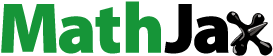
Abstract
Late leaf spot (LLS) disease causes over 70% yield loss to groundnut by reducing plant area available for photosynthesis. The objective of this research was to validate four SNP markers for the identification of LLS-resistant plants. A total of 188 F2 plants derived from a cross between LLS-resistant CS16 and LLS-susceptible Shitouqi were genotyped with four SNP markers proposed to be linked to LLS resistance in groundnut. Phenotypic data (LLS severity score) was taken on F2:3 families derived through selfing F2 lines. Polymorphism test revealed that marker GKAMA02GL779 could not distinguish between the two divergent parents, hence it was excluded from further analysis. Single marker analysis using Kruskal-Wallis analysis of variance and linear regression, both established strong association between three SNP markers (GKAMA02GL582, GKAMA02GL829 and GKAMA02GL975) and LLS resistance with adjusted R2 values of 0.758, 0.73 and 0.613, respectively. Three out of the four proposed markers for LLS resistance breeding have been validated and can be used in future breeding programmes to identify LLS-resistant groundnut lines.
REVIEWING EDITOR:
Introduction
Late leaf spot disease (LLS) is a major cause of yield loss in groundnut production (Ibrahim, Citation2010), accounting for over 70% of groundnut yield loss in Ghana. LLS is caused by the fungus known as Nothopassalora personata (Berk. & M.A. Curtis). Although chemical treatments have been recommended for the treatment of LLS, they are expensive and unsafe in certain situations and locations (Chandra et al., Citation2005; Muhammad & Bdliya, Citation2011; Khan et al., Citation2014). Host plant resistance, on the other hand, offers a safe, efficient and environmentally friendly way of controlling LLS (Ibrahim, Citation2010). The application of host plant resistance further improves the yield and quality of groundnuts produced by farmers (Shoba et al., Citation2012). However, the nature of the inheritance of LLS resistance is complex and polygenic (Dwivedi et al., Citation2002), thus making the identification of resistant and susceptible lines unreliable through conventional screening techniques (Leal-Bertioli et al., Citation2009). With good polymorphic molecular markers, a breeder can easily identify LLS-resistant and susceptible groundnut plants (Mace et al., Citation2006). Previous studies have used molecular markers to improve the efficiency of selection in breeding, especially in the case of low heritable traits, where phenotypic selection is difficult, expensive and lacks accuracy (Kelly et al., Citation2003; Guo et al., Citation2013; Mishra et al., Citation2015; Zongo et al., Citation2017). In recent times, the use of Single Nucleotide Polymorphic markers (SNPs) in molecular breeding has become more popular and user-friendly. Compared to other DNA markers such as Simple Sequence Repeats (SSRs), Random Amplified Polymorphic DNA (RAPD), Amplified Fragment Length Polymorphism (AFLP), and Restriction Fragment Length Polymorphism (RFLP) markers, SNP markers are more flexible, fast and also provide data management convenience (Morgil et al., Citation2020). However, the availability of SNPs and sophisticated software does not always guarantee that the discovered SNP can be converted into a valid marker (Mammadov et al., Citation2012). To ensure that the discovered SNP is a Mendelian locus, it has to be validated. The validation of a marker is the process of designing an assay based on the discovered polymorphism and then genotyping a panel of diverse germplasm or segregating population (Mammadov et al., Citation2012). Compared to the collection of unrelated lines, a segregating population is more informative as a validation panel because it allows the inspection of the discriminatory ability and segregation patterns of a marker, which helps the researcher to understand whether it is a Mendelian locus or a repetitive sequence that escaped the software filter (Mammadov et al., Citation2012). According to Nevill (Citation1981), resistance components of leaf spot disease are controlled by dominant gene action while resistance itself is governed by recessive genes. Previous works have identified and validated SSR markers, Transposable Element (AhTE) markers and SNP markers linked with LLS resistance in peanut (Mace et al., Citation2006; Khedikar et al., Citation2010; Shoba et al., Citation2012; Sujay et al., Citation2012; Gajjar et al., Citation2014; Kukanur et al., Citation2014; Kolekar et al., Citation2016; Divyadharsini et al., Citation2017; Pandey et al., Citation2017; Shirasawa et al., Citation2018, Oteng-Frimpong et al., Citation2022). Several QTLs linked to LLS resistance in groundnut have been suggested by previous authors with some major ones spanning across chromosomes A02, A03, A05, B03, and B10 (Khedikar et al., Citation2010; Kolekar et al., Citation2016; Zhou et al., Citation2016; Liang et al., Citation2017; Banla, Citation2018; Clevenger et al., Citation2018; Zhang et al., Citation2020; Oteng-Frimpong et al., Citation2022).
