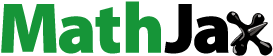
Abstract
The purpose of this study was to evaluate the effects of mulberry leaf powder (MLP) supplementation on the growth performance, serum biochemical markers, antioxidative capacity, and meat quality in Zhedong White Geese. With a focus on identifying the most beneficial MLP dosage, the study aimed at examining its capacity to improve poultry health and meat characteristics, supporting sustainable and health-oriented approaches to poultry farming. In this study, we allocated 240 healthy, 35-day-old Zhedong White Geese into four groups to examine the effects of differential MLP dosages (0%, 1.50%, 2.25%, and 3.00%) incorporated into their diets. Each groups consisted of six replicates, each containing ten geese, each group consisted of an equal number of male and female geese to ensure sex parity. The investigation focused on evaluating growth performance indicators, conducting serum biochemical analyses, assessing antioxidative capacities, and determining meat quality. The findings revealed that geese in the 1.50% and 2.25% MLP groups exhibited marked improvements in final body weight (FBW) and average daily gain (ADG) compared to the control group (p < 0.01). However, there was no significant difference in ADG between the control group and the 2.25% MLP group. Although feed-to-gain ratio (F/G) in these groups showed positive trends, they did not achieve statistical significance (p > 0.05). Serum analyses showed a significant upsurge in total superoxide dismutase (T-SOD) levels for the MLP-supplemented groups (p < 0.05), indicating enhanced antioxidative capacity. Furthermore, carcass trait evaluation demonstrated a significant increase in breast muscle yield (p < 0.05) and a decrease in shear force (p < 0.01) at 1.50% MLP supplementation. Texture Profile Analysis (TPA) showed significant ameliorations in the textural attributes of breast muscle, notably as reduced hardness and chewiness, at the 1.50% MLP dosage (p < 0.05). In contrast, the 3.00% MLP supplementation group exhibited a significant decrease in final body weight (FBW) and average daily gain (ADG) (p < 0.05), indicating that a higher dose of mulberry leaf powder may negatively impact growth performance and did not yield significant improvements in muscle texture (p > 0.05). To summarize, the study suggests that MLP supplementation, particularly within the 1.50% to 2.25% range, substantially bolsters growth performance and meat quality in Zhedong White Geese, with 1.50% MLP dosage being especially efficacious in refining muscle texture.
Introduction
The Zhedong White Goose, a breed characterized by its flavorful meat and graceful visage, originates from the southeastern coastal terrains of Zhejiang Province, China. Despite its popularity, breeding and feeding practices have not yet maximized its growth potential fully (Yang et al., Citation2023). As consumer demand for high-quality Zhedong White Goose meat escalates, the exploration and integration of novel, plant-based feed solutions are imperative to enhance growth performance and meat quality, thereby aligning with market needs and propelling the industry forward.
Emerging plant-based feeds, recognized for their sustainability and nutritional efficiency, are attracting significant interest. Among these, the mulberry leaf stands out as a ‘vegetal nutrient reservoir’, with a notable protein content exceeding 20%, approximately 3% fat content, and high digestibility. It is abundant in essential amino acids, vitamins, and minerals vital for animal growth and development (Srivastava et al., Citation2006). Additionally, mulberry leaves are rich in various bioactive substances such as flavonoids, alkaloids, γ-aminobutyric acid, and polysaccharides, which may play a potential role in enhancing antioxidant capacity, intestinal function, and immune regulation (Yu et al., Citation2018). Due to their rich nutritional and bioactive profiles, the use of mulberry leaves in livestock is gradually increasing, potentially improving animals’ antioxidative capacity, immune function, and gut health, thereby possibly affecting poultry growth performance and meat quality. For example, research by Ouyang et al. (Citation2020) indicated that incorporating mulberry leaf powder (MLP) into the diet of lambs improved blood metabolites and meat quality while maintaining growth and slaughter performance. Çelebi (Citation2024) demonstrated that black mulberry leaf extract significantly enhanced the microbial quality, lipid stability, and sensory attributes of raw chicken meat, extending its shelf life and indicating its potential as a natural antioxidant and antimicrobial agent in poultry meat products. Studies by Wang et al. (Citation2022) showed that adding 8% MLP to Tibetan pig diets significantly enhanced serum antioxidant levels, increased the lean meat percentage, and improved muscle fiber morphology. Research by So-In and Sunthamala (Citation2022) revealed that incorporating 10% MLP into broiler diets under high-density farming significantly enhanced growth performance, reduced blood lipid peroxidation levels, and increased levels of superoxide dismutase and catalase. While mulberry leaves have demonstrated p8romising effects in the livestock industry, their excessive application may adversely impact animal growth. Research conducted by Hou et al. (Citation2020) revealed that incorporating 5% to 10% of MLP into rabbit diets did not negatively affect their growth performance. However, supplementation exceeding 15% led to a reduction in the average daily weight gain of rabbits and an increase in the feed-to-growth ratio. Studies have indicated that adding 2% MLP to piglet diets does not produce adverse effects and can enhance serum metabolites and antioxidative capacity (SOD). Yet, concentrations beyond 6% were found to negatively impact growth performance (Ma et al., Citation2023).
Despite the exploration of these potential benefits in various studies, reports on the effects and mechanisms of mulberry leaves in Zhedong White Goose diets remain limited. This investigation aims to systematically assess the role of MLP supplementation in the dietary regimen of the Zhedong White Goose, focusing on growth, metabolic health, and meat quality. The findings are expected to provide a scientific basis for the feed formulation of the Zhedong White Goose and offer a new perspective on the application of mulberry leaves in poultry production, contributing to the sustainable development of the poultry industry and enriching the research on the application of mulberry leaves in animal nutrition.
