of media, temperature, light, pH and nutrient source on growth and development of B. oryzae causing brown leaf spot of paddy. International Journal of Current Microbiology and Applied Sciences, 7(07), 1713–1722. https://doi.org/10.20546/ijcmas.2018.707.203
- Dawit, A. & Thompson, J. (2020). The emerging importance of rice as a strategic crop in Ethiopia (Working Paper 44). Future Agricultures Consortium.
- Dawit, A., Tesfaye, A., Abebaw, A., Degu, A., & Tilahun T. Thompson, J. (2018). A historical analysis of rice commercialization in Ethiopia: The case of the Fogera Plain APRA working paper 18. Future Agricultures Consortium.
- Desalegn, Y., Muluadam, B., & Bayuh B. Dereje, B. (2019). Advances in rice research and development in Ethiopia. In Taye Tadesse, Mulugeta Atnaf, Dawit Alemu, Tilahun Tadesse, & Kiyoshi Shiratori (Eds.), Rice diseases and insect pests in Ethiopia (pp. 159–170). EIAR.
- Fahad, S., Noor, A. M., Arif, M., Alam, M., Khan, M., Ullah, I. A., Wahid, H., Mian, F., Jamal, I. A., Basir, Y., Hassan, A., Saud, S., Amanullah, S., Riaz, M., Wu, C., Khan, M. A., & Wang, D. (2019). Major constraints for global rice production. 1–22. https://doi.org/10.1016/B978-0-12-814332-2.00001-0.
- Hosagoudar, G. N., Shaiah, S., S. Kovi, B., & Babu, B. S. U. (2019). Evaluation of host plant resistance for blast and brown spot diseases of paddy in hill zone of Karnataka, India. International Journal of Current Microbiology and Applied Sciences, 8(03), 1294–1304. https://doi.org/10.20546/ijcmas.2019.803.153
- IRRI (International Rice Research Institute). (2013). Standard evaluation system for rice (SES) (5th edition, p. 56). International Rice Research Institute.
- Kumar, A., Ishwar, S. S., Jameel, A., & Vishal, G. (2016). Morpho-molecular diversity of B. oryzae causing brown spot of paddy. Indian Journal of Agricultural Sciences, 86(5), 615–620.
- Kumar, P., Vaishali, A., & Sundeep, K. (2011). Morpho-pathological and molecular characterization of B. oryzae in rice (Oryzae sativa). Journal of Phytopathology, 159(1), 51–56. https://doi.org/10.1111/j.1439-0434.2010.01726.x
- Laha, G. S., Singh, R., Ladhalakshmi, D., Sunder, S., Srinivas Prasad, M., Dagar, C. S., & Ravindra Babu, V. (2017). Importance and management of rice diseases: A global perspective. In S. C. Bhagirath, J. Khawar, M. Gulshan (Eds.), Rice production worldwide (pp. 303–360). Springer International Publishing AG. https://doi.org/10.1007/978-3-319-47516-5_13
- Mahboubeh, M., Yassoralipour, A., Tong, P. E., Abidin, Z. M. A., & Mui, W. Y. (2017). Morphological and molecular characterizations of rice blast fungus, Magnaporthe oryzae. Pakistan Journal of Agricultural Sciences, 54(4), 785–792.
- Martin, M. M. (2015). Inheritance of resistance to brown spot disease in upland rice in Uganda. A thesis submitted to the Directorate of Research and Graduate Training in partial fulfillment of the requirements for the award of the degree of master of science in plant breeding and seed systems of Makerere University [Thesis] (pp. 18–20). Makerere University.
- Meghana, S. N., & Hiremath, S. V. (2019). Cultural, morphological and molecular characterization of B. oryzae causing brown leaf spot of rice in Northern Karnataka. Journal of Pharmacognosy and Phytochemistry, 8(2), 1235–1239.
- Monisha, S., Praveen, N. M., & Ramanathan, A. (2019). Isolation, characterization and management of brown spot disease of rice. Journal of Pharmacognosy and Phytochemistry, 8(3), 4539–4545.
- Sobanbabu, G., Sabarinathan, K. G., Parthiban, V. K., & Ramamoorthy, V. (2018). Isolation, screening and identification of virulent Isolates of B. oryzae causing rice brown spot and Sarocladium oryzae causing sheath rot disease. International Journal of Current Microbiology and Applied Sciences, 7(09), 930–939. https://doi.org/10.20546/ijcmas.2018.709.112
- Soni, K., Atul, K., & Santwana, R. (2015). Morphological characterization of B. oryzae causing brown spot of paddy in Bihar. International Education and Research Journal, 1, 85–87.
- Tamirat, B., & Jember, T. (2017). Review on adoption, trend, potential, and constraints of rice production to livelihood in Ethiopia. International Journal of Research – Granthaalayah, 5(6), 644–658. https://doi.org/10.5281/zenodo.824116
- Tekalign, Z., Muluadam, B., & Wubneh, A. (2019). Survey and identification of rice diseases in South Gondar Zone, Amhara Region, Ethiopia. Journal of Agriculture and Crops, 5(58), 123–131. https://doi.org/10.32861/jac.58.123.131
- Teshome N. & Alemu, D. (2011). An overview of national rice research and development strategy and its implementation. FRG II project. In A. Kebebew, A. Dawit, S. Kiyoshi, & K. Abebe (Eds.), Empowering farmers innovation (vol. 2, pp. 1–2). Oromia Bureau of Finance and Economic Development, Ethiopian Institute of Agricultural Research, Addis Ababa, Ethiopia. FRG II Project; Empowering Farmers Innovation.
- Tilahun, T., Abebaw, A., & Dawit, A. (2018). Ethiopia: Enriching livelihoods with rice research. https://www.future-agricultures.org/blog/ethiopia-enriching-livelihoods-with-rice, retrieved on June 18, 2020.
- Valarmathi, P., & Ladhalakshmi, D. (2018). Morphological characterization of B. oryzae causing brown spot disease of rice. International Journal of Current Microbiology and Applied Sciences, 7(2), 161–170. https://doi.org/10.20546/ijcmas.2018.702.021
- Wasihun Y., & Flagote, A. (2016). Alemu assessment of diseases on rice (Oriza sativa L.) in major growing fields of Pawe district, Northwestern Ethiopia. Wireless Sensor Network, 42, 13–23.
- Yosep, S. M., Ndiwa, A. S. S., & Shirly, S. O. (2020). Brown spot disease severity, yield and yield loss relationships in pigmented upland rice cultivars from East Nusa Tenggara, Indonesia. Biodiversitas Journal of Biological Diversity, 21(4), 1625–1634. https://doi.org/10.13057/biodiv/d210443