In this study, we focused on four Kompetitive Allele Specific PCR (KASP) SNP markers designed from a major QTL qLLSA02 on chromosome A02 known to confer LLS resistance in CS16 (ICGV 86855) peanut variety (Pandey et al., Citation2017; Shirasawa et al., Citation2018). CS16 was derived from the GP-NC WS lines from North Carolina State University (NCSU) that were formed from a unique hybridization of cultivated peanut A. hypogaea with the wild diploid peanut A. cardenasii. It is widely believed that the LLS resistance in CS16 traces to A. cardenasii (PGI, 2017; Stalker, Citation2017; Bertioli et al., Citation2021). CS16 has served as source of resistance in several crosses and backcrosses with elite groundnut lines across the world (Stalker et al., Citation2013; Stalker, Citation2017; Jadhav et al., Citation2021). Validation of these markers will speed up the process of introgression of LLS resistance in groundnut. Validated markers in this project can also be used to identify LLS-resistant lines in future breeding programmes. Hence, the aim of the study was to validate four KASP SNP markers linked to LLS using F2 and F2:3 populations of groundnut in Ghana’s environment.
Materials and methods
Plant materials
Parental lines CS16 (LLS resistant) and Shitouqi (LLS susceptible) of Arachis hypogaea were obtained from the Centre d’Étude Régional pour l’Amélioration de l’Adaptation à la Sécheresse (CERAAS), Senegal and CSIR-Crops Research Institute of Ghana, respectively. The lines were first crossed in October 2019 to produce 188 F1 seeds. The F2 generation was derived by selfing F1 plants in the greenhouse in February 2020. F2:3 families for phenotypic evaluation were derived by allowing F2 plants to self-pollinate.
Molecular screening of F2 population
For each of the 188 F2 seeds, a small portion of cotyledon was sampled and shipped to Intertek Laboratory in Sweden for SNP genotyping along with parental checks. At Intertek lab, DNA was extracted from each sample by first grinding the samples with steel balls to break plant tissues. DNA lysis buffer was then added to the samples and incubated for 45 minutes. DNA was then purified by super-magnetic microparticles using a commercial purification kit (sbeadexTM) and instruments from LGC Group (LGC Biosearch Technologies, Hoddesdon, United Kingdom). Polymerase Chain Reaction (PCR) was conducted using the Kompetitive Allele Specific PCR (KASPTM) genotyping technology from LGC Group. Four KASP SNP markers located within major LLS QTL qLLSA02 on chromosome A02 of groundnut () were used for the PCR (CGIAR-EBP, Citation2021). The primer sequence of the markers remains a trade secret of the LGC group.
Table 1. KASP SNP markers linked to LLS resistance QTL (qLLSA02) in groundnut.
Molecular data analysis
For each sample, alleles controlling all four loci were identified and viewed with SNPviewer2 version 4.0.0 software from which quality of the data and summary statistics regarding the samples and markers were determined. The data was then exported to Flapjack format through the Excellence in Breeding - Galaxy Platform (http://13.250.212.83). The flapjack file revealed the allele combinations of the samples at the four LLS loci of interest. Chi-square test was used to test the null hypothesis that the alleles are out of Hardy Weinberg equilibrium at all four marker loci using the formula, where χ2 = Chi-square; Oi = observed value (actual value) and Ei = expected value.
Polymorphism test
A polymorphism test was conducted on the four SNP markers using the genotypic data of the two parents - CS16 and Shitouqi. CS16 variety is a known LLS-resistant line (Stalker, Citation2017), while Shitouqi is also known to be susceptible to LLS in Ghana (MoFA, Citation2019). Hence, the four SNP markers were expected to clearly distinguish between the two varieties at the molecular level.
Phenotypic screening of F2:3 population
After genotyping, 130 F2:3 families generated from the F2 population were grown in an augmented randomized complete block design with 10 blocks and two parental checks (CS16 and Shitouqi) in a field experiment laid out on the experimental fields of CSIR-Crops Research Institute of Ghana from March to July 2020. Each block had 15 plots (13 genotypes + 2 checks). The checks were replicated in each of the 10 blocks to help estimate block effects and error, thus bringing the total number of plots to 150. The experimental units were inoculated with 2 x 104 conidia/ml suspension of Nothopassalora personata 8 weeks after planting to create artificial epiphytotic conditions. A 9-point rating scale was used to score disease severity 12 weeks after planting, where disease severity score of 1 meant 0% foliar infection; 2 for 1–5%; 3 for 6 – 10%; 4 for 11 – 20%; 5 for 21 – 30%; 6 for 31 – 40%; 7 for 41 – 60%; 8 for 61 – 80% and 9 for 81 – 100% of foliar area infection with plants having almost all leaves defoliated leaving bare stems (Subrahmanyam et al.,Citation1995). Genotypes with a disease severity score of 1 – 3 were classified as resistant; 4 – 6 as moderate resistance and 7 – 9 as susceptible (Mohammed et al., Citation2018).