Materials and methods
In this study, all procedures involving the handling and slaughter of Zhedong geese were conducted in accordance with the guidelines and regulations approved by the Shanghai Science and Technology Commission (STCSM), license number SYXK (HU): 2015-0007. Prior to slaughter, geese were anesthetized using pentobarbital sodium at a dosage of 40 mg/kg body weight, administered through intravenous injection. The geese were closely monitored to ensure they were fully anesthetized and insensible to pain. Once confirmed, euthanasia was performed using cervical dislocation by trained personnel. The absence of vital signs was confirmed post-procedure. All personnel were trained according to these guidelines, ensuring the procedures were conducted humanely and ethically.
Geese and housing
This investigation included 240 35-day-old old Zhedong White Geese that we accommodated, inside a barn, in enclosures with dimensions of 2 meters by 2 meters and a height of 0.8 meters, outfitted with adequate feeders and water troughs to permit free access to sustenance and hydration. The facility was equipped with an advanced temperature and humidity control system, ensuring the maintenance of an ambient temperature ranging from 16 to 22°Celsius and humidity levels maintained between 50% and 65%. This setup was designed to foster an ideal growth milieu for the geese. The comprehensive study spanned seven weeks and was conducted at the Zhuanghang Comprehensive Experimental Station under the aegis of the Shanghai Academy of Agricultural Sciences. Here, stringent immunization and disease prevention measures were adhered to in alignment with the station’s established protocols.
Experiment design and management of treatments
For this study, 240 Zhedong White geese were procured from Xiangtian Swan Family Farm in Hexian (Ma’anshan City, Anhui, China). Mulberry leaf powder was supplied by Bozhou Hongshuo Biotechnology Co., Ltd. (Bozhou City, Anhui, China). Before the experiment, each goose was weighed and randomly assigned to one of four treatment groups, with each group consisting of six replicates of ten geese each (equal numbers of males and females). The analysis of the crude protein (CP) in the MLP was conducted following the procedures outlined by the Association of Official Analytical Chemists (AOAC, Citation2005), while the determination of metabolizable energy (ME) was performed as described by Miller and Judd (Citation1984). Crude fiber (CF) analysis followed the AOAC (Citation2000) procedures, ether extract (EE) followed the AOAC (Citation1930) procedures, calcium (Ca) was analyzed as per AOAC (Citation1985a), and phosphorus (P) was determined following AOAC (Citation1985b) methods. The dietary regimes for each group were meticulously formulated, taking into account the nutritional profile of MLP and its inclusion rates, ensuring adherence to the National Research Council’s (NRC, Citation1994) nutrition standards for geese. The control group was provided with a basal diet, whereas the experimental groups were supplemented with feeds incorporating 1.50%, 2.25%, and 3.00% MLP, respectively. Detailed information regarding the nutritional composition of the diets for each treatment group is presented in and .
Table 1. Ingredients and nutrient compositions of experimental diets (air dry basis).
Table 2. Ingredient composition of mulberry leaf powder (MLP).
Growth performance and relative organ weight
At the beginning (aged three weeks) and end (aged ten weeks) of the study, each Zhedong White goose, following an eight-hour fasting, was weighed to determine the initial (IBW) and final body weights (FBW). We calculated the average daily weight gain (ADG) for each geese. Throughout the study, we meticulously tracked feed intake for each group to ascertain the average daily feed intake (ADFI) and the efficiency of feed conversion into body mass, denoted as the feed-to-gain ratio (F/G). At the end of the experiment, two geese with comparable weights from each group were carefully selected and humanely euthanized. Vital organs, including the heart, liver, spleen, bursa of Fabricius, and abdominal fat, were precisely dissected and weighed, with their weights expressed as a percentage of the total body weight. The mathematical expressions used to quantify growth performance metrics are as follows:
The formula for calculating the relative organ weight is:
Serum biochemical parameters
At the end of the experiment, two Zhedong White geese of comparable weights were meticulously selected from each experimental group. Following a 12-hour fast, 5 mL of blood was gently extracted from the wing vein for the purpose of serum isolation and subsequent biochemical evaluation. These serum specimens were then entrusted to Shanghai Pinyi Biotechnology Co., Ltd. for analytical processing. The array of biochemical indices assessed included alanine transaminase (ALT), aspartate transaminase (AST), total protein (TP), albumin (ALB), globulin (GLB), blood urea nitrogen (BUN), glucose (GLU), total cholesterol (TC), total triglycerides (TG), high-density lipoprotein cholesterol (HDL-C), and low-density lipoprotein cholesterol (LDL-C). The biochemical parameters were determined using an automatic biochemical analyzer (HITACHI 7180, Hitachi, Japan) according to the manufacturer’s instructions.
Serum antioxidant parameters
The evaluation of serum antioxidant markers in the Zhedong White geese was conducted by Shanghai Renjie Biotechnology Co., Ltd. This comprehensive analysis encompassed the measurement of key antioxidative agents, namely total superoxide dismutase (T-SOD), malondialdehyde (MDA), and glutathione peroxidase (GSH-PX) within the serum. T-SOD and GSH-PX activities were measured using commercial assay kits (Nanjing Jiancheng Bioengineering Institute, Nanjing, China) according to the manufacturer’s protocols. MDA levels were determined using the thiobarbituric acid reactive substances (TBARS) assay. All antioxidant parameters were measured using the DENLEY DRAGON Wellscan MK 3 microplate reader (Thermo Fisher Scientific, Finland).
Meat nutrients analysis
At the end of the experiment, two geese with comparable weights from each group were carefully selected and humanely euthanized according to established animal welfare guidelines to minimize stress and suffering. From each goose, samples were collected from the left pectoral major and leg muscles. These samples underwent homogenization in a tissue disruptor to yield a uniform muscle tissue blend. Subsequently, the resultant homogenates were carefully deposited into designated containers for subsequent nutritional analysis. The analysis focused on determining the levels of crude protein (CP), moisture content (moisture), and ether extract (EE) in the muscle tissues, employing the advanced FoodScan meat analyzer (FOSS Denmark). The FoodScan Meat Analyzer utilizes near-infrared (NIR) spectroscopy to provide rapid and accurate measurements of these parameters.
Meat quality traits and texture profile analysis (TPA)
Following the processing of the geese, the right pectoral and thigh muscles were meticulously excised and weighed to ascertain the muscle mass proportion. Uniform samples, each measuring 3.5 cm × 3.5 cm, were subjected to boiling for one minute, subsequently undergoing comprehensive texture analysis. This assessment was conducted using the cutting-edge TA. new plus texture analyzer, provided by Shanghai Ruiwen Intelligent Technology Co., Ltd., to evaluate multiple textural properties including sample height (the thickness of the muscle sample), hardness, adhesiveness, springiness, chewiness, gumminess, and cohesiveness. Furthermore, sections of the breast and thigh muscles underwent water loss rate evaluation employing the YYW-2 strain-controlled unconfined pressure instrument from Nanjing Soil Instrument Factory. Shear force measurements were facilitated by the RH-N50 muscle tenderness tester from Nanjing Mingao Instrument Equipment Co., Ltd. The electrical conductivity of the meat was determined using the LF-STAR carcass meat quality electrical conductivity meter from MATTHAUS, Germany. The calculation of muscle mass proportion and water loss rate was guided by the following formulas:
The formulas for calculating muscle percentage and water loss rate are as follows:
Statistical analysis
The experimental data were processed using Microsoft Excel 2003 (Redmond, Washington, USA). Statistical analysis was conducted using SPSS software version 19.0 (Chicago, IL, USA). The data were analyzed using one-way analysis of variance (ANOVA) to determine the effect of different levels of MLP supplementation on various measured parameters. Descriptive statistics, including mean and standard error of the mean (SEM), were calculated for each treatment group. The Shapiro-Wilk test was used to assess the normality of data distributions, and Levene’s test verified the homogeneity of variances. When ANOVA indicated significant differences (p < 0.05), Duncan’s multiple range test was used as a post-hoc analysis to identify specific group differences. The results are reported as mean ± SEM, with a P-value of less than 0.05 considered statistically significant. The data were modeled using the following equation:
Where:
= dependent variable,
= overall mean value
= fixed effect of the jth of
factor
= residual value from unpredictable error.
Results
Growth performance
The results on growth performance are presented in . There was no significant difference in the initial body weights of the Zhedong White geese between the control group and various MLP treatment groups at the beginning of the experiment (p > 0.05). The FBW of geese in the 1.50% and 2.25% MLP groups were significantly higher than that of the control group (p < 0.01). Compared to the 1.50% MLP group, the FBW of the 3.00% MLP group was significantly lower (p < 0.05). The ADG of the 1.50% MLP group was significantly better than that of the control group and the 3.00% MLP group (p < 0.05). The ADFI of all MLP supplemented groups was significantly higher than that of the control group (p < 0.01). Although the F/G in the 1.50% and 2.25% MLP groups improved by 8.31% and 5.00% respectively, compared to the control group, these differences did not reach statistical significance (p > 0.05).
Table 3. Effects of different levels of mulberry leaf powder (MLP) supplementation in diets on growth performance of Zhedong white geese.
Relative organ weight
The results of the relative organ weights are presented in . No significant differences were observed in the relative weights of the heart, spleen, bursa of Fabricius, and abdominal fat among all treatment groups (p > 0.05). However, a significant reduction in the relative liver weight was noted in groups supplemented with 1.50% and 2.25% MLP compared to the control group (p < 0.05).
Table 4. Effects of different levels of mulberry leaf powder (MLP) supplementation in diets on relative organ weights of Zhedong white geese.
Serum biochemical parameters
The results of the serum biochemical analysis, as presented in , reveal no significant differences in the serum levels of ALT, AST, TP, ALB, GLOB, ALB/GLB ratio, ALP, BUN, Glu, TC, HDL-c, and LDL-c among the various treatment groups (p > 0.05). However, the level of TG in the serum of geese from the 3.00% MLP group was significantly lower compared to other treatment groups (p < 0.01).
Table 5. Effects of adding different proportions of mulberry leaf powder (MLP) in diet on serum biochemical indexes of Zhedong white geese.
Table 6. Effects of dietary supplementation with different proportions of mulberry leaf powder (MLP) on the proportion of muscle nutrients in Zhedong White geese.
Nutritional composition of goose muscles
As shown in , the analysis of the nutritional composition, including CP, moisture content and EE of the breast muscle and leg muscles in Zhedong White geese showed no significant differences across the control and MLP-supplemented groups (p > 0.05).
Oxidative stability and antioxidant levels in goose serum
The antioxidant results of goose serum are illustrated in . Compared with the control group, dietary supplementation with MLP significantly elevated the levels of T-SOD in goose serum (p < 0.05). No significant differences were observed in the levels of MDA and GSH-Px in goose serum (p > 0.05).