Phenotypic data analysis
Disease severity score data was subjected to the Shapiro-Wilk normality test (Shapiro & Wilk, Citation1965) and later normalized with square root transformation using Genstat 18th edition software. Analysis of variance was conducted with PBTools version 1.3 software (IRRI, Citation2020) using a special case of the Incomplete Block Design model yij = μ + αi + βj + εij, Where: yij is the observation of the i-th line in the block j; μ is the overall mean; αi is the effect of the i-th line; βj is the effect of the j-th incomplete block.
Single marker analysis
Genotypic data collected on the F2 plants together with phenotypic LLS severity scores collected on F2:3 families were subjected to single marker analysis using Kruskal-Wallis ANOVA and linear regression analysis with the aid of Genstat 18th edition software. LLS severity score from the F2:3 plants was used as the dependent variable (Y) while genotypic data collected from the F2 seeds was used as the independent variable.
Results
Molecular screening results
Each of the four SNP markers was used to screen 192 wells covering two PCR plates, out of which wells H11 and H12 were reserved as negative controls leaving 188 wells with DNA samples. A total of 724 allele calls were correctly identified, while 28 allele calls could not be identified () resulting in an allele call rate of 96.3%. SNP Viewer images were used to identify genotypic clusters, and to validate the quality of KASP assays for all four SNP markers, one of which is presented in . From chi-squared test, it was found that alleles in the F2 population were in Hardy Weinberg equilibrium at all four marker loci with p-values < 0.05.
Table 2. Summary statistics for molecular data.
Polymorphism test
Among the four SNP markers, GKAMA02GL779 was not polymorphic, which means it could not distinguish between the resistant and susceptible parents. On the other hand, the three remaining markers (GKAMA02GL582, GKAMA02GL975 and GKAMA02GL829) were polymorphic (). Hence, GKAMA02GL779 was excluded from further analysis.
Table 3. Genotype of parents at four loci linked to LLS resistance.
Phenotypic screening results
LLS severity observed in the F2:3 population ranged from 1.0 to 9.0. The mean LLS score for the population was 5.007 ± 0.40 with a standard deviation of 1.92. Analysis of Variance revealed a significant difference among the individual genotypes (p < 0.05). Three phenotypic groups were observed – resistant lines, moderately resistant lines and susceptible lines in the proportion of 47:48:35 respectively.
Single-marker analysis
Single-marker analysis was conducted with two approaches – Kruskal-Wallis analysis of variance and linear regression.
Kruskal-Wallis analysis of variance
Significant differences were detected among genotypic groups at all three marker loci in terms of LLS resistance status. For markers GKAMA02GL829 and GKAMA02GL975, individuals with G:G genotype mostly showed resistance phenotype while G:C and C:C genotypes showed moderate resistance and susceptibility respectively to LLS (). For marker GKAMA02GL582, A:A genotypes were generally susceptible, C:A genotypes showed moderate resistance, while C:C showed resistance to LLS.
Table 4. Phenotype of genotypic groups.
Linear regression
Linear regression was further used to establish a relationship between the three polymorphic markers and LLS severity scores. LLS severity score was used as the dependent variable (Y) while genotypic data from the three polymorphic markers was used as independent variable in three separate single marker analysis (SMA). Marker GKAMA02GL582 had an adjusted R2 value of 0.73 accounting for 73.4% of variation observed in the phenotype with a standard error of 0.409. The other two SNP markers GKAMA02GL829 and GKAMA02GL975 had adjusted R2 values of 0.613 and 0.758 accounting for 61.3% and 75.8% of observed LLS variation respectively () (). The regression models used for the analysis were significant (P < 0.001) for all three markers.
Figure 2. Regression of three SNP markers on LLS scores. (a) SNP GKAMA02GL829, (b) SNP GKAMA02GL975 (c) SNP GKAMA02GL582.

Table 5. Goodness of fit statistics.