Meat quality
The results pertaining to meat quality are depicted in . Dietary supplementation with 1.50% MLP significantly enhanced the percentage of breast muscle in geese compared to the control group (p < 0.05), while the inclusion of 1.50% and 2.25% MLP led to a significant decrease in the percentage of leg muscle in geese (p < 0.01). The supplementation of MLP had no significant effect on the water holding capacity of either breast or leg muscles in geese (p > 0.05). The electrical conductivity of leg muscles in geese from the 2.25% and 3.00% MLP groups was significantly higher than that in the 1.50% MLP group (p < 0.05), showing increases of 20.16% and 13.43% respectively, compared to the control group, though these differences were not statistically significant (p > 0.05). The dietary inclusion of MLP reduced the shear force of breast muscle in geese, with the 1.50% and 3.00% MLP groups showing a significant decrease in breast muscle shear force compared to the control group (p < 0.01), but no significant effect of MLP on the shear force of leg muscles was observed (p > 0.05).
Texture profile analysis
As illustrated in , the analysis of the textural properties of goose muscles revealed a biphasic response in the hardness of breast muscles to increasing dietary MLP levels, characterized by an initial decrease followed by an elevation. Specifically, the hardness of the breast muscles in the groups supplemented with 1.50% and 2.25% MLP was significantly lower than that observed in the control group (p < 0.05), whereas the group supplemented with 3.00% MLP did not exhibit a statistically significant difference in comparison to the control group (p > 0.05). In contrast, the hardness of leg muscles displayed an opposite trend, initially increasing and then decreasing with higher MLP supplementation. The leg muscles in the 1.50% MLP supplementation group demonstrated significantly higher hardness compared to the control group (p < 0.05), while the 2.25% and 3.00% MLP supplementation groups showed no significant differences (p > 0.05). Furthermore, the chewiness of breast muscles also exhibited a similar biphasic pattern with MLP supplementation, where the 1.50% MLP group presented significantly lower chewiness compared to both the control and 3.00% MLP groups (p < 0.05). However, the chewiness of leg muscles was not significantly influenced by the MLP supplementation (p > 0.05). The gumminess of breast muscles followed a comparable biphasic response to MLP supplementation, with the 1.50% MLP group showing significantly reduced gumminess compared to the control and 3.00% MLP groups (p < 0.05). The MLP supplementation did not significantly impact the gumminess of leg muscles (p > 0.05). Adhesiveness in breast muscles demonstrated a decrease followed by an increase with higher MLP levels, where the 1.50% MLP group had significantly lower adhesiveness than the control and 3.00% MLP groups (p < 0.05). The adhesiveness of leg muscles was not significantly affected by MLP supplementation (p > 0.05). The supplementation of MLP did not exert a significant impact on the springiness of both breast and leg muscles (p > 0.05). The cohesiveness of breast muscles displayed a decrease followed by an increase with MLP supplementation, with the cohesiveness in the 1.50% and 2.25% MLP groups being significantly lower than that in the control groups (p < 0.05). The cohesiveness of leg muscles was not significantly affected by the levels of MLP supplementation (p > 0.05).
Discussion
In recent years, mulberry leaves have emerged as a focal point in animal nutrition research, attributed to their rich nutritional profile and potential health benefits. Extensive investigations have demonstrated the efficacy of incorporating mulberry leaves as a feed additive in livestock and poultry production. Our study revealed that geese supplemented with 1.50% and 2.25% MLP exhibited significant enhancements in final body weight compared to the control group devoid of MLP supplementation. This finding aligns with that presented by Ding et al. (Citation2021), who observed that fermented MLP improved growth performance and meat quality in broiler chickens, thereby underscoring the positive role of MLP in growth promotion. Moreover, the ADFI across all MLP-supplemented groups significantly exceeded that of the control group, likely due to the augmented nutritional value and palatability imparted by the MLP, which in turn stimulated appetite. This observation was corroborated by the findings of Huyen et al. (Citation2012). Although improvements in the feed conversion ratio (F/G) were noted in the 1.50% and 2.25% MLP groups relative to the control group, the differences did not reach statistical significance, suggesting that the beneficial impact of MLP on feed efficiency might necessitate extended research durations to manifest. However, when MLP supplementation was increased to 3.00%, a decline in the final body weight of the geese was observed. This result is in accordance with that of Liu et al. (Citation2019), who reported that incorporating up to 12% MLP in the diet of fattening pigs diminished final body weight and average daily gain while elevating the F/G ratio. The study by Wang et al. (Citation2017) supports our findings, indicating that the inclusion of more than 4% MLP in the diet adversely affected the growth performance of Sichuan white geese and potentially led to diarrhea and reduced subcutaneous fat deposition. These findings suggest the existence of an optimal supplementation range for MLP, wherein moderate levels can maximize growth performance in geese. This phenomenon may be attributed to certain components within mulberry leaves that are beneficial in moderate quantities but may exert adverse effects when excessive, due to antinutritional factors or other mechanisms. Hassan et al. (Citation2020) highlighted the potential positive effects of mulberry leaves in enhancing feed digestibility in ruminants, while also emphasizing the importance of maintaining appropriate supplementation levels to unlock their potential benefits. The high crude fiber content and potential antinutritional factors such as tannic acid in mulberry leaves might impede the digestive and absorptive capabilities and overall health of livestock and poultry at elevated doses. The outcomes related to relative organ weights demonstrated that the liver relative weights in the 1.50% and 2.25% MLP supplementation groups were significantly lower than those in the control group, suggesting a hepatoprotective effect of MLP. This finding is in agreement with Hou et al. (Citation2020), who found that supplementation with mulberry leaf extract, specifically 1-deoxynojirimycin (DNJ), significantly reduced the relative liver weight in goslings. Similarly, Park and Kim (Citation2012) reported that adding 1–3% mulberry leaf powder to broiler diets reduced the relative liver weight.