Discussion
Until recently, identification and utility of SNPs in tetraploid genotypes of groundnut were rare unless tetraploid was synthetic (Knapp et al., Citation2011). With improved methodologies, several putative SNPs have been identified, some of which are linked to diseases and other important traits of groundnut (Knapp et al., Citation2011; Zhou et al., Citation2014; Kolekar et al., Citation2016; Clevenger et al., Citation2017; Shirasawa et al., Citation2016; Pandey et al., Citation2017; Agarwal et al., Citation2018; Shaibu et al., Citation2020; Oteng-Frimpong et al., Citation2022). Shirasawa et al. (Citation2018) used a double-digest restriction-site associated DNA sequencing (ddRAD-seq) technique based on next-generation sequencing (NGS) for genotyping analysis across recombinant inbred lines derived from a cross between a susceptible line, TAG 24, and a resistant line, GPBD 4 to identify QTLs on Chromosome A02 and A03 for LLS and rust respectively. Shirasawa et al. (Citation2018) further selected four resistance–related genes with deleterious mutations located in 1.4∼ Mb genome region as candidates for LLS resistance.
Other studies have traced LLS resistance on A02 chromosome to the wild peanut species A. cardenasii (Pandey et al., Citation2017; Banla, Citation2018). In this study, the resistant variety CS16 had A. cardenasii background (PGI, 2017; Stalker, Citation2017, Bertioli et al., Citation2021) with the desired qLLSA02 region from which the four SNP markers used in this present work were developed (Pandey et al., Citation2017). These markers have also been validated and used in a marker-assisted programme in India that sort to improve groundnut for rust, LLS and high oleate. (Jadhav et al., Citation2021).
Marker validation in this present study was done by single-marker analysis at two levels using Kruskal-Wallis ANOVA and linear regression. A similar analysis pattern was used by Sukruth et al. (Citation2015) and Gajjar et al. (Citation2014) to validate marker-trait association between LLS and five SSR markers (GM2009, GM2301, GM2079, GM1536 and GM1954).
Kruskal-Wallis ANOVA in this study revealed highly significant association between the three polymorphic markers and LLS phenotype with p values < 0.05. The significance of marker-trait association was further confirmed by linear regression, where SNP markers GKAMA02GL582, GKAMA02GL975 and GKAMA02GL829 had R2 values greater than 0.5, which indicate strong effects of the markers on LLS resistance (Kelly et al., Citation2003). Hence, the three markers (GKAMA02GL582, GKAMA02GL975 and GKAMA02GL829) can be used effectively in early-generation selections in groundnut breeding for LLS resistance in Ghana when using CS16 as the resistance source.
Conclusion
Three out of four proposed SNP markers have been validated as markers linked to LLS resistance locus (qLLSA02) in Ghana’s environment.
Author contributions
DBA conducted the experiment, collected and analyzed the data for his PhD thesis, and drafted the manuscript. DDK, EJSY, AS, OK and AJY conceived and designed the study, helped in data analysis and reviewed the manuscript. MW helped in genotyping the population and analysing the genotypic data.
Acknowledgement
We also acknowledge IFAD for the genotyping support through the EBCA project.
Disclosure statement
No potential conflict of interest was reported by the author(s).
Data availability statement
Data will be made available upon reasonable request.
Additional information
Funding
Notes on contributors
Benjamin Aboagye Danso
Benjamin Aboagye Danso is a Plant Breeder and Lecturer at the Faculty of Agriculture Education in Akenten Appiah-Menka University of Skills Training and Entrepreneurial Development. Prior to this, he was a PhD student in Plant Breeding at the West Africa Centre for Crop Improvement.
Daniel Kwadjo Dzidzienyo
Daniel Kwadjo Dzidzienyo is a Senior Lecturer at the Biotechnology Centre and also the Deputy Director, Administration, Teaching and Learning at the West Africa Centre for Crop Improvement (WACCI), College of Basic and Applied Sciences, University of Ghana.
John Saviour Yaw Eleblu
John Saviour Yaw Eleblu is a Senior Lecturer at the Biotechnology Centre and also the Coordinator for Research Programmes at the West Africa Centre for Crop Improvement (WACCI), College of Basic and Applied Sciences, University of Ghana.
Sylvester Addy
Sylvester Addy is a Senior Research Scientist at CSIR-Crops Research Institute of Ghana, Kumasi. Sylvester does research in Plant Breeding, Biotechnology and Agronomy.
William Manilal
William Manilal is a research scientist with significant expertise in international agriculture and multinational seed systems. He works with the Integrated Breeding Platform, IBP
Kwadwo Ofori
Kwadwo Ofori is a Professor at the Department of Crop Science and also the Associate Director for Academic and Students Affairs at the West Africa Centre for Crop Improvement (WACCI), College of Basic and Applied Sciences, University of Ghana.
James Yaw Asibuo
James Yaw Asibuo is a Principal Research Scientist working with the CSIR-Crops Research Institute of Ghana, Kumasi. His research interests cover molecular breeding, legume seed systems and biotechnology.
References
- Agarwal, G., Clevenger, J., Pandey, M. K., Wang, H., Shasidhar, Y., Chu, Y., Fountain, J. C., Choudhary, D., Culbreath, A. K., Liu, X., Huang, G., Wang, X., Deshmukh, R., Holbrook, C. C., Bertioli, D. J., Ozias-Akins, P., Jackson, S. A., Varshney, R. K., & Guo, B. (2018). High-density genetic map using whole-genome resequencing for fine mapping and candidate gene discovery for disease resistance in peanut. Plant Biotechnology Journal, 16(11), 1–9. https://doi.org/10.1111/pbi.12930
- Banla, E. M. (2018). Characterization of cultivated groundnut (Arachis hypogea L.) and genetic analysis of late leaf spot resistance in Togo [PhD thesis]. University of Ghana, Legon.
- Bertioli, D. J., Cannon, S. B., Froenicke, L., Huang, G., Farmer, A. D., Cannon, E. K. S., Liu, X., Gao, D., Clevenger, J., Dash, S., Ren, L., Moretzsohn, M. C., Shirasawa, K., Huang, W., Vidigal, B., Abernathy, B., Chu, Y., Niederhuth, C. E., Umale, P., … Ozias-Akins, P. (2016). The genome sequences of Arachis duranensis and Arachis ipaensis, the diploid ancestors of cultivated peanuts. Nature Genetics, 48(4), 438–446. https://doi.org/10.1038/ng.3517
- Bertioli, D. J., Clevenger, J., Godoy, I. J., Stalker, H. T., Wood, S., Santos, J. F., Ballén-Taborda, C., Abernathy, B., Azevedo, V., Campbell, J., Chavarro, C., Chu, Y., Farmer, A. D., Fonceka, D., Gao, D., Grimwood, J., Halpin, N., Korani, W., Michelotto, M. D., … Leal-Bertioli, S. C. M. (2021). Legacy genetics of Arachis cardenasii in the peanut crop shows the profound benefits of international seed exchange. Proceedings of the National Academy of Sciences of the United States of America, 118(38), e2104899118. https://doi.org/10.1073/pnas.2104899118
- CGIAR-Excellence in Breeding Platform (EBP). (2021). Low & mid-density genotyping services. https://excellenceinbreeding.org/module3/kasp
- Chandra, B., Viswavidyalaya, K., & Bengal, W. (2005). Management of leafspot (Cercospora arachidicola and Phaeoisariopsis personata). 75, 3–4.
- Clevenger, J., Chu, Y., Chavarro, C., Agarwal, G., Bertioli, D. J., Leal-Bertioli, S. C. M., Pandey, M. K., Vaughn, J., Abernathy, B., Barkley, N. A., Hovav, R., Burow, M., Nayak, S. N., Chitikineni, A., Isleib, T. G., Holbrook, C. C., Jackson, S. A., Varshney, R. K., & Ozias-Akins, P. (2017). Genome-wide SNP Genotyping resolves signatures of selection and tetrasomic recombination in peanut. Molecular Plant, 10(2), 309–322. https://doi.org/10.1016/j.molp.2016.11.015
- Clevenger, J., Chu, Y., Chavarro, C., Botton, S., Culbreath, A., Isleib, T. G., Holbrook, C. C., & Ozias-Akins, P. (2018). Mapping late leaf spot resistance in peanut (Arachis hypogaea) using QTL-seq reveals markers for marker-assisted selection. Frontiers in Plant Science, 9, 83. https://doi.org/10.3389/fpls.2018.00083
- Divyadharsini, R., Prabhu, R., Manivannan, N., & Vindhivavarman, P. (2017). Validation of SSR markers for foliar disease resistance in groundnut (Arachis hypogaea L.). International Journal of Current Microbiology and Applied Sciences, 6(4), 1310–1317. https://doi.org/10.20546/ijcmas.2017.604.160
- Dwivedi, S. L., Pande, S., Rao, J. N., & Nigam, S. N. (2002). Components of resistance to late leaf spot and rust among interspecific derivatives and their significance in a foliar disease resistance breeding in groundnut (Arachis hypogaea L.). Euphytica, 125(1), 81–88. https://doi.org/10.1023/A:1015707301659
- Gajjar, K. N., Mishra, G. P., Radhakrishnan, T., Dodia, S. M., Rathnakumar, A. L., Kumar, N., Kumar, S., & Kumar, A. (2014). Validation of SSR markers linked to the rust and late leaf spot diseases resistance in diverse peanut genotypes. Australian Journal of Crop Science, 8(6), 927–936.