Our study identified that a supplementation level of 3.00% MLP significantly reduced serum triglyceride (TG) levels in meat geese, whereas levels of 1.50% and 2.25% did not exhibit similar effects. Additionally, serum HDL-c levels in geese displayed a trend of initial decrease followed by an increase with the dietary inclusion of MLP. These outcomes suggest that specific components within MLP might exert modulatory actions that vary with the dosage. The notable reduction in TG levels could be attributed to the antioxidative and lipid-regulating properties of flavonoid compounds present in MLP. Flavonoids have been demonstrated to ameliorate lipid metabolism, lower serum TG levels, and enhance the activity of antioxidative enzymes, thereby mitigating oxidative stress (Huang et al., Citation2021). Furthermore, MLP might modulate hepatic lipid metabolic pathways, such as activating PPARα and reducing the expression of SREBP-1c, thereby promoting fatty acid β-oxidation and inhibiting lipid synthesis (Liu et al., Citation2020).
Regarding the changes in HDL-c, the addition of MLP has been shown to impact serum lipoprotein levels, particularly elevating HDL-c at higher doses. This may reflect the complex effects of MLP components on lipoprotein metabolism, where different constituents or their interactions might exert diverse influences on HDL-c metabolism at varying dosages. For instance, certain flavonoids in mulberry leaves might regulate HDL-c levels by affecting the expression or activity of lipoprotein receptors in the liver (Lee et al., Citation2020). Another possibility involves the promotive effects of components in MLP on reverse cholesterol transport, thereby enhancing HDL-c levels and improving cardiovascular health (Zhang et al., Citation2022). However, the downward trend in HDL-c observed in the 1.50% MLP group could be associated with individual variations among the experimental animals, housing conditions, and feed intake during the experimental period.
Moreover, supplementation with MLP significantly elevated total serum superoxide dismutase (SOD) levels in geese. This finding aligns with the research by So-In and Sunthamala. (2022), who observed that MLP could enhance the antioxidative status and blood biochemical parameters in broilers reared under high-density fattening conditions. This is attributed to the rich content of antioxidative constituents such as polyphenols and flavonoids in MLP. These antioxidative components can activate the body’s antioxidative enzyme systems, further strengthening the organism’s resistance to oxidative stress. They are capable of scavenging free radicals, thereby augmenting the body’s overall antioxidative capacity and safeguarding cells from oxidative damage (Zheng et al., Citation2022).
The experimental outcomes revealed that supplementation with 1.50% MLP significantly enhanced the percentage of breast muscle, which might be attributed to the mechanism of action of specific components within the MLP. The increase in breast muscle percentage is likely due to the stimulatory effects of bioactive substances such as flavonoids present in MLP. Flavonoids have been demonstrated to activate the proliferation and differentiation of muscle cells, leading to an increase in muscle fiber formation (Margareta et al., Citation2015). This aligns with the findings of Chen et al. (Citation2019), who observed that MLP could positively influence muscle growth by modulating the gut microbiome balance, thereby improving the absorption and utilization of nutrients. However, the significant reduction in the leg muscle ratio under 1.50% and 2.25% MLP supplementation suggests subtle differences in the effects of MLP on various muscle types, warranting further investigation.
The observed lack of significant impact of MLP on water holding capacity and shear force is consistent with the findings of Ding et al. (Citation2021), indicating that while MLP can enhance nutrient absorption and muscle amino acid content, it may not necessarily affect the moisture retention characteristics of the meat. Although MLP can increase muscle mass, its influence on muscle fiber water retention and structural compactness is limited. This may be due to the fact that muscle water holding capacity is closely related to muscle fiber type, intramuscular fat content, and the degree of collagen cross-linking within the muscle, which are likely regulated by multiple physiological factors, with the role of MLP possibly obscured by other factors (Park & Kim, Citation2012).
Shear force is a crucial indicator of meat tenderness, with lower shear force values generally indicating better tenderness. The study’s results show a reduction in breast muscle shear force under 1.50% and 3.00% MLP supplementation, suggesting an improvement in muscle tenderness, consistent with previous research findings (Ouyang et al., Citation2020). Active components in MLP, such as flavonoids, may influence the denaturation and reorganization process of muscle proteins. The denaturation of muscle proteins during meat processing and cooking can lead to moisture loss and muscle fiber contraction, thereby affecting muscle tenderness. Although MLP supplementation did not significantly affect MDA levels in this study, a notable enhancement in T-SOD activity was observed. This suggests that MLP’s antioxidative effects may operate through various antioxidative pathways, thereby preserving the structural integrity of muscle fibers and reducing shear force. The observed discrepancy might be attributed to the fact that MDA primarily reflects the level of lipid peroxidation, whereas the increase in T-SOD activity is indicative of an early cellular antioxidative response, suggesting that MLP may inhibit the formation of free radicals at an earlier stage rather than directly affecting the end products of lipid peroxidation (Aziz-Aliabadi et al., Citation2023). Future research should further explore the effects of MLP on other antioxidative pathways to comprehensively evaluate its potential protective mechanisms.
Changes in texture profile analysis (TPA) indicate the potential impact of MLP supplementation on muscle texture. The biphasic response of breast muscle hardness may reflect the complex regulation of active components in MLP on muscle fiber reorganization and collagen maturation. At lower concentrations, antioxidative components in MLP might preferentially protect muscles from oxidative damage, maintaining fiber softness; while at higher concentrations, the impact of antinutritional factors in MLP might affect the structural integrity of the muscle, leading to an increase in hardness. This may be due to the action of specific components in MLP in leg muscles differing from that in breast muscles, related to differences in muscle fiber types and metabolic characteristics. Leg muscles, predominantly composed of slow-twitch fibers, rely more on aerobic metabolism and may respond differently to certain components in MLP compared to fast-twitch fiber-dominated breast muscles (Ning et al., Citation2021).
The reduction in chewiness and stickiness of breast muscle at lower MLP concentrations might be associated with MLP’s effect on protease activity within the muscle. Proteases, such as calpains and cathepsins, are involved in the degradation process of muscle proteins, affecting muscle texture characteristics. Certain components in MLP may stimulate or inhibit the activity of these enzymes, thereby influencing the degree of muscle fiber degradation and consequently reducing the chewiness and stickiness of the muscle (Khan et al., Citation2020). This effect might be diminished at higher MLP concentrations due to the influence of antinutritional factors. The change in adhesiveness reflects MLP’s impact on muscle protein interactions, with MLP’s influence on the adhesiveness and cohesiveness of breast muscle potentially related to its action on collagen and myosin. Collagen is the main structural protein in muscles, while myosin is a key protein in muscle contraction. Components in MLP may affect the cross-linking and structure of these proteins, thereby altering muscle adhesiveness and cohesiveness. Additionally, the denaturation and gelation of collagen during heating might also affect muscle texture characteristics (Westphalen et al., Citation2005). Although MLP’s impact on overall muscle water holding capacity is not significant, it may subtly modulate the distribution of water within and outside muscle cells, affecting muscle viscosity and adhesiveness. Components in MLP might alter the permeability of muscle cell membranes or the state of water binding within muscles, thereby influencing muscle texture characteristics (Makchuay et al., Citation2023).
Differences in the response of breast and leg muscles to MLP supplementation may be attributed to physiological and biochemical differences between the two muscle types. Breast muscles are primarily composed of white fibers, while leg muscles are predominantly red fibers. These muscle fibers exhibit significant differences in energy metabolism, with white fibers primarily relying on glycolysis for energy, whereas red fibers depend on oxidative metabolism. These metabolic differences may lead to varying responses of breast and leg muscles to nutritional supplementation such as MLP, subsequently affecting muscle texture characteristics (Zhao et al., Citation2019). Furthermore, the microenvironment within breast and leg muscles, including blood supply, oxygen, and nutrient availability, also differs. These differences might also affect the distribution and efficacy of active components in MLP within the muscles, resulting in distinct performances in TPA tests between breast and leg muscles (Liu et al., Citation2017).
Conclusion
This study demonstrates that at appropriate supplementation levels (1.50% to 2.25%), MLP exerts a positive effect on the growth performance of Zhedong White Geese, though excessive addition may entail potential risks. The findings further reveal that moderate MLP supplementation significantly improves the lipid metabolism in Zhedong white geese, notably through the reduction of TG levels, while simultaneously boosting the antioxidative capacity in serum. Moreover, MLP showcases positive effects on meat quality, especially in terms of muscle hardness and tenderness. These discoveries underscore the potential application of MLP in enhancing poultry growth performance and meat quality characteristics. In practical applications, the dosage of MLP should be carefully controlled to ensure that while production efficiency is enhanced, the health and welfare of the animals are also maintained. For industrial applications, MLP can be used as a natural feed additive to improve the nutritional value of poultry diets and reduce the need for synthetic additives. The antioxidative properties of MLP can enhance bird health and resistance to oxidative stress. Producers should start with lower MLP levels and adjust based on the flock’s responses, optimizing dosage to avoid adverse effects and achieve sustainable and profitable farming operations.
Author contributions
Xianze Wang designed and conceived the experiments. Huiying Wang and Daqian He optimized the details of the experiments and made critical revisions to the manuscript. Guangquan Li, Yi Liu, Cui Wang, Yunzhou Yang and Shaoming Gong participated in the collection of samples and data. Xianze Wang performed the data analysis and drafted the manuscript. All authors listed have reviewed and agreed to be accountable for all aspects of the work.
Ethical approval
The animal protocol for this study was approved by the Shanghai Science and Technology Commission (STCSM), with the license number SYXK (HU): 2015-000 7, and was conducted in accordance with the authorized guidelines and regulations. All possible measures were taken to minimize the suffering of the geese involved in the study.
Disclosure statement
No potential conflict of interest was reported by the author(s).
Data availability statement
The data that support the findings of this study are available in ‘figshare’ at https://figshare.com/articles/dataset/_mlp_/25404400.
Additional information
Funding
Notes on contributors
Xianze Wang
Xianze Wang is an assistant researcher at the Livestock and Veterinary Research Institute of the Shanghai Academy of Agricultural Sciences. His main research focus is on the nutrition of meat geese.
Guangquan Li
Guangquan Li is a research intern at the Livestock and Veterinary Research Institute of the Shanghai Academy of Agricultural Sciences. His main research direction is the production management of meat geese.
Yi Liu
Yi Liu is an assistant researcher at the Livestock and Veterinary Research Institute of the Shanghai Academy of Agricultural Sciences, with a primary focus on the breeding of meat geese.
Yunzhou Yang
Yunzhou Yang is an associate researcher at the Livestock and Veterinary Research Institute of the Shanghai Academy of Agricultural Sciences. His primary research direction is the breeding of meat geese.
Cui Wang
Cui Wang is an associate researcher at the Livestock and Veterinary Research Institute of the Shanghai Academy of Agricultural Sciences, focuses mainly on the breeding of meat geese.
Shaoming Gong
Shaoming Gong is a senior livestock officer at the Livestock and Veterinary Research Institute of the Shanghai Academy of Agricultural Sciences, with a main research direction in the rearing and management of laying ducks.
Huiying Wang
Huiying Wang is a researcher at the Livestock and Veterinary Research Institute of the Shanghai Academy of Agricultural Sciences. Her main research direction is the healthy cultivation of meat geese.
Daqian He
Daqian He is a researcher at the Livestock and Veterinary Research Institute of the Shanghai Academy of Agricultural Sciences. His main research direction is the breeding of meat geese.
References
- AOAC. (1930). Official methods of analysis of AOAC International, Method 920.39. Crude Fat (Ether Extract) in Animal Feed. AOAC International.