- Guo, B., Pandey, M. K., He, G., Zhang, X., Liao, B., Culbreath, A., Varshney, R. K., Nwosu, V., Wilson, R. F., & Stalker, H. T. (2013). Recent advances in molecular genetic linkage maps of cultivated peanut. Peanut Science, 40(2), 95–106. https://doi.org/10.3146/PS13-03.1
- Ibrahim, Y. J. (2010). Screening of groundnuts (Arachis hypogaea L.) for resistance to early and late leaf spot [MSc. thesis]. Kwame Nkrumah University of Science and Technology.
- International Rice Research Institute (IRRI). (2020). Plant breeding tools (PBTools) Version: 1.3. http://bbi.irri.org
- Jadhav, Mangesh Pralhad, Patil, Malagouda D., Hampannavar, Mahesh, Dattatreya, Pavana, Shirasawa, Kenta, Pasupuleti, Janila, Pandey, Manish K., Varshney, Rajeev K., Bhat, Ramesh S., Venkatesh, (2021). Enhancing oleic acid content in two commercially released peanut varieties through marker-assisted backcross breeding. Crop Science, 61(4): 2435–2443. https://doi.org/10.1002/csc2.20512
- Kelly, J. D., Gepts, P., Miklas, P. N., & Coyne, D. P. (2003). Tagging and mapping of genes and QTL and molecular marker-assisted selection for traits of economic importance in bean and cowpea. Field Crops Research, 82(2-3), 135–154. https://doi.org/10.1016/S0378-4290(03)00034-0
- Khan, A. R., Ijaz, M., Haq, I. U., Farzand, A., & Tariqjaved, M. (2014). Management of Cercospora leaf spot of groundnut (Cercospora arachidicola & use of systemic fungicides. Cercetari Agronimice in Moldova, XLVII(2), 98–102.
- Khedikar, Y. P., Gowda, M. V. C., Sarvamangala, C., Patgar, K. V., Upadhyaya, H. D., & Varshney, R. K. (2010). A QTL study on late leaf spot and rust revealed one major QTL for molecular breeding for rust resistance in groundnut (Arachis hypogaea L.). Theoretical and Applied Genetics, 121(5), 971–984. https://doi.org/10.1007/s00122-010-1366-x
- Knapp, S. J., Ozias-Akins, P., Hoisington, D., Aruna, R., & Varshney, R. (2011). G4008.06: Single nucleotide polymorphism discovery, validation, and mapping in groundnut. Project updates. CGIAR Generation Challenge Program.
- Kolekar, R. M., Sujay, V., Shirasawa, K., Sukruth, M., Khedikar, Y. P., Gowda, M. V. C., Pandey, M. K., Varshney, R. K., & Bhat, R. S. (2016). QTL mapping for late leaf spot and rust resistance using an improved genetic map and extensive phenotypic data on a recombinant inbred line population in peanut (Arachis hypogaea L.). Euphytica, 209(1), 147–156. https://doi.org/10.1007/s10681-016-1651-0
- Kukanur, S., Savithramma, D. L., Duddagi, S., Vijayabharathi, A., Mallikarjuna, B. P., & Pushpa, H. D. (2014). Validation of SSR markers linked to late leaf spot and rust in Groundnut (Arachis hypogaea L.). Ecology, Environment and Conservation, 20(1), 357–361.
- Leal-Bertioli, S. C. M., José, A. C. V. F., Alves-Freitas, D. M. T., Moretzsohn, M. C., Guimarães, P. M., Nielen, S., Vidigal, B. S., Pereira, R. W., Pike, J., Fávero, A. P., Parniske, M., Varshney, R. K., & Bertioli, D. J. (2009). Identification of candidate genome regions controlling disease resistance in Arachis. BMC Plant Biology, 9(1), 112. https://doi.org/10.1186/1471-2229-9-112
- Liang, Y., Baring, M., Wang, S., & Septiningsih, E. M. (2017). Mapping QTLs for leafspot resistance in peanut using SNP-based next-generation sequencing markers. Plant Breeding and Biotechnology, 5(2), 115–122. https://doi.org/10.9787/PBB.2017.5.2.115
- Mace, E. S., Phong, D. T., Upadhyaya, H. D., Chandra, S., & Crouch, J. H. (2006). SSR analysis of cultivated groundnut (Arachis hypogaea L.) germplasm resistant to rust and late leaf spot diseases. Euphytica, 152(3), 317–330. https://doi.org/10.1007/s10681-006-9218-0
- Mammadov, J., Aggarwal, R., Buyyarapu, R., & Kumpatla, S. (2012). SNP markers and their impact on plant breeding. International Journal of Plant Genomics, 2012, 728398. https://doi.org/10.1155/2012/728398
- Mishra, G. P., Radhakrishnan, T., Kumar, A., Thirumalaisamy, P. P., Kumar, N., Bosamia, T. C., Nawade, B., & Dobaria, J. R. (2015). Advancements in molecular marker development and their applications in the management of biotic stresses in peanuts. Crop Protection, 77, 74–86. https://doi.org/10.1016/j.cropro.2015.07.019
- MoFA. (2019). Catalogue of crop varieties released and registered in Ghana. Ministry of Food and Agriculture.
- Mohammed, K., Afutu, E., Odong, T., Okello, D., Nuwamanya, E., Grigon, O., Rubaihayo, P., & Okori, P. (2018). Assessment of groundnut (Arachis hypogaea L.) genotypes for yield and resistance to late leaf spot and rosette diseases. Journal of Experimental Agriculture International, 21(5), 1–13. https://doi.org/10.9734/JEAI/2018/39912
- Morgil, H., Gercek, Y. C., & Tulum, I. (2020). Single nucleotide polymorphisms (SNPs) in plant genetics and breeding. In M. Çalışkan, O. Erol, & G. C. Öz (Eds.), The recent topics in genetic polymorphisms. IntechOpen. https://doi.org/10.5772/intechopen.91886
- Muhammad, A., & Bdliya, B. (2011). Effects of variety and fungicidal rate on Cercospora leaf spot disease of groundnut in the Sudan Savanna. Nigerian Journal of Basic and Applied Sciences, 19(1), 135–141. https://doi.org/10.4314/njbas.v19i1.69359
- Nevill, D. J. (1981). Components of resistance to Cercospora arachidicola and Cercosporidium personatum in groundnuts (Arachis hypogaea). Annals of Applied Biology, 99, 77–86.
- Oteng-Frimpong, R., Karikari, B., Sie, E. K., Kassim, Y. B., Puozaa, D. K., Rasheed, M. A., Fonceka, D., Okello, D. K., Balota, M., Burow, M., & Ozias-Akins, P. (2022). Multi-locus genome-wide association studies reveal genomic regions and putative candidate genes associated with leaf spot diseases in African groundnut (Arachis hypogaea L.) germplasm. Frontiers in Plant Science, 13, 1076744. PMID: 36684745; PMCID: PMC9849250. https://doi.org/10.3389/fpls.2022.1076744
- Pandey, M. K., Khan, A. W., Singh, V. K., Vishwakarma, M. K., Shasidhar, Y., Kumar, V., Garg, V., Bhat, R. S., Chitikineni, A., Janila, P., Guo, B., & Varshney, R. K. (2017). QTL-seq approach identified genomic regions and diagnostic markers for rust and late leaf spot resistance in groundnut (Arachis hypogaea L.). Plant Biotechnology Journal, 15(8), 927–941. https://doi.org/10.1111/pbi.12686
- Peanut Genome Initiative, PGI. (2017). 2012–2017 Accomplishment report to the U.S. Peanut Industry. October, 2017.