- AOAC. (1985a). Official methods of analysis of AOAC International, Method 983.19. Calcium (Ca) in mechanically separated poultry and beef. AOAC International.
- AOAC. (1985b). Official methods of analysis of AOAC International, Method 995.11. ‘Phosphorus (P) Colorimetric Method in Foods. AOAC International.
- AOAC. (2000). Official methods of analysis of AOAC International, Method 978.10. ‘Fiber (Crude) in animal feed and pet food. AOAC International.
- AOAC. (2005). Official methods of analysis of AOAC International, Method 984.13. Protein (Crude) in Animal Feed and Pet Food. AOAC International.
- Aziz-Aliabadi, F., Noruzi, H., & Hassanabadi, A. (2023). Effect of different levels of green tea (Camellia Sinensis) and Mulberry (Morus Alba) leaves powder on performance, carcass characteristics, immune response and intestinal morphology of broiler chickens. Veterinary Medicine and Science, 9(3), 1–14. https://doi.org/10.1002/vms3.1133
- Çelebi, Y. (2024). Improvement of quality characteristics and shelf life extension of raw chicken meat by using Black Mulberry Leaf (Morus nigra L.) Extracts. Journal of Food Quality, 2024, 1–11. https://doi.org/10.1155/2024/5822690
- Chen, Y., Ni, J., & Li, H. (2019). Effect of green tea and mulberry leaf powders on the gut microbiota of chicken. BMC Veterinary Research, 15(1), 77. https://doi.org/10.1186/s12917-019-1822-z
- Ding, Y., Jiang, X., Yao, X., Zhang, H., Song, Z., He, X., & Cao, R. (2021). Effects of feeding fermented mulberry leaf powder on growth performance, slaughter performance, and meat quality in Chicken Broilers. Animals: An Open Access Journal from MDPI, 11(11), 3294. https://doi.org/10.3390/ani11113294
- Hassan, F., Arshad, M., Li, M., Rehman, M., Loor, J., & Huang, J. (2020). Potential of mulberry leaf biomass and its flavonoids to improve production and health in ruminants: Mechanistic insights and prospects. Animals: An Open Access Journal from MDPI, 10(11), 2076. https://doi.org/10.3390/ani10112076
- Hou, Q., Qian, Z., Wu, P., Shen, M., Li, L., & Zhao, W. (2020). 1-Deoxynojirimycin from mulberry leaves changes gut digestion and microbiota composition in geese. Poultry Science, 99(11), 5858–5866. https://doi.org/10.1016/j.psj.2020.07.048
- Huang, Z., Lv, Z., Dai, H., Li, S., Jiang, J., Ye, N., Zhu, S., Wei, Q., & Shi, F. (2021). Dietary mulberry-leaf flavonoids supplementation improves liver lipid metabolism and ovarian function of aged breeder hens. Journal of Animal Physiology and Animal Nutrition, 106(6), 1321–1332. https://doi.org/10.1111/jpn.13658
- Huyen, N., Wanapat, M., & Navanukraw, C. (2012). Effect of Mulberry Leaf Pellet (MUP) Supplementation on rumen fermentation and nutrient digestibility in beef cattle fed on rice straw-based diets. Animal Feed Science and Technology, 175(1-2), 8–15. https://doi.org/10.1016/j.anifeedsci.2012.03.020
- Khan, I., Parker, N., Löhr, C., & Cherian, G. (2020). Docosahexaenoic acid (22:6 N-3)-rich microalgae along with methionine supplementation in broiler chickens: Effects on production performance, breast muscle quality attributes, lipid profile, and incidence of white striping and myopathy. Poultry Science, 100(2), 865–874. https://doi.org/10.1016/j.psj.2020.10.069
- Lee, S., Lee, M., Chang, E., Lee, Y., Lee, J., Kim, J., Kim, C., Kim, I., & Kim, Y. (2020). Mulberry fruit extract promotes serum HDL-cholesterol levels and suppresses hepatic microRNA-33 expression in rats fed high cholesterol/cholic acid diet. Nutrients, 12(5), 1499. https://doi.org/10.3390/nu12051499
- Liu, Y., Tang, G., Yang, J., Li, W., & China, P. (2017). Effects of dietary conjugated linoleic acid on lipid peroxidation in breast and thigh muscles of broiler chickens. Czech Journal of Animal Science, 62(8), 331–338. https://doi.org/10.17221/95/2016-CJAS
- Liu, Y., Li, Y., Peng, Y., He, J., Xiao, D., Chen, C., Li, F., Huang, R., & Yin, Y. (2019). Dietary mulberry leaf powder affects growth performance, carcass traits and meat quality in finishing pigs. Journal of Animal Physiology and Animal Nutrition, 103(6), 1934–1945. https://doi.org/10.1111/jpn.13203
- Liu, Y., Li, Y., Xiao, Y., Peng, Y., He, J., Chen, C., Xiao, D., Yin, Y., & Li, F. (2020). Mulberry leaf powder regulates antioxidative capacity and lipid metabolism in finishing pigs. Animal Nutrition (Zhongguo xu mu Shou yi Xue Hui), 7(2), 421–429. https://doi.org/10.1016/j.aninu.2020.08.005
- Ma, J., Wang, J., Jin, X., Liu, S., Tang, S., Zhang, Z., Long, S., & Piao, X. (2023). Effect of dietary supplemented with mulberry leaf powder on growth performance, serum metabolites, antioxidant property and intestinal health of weaned piglets. Antioxidants, 12(2), 307. https://doi.org/10.3390/antiox12020307
- Makchuay, T., Tongchitpakdee, S., & Ratanasumawong, S. (2023). Effect of mulberry leaf tea on texture, microstructure, starch retrogradation, and antioxidant capacity of rice noodles. Journal of Food Processing and Preservation, 2023, 1–9. https://doi.org/10.1155/2023/2964013
- Margareta, O., Diana, C. R., Maria, C. G., Mariana, R., Tatiana, P., & Iulia, V. (2015). Effect of Dietary Mulberry (Morus Alba) leaves on performance parameters and quality of breast meat of broilers. The Indian Journal of Animal Sciences, 85(3), 291–295. https://doi.org/10.56093/ijans.v85i3.47328
- Miller, D. S., & Judd, P. A. (1984). The metabolisable energy value of foods. Journal of the Science of Food and Agriculture, 35(1), 111–116. https://doi.org/10.1002/jsfa.2740350118
- National Research Council (NRC). (1994). Nutrient requirements of poultry (9th ed., pp. 40–41). National Academy Press.
- Ning, L., Gao, L., Zhou, W., Liu, S., Chen, X., & Pan, Q. (2021). Beneficial effects of dietary mulberry leaf along with multi-enzyme premix on the growth, immune response and disease Resistance of Golden Pompano Trachinotus Ovatus. Aquaculture, 535, 736396. https://doi.org/10.1016/j.aquaculture.2021.736396
- Ouyang, J., Hou, Q., Wang, M., Zhao, W., Feng, D., Pi, Y., & Sun, X. (2020). Effects of dietary mulberry leaf powder on growth performance, blood metabolites, meat quality, and antioxidant enzyme-related gene expression of fattening hu lambs. Canadian Journal of Animal Science, 100(3), 510–521. https://doi.org/10.1139/cjas-2019-0119
- Park, C., & Kim, Y. (2012). Effects of dietary supplementation of mulberry leaves powder on chicken meat quality stored during cold storage. Food Science of Animal Resources, 32(2), 184–189. https://doi.org/10.5851/kosfa.2012.32.2.184
- Hou, Q. R., Zhang, J., Chen, T., Zhao, W. G., & Li, L. (2018). Effects of dietary supplement of mulberry leaf (Morus alba) on growth and meat quality in rabbits. Indian Journal of Animal Research, 54(of), 317–321. https://doi.org/10.18805/ijar.B-1006
- So-In, C., & Sunthamala, N. (2022). The Effects of Mulberry (Morus Alba Linn.) Leaf supplementation on growth performance, blood parameter, and antioxidant status of broiler chickens under high stocking density. Veterinary World, 15(11), 2715–2724. https://doi.org/10.14202/vetworld.2022.2715-2724
- Srivastava, S., Kapoor, R., Thathola, A., & Srivastava, R. (2006). Nutritional quality of leaves of some genotypes of mulberry (Morus Alba). International Journal of Food Sciences and Nutrition, 57(5-6), 305–313. https://doi.org/10.1080/09637480600801837
- Wang, C., Yang, F., Wang, Q., Zhou, X., Xie, M., Kang, P., Wang, Y., & Peng, X. (2017). Nutritive value of mulberry leaf meal and its effect on the performance of 35–70-Day-Old Geese. The Journal of Poultry Science, 54(1), 41–46. https://doi.org/10.2141/jpsa.0160070
- Wang, S., Tang, C., Li, J., Wang, Z., Meng, F., Luo, G., Xin, H., Zhong, J., Wang, Y., Li, B., Li, Z., Chen, L., Hu, B., & Lin, S. (2022). The effects of dietary inclusion of mulberry leaf powder on growth performance, carcass traits and meat quality of Tibetan Pigs. Animals: An Open Access Journal from MDPI, 12(20), 2743. https://doi.org/10.3390/ani12202743
- Westphalen, A., Briggs, J., & Lonergan, S. (2005). Influence of pH on rheological properties of porcine Myofibrillar protein during heat induced gelation. Meat Science, 70(2), 293–299. https://doi.org/10.1016/j.meatsci.2005.01.015
- Yang, Y., Wang, C., Li, G., Wang, X., Qiu, S., Liu, Y., Wang, H., & He, D. (2023). Significant association of mutations close to LCORL gene with growth performance in Zhedong White Geese (Anser Cygnoides). Animal Genetics, 54(5), 628–631. https://doi.org/10.1111/age.13342
- Yu, Y., Li, H., Zhang, B., Wang, J., Shi, X., Huang, J., Yang, J., Zhang, Y., & Deng, Z. (2018). Nutritional and functional components of mulberry leaves from different varieties: Evaluation of their potential as food materials. International Journal of Food Properties, 21(1), 1495–1507. https://doi.org/10.1080/10942912.2018.1489833
- Zhang, B., Wang, Z., Huang, C., Wang, D., Chang, D., Shi, X., Chen, Y., & Chen, H. (2022). Positive effects of mulberry leaf extract on egg quality, lipid metabolism, serum biochemistry, and antioxidant indices of laying hens. Frontiers in Veterinary Science, 9, 1005643. https://doi.org/10.3389/fvets.2022.1005643
- Zhao, X., Yang, R., Bi, Y., Bilal, M., Kuang, Z., Iqbal, H. M. N., & Luo, Q. (2019). Effects of Dietary Supplementation with Mulberry (Morus alba L.) Leaf Polysaccharides on Immune Parameters of Weanling Pigs. Animals, 10(1), 35.https://doi.org/10.3390/ani10010035
- Zheng, Q., Tan, W., Feng, X., Feng, K., Zhong, W., Liao, C., Liu, Y., Li, S., & Hu, W. (2022). Protective effect of flavonoids from mulberry Leaf on AAPH-induced oxidative damage in sheep erythrocytes. Molecules, 27(21), 7625. https://doi.org/10.3390/molecules27217625