- Shaibu, S. A., Sneller, C., Motagi, B. N., Chepkoech, J., Chepngetich, M., Miko, Z. L., Isa, A. M., Ajeigbe, H. A., & Mohammed, S. G. (2020). Genome-wide detection of SNP markers associated with four physiological traits in groundnut (Arachis hypogaea L.) mini core collection. Agronomy, 10(2), 192. https://doi.org/10.3390/agronomy100201
- Shapiro, S. S., & Wilk, M. B. (1965). An analysis of variance test for normality (complete samples). Biometrika, 52(3-4), 591–611. https://doi.org/10.2307/2333709
- Shirasawa, K., Bhat, R. S., Khedikar, Y. P., Sujay, V., Kolekar, R. M., Yeri, S. B., Sukruth, M., Cholin, S., Asha, B., Pandey, M., Varshney, R. K., & Gowda, M. V. C. (2018). Sequencing analysis of genetic loci for resistance for late leaf spot and rust in peanut (Arachis hypogaea L. (2018). Frontiers in Plant Science, 9, 1727. https://doi.org/10.3389/fpls.2018.01727
- Shirasawa, K., Kuwata, C., Watanabe, M., Fukami, M., Hirakawa, H., & Isobe, S. (2016). Target amplicon sequencing for genotyping genome-wide single nucleotide polymorphisms identified by whole-genome resequencing in peanut. The Plant Genome, 9(3), plantgenome2016.06.0052. https://doi.org/10.3835/plantgenome2016.06.0052
- Shoba, D., Manivannan, N., Vindhiyavarman, P., & Nigam, S. N. (2012). SSR markers associated for late leaf spot disease resistance by bulked segregant analysis in groundnut (Arachis hypogaea L.). Euphytica, 188(2), 265–272. https://doi.org/10.1007/s10681-012-0718-9
- Stalker, H. T. (2017). Utilizing wild species for peanut improvement. Crop Science, 57(3), 1102–1120. https://doi.org/10.2135/cropsci2016.09.0824
- Stalker, H. T., Tallury, S. P., Ozias-Akins, P., Bertioli, D. J., & Bertioli, S. C. L. (2013). The Value of diploid peanut relatives for breeding and genomics. Peanut Science, 40(2), 70–88. https://doi.org/10.3146/PS13-6.1
- Subrahmanyam, P., McDonald, D., Waliyar, F., Reddy, L. J., Nigam, S. N., Gibbons, R. W., Ramanatha, R. V., Singh, A. K., Pande, S., Reddy, P. M., & Subba, R. (1995). Groundnut diseases. Information bulletin no. 47 ICRISAT
- Sujay, V., Gowda, M. V., Pandey, M. K., Bhat, R. S., Khedikar, Y. P., Nadaf, H. L., Gautami, B., Sarvamangala, C., Lingaraju, S., Radhakrishan, T., Knapp, S. J., & Varshney, R. K. (2012). Quantitative trait locus analysis and construction of consensus genetic map for foliar disease resistance based on two recombinant inbred line populations in cultivated groundnut (Arachis hypogaea L.). Molecular Breeding: new Strategies in Plant Improvement, 30(2), 773–788. https://doi.org/10.1007/s11032-011-9661-z
- Sukruth, M., Paratwagh, S. A., Sujay, V., Kumari, V., Gowda, M. V. C., Nadaf, H. L., Motagi, B. N., Lingaraju, S., Pandey, M. K., Varshney, R. K., & Bhat, R. S. (2015). Validation of markers linked to late leaf spot and rust resistance, and selection of superior genotypes among diverse recombinant inbred lines and backcross lines in peanut (Arachis hypogaea L.). Euphytica, 204(2), 343–351. https://doi.org/10.1007/s10681-014-1339-2
- Zhang, H., Chu, Y., Dang, P., Tang, Y., Jiang, T., Clevenger, J. P., Ozias-Akins, P., Holbrook, C., Wang, M. L., Campbell, H., Hagan, A., & Chen, C. (2020). Identification of QTLs for resistance to leaf spots in cultivated peanut (Arachis hypogaea L.) through GWAS analysis. TAG. Theoretical and Applied Genetics. Theoretische Und Angewandte Genetik, 133(7), 2051–2061. https://doi.org/10.1007/s00122-020-03576-2
- Zhou, X., Xia, Y., Ren, X., Chen, Y., Huang, L., Huang, S., Liao, B., Lei, Y., Yan, L., & Jiang, H. (2014). Construction of a SNP-based genetic linkage map in cultivated peanut based on large scale marker development using next-generation double-digest restriction-site-associated DNA sequencing (ddRADseq). BMC Genomics, 15(1), 351. https://doi.org/10.1186/1471-2164-15-351
- Zhou, X., Xia, Y., Liao, J., Liu, K., Li, Q., Dong, Y., Ren, X., Chen, Y., Huang, L., Liao, B., Lei, Y., Yan, L., & Jiang, H. (2016). Quantitative trait locus analysis of late leaf spot resistance and plant-type-related traits in cultivated peanut (Arachis hypogaea L.) under multi-environments. PloS One, 11(11), e0166873. https://doi.org/10.1371/journal.pone.0166873
- Zongo, A., Khera, P., Sawadogo, M., Shasidhar, Y., Sriswathi, M., Vishwakarma, M. K., Sankara, P., Ntare, B. R., Varshney, R. K., Pandey, M. K., & Desmae, H. (2017). SSR markers associated with early leaf spot disease resistance through selective genotyping and single marker analysis in groundnut (Arachis hypogaea L.). Biotechnology Reports, 15, 132–137. https://doi.org/10.1016/j.btre.2017.07.005